Open Access
EDITORIAL
Sustainable Development of Energy Systems and Climate Systems: Key Issues and Perspectives
1 Center for Sustainable Development and Energy Policy Research (SDEP), School of Energy and Mining Engineering, China University of Mining and Technology (Beijing), Beijing, 100083, China
2 Beijing Key Laboratory for Precise Mining of Intergrown Energy and Resources, China University of Mining and Technology (Beijing), Beijing, 100083, China
* Corresponding Author: Bing Wang. Email:
Energy Engineering 2023, 120(8), 1763-1773. https://doi.org/10.32604/ee.2023.027846
Received 17 November 2022; Accepted 28 February 2023; Issue published 05 June 2023
Abstract
Climate change and energy security issues are prominent challenges in current energy system management, which should be governed synergistically due to the feedback relationships between them. The “Energy Systems Management and Climate Change” Special Collection Issue in the journal of Energy Engineering provide insights into the field of energy systems management and climate change. From an extended perspective, this study discusses the key issues, research methods and models for energy system management and climate change research. Comprehensive and accurate prediction of energy supply and demand, the evaluation on the energy system resilience to climate change and the coupling methodology application of both nature and social science field maybe the frontier topics around achieving sustainable development goals of energy systems.Graphical Abstract
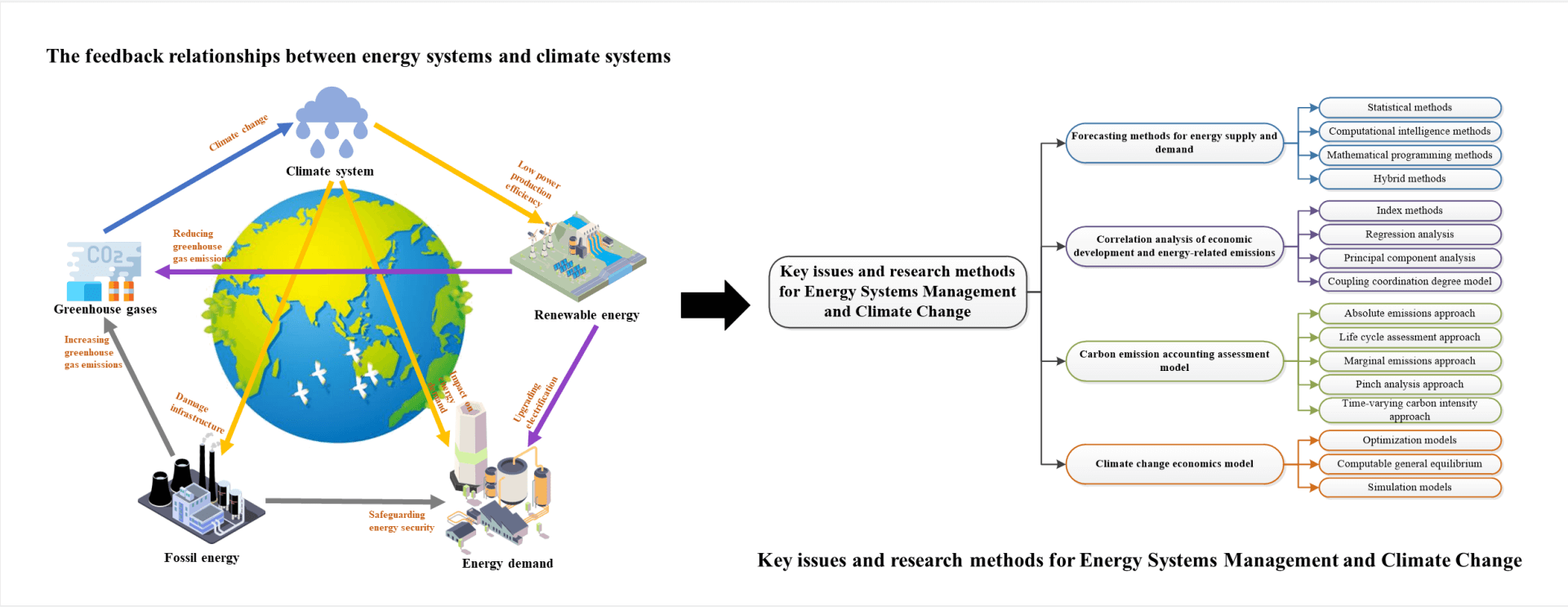
Keywords
Climate change and energy security concerns are prominent challenges for energy systems management currently, and they have a close but complex relationship with each other [1]. On the one hand, energy systems management is capable to cope with climate change by reducing greenhouse gas emissions in a significant way. Energy production and consumption, mainly fossil energy aspects, has produced massive greenhouse gases (GHG), which is central to mitigate climate change. According to the statistics from the International Energy Agency, the global CO2 emissions from energy combustion and industrial activities totaled 36.3 Gt, accounting for 89% of all emissions worldwide in 2021 [2]. On the other hand, variable weather patterns and extreme weather events can have a negative impact on energy supply, demand, and transportation. For example, the climatic sensitivity of biomass, hydropower, solar and wind power would lower the power production efficiency [3,4]. Climate change has also influenced energy demand through the changes in heating and cooling demand [5]. In general, the twin linkages between managing energy systems and combating climate change should be taken seriously.
