Open Access
VIEWPOINT
Poly(ADP-ribose), adherens junctions, vinculin and the actin cytoskeleton: Current evidence, future perspectives and implications
1 Instituto de Investigaciones Biológicas Clemente Estable, Ministerio de Educación y Cultura, Montevideo, 11600, Uruguay
2 Centro Universitario Regional Litoral Norte, Universidad de la República, Salto, 50000, Uruguay
* Corresponding Author: LAURA LAFON-HUGHES. Email: Array
BIOCELL 2022, 46(12), 2531-2535. https://doi.org/10.32604/biocell.2022.022713
Received 22 March 2022; Accepted 21 June 2022; Issue published 10 August 2022
Abstract
Poly(ADP-ribose) (PAR) is a highly negatively charged polymer. PAR is synthesized by poly(ADP-ribose)polymerases (PARPs) and is involved in the assembly and stabilization of macromolecular complexes. Here, the presence and putative roles of poly(ADP-ribosyl)ation (PARylation) associated to adherens junctions (AJ) and the actin cytoskeleton in epithelial and Schwann cells, is reviewed. The hypothesis generated by analogy, stating that PAR is associated to AJ in other cell types, is postulated. According to this hypothesis, PAR associated to puncta adherentia in chemical synapses would participate in plasticity, learning and memory. In turn, PAR associated to fascia adherens in cardiomyocytes, would affect heart beating. PARP inhibitors are currently under development and clinical testing. Basic research in different tissues will probably influence their clinical uses.Keywords
An Introduction to Poly(ADP-ribose) (PAR)
Poly(ADP-ribosyl)ation (PARylation) is a biochemical reaction consisting on the synthesis of a linear or branched polymer called poly(ADP-ribose) (PAR), comprising up to 400 ADP-ribose monomers. PAR is covalently bound to proteins as a posttranslational modification, or to DNA (Matta et al., 2020). With two negatively charged phosphates per unit and substructures that can be recognized by selected protein domains-such as Macro, WWE, RNA recognition motif (RRM) or PBM-, PAR can be regarded as a “glue” that facilitates assembly and stabilization of certain macromolecular complexes (Hottiger, 2015; Leung, 2014). Although PAR remained unnoticed until 1963 (Chambon et al. 1963, reviewed in Kraus, 2015), it is involved in several cellular processes. Moreover, PAR metabolism displays alterations in several inflammatory pathologies including diabetes, cardiovascular disease, infections, autoimmune disease, cancer and neurodegeneration (Bai, 2015; Cerboni et al., 2010; Masutani et al., 2005; Strosznajder et al., 2012; Virag, 2002).
PARylation is catalyzed by four enzymes: PARP-1, PARP-2, tankyrase-1 (TNKS-1) and tankyrase-2 (TNKS-2). PARP-1/2 and TNKS-1/2 belong to a larger molecular family (PARP family, or ARTDs) that does also include inactive members-probably playing regulatory roles through substrate competition-, as well as intracellular enzymes with mono(ADP-ribosyl)ating activity (Lüscher et al., 2022). PARP-1, the canonical PARP, has been deeply involved in all nuclear functions, including chromatin structure modulation, transcriptional regulation, imprinting (Lafon-Hughes et al., 2008), DNA repair and cellular response to stress. PARP-1 and PARP-2 have partially overlapping functions (Bai, 2015).
TNKS-1/2 lack the DNA binding domain of PARP-1/2. They have an Ankyrin binding domain, present in several sub-membrane region proteins, instead (Chi and Lodish, 2000). TNKS is required during mitosis, colocalizes with the Golgi system (Smith and de Lange, 1999; Wahlberg et al., 2012), and is involved in cell junction assembly and Wnt/Axin/β-catenin signaling (Bao et al., 2012; Chi and Lodish, 2000; Yeh et al., 2006).
