Open Access
ARTICLE
MELLT3 protects against cerebral ischemia-reperfusion (I/R) injury through up-regulation of m6A modification
1 Department of Neurosurgery, The First Affiliated Hospital, Zhejiang University School of Medicine, Hangzhou, 310003, China
2 Department of Neurology, Puren Hospital Affiliated to Wuhan University of Science and Technology, Wuhan, 430081, China
3 Cancer Institute of Integrated Traditional Chinese and Western Medicine, Zhejiang Academy of Traditional Chinese Medicine, Tongde Hospital of Zhejiang Province, Hangzhou, 310012, China
4 Department of Animal Experimental Center, The First Affiliated Hospital, Zhejiang University School of Medicine, Hangzhou, 10003, China
5 Department of Anesthesiology, Tongde Hospital of Zhejiang Province, Hangzhou, 310012, China
6 Department of Neurology, Yantian Hospital of Southern University of Science and Technology, Shenzhen, 518000, China
* Corresponding Authors: HONGWEI WANG. Email: ; LIFANG ZHENG. Email:
BIOCELL 2023, 47(3), 619-626. https://doi.org/10.32604/biocell.2023.026016
Received 11 August 2022; Accepted 24 October 2022; Issue published 03 January 2023
Abstract
Ischemic cerebrovascular disease is a leading cause of death globally and is often exacerbated by cerebral ischemic/reperfusion injury (CIRI). The exact mechanisms underlying I/R injury are unclear. In this study, we aimed to determine the role of m6A-modified methylase complex methyltransferase-like 3 (METTL3) in cerebral ischemia-reperfusion (I/R) injury. We found that m6A and METTL3 levels increased in OGD/RX-induced mouse astrocytes-cerebellar (MA-C) and the brain of middle cerebral artery occlusion (MCAO) model mice. METTL3 siRNA treatment reduced OGD-RX-induced MAC cell viability and proliferation, which increased with METTL3 over-expression. Flow cytometry analysis showed that silencing METTL3 significantly enhanced OGD/RX-induced MAC apoptosis, which was significantly reduced with METTL3 up-regulation. In an MCAO model, METTL3 overexpression significantly reduced cerebral infarction area and decreased brain cell apoptosis, indicating that METTL3 OE treatment could ameliorate brain edema and injury. Thus, METTL3 could be used as a target to treat I/R injury.Keywords
Ischemic cerebrovascular disease is a leading cause of death and the most common cause of adult disability worldwide (Yuan et al., 2021). Cerebral ischemia is mainly caused by thrombosis or embolism of blood vessels, insufficient blood perfusion in brain tissue, and hypoxia, which in turn initiates ischemic cerebrovascular disease, and eventually leads to neuronal death and neurological impairment (Lim et al., 2021). The blood vessels in the ischemic area can be recanalized naturally or by iatrogenic recanalization after thrombolysis, anticoagulation, or vasodilation to restore blood perfusion. However, this exacerbates some cell dysfunction and structural damage, resulting in cerebral ischemic/reperfusion injury (CIRI), which reduces the efficacy of treatment and worsens the prognosis in patients with ischemic stroke (Feske, 2021). The development of effective treatment strategies for CIRI is, therefore, of great medical importance.
Epigenetic regulation is an important regulatory mechanism for cell phenotypic plasticity. The N6-methyladenine (m6A) modification is the most abundant type of RNA methylation modification and is involved in regulating the transcription of many RNAs. However, this modification is difficult to detect because of its biological function. Advances in technology have allowed the detection of up-regulated m6A modification, which may provide insights into the functions of this modification (He and He, 2021). Recent studies showed that m6A methylation is catalyzed by the methyltransferases RNA binding motif protein-15, zinc finger CCCH-type containing 13, methyltransferase-like 3 (METTL3), METTL14, Wilms’ tumor 1-associating protein (WTAP), and KIAA1429 (writer), and its removal is mediated by demethylases including FTO and ALKBH5 (eraser) (Pinello et al., 2018; Tuncel and Kalkan, 2019). M6A modification produces rapid, reversible effects on gene expression and regulates cell phenotype transformation under external stimuli. Silencing of WTAP could protect myocardial ischemia-reperfusion (I/R) injury by modification of ATF4 mRNA through m6A regulation (Wang et al., 2021). Silencing METTL3 could attenuate renal injury by targeting TAB3 m6A modifications and insulin-like growth factor 2 mRNA binding protein 2 (IGF2BP2) expression (Zhao et al., 2022). M6A modification is associated with various neurobiological processes, including learning and memory neurogenesis, synaptic plasticity, and axonal growth (Li et al., 2017). Furthermore, the m6A level in brain tissue changes significantly during I/R injury (Xu et al., 2020a; Yi et al., 2021). Up-regulation of ALKBH5 or FTO could alleviate I/R-induced neuronal injury (Xu et al., 2020a). YT521-B homology domain-containing family (YTHDF1) plays a crucial effect in I/R injury, and m6A modification is important for RNA stability (Zheng et al., 2020). Although the number of studies on neurological disorders and m6A modification has gradually increased, there has been only a little research on the role of m6A in CIRI (Diao et al., 2020). Moreover, the function of METTL3 in CIRI remains unclear.
