Open Access
ARTICLE
Experimental Investigation on Fracture Performance of Short Basalt Fiber Bundle Reinforced Concrete
1
School of Water Conservancy and Civil Engineering, Zhengzhou University, Zhengzhou, 450001, China
2
Henan Traffic Engineering Technology Co., Ltd., Zhengzhou, 450015, China
3
Key Laboratory of Deep Underground Science and Engineering (Ministry of Education), College of Architecture and Environment,
Sichuan University, Chengdu, 610065, China
4
Henan Provincial Communications Planning & Design Institute Co., Ltd., Zhengzhou, 450015, China
* Corresponding Author: Pu Zhang. Email:
Structural Durability & Health Monitoring 2022, 16(4), 291-305. https://doi.org/10.32604/sdhm.2022.015097
Received 21 November 2020; Accepted 15 December 2020; Issue published 03 January 2023
Abstract
In this paper, a notched three-point bending test is used to study the fracture performance of the short basalt fiber bundle reinforced concrete (SBFBRC). To compare and analyze the enhancement effect of different diameters and different content of basalt fiber bundles on the fracture performance of concrete, some groups are set up, and the P-CMOD curves of each group of specimens are measured, and the fracture toughness and fracture energy of each control group are calculated. The fracture toughness and fracture energy are two important fracture performance parameters to study the effect and law of the new basalt fiber bundles on the fracture performance of concrete. The research results show that the diameter and content of the new basalt fiber bundles have a certain effect on the fracture performance of concrete. With the increase of the content of basalt fiber bundles, the peak load, crack initiation toughness, instability toughness and fracture energy of SBFBRC are greatly improved compared with the benchmark group. When the fiber bundle diameter is 0.2 mm, the peak load increases by 69.5% compared with the reference group. The instability toughness reaches its maximum value at 0.2 mm diameter, which is 59.7% higher than the benchmark.Keywords
Basalt fiber (abbreviated as BF), a hot topic in recent years, is being more and more widely used in engineering for its excellent physical and mechanical properties, such as high elastic modulus and high tensile strength, green environmental protection and low cost. There were abundant studies on the mechanical properties of basalt fiber reinforced concrete both at home and abroad. Mehran et al. [1–9] found that an appropriate amount of basalt fiber can significantly improve the flexural strength of concrete. However, Arslan et al. [10–12] found that a small amount of short-cut basalt fiber did not improve the compressive strength while increasing the content will lead to the decrease of the compressive strength. Kabay et al. [13–15] studied the influence of basalt fiber on the mechanical properties of concrete with 0%~2% fiber volume fraction, and the results showed that the compressive strength of concrete decreased with the addition of fiber. Borhan [16] and Niu et al. [17] have investigated the working performance of basalt fiber reinforced concrete and they found that the addition of short-cut basalt fiber can significantly reduce the fluidity of concrete mixture, and the fiber agglomeration phenomenon is serious with the increase of the content. All these performances can be explained by the following two reasons: (1) Basalt fiber is easy to agglomerate when mixed because it is soft and fine, and there are more pores in the mixture, which increases the weak interface inside the concrete and reduces the strength of concrete. (2) Due to the water absorption of basalt fiber, the reduction of flowing water in the mixture results in a significant drop in the fluidity of concrete, and the production and development of the strength of the concrete matrix are also affected for the same reason [18].
The expansion of micro-cracks and macro-cracks in concrete can be effectively inhibited by fibers, which was considered to be a credible measure to improve the crack resistance of concrete, and it has been used in all levels of road surface and bridge deck paving. In recent years, a large number of experts and scholars at home and abroad have studied the fracture performance of fiber-reinforced concrete [19]. Gao [6] and Arslan [10] investigated fracture behavior of basalt fiber reinforced concrete (BFRC) and glass fiber reinforced concrete (GFRC) comparatively. The results showed that the effects of the fiber contents on fracture energy were very significant. Similar trend were observed by Hossain et al. [20–23] carried out the fracture test of steel fiber concrete, and the study found that increasing the length of steel fiber can correspondingly increase the fracture energy of concrete under the conditions of other parameters. Compared with chopped basalt fiber, the surface of the new basalt fiber bundle [24] is rougher, which is more conducive to enhancing the fracture energy of concrete. In this experiment, a notched three-point bending beam was used to study the effect of the new basalt fiber bundle on the fracture performance of concrete. Some control groups were set up to determine the P-CMOD curve of each group of specimens, and the fracture characteristic parameters of each control group were obtained through theoretical calculations. Basalt fiber bundles with different diameters and contents were compared to analyze the strengthening effect on the fracture properties of concrete.