Current Evidence Associating PAR with Adherens Junctions (AJ), Vinculin and Actin Microfilaments
TNKS–dependent PARylation of VCL vs. epithelial cell morphology
In epithelial cells, some actin microfilaments are anchored to cell-matrix integrin-harboring focal adhesions (FA) while a characteristic subcortical actin ring is bound to a cadherin and catenin-rich cell-cell adherens junction (AJ) belt or zonula adherens (Domke et al., 2014; Niessen and Gottardi, 2008). Some zonula AJ proteins, like β-catenin, are called nuclear and AJ complexes proteins (NaCos) because they help coordinate cell shape and polarity with cycling dynamics, alternatively being integral components of zonula AJ complexes or acting as transcription factors (Aho et al., 2009; Balda, 2003). A relevant actor in anchoring the actin cytoskeleton to both zonula AJ and FA is Vinculin (VCL) (Bays and DeMali, 2017). Being involved in mechanosensing, VCL recruitment to zonula AJ is force-dependent (Le Duc et al., 2010). VCL regulates cell-cell contact stability (Seddiki et al., 2018) and facilitates mechanical coupling between actin microfibers and the extracellular matrix (Angulo-Urarte et al., 2020). Interestingly, VCL regulates epithelial cells characteristics including cell polarity, cycling, adhesion and migration (Coll et al., 1995; Maddugoda et al., 2007; Pal et al., 2019; Peng et al., 2010; Raz and Geiger, 1982; Sumida et al., 2011; Xu et al., 1998a; Xu et al., 1998b). VCL has also been detected in the nucleus, indicating that it might be a NaCo (Flachs et al., 2019; Hwang et al., 2017).
Epithelial cells harbor a PAR belt, detected by immunoctytofluorescence under confocal microscopy (ICF-CFM). The PAR belt colocalizes with zonula AJ, VCL and the subcortical actin ring. In contrast, FA display no PARylation signal (Lafon-Hughes et al., 2014). Therefore, the VCL pool PARylated in epithelial cells extracts, would correspond to VCL bound to zonula AJ (Vilchez Larrea et al., 2021).
The PAR belt is lost under treatment with cytochalasin D, an actin polymerization inhibitor (Lafon-Hughes et al., 2014). Conversely, in the presence of TNKS inhibitors, neither the PAR belt nor the subcortical actin ring are assembled, while cell shape becomes more irregular, mesenchymal-like (Lafon-Hughes et al., 2014; Vilchez Larrea et al., 2021). VCL harbors a TNKS binding motif (TBM) required to achieve TNKS-dependent PARylation. The overexpression of a chicken VCL gene carrying a point mutation in the TBM, unlike wt-VCL, induces a cell shape change reminiscent of an epithelial to mesenchymal transition (EMT) (Vilchez Larrea et al., 2021). Accordingly, in an EMT model, under TGF-β induction of EMT, the PAR belt is lost together with epithelial markers, characteristic cell shape and low F-actin anisotropy (Schacke et al., 2019). In the meantime, nuclear PAR increases. Conversely, a treatment with Olaparib, an inhibitor of PARP-1/2, diminishes nuclear PAR, preserves epithelial morphology/anisotropy and molecular markers, and restores the PAR belt, indicating the importance of the PAR belt and its close relation with the actin cytoskeleton (Schacke et al., 2019). This work highlights the fact that Olaparib, approved as an anticancer drug following a synthetic lethality paradigm, may show effects beyond the promotion of cell death through DNA repair failure. Olaparib can deeply affect the cell morphological and functional phenotype, including the PAR belt, suggesting the existence of a crosstalk among nuclear PARPs and TNKS.
Schwann cells autotypic AJ regions are PARylated and total PAR distribution and quantity parallels F-actin
The presence of PAR associated to AJ-rich regions in non-epithelial tissues could be biologically meaningful. In fact, in peripheral nerves, Schwann cells harbor autotypical AJ that fix the successive myelin layers (Fannon et al., 1995). Such autotypical AJ, with a molecular composition similar to epithelial zonula AJ (e.g., E-cadherin rich), are distributed in regions called paranodes (PN) and Schmidt-Lanterman incisures (SLI). Analysis of Schwann cells supported PAR presence in PN and SLI. Moreover, in a mice model of Charcot-Marie-Tooth demyelinating pathology, PAR distribution and quantity alterations parallel the F-actin changes (Lafon Hughes et al., 2017). This work indicates that PAR is associated with autotypical AJ and the actin cytoskeleton. Moreover, it links PARylation and myelination.