In this study, we constructed a middle cerebral artery occlusion and reperfusion (MACO/R) model in vivo and an oxygen-glucose deprivation-reoxygenation (OGD/RX) model in vitro to elucidate the effect of METTL3 on cerebral I/R injury and the underlying molecular mechanisms. These findings could provide a basis for the development of novel METTL3 m6A modification-based therapeutics for ischemic stroke.
MA-C (Mouse Astrocytes-cerebellar) were prepared from a post-natal day (PND) 7 cerebellum in C57BL/6J mice using a previously described protocol (Iwata-Ichikawa et al., 1999). All protocols followed in this study are described in our previous work (Jin et al., 2020).
Establishment of the oxygen-glucose deprivation/reoxygenation (OGD/RX) model
The primary astrocyte OGD model was established as previously described (Lin et al., 2016). Briefly, the MA-C cells were cultured in Dulbecco’s modified Eagle medium (DMEM) without glucose for 6 h under hypoxic conditions (95% N2 and 5% O2). For reperfusion, the cells were incubated with high glucose DMEM medium under normoxic conditions for 12 or 24 h.
Cell viability and proliferation
MA-C cells were seeded at a density of 2 × 104 cells/well into 96-well plates and cultured for 14 days. The OGD/RX model was established as described. CCK8 was added (10 μL/well) to the wells and incubated for 2 h. The absorbance at 450 nm was then measured. Cell proliferation was determined using a Click-iT EdU Imaging Kit (Invitrogen) according to the manufacturer’s instructions.
The METTL3 siRNA, plasmid, and NC control were synthesized by GenePharma (Shanghai, China). Astrocyte MA-C cells were transfected using Lipofectamine 2000 (Invitrogen) according to the manufacturer’s instructions.
The oligonucleotide sequences used were as follows:
METTL3 siRNA-1:
5′-CUGCAAGUAUGUUCACUAUGA-3′
5′-UCAUAGUGAACAUACUUGCAG-3′
METTL3 siRNA-2:
5′-CGUCAGUAUCUUGGGCAAGUU-3′
5′-AACUUGCCCAAGAUACUGACG-3′
Briefly, cells from the different treatment groups were collected and lysed using RIPA lysis buffer. Protein concentration was determined for each sample using the BCA protein assay kit (Sigma). Next, a certain amount of protein was separated by 10% sodium dodecyl sulfate-polyacrylamide gel electrophoresis and transferred to a polyvinylidene fluoride membrane. The membranes were blocked with a sealing agent for 2 h, and incubated overnight with a primary antibody (anti-METTL3; Abcam). The membranes were then washed and incubated with the corresponding secondary antibodies for 2 h, and developed using enhanced chemiluminescence reagents. Protein expression was quantified by evaluation of the protein bands.