2.1 Raw Materials and Experimental Design
The cement used was 42.5 ordinary Portland cement. Natural sand with a fineness modulus of 2.85 was utilized as fine aggregate. The crushed stone was composed of two particle sizes of 5–10 mm and 10–20 mm, and the ratio was 4:6. The superplasticizer used was a kind of polycarboxylate-type high-performance superplasticizer in powder form. The basalt fiber bundles used in this paper were made of basalt fiber monofilament strands impregnated with glue and then cut short. As shown in Fig. 1, the fiber bundle length was 30 mm, and the diameter was 0.2, 0.5, 0.8 mm, respectively. The basalt fiber monofilament parameters are shown in Table 1.
Figure 1: Basalt fiber bundles of different diameters Adapted with permission from reference [25], Copyright © 2022, Journal of Physics: Conference Series
The cement uses 42.5 ordinary Portland cement produced by Tianrui Group Zhengzhou Cement Company, China. All indicators meet the requirements of the «General Portland Cement» GB175-2007 standard. See Table 2 for specific performance parameters.
The fine aggregate is selected from a certain local river sand with a fineness modulus of 2.85, which is medium sand. All aspects of performance meet the requirements of “Standard for Quality and Inspection Methods of Sand and Stone for Common Concrete” JGJ52-2006. See Table 3 for the results of particle screening.
Crushed stone was used as the coarse aggregate in this article. From the classification of gradation, it can be known that the gravel is a continuous gradation. The crushed stone is composed of two particle sizes of 5–10 mm and 10–20 mm, and the ratio is 4:6, as shown in Figs. 2 and 3. All indicators comply with “Standard for Quality and Inspection Methods of Sand and Stone for Common Concrete” JGJ52-2006. The basic physical and mechanical properties of coarse aggregate are shown in Table 4.
Figure 2: Particle size of 5~10 mm
Figure 3: Particle size of 10~20 mm
As a polymer surfactant, superplasticizer has strong solid-liquid interfacial activity. On the one hand, the incorporation of superplasticizers can greatly increase the fluidity of the cement paste; on the other hand, the incorporation of superplasticizers can greatly reduce the water consumption of concrete. The water reduction rate of ordinary water reducing agents is generally 5%~10%, and the water reducing rate of superplasticizers can generally reach about 3 times that of the former, and has a higher reasonable dosage range than ordinary water reducing agents. Considering that the BFRC water glue is relatively low, it will be difficult to form concrete if it is not mixed with superplasticizers. Therefore, the incorporation of superplasticizer plays a decisive role in the formation of high-performance concrete.
The water-reducing agent is a polycarboxylic acid-based high-performance water-reducing agent produced by Beijing Muhu Admixture Co., Ltd. (China) in powder form.
High-strength concrete with a strength grade of C60 was used as the reference material for this test. The mix proportion is presented in Table 5.
According to Chinese National standard DL/T5332-2005, the fracture behavior of short basalt fiber bundle reinforced concrete (SBFBRC) is tested by using a notched three-point bending beam. The size of the specimen is 100 mm × 100 mm × 400 mm. The width of the prefabricated crack is 2 mm ± 1 mm, and its length is 40 mm, prefabricated with a steel plate, and the seam height ratio
The influence of fiber bundle content and diameter on the fracture performance of concrete was studied in this experiment by 18 specimens, divided into 6 groups. Details can be seen in Table 6.
Specimens were all stirred by a forced mixer and vibrated on a shaking table. The feeding sequence was: firstly, put stones and sand in order and dry mixed for 30 s, then added cement and water to mix for 30 s. After these steps, the fibers were evenly distributed into the mixing pot while stirring and mixed for 1.5 min, to make sure the concrete is well mixed. The mixing time of fiber bundle should be strictly controlled to prevent damage to the surface of fiber bundle caused by long mixing time. After 24 h, the formwork shall be removed immediately and put into the standard curing room for curing.