A role of VCL PARylation in synaptic plasticity, learning and memory?
Fig. 1 highlights the analogous structure of AJ complexes from different cell types in spite of their dissimilar subcellular distribution patterns. The figure depicts the core structure of AJ (Fig. 1A), the epithelial cells with their belt (Fig. 1B), Schwann cells with AJ at autotypic junctions at PN and SLI (Fig. 1C), neuronal synapses with synaptic clips called transynaptic puncta adherentia (Fig. 1D) and cardiomyocytes with fascia adherentia in their intercalated discs (Fig. 1E). Fig. 1 does not pretend to be exhaustive; it is just showing well-documented examples. PAR has been detected in epithelial zonula adherens and is Schwann cell PN and SLI. PAR detection in puncta adherentia and fascia adherens, with different implications, is envisaged.
Figure 1: Examples of cell types where AJ fundamental structure is conserved while AJ distribution varies. (A) AJ share a common molecular structure. Two neighbor membranes are represented as blue segments. Cadherins (yellow) have an extracellular domain that mediates adhesion, a single pass transmembrane domain, and a cytoplasmic domain that interacts with catenins (blue ovals) to anchorage F-actin (green). VCL (red) is recruited to the AJ-actin cytoskeleton interphase to support tension. (B) Zonula adherens in epithelial cells. (C) Autotypic AJ in paranodes (PN) and Schmidt-Lanterman Incisures (SLI) in Schwann cells. (D) Transynaptic puncta adherentia in a neuronal chemical synapse, joining the presynaptic and postsynaptic components (E) Fascia adherentia in intercalated discs in cardiomyocytes.
In chemical synapses, transynaptic AJ complexes called puncta adherentia clip the presynaptic active zone (PRE) and postsynaptic density (POST) (Kilinc, 2018; Uchida et al., 1996). Cadherins are involved in synaptic plasticity and long-term potentiation (LTP). Synaptic activity modulates synaptic adhesiveness (Bekirov et al., 2002; Benson, 2000; Bozdagi et al., 2004; Fields and Itoh, 1996; Tang et al., 1998). Catenins do also regulate synapses. For example, upon depolarization, β-catenin relocation promotes synaptic structural and functional changes (Kilinc, 2018). VCL is required for neuronal mechanosensing (Wang et al., 2021) and participates in synaptic plasticity (Liśkiewicz et al., 2020). F-actin does also play a role in synaptic structure and function (Bucher et al., 2020; Gentile et al., 2022; Lavoie-Cardinal et al., 2020; Zhang and Benson, 2001). To sum up, cumulative evidence indicates that puncta AJ, VCL, mechanical forces and the actin cytoskeleton play concerted crucial roles in synaptic establishment and plasticity. One wonders (i) whether PAR is associated to puncta AJ, and in such case, (ii) whether PARylation is involved in synaptic plasticity, LTP, memory and learning. We cannot answer the former issue yet. Regarding the latter, independent evidence indicates that PARylation can be induced by depolarization or neurotrofin treatment (Homburg et al., 2000; Visochek, 2005), and that LTP, memory and learning require PARylation (Berger et al., 2018; Cohen-Armon, 2004; Goldberg et al., 2009; Strosznajder et al., 2012). To what extent nuclear PAR or puncta AJ-associated PAR contribute to the cited results, remains to be established. Thus, the presence of PAR associated with puncta AJ in chemical synapses is a reasonable working hypothesis. By analogy to epithelial AJ, TNKS involvement in the PARylation of VCL associated with puncta AJ is expected. Indeed, in primary hippocampal neurons, TNKS is detected in the soma and neurites, partially co-localizing with PAR signals. Moreover, TNKS inhibitor XAV939 suppresses neurite outgrowth and synaptogenesis (Mashimo et al., 2022). It would be worth verifying the colocalization among puncta AJ, VCL, synaptic components, F-actin and PAR. If confirmed, it would also be tempting to analyze if there are changes in puncta AJ-associated PARylation associated to plasticity, LTP, learning and memory.