Reverse transcriptase-quantitative polymerase chain reaction (RT-qPCR)
Total RNA was extracted using TRIzol (Invitrogen) and reverse transcribed to cDNA using Takara RT reagent (Takara). Relative quantitative PCR was performed using a SYBR PrimeScript mRNA RT-PCR Kit (TaKaRa) on an Applied Biosystems Real-time PCR System. The comparative threshold cycle (2−∆∆Ct) method was used for relative quantification. All reactions were performed in triplicate. The following primers were used:
METTL3-Mus-FP: 5′-GAAACAGCTGGACTCGCTTC-3′
METTL3-Mus-RP: 5′-GGCACGGGACTATCACTACG-3′
FTO-Mus-FP: 5′-GACACTTGGCTTCCTTACCTG-3′
FTO-Mus-RP: 5′-CTCACCACGTCCCGAAACAA-3′
METTL14-Mus FP: 5′-GCTAAGTCAAACACTCCTCCCA-3′
METTL14-Mus RP: 5′-TATTCTTCCAGAGGGGGCTC-3′
YTHDF2-Mus FP: 5′-GAGCAGAGACCAAAAGGTCAAG-3′
YTHDF2-Mus RP: 5′-CTGTGGGCTCAAGTAAGGTTC-3′
ALKBH5-Mus FP: 5′-CGCGGTCATCAACGACTACC-3′
ALKBH5-Mus RP: 5′-ATGGGCTTGAACTGGAACTTG-3′
Cell apoptosis was examined by flow cytometry analysis. The cells were stained with an Annexin V/fluorescein isothiocyanate kit (BD Biosciences) according to the manufacturer’s instructions.
Establishment of the Focal Cerebral Ischemia-Reperfusion Injury Model
We used MCAO to establish a CIRI model as described in our previous publication (Jin et al., 2020). A total of 24 C57BL/6 J mice (6 w, male: 20–25 g) were randomly grouped (n = 6) as follows: the Blank group, MCAO/R, MCAO/R+Vector, MCAO/R+METTL3 OE. For the transfection of METTL3 OE, three plasmid components of the lentiviral vector system (envelope plasmid pHelper2.0, envelope structure plasmid pHelper1.0, and target gene recombinant plasmid PLVX-MeTTL3-IRES-Zsgreen1; 2:3:4) were transfected into 293T cells with liposomes for packaging and later transfected to the brain. The general operation process was as follows: after the mice were anesthetized using 4% chloral hydrate, we inserted a 6–0 silicon-coated nylon monofilament into the left common carotid artery to occlude the origin of the MCA and removed the suture after 1 h. The mice were then anesthetized and decapitated to obtain the brains.
2,3,5-Triphenyltetrazolium chloride (TTC) staining
TTC staining was performed to calculate the infarct volume. The brain sections were placed in 2% TTC and incubated at 37°C for 30 min in the dark. The sections were then fixed in 10% paraformaldehyde. Infarcted areas appeared white and non-infarcted areas appeared red. The infarct size was calculated as follows: infarct size = (infarcted area/total brain area) × 100%.
Neurological deficit score assessment
After MCAO, Neurological deficit scores (Bederson scores) were determined at 24 h according to the Longa 5 score criteria (Longa et al., 1989). The grade levels of neurological deficits were described in our previous work (Jin et al., 2020).
Hematoxylin and eosin (HE) staining
The paraffin-embedded sections were deparaffinized and hydrated in an ethanol gradient. The slide was then hematoxylin stained for 5 min and subsequently stained with eosin staining solution for 2 min before observation under a light microscope (Olympus).
The prepared paraffin sections were deparaffinized by water, blocked with 5% normal goat serum for 20 min, and allowed to react with METTL3 antibody (1:500; Abcam) at 4°C overnight. The sections were then incubated with HRP-lgG for 30 min, and DAB was added to interact for 10 min. After counterstaining with hematoxylin, the sections were dehydrated and observed under a light microscope.
The experimental data were analyzed using GraphPad Prism 8.0 software and expressed as the mean ± SD. We used Student’s t-test or one-way ANOVA for comparisons of differences between groups. P < 0.05 was considered statistically significant.
Methyltransferase-like 3 is down-regulated in middle cerebral artery occlusion model
Altered cerebral m6A modifications can be a potential therapeutic target in cerebral I/R injury (Yi et al., 2021). We established an MCAO model and determined the changes in m6A levels in brain tissue at different time points (24, 48 h) and in the mock group after reperfusion. After MACO, the m6A levels were significantly down-regulated for 24 and 48 h, compared to that in the mock group, indicating that cerebral I/R injury was associated with m6A (Fig. 1A). We also studied m6A-related methylase (ALKBH5, FTO, METTL3, METTL14, and YTHDF2) expression after MCAO and found that METTL3 mRNA was the most significantly down-regulated after 24 or 48 h of MCAO (Fig. 1B). Therefore, we investigated whether METTL3 was regulated in brain injury and found that METTL3 protein and mRNA was reduced after 12 or 24 h OGD/RX (Figs. 2A–2B). A schematic of RNA m6A methylation is shown in Fig. 1C.