Since there is a feedback relationship between energy systems management and climate change, the synergistic governance of both systems should be improved. The study takes a starting point in work presented in the special issue “Energy Systems Management and Climate Change”, published in the Energy Engineering and puts this work into a broader context. It is expected to review the deficiencies of the research status and discuss the future research perspectives. Firstly, this study comprehensively reviews the current researches on energy systems management measurements for coping with climate change and mitigation practices for the impacts of climate change on energy systems. Secondly, research methods and models for key issues, such as carbon emission accounting, energy supply and demand forecasting, and climate change economics research are also discussed. Finally, the future perspectives are presented towards innovative energy systems management under the goals of carbon peaking and carbon neutrality.
2 Key Issues for Energy Systems Management and Climate Change Research
2.1 Energy Systems Management for Coping with Climate Change
Energy efficiency improvement, energy structure optimization, carbon sequestration and energy-related services innovation are the promising perspectives for energy systems management practice. Firstly, the improvement of energy efficiency can be carried out in fossil energy development, thermal power generation, industry, construction and electricity demand side. Secondly, the energy structure can be optimized by increasing the amount of zero-carbon energy and expanding the electrification level in various industries, such as industry, transport, manufacturing and agriculture. Thirdly, technical and ecological carbon sequestration are available to reduce carbon emissions. Finally, energy audit, energy monitoring and carbon emission accounting can be categorized as energy-related (ER) services. The conceptual framework for energy systems management to address climate change is shown in Fig. 1.
Figure 1: Conceptual framework for energy systems management to address climate change
2.1.1 Energy Efficiency Improvement
Energy efficiency improvement, as a prospective pathway in the short and middle term, is significant for reducing GHG emissions by minimizing energy losses on both the supply and demand sides. Energy production efficiency can be promoted by in-situ fluidized mining of deep underground coal resources, CO2 enhanced oil recovery, ultra-supercritical power generation, and combined heat and power techniques [6,7]. Industrial process reengineering, green building retrofitting and demand response programming are the feasible measures for improving energy end-use efficiency [8]. The green exploration of fossil energy and the deep decarbonization of end-use sectors have become the life-cycle research hotspots by scholars.
2.1.2 Energy Structure Adjustment
Energy structure adjustment can be achieved by promoting electricity substitution in the consumption sectors and increasing the proportion of zero-carbon energy sources. Numerous countries have launched renewable energy development plans to achieve the goals of carbon emissions reduction [9]. In China, the Ministry of Water Resources has proposed to promote the green development of small hydropower and emphasized that the small hydroelectric power generation with green certification will be completely purchased by the grid companies [10]. The implementation of solar roof demonstration programs can realize the effective utilization of solar energy by rooftop photovoltaic generation technology [11]. From the perspectives of local governments, power generation enterprises and grid companies, Wang et al. established a conceptual game decision model for the renewable energy industry to deal with the dilemma of insufficient willingness to consume renewable energy [12]. Future requirements for efficient, economical, and complementary energy storage systems will be centralized on the integration of physical and chemical energy storage techniques [13].