From the perspective of fiber materials, basalt fibers are softer and thinner than metal fibers, such as steel fibers. The post-adding fiber method can make the fiber dispersion more uniform, and it can avoid the fiber breakage and damage to the test results, caused by the collision of coarse aggregates during the mixing process when only add sand, stone and basalt fiber in the agitator.
Electro-hydraulic servo hydraulic universal testing machine was used in this experiment, the maximum range of which was 100 KN. The load sensor adopted YBY-100 KN load sensor with the range of 10t; the model of displacement sensor was YHD-10; the model of clamp extensometer was YSJ-2/5. The schematic diagram of the test device is shown in Fig. 4.
Figure 4: Loading device for fracture test
Before starting the test, weighed each test piece and recorded the data. Sticked prefabricated processed steel sheets on both sides of the crack with a thickness of 5 mm. After being fixed, inserted the clip-on extensometer between the two steel sheets and fixed it to check whether it has been fixed to avoid missing data during the test. Placed the test piece on the supports on both sides and checked whether the test piece is stable. Placed two displacement meters and one force sensor at the mid-span position on the upper part of the test piece. After placing the test piece in the specified position, turned on the Donghua data acquisition system on the computer connected to the load sensor, displacement meter and clip extensometer, and checked whether each channel is working properly, then started the test machine; make the test piece, the force transmission device and the load sensor were in contact slowly and evenly. When the three were about to be in contact, the data acquisition system was turned on and the acquisition point was cleared.
The fracture test adopted displacement loading, and the recorded rate was 0.1 mm/min, which was convenient for collecting stable data. The acquisition frequency was 1 time/s. The two displacement sensors collected the deflection of the mid-span specimen, the force sensor collected the mid-span load, and the clip extensometer collected the change in the crack width. The changing trends of these three can be seen from the acquisition system.
2.4 Fracture Toughness of Concrete
The stress characteristics of concrete are closely related to the development of cracks. The results of concrete fracture test showed that the crack propagation process was divided into three stages: initial cracking, stable propagation and instability failure. For concrete members with cracks, due to the stable expansion stage at the crack tip, the load-displacement curve showed obvious nonlinear characteristics.
The double-K fracture model is essentially a nonlinear fracture model for concrete based on linear elastic fracture mechanics and considering the influence of cohesive forces acting on the fracture process zone. In the double-K fracture model, two basic fracture parameters are introduced to describe the whole process of concrete fracture failure. Among them, the fracture toughness corresponding to the initial initiation state is called the initiation toughness, and the fracture toughness corresponding to the instability state is called the instability toughness. According to these two parameters, the development state of the crack can be determined. The P-CMOD curve was drawn based on the data of mid span load and crack opening displacement, and the instability toughness
Fracture energy of concrete is defined as the energy required per unit area of crack propagation. The P-δ curve can be drawn according to the data of mid span load and displacement, and the fracture energy of concrete is obtained by the formula
3 Experimental Study on Fracture Behavior of SBFBRC
3.1 Results and Analysis of Fracture Toughness
3.1.1 Load-Crack Opening Displacement Curve in Fracture Test
The P-CMOD curve of each group of specimens is shown in Fig. 5 below.
Figure 5: P-CMOD curve of each specimen
It can be seen from Fig. 5 that with the increase of basalt fiber bundle content, the peak load and crack opening displacement increased gradually, and the peak load increased by 78%. When the fine cracks began to appear at the crack tip, under the action of load, the crack of the reference group keep extending upward, losing the bearing capacity very quickly, showing obvious brittle failure, and the cross section of the specimen was relatively flat. The incorporation of fiber bundles increased the bearing capacity of the specimen, delayed the failure of the specimen, and increased the toughness of the concrete.
According to the double-K fracture model, the unstable fracture toughness and initial fracture toughness of each specimen were calculated, which can be seen in Table 7.
Both peak load and initial fracture toughness gradually increased with the increase of the volume of basalt fiber bundles, as shown in Figs. 4a and 5a. When the basalt fiber bundle volume content was 0.2% and 0.4%, the peak load increased by 52.2% and 62.2% compared with the reference group. The peak load and initial fracture toughness increased by 78.1% and 74.5%, respectively compared with the reference group, reaching maximum when the basalt fiber bundle volume content was 0.6%. With the increase of the content, the unstable fracture toughness did not show a monotonous increase trend. It increased gradually at the content of 0%~0.2% and 0.4%~0.6%, and increased by 77.3% compared with the reference group when the content of basalt fiber bundle was 0.6%. From an overall point of view, with the increase of the content, the peak load, initial fracture toughness and unstable toughness were all significantly improved compared to the reference group.