Figure 1: Methyltransferase-like 3 (METTL3) is down-regulated in middle cerebral artery occlusion (MCAO). (A) Total m6A RNA after MCAO (24 or 48 h) was determined using reverse transcription-quantitative polymerase chain reaction (RT-qPCR). **P < 0.01, ***P < 0.001. (B) The expression levels of related methyltransferases were detected after MCAO (24 or 48 h) by RT-qPCR. *P < 0.05, **P < 0.01, ***P < 0.001. (C) Schematic representation of RNA m6A methylation.
Figure 2: Level of methyltransferase-like 3 (METTL3) under oxygen-glucose deprivation-reoxygenation (OGD/RX) conditions. (A–B) METTL3 expression was determined with or without OGD/RX (12 or 48 h) treatment by western blot or reverse transcription-quantitative polymerase chain reaction. **P < 0.01, ***P < 0.001.
METTL3 protects against OGD-RX-induced MAC astrocyte injury
To elucidate the protective role of MELLT3 on OGD/RX-induced injury, we pre-treated MAC cells with METTL3 siRNA or plasmid and observed the changes in cell viability, proliferation, and apoptosis under different treatments. In this study, we used these two siRNAs mixed together. We then found that METTL3 siRNA down-regulated METTL3 expression and METTL3 plasmid up-regulated it, which confirmed effective transfection (Fig. 3A). After transfection with MATTL3 siRNA or plasmid, the m6A expression was significantly down-regulated or up-regulated, respectively (Fig. 3B). CCK-8 and EdU detection and flow cytometry showed that cell proliferation was significantly reduced and cell damage aggravated after METTL3 knockdown, while the number of proliferation cells increased significantly and the damage significantly alleviated after transfection with METTL3 plasmid (Figs. 3C–3E). These data suggest that METTL3 is involved in MAC cell damage under OGD/RX conditions.
Figure 3: Methyltransferase-like 3 (METTL3) has protective effects on oxygen-glucose deprivation-reoxygenation (OGD-RX)-induced middle cerebral artery (MAC) astrocyte injury. (A) Reverse transcription-quantitative polymerase chain reaction was performed to determine the level of METTL3 and m6A after transfection with METTL3 siRNA or plasmid. ***P < 0.001. (B–C) Cell viability and proliferation were detected after transfection with METTL3 siRNA or plasmid in OGD/RX by using CCK-8 assay or EdU analysis. **P < 0.01, ***P < 0.001. (D–E) Flow cytometric detection of cell apoptosis after transfection with METTL3 siRNA or plasmid in OGD/RX. **P < 0.01, ***P < 0.001.
Over-expression of METTL3 alleviates MCAO-induced cerebral injury
To verify the protective effect of METTL3 in cerebral infarction injury, we transfected mice with the METTL3 plasmid and subsequently established the MCAO model. We determined the cerebral infarction volume using TTC, and our data showed that the infarct injury size was significantly increased in the MCAO/R group. Over-expression of METTL3 significantly reduced the infarction volume under MCAO/R conditions (Figs. 4A and 4B) and could improve the neurological behavior (Bederson score) in mice (Fig. 4C). In brain tissue, we observed the up-regulation of METTL3 mRNA after treatment with the METTL3 plasmid in mice under MCAO/R condition (Fig. 4D). HE staining showed significantly reduced cerebral edema after METTL3 over-expression (Fig. 5A). Immunohistochemistry showed that compared with the MACO group, METTL3 levels increased following transfection with MELLT3 plasmid in MCAO (Figs. 5B and 5C).