Carbon sequestration is divided into technical carbon sequestration and ecological carbon sequestration. The carbon reduction and sequestration technologies refer to storing and reutilizing CO2 emitted from the production process, including carbon capture, utilization, and storage (CCUS), bioenergy with carbon capture and storage (BECCS) and direct air carbon capture and storage (DACCS). Ecological carbon sequestration means that excess carbon is fixed as organic carbon in the plants and soil, such as forest carbon sequestration, grassland carbon sequestration, wetland carbon sequestration and marine carbon sequestration. The current economic status and future development trends of CCUS technology have been studied and sorted out in detail, and the governmental supportive policies will have a decisive effect on the maturity, cost reduction and deployment of CCUS technology [14].
2.1.4 Energy-Related (ER) Services
Energy audit, energy monitoring and carbon emission accounting can be classified as energy-related (ER) services [15]. Energy audit is used to strengthen energy management by evaluating energy consumption for end-use sectors, while energy monitoring can provide enterprises and governments with effective data to reduce energy management costs and improve energy management efficiency. Furthermore, carbon emission accounting and its driver analysis can help the emissions producer to formulate corresponding measures to reduce carbon emissions.
2.2 Alleviating the Impacts of Climate Change on Energy Systems
The vulnerability of the energy system to climate change is mainly reflected in three aspects of energy supply, energy demand and energy transportation. Table 1 shows the vulnerability and adaptive measures of energy systems to climate change.
In terms of energy supply, the impacts of climate change on fossil fuel and nuclear power generation are primarily associated with the generation cycle efficiency and cooling water demand [1]. And the offshore oil and gas production facilities are also vulnerable to extreme events. Compared to fossil energy generation, renewable energy generation is more influenced by climate conditions, such as rainfall, wind speed, river runoff and extreme weather events.
The impact of climate change on energy demand is mainly manifested by changes in the demand for heating and cooling. For example, rising temperatures will reduce heating demand and increase cooling demand. Moreover, the changes in cooling demand are more pronounced and uncertain than heating demand, which has posed the challenge for future energy demand forecasting [16].
For energy transportation, climate change can disrupt the transportation and distribution of electricity, oil, gas and other fuels. Exposed transmission lines and pipelines can be destroyed by hurricanes, snowfall and landslides, which can increase energy costs. The oil and gas pipelines constructed in permafrost areas will be vulnerable to destruction due to melting permafrost in the warming climates. Waterborne energy transport channels may be affected by the falling water levels as a result of reduced precipitation or blocked by excessive precipitation and frozen water [17].
3 Methods and Models for Assessing Sustainability of Energy Systems and Climate Systems
Research methods and models for energy supply and demand projections, correlation analysis between economic development and carbon emissions, carbon emission accounting assessment and climate change economics are efficient and powerful for assessing the sustainability of energy systems and climate systems, as shown in Fig. 2.
Figure 2: Methods and models for assessing sustainability of energy systems and climate systems
3.1 Forecasting Methods for Energy Supply and Demand
The forecasts of energy supply and demand are the theoretical foundation for optimizing the structure of energy production and consumption to cope with climate change, and an important basis for the government to formulate long-term energy development planning. The existing forecasting methods are divided into statistical, computational intelligence (CI), mathematical programming (MP) and hybrid methods [24]. The statistical methods include regression analysis, time series methods, autoregressive conditional heteroscedasticity (ARCH) methods and so on [25]. The computational intelligence methods include machine learning methods, knowledge-based methods, grey prediction and metaheuristic methods. The hybrid method is the combination of different independent methods, such as artificial neural network-autoregressive integrated moving average model (ANN-ARIMA), and cooperative ant colony optimization-genetic algorithm (COR-ACO-GA). Three cases of Iran’s annual demand for electricity, gas and oil are used to validate the COR-ACO-GA model, and the conclusion was drawn that the hybrid model is more accurate than ANN and adaptive neuro-fuzzy inference systems [26]. Research objectives and complexity are the key determinants in the selection of forecasting methods.