The change of basalt fiber bundle diameter will also affect the fracture toughness of concrete. The relationship between basalt fiber bundle diameter and peak load was shown in Fig. 7. With the increase of diameter, the peak load decreased gradually, but it was still higher than that of the reference group. When the diameter of fiber bundle was 0.2 mm, the peak load increased by a maximum of 69.5% compared with that of the reference group. Fig. 6 give the relationship between fracture toughness and fiber bundle diameter. As shown in the figure, the initial fracture toughness increases by 59.7% compared with the reference group, reaching a maximum when the fiber diameter was 0.2 mm. The cracking toughness first increased and then decreased with the increase of basalt fiber bundle diameter.
Figure 6: The relationship between fracture toughness and basalt fiber bundle content and diameter
Figure 7: The relationship between peak load and basalt fiber bundle content and diameter
3.1.3 Analysis of Test Results
1. After the plain concrete reaches the crack initiation load, cracks appear. The stable expansion stage of the crack was very short, and the specimen will fracture and fail soon, reaching the peak load. The unstable failure stage was relatively fast; After mixing the basalt fiber bundles, due to the bridging traction of the fiber bundles, the peak load of the concrete was increased, and the slope of the descending section of the curve was small, and the fracture toughness of the concrete was promoted.
2. With the increase of the content of basalt fiber bundles, the number of fiber bundles running through the fracture surface increased accordingly. Hence, the fibers can bear more stress, and the toughness and fracture ultimate load of concrete were gradually increased.
3. Compared with the basalt fiber bundles with diameters of 0.5 and 0.8 mm, the basalt fiber bundles with diameters of 0.2 mm had better effects on increasing the peak load and unstable fracture toughness of concrete. When the fiber bundle content was certain, it was beneficial to increase the length-diameter ratio of the basalt fiber bundle appropriately to improve the fracture toughness of concrete.
3.2 Results and Analysis of Fracture Energy
3.2.1 Midspan Load Displacement Curve of Fracture Test
After adding basalt fiber bundle, the peak load and mid-span deflection of the specimen were greatly improved. Due to the low loading rate, the load-displacement curve of the specimen can be measured more completely by the testing machine, as shown in Fig. 8.
Figure 8: The mid-span load-displacement curves of the specimens
The relationship between fracture energy and the content and diameter of basalt fiber bundle were shown in Fig. 9.
Figure 9: The relationship between fracture energy and basalt fiber bundle content and diameter
The experimental results shown that the addition of basalt fiber bundles greatly increased the fracture energy of concrete, and the fracture performance of concrete was improved; With the increase of basalt fiber bundle volume, the fracture energy of basalt fiber bundle concrete increased gradually. When the basalt fiber bundle volume content was 0.6%, the fracture energy increased by 182.3% compared with the reference group. The change of basalt fiber bundle diameter also greatly affected the fracture performance of concrete. And with the increase of basalt fiber bundle diameter, the fracture energy first increased and then decreased, reaching the peak value when the diameter was 0.2 mm, which was 180.4% higher than the reference group.
When fine crack appeared at the tip of the prefabricated crack, the crack of plain concrete specimen will rapidly expand upward, and the plain concrete will soon lose the bearing capacity and fracture, which shown obvious brittle failure; With the addition of basalt fiber bundles, due to the bridging effect of the fiber, crack propagation was restrained, the bearing capacity of concrete was increased, the peak load of specimens was improved, and the softening curve of basalt fiber bundle concrete was slowed down effectively, accordingly the fracture energy of concrete was significantly increased.
With the increase of basalt fiber bundle content, more fiber bundle will play bridging role, which led to the enhancement of fracture energy of concrete. Compared with the basalt fiber bundles with diameters of 0.5 and 0.8 mm, 0.2 mm diameter had better enhancement effect on concrete fracture energy. It can be seen that when the content of the basalt fiber bundle was unchanged, it is better to control the basalt fiber bundle diameter at about 0.2 mm to improve the fracture performance of concrete. In this case, the bond strength between the fiber bundle and the matrix can better match the fiber properties, reaching an equilibrium limit state, which shows that the fracture energy is greatly enhanced.