Figure 4: Over-expression of methyltransferase-like 3 (METTL3) reduced infarction volume in middle cerebral artery occlusion (MCAO). (A–B) TTC was used to determine the infarction volume after transfection with METTL3 plasmid in MACO. **P < 0.01, ***P < 0.001. (C) Bederson scores for a mouse behavior. **P < 0.01, ***P < 0.001. (D) RT-qPCR analysis to examine METTL3 mRNA expression in MCAO after transfection with METTL3 plasmid. ***P < 0.001.
Figure 5: Over-expression of methyltransferase-like 3 (METTL3) alleviated middle cerebral artery occlusion (MCAO)-induced cerebral injury. (A) HE staining showing the degree of cerebral edema. (B–C) METTL3 expression in different groups was determined using immunohistochemistry analysis. ***P < 0.001, ###P < 0.001.
Cerebral ischemia is a cerebrovascular disease characterized by reduced cerebral blood flow and accompanied by pathological changes in the brain (Herpich and Rincon, 2020; Neag et al., 2022). The incidence of cerebral ischemia has increased significantly and adversely affects human health worldwide (Paul and Candelario-Jalil, 2021; Vongsfak et al., 2021). Tissue damage and dysfunction caused by reperfusion of ischemic zone blood flow after ischemia is referred to as brain I/R injury, which is one of the complications of cerebral ischemia.
In mammals, m6A modifications are most frequently observed in the brain and regulate synaptic plasticity, axonal growth, learning and memory, and stress responses. M6A modification in RNA has been linked to the pathophysiological processes of many diseases, such as I/R injury of the myocardium, brain, and kidney (Xu et al., 2020a; Zhang et al., 2021; Zhao et al., 2022; Zhuang et al., 2019). FTO up-regulation reduced OGD/R-induced neuronal damage (Xu et al., 2020a). Inhibition of WTAP alleviates myocardial I/R injury by suppressing ATF4 expression (Lu et al., 2021). Knockdown of METTL14 protects against kidney I/R injury in vitro and in vivo by suppressing YAP1 expression (Xu et al., 2020b). METTL3 is the primary factor related to aberrant m6A modification and plays a vital role in renal and cardiac I/R injury (Li et al., 2021; Meng et al., 2020). Suppression of METTL3 attenuates renal injury by TAB3/IGF2BP2 or forkhead box D1 methylation (Meng et al., 2020; Wang et al., 2022). METTL3 could protect against I/R-induced myocardial injury by regulation of autophagy and m6A levels (Song et al., 2019). Furthermore, METTL3-mediated m6A modification promotes the formation of stress granules at the early stages of brain I/R injury (Si et al., 2020). Our results also showed that m6A was down-regulated after MCAO or OGD/RX. Further, we also observed m6A-related methylase levels (Fig. 1) and found METTL3 was the most downregulated in the MCAO mice cerebral cortex and was also reduced in OGD/RX-induced MAC cells (Fig. 2). The experimental results showed contrasting effects of METTL3 and TUG1 levels in the brain and kidneys. TUG1 was upregulated in middle cerebral artery occlusion/reperfusion (MCAO/R) mice; however, it was decreased in renal I/R-induced mice (Chen et al., 2021; He et al., 2022). These inconsistencies are likely because of the differences in expression in different tissues. In the future, we will detect target gene expression in many different tissues. A large body of evidence indicates that the process of I/R is involved in the proliferation and apoptosis of astrocytes. METTL3 also regulates cell proliferation and apoptosis (Gao et al., 2020). Our results demonstrated that transfection with METTL3 plasmid enhanced cell viability and reduced apoptosis in OGD/R-treated MAC cells, and knockdown of METTL3 had the opposite effect. These data indicated that changes in METTL3 could modulate neuroprotection (Fig. 3). We also illustrated the protective effects of METTL3 under MACO/R conditions. Interestingly, compared to the sham group, an increase in METTL3 could reduce cerebral infarction volume (Fig. 4). Furthermore, in the control group, the nuclear membrane was clear, and the nucleolus was obvious. In the model group (MCAO/R), the arrangement of nerve cells was disordered, and the number of neurons was significantly reduced; the space around the cells was enlarged, and empty gaps of different sizes were seen; the nuclei were hyperchromatic. In the treatment group (METTL3 OE+MACO/R), cell edema was reduced significantly, and no other lesions were observed. Thus, METTL3 up-regulation could protect against cerebral I/R injury.