3.2 Correlation Analysis of Economic Development and Energy-Related Emissions
The low carbon development of economy and society has been a core issue for the sustainable development of the global energy and climate systems [27,28]. And how to decouple economic development from energy consumption and carbon emissions has been widely discussed by scholars. Liu et al. investigated the effect of greenization on the marginal utility of carbon intensity by using the integrated index method and regression analysis, and concluded that the urbanization level and technological development slowed the reduction in the marginal utility of carbon intensity [29]. Song et al. employed principal component analysis and the coupling coordination degree model to analyze the development level of industrial coordination in the Bohai Rim Economic Circle, and demonstrated the negative correlation between industrial coupling coordination and carbon intensity using the Pearson correlation test [30].
3.3 Carbon Emission Accounting Assessment Model
Carbon emission accounting is the basis for evaluating the current state of carbon emissions and formulating low-carbon development strategies. Numerous factors impact carbon emissions, including energy intensity, economic structure, population, and existing technical conditions. Besides, the specific mitigation measures will also impact how many reductions by GHG. The main methods of carbon emission accounting are the absolute emissions approach, life cycle assessment approach, marginal emissions approach and pinch analysis approach, and time-varying carbon intensity approach [31]. The time-varying carbon intensity approach was employed to examine the temporal and spatial GHG emission intensity of the Chinese power system [32]. Gong et al. employed the expanded Kaya method to account for energy-related carbon emissions from primary, secondary, and tertiary industries in Shandong Province, and put forward suggestions to further optimize the energy structure and improve energy efficiency [33].
3.4 Climate Change Economics Model
Climate change is a complicated collection of political, economic, environmental, as well as ethical issues, rather than the simple scientific issues of past decades. Relevant research must therefore be interdisciplinary and multidimensional. The most widely known model for climate change economics is integrated assessment modeling (IAM). There are many kinds of IAMs, including optimization models, computable general equilibrium (CGE), and simulation models. The optimization models can be divided into welfare maximization models and cost minimization models according to the objective function, whereas the dynamic integrated model of climate and economy (DICE), climate framework for uncertainty, negotiation, and distribution (FUND), regional integrated model of climate and the economy (RICE) belong to the welfare maximization models [34]. And the integrated model to assess the greenhouse effect (IMAGE) and the global climate model (GIM) are included in the simulation models. Moreover, IAMs should be established based on the economic level, carbon emissions, and data availability of different countries and regions.
4 Conclusions and Perspectives
The studies of energy system management and climate change are the future of the energy and climate systems due to increasingly vulnerable energy systems and increasingly harsh climate conditions. With articles published in special issues as a starting point, this study discusses key issues and assessment methods and models in research on energy system management and climate change.
• Considerable investigations on energy system management and climate change have revealed that the studies concentrate on energy systems management measurements for coping with climate change and mitigation practices for the impacts of climate change on energy systems. Energy efficiency improvement, energy structure optimization, carbon sequestration and energy-related services are the promising perspectives for energy systems management practice. And the vulnerability of energy systems towards climate change requires a three-pronged approach to energy supply, energy demand and energy transport.
• Coupling research methods and models of energy, economy and carbon emissions have been the focus of research on energy system management and climate change. The improvement of existing methods and tools helps to overcome the complexity of research in this field and drive the energy system and climate system toward decarbonization and sustainability.
The topic of “Energy Systems Management and Climate Change” has received wide attention by academic journals. Energy Engineering has released a special issue named as “Energy Systems Management and Climate Change” in 2020. The newly built journal Carbon Neutrality also polished its first issue for declaring the target for a net-zero carbon future. With the intensive attention on carbon neutrality, the newly special issue “Innovative Energy Systems Management under the Goals of Carbon Peaking and Carbon Neutrality” requires valuable submissions from researchers around the world.
The researches on energy system management and climate change have established a scientific research framework to connect their complex feedbacks. It is an interdisciplinary task aimed at the comprehensive and accurate prediction of energy supply and demand, the evaluation of the energy system resilience to climate change and the coupling methodology application of both nature and social science field.