In this paper, based on tests, the fracture toughness and fracture energy of SBFBRC were studied, and the improvement mechanism of concrete fracture performance by adding basalt fiber bundles was analyzed. The following conclusions can be drawn from the results presented in this paper:
(1) The incorporation of fiber bundles can significantly improve the fracture performance of concrete. The volume content and diameter both have influence on the fracture performance.
(2) The peak load increased gradually with the increase of basalt fiber bundle volume content, reaching a maximum when the basalt fiber bundle volume content was 0.6%, which was 78.1% higher than that of the reference group. On the whole, with the increase of mixing amount, peak load, crack initiation toughness, instability toughness and fracture energy are improved greatly compared with the reference group.
(3) The peak load increased by 69.5% compared with the reference group when the fiber bundle diameter was 0.2 mm, and the unstable fracture toughness increased by 59.7% compared with the reference group, reaching a maximum value, when the basalt fiber bundle diameter was 0.2 mm.
Funding Statement: This work is supported by the financial support from the Key R&D Projects of the Ministry of Transport (2018-MS5-136), Henan Province Transportation Science and Technology Plan Project (2018J2, 2019J-2-10, 2020J-2-7).
Conflicts of Interest: The authors declare that they have no conflicts of interest to report regarding the present study.
References
1. Mehran, K. (2021). Experimental and analytical study of hybrid fiber reinforced concrete prepared with basalt fiber under high temperature. Fire and Materials, 46(1), 205–226. [Google Scholar]
2. Zhou, H., Jia, B., Huang, H., Mou, Y. L. (2020). Experimental study on basic mechanical properties of basalt fiber reinforced concrete. Materials, 13(6), 1362. DOI 10.3390/ma13061362. [Google Scholar] [CrossRef]
3. Alnahhal, W., Aljidda, O. (2018). Flexural behavior of basalt fiber reinforced concrete beams with recycled concrete coarse aggregates. Construction & Building Materials, 169(1), 165–178. DOI 10.1016/j.conbuildmat.2018.02.135. [Google Scholar] [CrossRef]
4. Katkhuda, H., Shatarat, N. (2017). Improving the mechanical properties of recycled concrete aggregate using chopped basalt fibers and acid treatment. Construction and Building Materials, 140(1), 328–335. DOI 10.1016/j.conbuildmat.2017.02.128. [Google Scholar] [CrossRef]
5. Jalasutram, S., Sahoo, D. R., Matsagar, V. (2017). Experimental investigation of the mechanical properties of basalt fiber-reinforced concrete. Structural Concrete, 18(2), 292–302. DOI 10.1002/suco.201500216. [Google Scholar] [CrossRef]
6. Gao, C. H. (2020). Dynamic and static splitting-tensile properties of basalt fiber-reinforced cemented clay under freeze-thaw cycles. Journal of Materials in Civil Engineering, 32(10), 06020014. DOI 10.1061/(ASCE)MT.1943-5533.0003350. [Google Scholar] [CrossRef]
7. Kizilkanat, A. B., Kabay, N., Akyüncü, V., Chowdhury, S., Akça, A. H. (2015). Mechanical properties and fracture behavior of basalt and glass fiber reinforced concrete: An experimental study. Construction and Building Materials, 100(4), 218–224. DOI 10.1016/j.conbuildmat.2015.10.006. [Google Scholar] [CrossRef]
8. Branston, J., Booya, E., Gorospe, K., Adesina, A., Das, S. (2019). Microstructure and mechanical properties of basalt fibre reinforced concrete. 17th Euroseminar on Microscopy Applied to Building Materials, pp. 121–127. Toronto, Canada. [Google Scholar]
9. Iyer, P., Kenno, S. Y., Das, S. (2016). Performance of fiber-reinforced concrete made with basalt-bundled fibers. Advances in Civil Engineering Materials, 5(1), 107–123. DOI 10.1520/ACEM20150027. [Google Scholar] [CrossRef]
10. Arslan, M. E. (2016). Effects of basalt and glass chopped fibers addition on fracture energy and mechanical properties of ordinary concrete: CMOD measurement. Construction and Building Materials, 114, 383–391. DOI 10.1016/j.conbuildmat.2016.03.176. [Google Scholar] [CrossRef]