Our data showed that m6A is down-regulated in the MCAO and OGD/RX models. Up-regulation of METTL3 reduces m6A levels and ameliorates I/R injury in vivo, enhances MAC cell viability, and reduces cell apoptosis under OGD/RX in vitro. These findings indicate that METTL3 is a potential candidate for the treatment of cerebral I/R injury.
Availability of Data and Materials: The datasets generated during and/or analysed during the current study are available from the corresponding author on reasonable request.
Author Contribution: LZ and HW designed the study; JJ, XW, XZ, and JN performed the experiments; LZ, YC, and HC analyzed the data. LZ wrote the manuscript. LZ and HW reviewed and edited the manuscript. All authors have read and approved the final manuscript.
Ethics Approval: All animal experiments were approved by the First Affiliated Zhejiang Hospital, Zhejiang University of Medical Ethics Committee (No. 2022-1564) and the Medical Faculty Ethics Committee of the First Affiliated Zhejiang Hospital, Zhejiang University in accordance with the National Institutes of Health Guide for Care and Use of Laboratory Animals (NIH Publications, No. 8023, revised 1978).
Funding Statement: This work was supported by the Natural Science Foundation of Guangdong Province (Grant No. 2020A151501287), the General Project of Science and Technology Innovation Commission of Shenzhen (Grant Nos. JCYJ20210324134800001, JCYJ20190808103401655), Basic Public Welfare Research Project of Zhejiang Province (Grant No. LGF21H090011); and the National Natural Science Foundation of China (Grant No. 82174132).
Conflicts of Interest: The authors declare that they have no conflicts of interest to report regarding the present study.
References
Chen L, Xu JY, Tan HB (2021). LncRNA TUG1 regulates the development of ischemia-reperfusion mediated acute kidney injury through miR-494-3p/E-cadherin axis. Journal of Inflammation 18: 12. DOI 10.1186/s12950-021-00278-4. [Google Scholar] [CrossRef]
Diao MY, Zhu Y, Yang J, Xi SS, Wen X, Gu Q, Hu W (2020). Hypothermia protects neurons against ischemia/reperfusion-induced pyroptosis via m6A-mediated activation of PTEN and the PI3K/Akt/GSK-3β signaling pathway. Brain Research Bulletin 159: 25–31. DOI 10.1016/j.brainresbull.2020.03.011. [Google Scholar] [CrossRef]
Feske SK (2021). Ischemic stroke. American Journal of Medicine 134: 1457–1464. DOI 10.1016/j.amjmed.2021.07.027. [Google Scholar] [CrossRef]
Gao L, Li X, Nie X, Guo Q, Liu Q, Qi Y, Liu J, Lin B (2020). Construction of novel mRNA-miRNA-lncRNA regulatory networks associated with prognosis of ovarian cancer. Journal of Cancer 11: 7057–7072. DOI 10.7150/jca.49557. [Google Scholar] [CrossRef]
He PC, He C (2021). m6A RNA methylation: From mechanisms to therapeutic potential. EMBO Journal 40: e105977. DOI 10.15252/embj.2020105977. [Google Scholar] [CrossRef]
He Z, Zhao Y, Zhu Y, Wang W, Liu X, Lu F (2022). Interfering TUG1 attenuates cerebrovascular endothelial apoptosis and inflammatory injury after cerebral ischemia/reperfusion via TUG1/miR-410/FOXO3 ceRNA axis. Neurotoxicity Research 40: 1–13. DOI 10.1007/s12640-021-00446-7. [Google Scholar] [CrossRef]
Herpich F, Rincon F (2020). Management of acute ischemic stroke. Critical Care Medicine 48: 1654–1663. DOI 10.1097/CCM.0000000000004597. [Google Scholar] [CrossRef]