• A powerful and adaptive energy supply and demand prediction is essential for modelling the dynamic equilibrium between energy systems and climate change. The field of climate change economics considers the range of numerous decades or several centuries while energy supply and demand prediction will be faithful in a medium and short term. The uncertainties in climate change impacts and the prediction for energy supply and demand require a timely scenario analysis with the dynamicity in energy reserve, economic development and political instability, etc.
• The research on the adaptation of energy systems to climate change is relatively inadequate. Numerous studies have focused on how to cope with climate change through carbon emissions reduction by energy system adjustment. This aspect is of significance in controlling the basic causes of climate change. The impacts of climate change on energy systems, however, should be highly emphasized because of the increasing penetration of climate dependent renewables in the energy mix. Thus, vulnerability assessment is necessary to build a climate-resilient energy system and the fairness and effectiveness of climate change adaptation and mitigation actions should be considered with measurable responsibility.
• The strong characteristic of interdisciplinary requires the coupling applications of various social and nature science methods. Climate change is caused by social transformation and discussed in atmospheric science and biochemistry. The nature, underlying causes or impacts of climate change should be analyzed by integrating multiple methods. The coupling application of methods for energy development planning and climate change integrated assessment models will become the focus of future climate change modelling.
Funding Statement: This research is supported by the Fundamental Research Funds for the Central Universities (2022SKNY01, 2022YJSNY04).
Conflicts of Interest: The authors declare that they have no conflicts of interest to report regarding the present study.
References
1. IPCC (2014). Climate change 2014: Mitigation of climate change. Cambridge, UK and New York, NY, USA: Cambridge University Press. https://doi.org/10.1017/CBO9781107415416 [Google Scholar] [CrossRef]
2. IEA (2022). Global energy review: CO2 emissions in 2021. Paris: International Energy Agency. https://www.iea.org/reports/global-energy-review-co2-emissions-in-2021-2 [Google Scholar]
3. Wang, B., Ke, R. Y., Yuan, X. C., Wei, Y. M. (2014). China's regional assessment of renewable energy vulnerability to climate change. Renewable & Sustainable Energy Reviews, 40(1), 185–195. https://doi.org/10.1016/j.rser.2014.07.154 [Google Scholar] [CrossRef]
4. Wang, B., Liang, X. J., Zhang, H., Wang, L., Wei, Y. M. (2014). Vulnerability of hydropower generation to climate change in China: Results based on grey forecasting model. Energy Policy, 65, 701–707. https://doi.org/10.1016/j.enpol.2013.10.002 [Google Scholar] [CrossRef]
5. Yalew, S. G., van Vliet, M. T. H., Gernaat, D. E. H. J., Ludwig, F., Miara, A. et al. (2020). Impacts of climate change on energy systems in global and regional scenarios. Nature Energy, 5(10), 794–802. https://doi.org/10.1038/s41560-020-0664-z [Google Scholar] [CrossRef]
6. Xie, H., Ju, Y., Ren, S., Gao, F., Liu, J. et al. (2019). Theoretical and technological exploration of deep in situ fluidized coal mining. Frontiers in Energy, 13(4), 603–611. https://doi.org/10.1007/s11708-019-0643-x [Google Scholar] [CrossRef]
7. Mohtaram, S., Sun, H., Lin, J., Chen, W., Sun, Y. (2020). Multi-objective evolutionary optimization & 4E analysis of a bulky combined cycle power plant by CO2/CO/NOx reduction and cost controlling targets. Renewable and Sustainable Energy Reviews, 128, 109898. https://doi.org/10.1016/j.rser.2020.109898 [Google Scholar] [CrossRef]