11. Ma, J., Qiu, X., Cheng, L., Wang, Y. (2011). Experimental research on the fundamental mechanical properties of presoaked basalt fiber concrete. In: Advances in FRP composites in civil engineering, pp. 85–88. Berlin, Heidelberg: Springer. [Google Scholar]
12. Jiang, C. H., Fan, K., Wu, F., Chen, D. (2014). Experimental study on the mechanical properties and microstructure of chopped basalt fibre reinforced concrete. Materials and Design, 58(8), 187–193. DOI 10.1016/j.matdes.2014.01.056. [Google Scholar] [CrossRef]
13. Kabay, N. (2014). Abrasion resistance and fracture energy of concretes with basalt fiber. Construction & Building Materials, 50(5), 95–101. DOI 10.1016/j.conbuildmat.2013.09.040. [Google Scholar] [CrossRef]
14. Li, M., Gong, F., Wu, Z. S. (2020). Study on mechanical properties of alkali-resistant basalt fiber reinforced concrete. Construction and Building Materials, 245, 118424. DOI 10.1016/j.conbuildmat.2020.118424. [Google Scholar] [CrossRef]
15. Sarkar, A., Hajihosseini, M. (2019). The effect of basalt fibre on the mechanical performance of concrete pavement. Road Materials & Pavement Design, 21(6), 1726–1737. DOI 10.1080/14680629.2018.1561379. [Google Scholar] [CrossRef]
16. Borhan, T. M. (2012). Properties of glass concrete reinforced with short basalt fibre. Materials & Design, 42(12), 265–271. DOI 10.1016/j.matdes.2012.05.062. [Google Scholar] [CrossRef]
17. Niu, D., Su, L., Luo, Y., Huang, D. G., Luo, D. M. (2020). Experimental study on mechanical properties and durability of basalt fiber reinforced coral aggregate concrete. Construction and Building Materials, 237(1), 117628. DOI 10.1016/j.conbuildmat.2019.117628. [Google Scholar] [CrossRef]
18. Xue, Q. C., Zhang, J. G., He, J. (2016). Experimental study of fracture properties for basalt-fiber-reinforced concrete. Journal of Harbin Engineering University, 37(8), 1027–1033 (in Chinese). [Google Scholar]
19. Ma, Y. H., Ma, H. X., Guan, X. (2019). Experimental study and numerical simulation on fracture properties of fiber reinforced concrete. Journal of Highway and Transportation Research and Development, 36(12), 37–46 (in Chinese). [Google Scholar]
20. Hossain, K. M. A., Lachemi, M., Sammour, M., Sonebi, M. (2013). Strength and fracture energy characteristics of self-consolidating concrete incorporating polyvinyl alcohol, steel and hybrid fibres. Construction & Building Materials, 45(2), 20–29. DOI 10.1016/j.conbuildmat.2013.03.054. [Google Scholar] [CrossRef]
21. Yoo, D. Y., Lee, J. H., Yoon, Y. S. (2013). Effect of fiber content on mechanical and fracture properties of ultra high performance fiber reinforced cementitious composites. Composite Structures, 106(1–3), 742–753. DOI 10.1016/j.compstruct.2013.07.033. [Google Scholar] [CrossRef]
22. Alberti, M. G., Enfedaque, A., Gálvez, J. C. (2016). Fracture mechanics of polyolefin fibre reinforced concrete: Study of the influence of the concrete properties, casting procedures, the fibre length and specimen size. Engineering Fracture Mechanics, 154(6), 225–244. DOI 10.1016/j.engfracmech.2015.12.032. [Google Scholar] [CrossRef]
23. Bayramov, F., Tasdemir, C., Tasdemir, M. A. (2004). Optimization of steel fiber reinforced concretes by means of statistical response surface method. Cement and Concrete Composites, 26(3), 665–675. [Google Scholar]
24. Shao, J. Q., Wang, W., Zhang, P. (2019). Chopped basalt fiber bundles used to reinforce cement concrete and its preparation method. Chinese Patent, 2(22), 245–251. [Google Scholar]
25. Shao, J. G., Wang, S., Liu, Y., Zhang, P., Tang, G. B. et al. (2022). Experimental study of basic mechanical properties of short basalt fiber bundle reinforced concrete. Journal of Physics: Conference Series, 2158(1), 012037. [Google Scholar]
Cite This Article
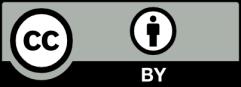