Iwata-Ichikawa E, Kondo Y, Miyazaki I, Asanuma M, Ogawa N (1999). Glial cells protect neurons against oxidative stress via transcriptional up-regulation of the glutathione synthesis. Journal of Neurochemistry 72: 2334–2344. DOI 10.1046/j.1471-4159.1999.0722334.x. [Google Scholar] [CrossRef]
Jin J, Wang H, Zheng X, Xie S, Zheng L, Zhan R (2020). Inhibition of LncRNA MALAT1 attenuates cerebral ischemic reperfusion injury via regulating AQP4 expression. European Neurology 83: 581–590. DOI 10.1159/000511238. [Google Scholar] [CrossRef]
Li B, Cao Y, Sun M, Feng H (2021). Expression, regulation, and function of exosome-derived miRNAs in cancer progression and therapy. FASEB Journal 35: e21916. DOI 10.1096/fj.202100294RR. [Google Scholar] [CrossRef]
Li L, Zang L, Zhang F, Chen J, Shen H et al. (2017). Fat mass and obesity-associated (FTO) protein regulates adult neurogenesis. Human Molecular Genetics 26: 2398–2411. DOI 10.1093/hmg/ddx128. [Google Scholar] [CrossRef]
Lim S, Kim TJ, Kim YJ, Kim C, Ko SB, Kim BS (2021). Senolytic therapy for cerebral ischemia-reperfusion injury. International Journal of Molecular Sciences 22: 11967. DOI 10.3390/ijms222111967. [Google Scholar] [CrossRef]
Lin SP, Ye S, Long Y, Fan Y, Mao HF, Chen MT, Ma QJ (2016). Circular RNA expression alterations are involved in OGD/R-induced neuron injury. Biochemical and Biophysical Research Communications 471: 52–56. DOI 10.1016/j.bbrc.2016.01.183. [Google Scholar] [CrossRef]
Longa EZ, Weinstein PR, Carlson S, Cummins R (1989). Reversible middle cerebral artery occlusion without craniectomy in rats. Stroke 20: 84–91. DOI 10.1161/01.STR.20.1.84. [Google Scholar] [CrossRef]
Lu Y, Wang YL, Liu Q, Zhou P, Mei PY, Li JS, Zheng ZK (2021). MiRNA-122 promotes ischemia-reperfusion injury after lung transplantation via the toll-like receptor signaling pathway. Current Medical Science 41: 1231–1238. DOI 10.1007/s11596-021-2487-y. [Google Scholar] [CrossRef]
Meng F, Liu Y, Chen Q, Ma Q, Gu S, Cui R, Cao R, Zhao M (2020). METTL3 contributes to renal ischemia-reperfusion injury by regulating Foxd1 methylation. American Journal of Physiology Renal Physiology 319: F839–F847. DOI 10.1152/ajprenal.00222.2020. [Google Scholar] [CrossRef]
Neag MA, Mitre AO, Burlacu CC, Inceu AI, Mihu C, Melincovici CS, Bichescu M, Buzoianu AD (2022). miRNA involvement in cerebral ischemia-reperfusion injury. Frontiers in Cellular Neuroscience 16: 901360. DOI 10.3389/fnins.2022.901360. [Google Scholar] [CrossRef]
Paul S, Candelario-Jalil E (2021). Emerging neuroprotective strategies for the treatment of ischemic stroke: An overview of clinical and preclinical studies. Experimental Neurology 335: 113518. DOI 10.1016/j.expneurol.2020.113518. [Google Scholar] [CrossRef]
Pinello N, Sun S, Wong JJ (2018). Aberrant expression of enzymes regulating m6A mRNA methylation: Implication in cancer. Cancer Biology Medicine 15: 323–334. DOI 10.20892/j.issn.2095-3941.2018.0365. [Google Scholar] [CrossRef]
Si W, Li Y, Ye S, Li Z, Liu Y, Kuang W, Chen D, Zhu M (2020). Methyltransferase 3 mediated miRNA m6A methylation promotes stress granule formation in the early stage of acute ischemic stroke. Frontiers in Molecular Neuroscience 13: 103. DOI 10.3389/fnmol.2020.00103. [Google Scholar] [CrossRef]
Song H, Feng X, Zhang H, Luo Y, Huang J et al. (2019). METTL3 and ALKBH5 oppositely regulate m6A modification of TFEB mRNA, which dictates the fate of hypoxia/reoxygenation-treated cardiomyocytes. Autophagy 15: 1419–1437. DOI 10.1080/15548627.2019.1586246. [Google Scholar] [CrossRef]