8. Zuo, J., Zhao, Z. Y. (2014). Green building research-current status and future agenda: A review. Renewable & Sustainable Energy Reviews, 30(1), 271–281. https://doi.org/10.1016/j.rser.2013.10.021 [Google Scholar] [CrossRef]
9. Ridwan, L. I., Ajide, K. B., Usman, M., Kousar, R. (2022). Heterogeneous effects of renewable energy and structural change on environmental pollution in Africa: Do natural resources and environmental technologies reduce pressure on the environment? Renewable Energy, 200(8), 244–256. https://doi.org/10.1016/j.renene.2022.09.134 [Google Scholar] [CrossRef]
10. Shi, J. (2022). Assessment framework of green intelligent transformation of small hydropower in China. Energy Engineering, 119(2), 681–697. https://doi.org/10.32604/ee.2022.014942 [Google Scholar] [CrossRef]
11. Uzair, M., Al-Kafrawi, S., Al-Janadi, K., Al-Bulushi, I. (2022). A low-cost, real-time rooftop IoT-based photovoltaic (PV) system for energy management and home automation. Energy Engineering, 119(1), 83–101. https://doi.org/10.32604/EE.2022.016411 [Google Scholar] [CrossRef]
12. Wang, B., Deng, K., He, L., Sun, Z. (2021). Behaviours of multi-stakeholders under China’s renewable portfolio standards: A game theory-based analysis. Energy Engineering, 118(5), 1333–1351. https://doi.org/10.32604/EE.2021.014258 [Google Scholar] [CrossRef]
13. Mohtaram, S., Wu, W., Aryanfar, Y., Yang, Q., García Alcaraz, J. L. (2022). Introducing and assessment of a new wind and solar-based diversified energy production system intergrading single-effect absorption refrigeration, ORC, and SRC cycles. Renewable Energy, 199, 179–191. https://doi.org/10.1016/j.renene.2022.08.069 [Google Scholar] [CrossRef]
14. Jiang, K., Ashworth, P., Zhang, S., Liang, X., Sun, Y. et al. (2020). China's carbon capture, utilization and storage (CCUS) policy: A critical review. Renewable & Sustainable Energy Reviews, 119(4), 109601. https://doi.org/10.1016/j.rser.2019.109601 [Google Scholar] [CrossRef]
15. Kovalko, O., Eutukhova, T., Novoseltsev, O. (2022). Energy-related services as a business: Eco-transformation logic to support the low-carbon transition. Energy Engineering, 119(1), 103–121. https://doi.org/10.32604/EE.2022.017709 [Google Scholar] [CrossRef]
16. Hieronymus, M., Nycander, J. (2020). Interannual variability of the overturning and energy transport in the atmosphere and ocean during the late twentieth century with implications for precipitation and sea level. Journal of Climate, 33(1), 317–338. https://doi.org/10.1175/JCLI-D-19-0204.1 [Google Scholar] [CrossRef]
17. Chen, Y., Li, M., Cao, J., Cheng, S., Zhang, R. (2021). Effect of climate zone change on energy consumption of office and residential buildings in China. Theoretical and Applied Climatology, 144(1–2), 353–361. https://doi.org/10.1007/s00704-021-03544-w [Google Scholar] [CrossRef]
18. Henry, C. L., Pratson, L. F. (2019). Differentiating the effects of climate change-induced temperature and streamflow changes on the vulnerability of once-through thermoelectric power plants. Environmental Science & Technology, 53(7), 3969–3976. https://doi.org/10.1021/acs.est.8b05718 [Google Scholar] [PubMed] [CrossRef]
19. Liu, B., Lund, J. R., Liu, L., Liao, S., Li, G. et al. (2020). Climate change impacts on hydropower in Yunnan. China. Water, 12(1), 197. https://doi.org/10.3390/w12010197 [Google Scholar] [CrossRef]
20. Rosende, C., Sauma, E., Harrison, G. P. (2019). Effect of climate change on wind speed and its impact on optimal power system expansion planning: The case of Chile. Energy Economics, 80(6), 434–451. https://doi.org/10.1016/j.eneco.2019.01.012 [Google Scholar] [CrossRef]
21. Ding, R., Shi, W. (2022). Contributions of climate change to cereal yields in Tibet, 1993–2017. Journal of Geographical Sciences, 32(1), 101–116. https://doi.org/10.1007/s11442-022-1938-0 [Google Scholar] [CrossRef]