Tuncel G, Kalkan R (2019). Importance of m N6-methyladenosine m6A RNA modification in cancer. Medical Oncology 36: 36. DOI 10.1007/s12032-019-1260-6. [Google Scholar] [CrossRef]
Vongsfak J, Pratchayasakul W, Apaijai N, Vaniyapong T, Chattipakorn N, Chattipakorn SC (2021). The alterations in mitochondrial dynamics following cerebral ischemia/reperfusion injury. Antioxidants 10: 1384. DOI 10.3390/antiox10091384. [Google Scholar] [CrossRef]
Wang J, Zhang J, Ma Y, Zeng Y, Lu C et al. (2021). WTAP promotes myocardial ischemia/reperfusion injury by increasing endoplasmic reticulum stress via regulating m6A modification of ATF4 mRNA. Aging 13: 11135–11149. DOI 10.18632/aging.202770. [Google Scholar] [CrossRef]
Wang JN, Wang F, Ke J, Li Z, Xu CH et al. (2022). Inhibition of METTL3 attenuates renal injury and inflammation by alleviating TAB3 m6A modifications via IGF2BP2-dependent mechanisms. Science Translational Medicine 14: eabk2709. DOI 10.1126/scitranslmed.abk2709. [Google Scholar] [CrossRef]
Xu K, Mo Y, Li D, Yu Q, Wang L et al. (2020a). N6-methyladenosine demethylases Alkbh5/Fto regulate cerebral ischemia-reperfusion injury. Therapeutic Advances in Chronic Disease 11: 2040622320916024. DOI 10.1177/2040622320916024. [Google Scholar] [CrossRef]
Xu Y, Yuan XD, Wu JJ, Chen RY, Xia L, Zhang M, Han CH, Mou S (2020b). The N6-methyladenosine mRNA methylase METTL14 promotes renal ischemic reperfusion injury via suppressing YAP1. Journal of Cellular Biochemistry 121: 524–533. DOI 10.1002/jcb.29258. [Google Scholar] [CrossRef]
Yi D, Wang Q, Zhao Y, Song Y, You H, Wang J, Liu R, Shi Z, Chen X, Luo Q (2021). Alteration of N6-Methyladenosine mRNA methylation in a rat model of cerebral ischemia-reperfusion injury. Frontiers in Cellular Neuroscience 15: 605654. DOI 10.3389/fnins.2021.605654. [Google Scholar] [CrossRef]
Yuan Q, Yuan Y, Zheng Y, Sheng R, Liu L, Xie F, Tan J (2021). Anti-cerebral ischemia reperfusion injury of polysaccharides: A review of the mechanisms. Biomedicine Pharmacotherapy 137: 111303. DOI 10.1016/j.biopha.2021.111303. [Google Scholar] [CrossRef]
Zhang J, Luo CJ, Xiong XQ, Li J, Tang SH, Sun L, Su Q (2021). MiR-21-5p-expressing bone marrow mesenchymal stem cells alleviate myocardial ischemia/reperfusion injury by regulating the circRNA_0031672/miR-21-5p/programmed cell death protein 4 pathway. Journal of Geriatric Cardiology 18: 1029–1043. DOI 10.11909/j.issn.1671-5411.2021.12.004. [Google Scholar] [CrossRef]
Zhao L, Liang X, Wang L, Zhang X (2022). The role of miRNA in ovarian cancer: An overview. Reproductive Sciences 29: 2760–2767. DOI 10.1007/s43032-021-00717-w. [Google Scholar] [CrossRef]
Zheng L, Tang X, Lu M, Sun S, Xie S, Cai J, Zan J (2020). microRNA-421-3p prevents inflammatory response in cerebral ischemia/reperfusion injury through targeting m6A Reader YTHDF1 to inhibit p65 mRNA translation. International Immunopharmacology 88: 106937. DOI 10.1016/j.intimp.2020.106937. [Google Scholar] [CrossRef]
Zhuang C, Zhuang C, Luo X, Huang X, Yao L et al. (2019). N6-methyladenosine demethylase FTO suppresses clear cell renal cell carcinoma through a novel FTO-PGC-1alpha signalling axis. Journal of Cellular and Molecular Medicine 23: 2163–2173. DOI 10.1111/jcmm.14128. [Google Scholar] [CrossRef]
Cite This Article
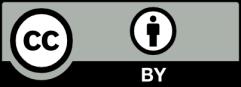