22. Cao, H., Dong, H., Ren, Y., Wang, Y., Li, N. et al. (2022). Bi-level energy management model of grid-connected microgrid community. Energy Engineering, 119(3), 965–984. https://doi.org/10.32604/ee.2022.020051 [Google Scholar] [CrossRef]
23. Zhou, Y., Eom, J., Clarke, L. (2013). The effect of global climate change, population distribution, and climate mitigation on building energy use in the US and China. Climatic Change, 119(3–4), 979–992. https://doi.org/10.1007/s10584-013-0772-x [Google Scholar] [CrossRef]
24. Deroubaix, A., Labuhn, I., Camredon, M., Gaubert, B., Monerie, P. A. et al. (2021). Large uncertainties in trends of energy demand for heating and cooling under climate change. Nature Communications, 12(1). https://doi.org/10.1038/s41467-021-25504-8 [Google Scholar] [PubMed] [CrossRef]
25. Wang, Y., Wu, C. (2012). Forecasting energy market volatility using GARCH models: Can multivariate models beat univariate models? Energy Economics, 34(6), 2167–2181. https://doi.org/10.1016/j.eneco.2012.03.010 [Google Scholar] [CrossRef]
26. Ghanbari, A., Kazemi, S. M. R., Mehmanpazir, F., Nakhostin, M. M. (2013). A cooperative ant colony optimization-genetic algorithm approach for construction of energy demand forecasting knowledge-based expert systems. Knowledge-Based Systems, 39, 194–206. https://doi.org/10.1016/j.knosys.2012.10.017 [Google Scholar] [CrossRef]
27. Usman, M., Balsalobre-Lorente, D., Jahanger, A., Ahmad, P. (2023). Are mercosur economies going green or going away? An empirical investigation of the association between technological innovations, energy use, natural resources and GHG emissions. Gondwana Research, 113(1), 53–70. https://doi.org/10.1016/j.gr.2022.10.018 [Google Scholar] [CrossRef]
28. Makhdum, M. S. A., Usman, M., Kousar, R., Cifuentes-Faura, J., Radulescu, M. et al. (2022). How do institutional quality, natural resources, renewable energy, and financial development reduce ecological footprint without hindering economic growth trajectory? Evidence from China. Sustainability, 14(21), 13910. https://doi.org/10.3390/su142113910 [Google Scholar] [CrossRef]
29. Liu, Y., Jiao, R., Zhao, L., Liu, K. (2021). Impact of greenization on the marginal utility of intensity of carbon emissions and factors affecting it in China. Energy Engineering, 118(2), 363–378. https://doi.org/10.32604/EE.2021.013472 [Google Scholar] [CrossRef]
30. Song, M., Zhang, L., Zhang, M., Li, D., Zhu, Y. (2021). Relationship between industrial coupling coordination and carbon intensity in the Bohai rim economic circle. Energy Engineering, 118(1), 143–161. https://doi.org/10.32604/EE.2020.013834 [Google Scholar] [CrossRef]
31. Kachoee, M. S., Salimi, M., Amidpour, M. (2018). The long-term scenario and greenhouse gas effects cost-benefit analysis of Iran's electricity sector. Energy, 143, 585–596. https://doi.org/10.1016/j.energy.2017.11.049 [Google Scholar] [CrossRef]
32. Khan, I., Jack, M. W., Stephenson, J. (2018). Analysis of greenhouse gas emissions in electricity systems using time-varying carbon intensity. Journal of Cleaner Production, 184(3), 1091–1101. https://doi.org/10.1016/j.jclepro.2018.02.309 [Google Scholar] [CrossRef]
33. Gong, W., Zhu, B., Wang, C., Fan, Z., Zhao, M. et al. (2021). Factor decomposition and regression analysis of the energy related carbon emissions in Shandong, China: A perspective of industrial structure. Energy Engineering, 118(4), 981–994. https://doi.org/10.32604/EE.2021.014554 [Google Scholar] [CrossRef]
34. CIESIN (1995). Thematic guide to integrated assessment modeling of climate change. Center for International Earth Science Information Network. http://sedac.ciesin.columbia.edu/mva/iamcc.tg/TGHP.html [Google Scholar]
Cite This Article
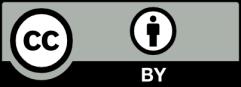