A role of VCL PARylation in cardiomyocytes?
VCL knockout results in brain and heart defects during embryonic development (Xu et al., 1998a). Cardiomyocytes cell-cell AJ constitute the fascia adherens, located in intercalated disks (ICD). The fascia adherens is involved in mechanical coupling and reinforcement of cardiomyocytes, withstanding repeated cycles of actomyosin-mediated contractile force. VCL is a critical link to contractile actomyosin (Merkel et al., 2019). Components of the fascia AJ including N-cadherin, α-E-catenin, β-catenin, and VCL play important roles in cardiac development, disease, and arrhythmias. In fact, cardiac-myocyte–specific VCL knockout mice display cardiac AJ/ICD abnormalities, leading to ventricular tachycardia and sudden death. VCL is necessary for preservation of ICD ultra-structure as well as cardiac function (Sheikh et al., 2009). It is highly probable that PAR is present in fascia AJ, strengthening the junctions and participating in differentiation maintenance.
Future Perspectives and Clinical Implications
The presence of PAR associated to grouped AJ subject to high-tension forces and recruiting VCL is likely to have important structural, physiological and pathological relevance in contexts as different as epithelial cell phenotype maintenance, myelination by Schwann cells, synaptic plasticity, cardiac development and heart beating. PARP-1/2 and TNKS inhibitors are being actively developed and introduced to the clinic. Basic research regarding the effect of such inhibitors in different tissues (not limited to the ones here addressed) will likely lead to context-dependent restrictions and expansions in inhibitors clinical uses.
Acknowledgement: I am grateful to Dr. Silvia Fernández-Villamil (INGEBI-CONICET, Argentina) and Dr. Sergei Nechaev (UND, USA) for critical manuscript reading.
Author Contribution: The author confirms sole responsibility for the following: study conception and design, data collection, analysis and interpretation of results, and manuscript preparation.
Ethics Approval: Not applicable.
Funding Statement: The author received no specific funding for this study.
Conflicts of Interest: The author declares that she has no conflicts of interest to report regarding the present study.
References
Aho S, Lupo J, Coly PA, Sabine A, Castellazzi M, Morand P, Sergeant A, Manet E, Boyer V, Gruffat H (2009). Characterization of the ubinuclein protein as a new member of the nuclear and adhesion complex components (NACos). Biology of the Cell 101: 319–334. DOI 10.1042/BC20080072. [Google Scholar] [CrossRef]
Angulo-Urarte A, van der Wal T, Huveneers S (2020). Cell-cell junctions as sensors and transducers of mechanical forces. Biochimica et Biophysica Acta (BBA)-Biomembranes 1862: 183316. DOI 10.1016/j.bbamem.2020.183316. [Google Scholar] [CrossRef]
Bai P (2015). Review biology of poly (ADP-Ribose) polymerases: The factotums of cell maintenance. Molecular Cell 58: 947–958. DOI 10.1016/j.molcel.2015.01.034. [Google Scholar] [CrossRef]
Balda M (2003). Epithelial cell adhesion and the regulation of gene expression. Trends in Cell Biology 13: 310–318. DOI 10.1016/S0962-8924(03)00105-3. [Google Scholar] [CrossRef]
Bao R, Christova T, Song S, Angers S, Yan X, Attisano L (2012). Inhibition of tankyrases induces axin stabilization and blocks wnt signalling in breast cancer cells. PLoS One 7: e48670. DOI 10.1371/journal.pone.0048670. [Google Scholar] [CrossRef]
Bays JL, DeMali KA (2017). Vinculin in cell-cell and cell-matrix adhesions. Cellular and Molecular Life Sciences 74: 2999–3009. DOI 10.1007/s00018-017-2511-3. [Google Scholar] [CrossRef]
Bekirov IH, Needleman LA, Zhang W, Benson DL (2002). Identification and localization of multiple classic cadherins in developing rat limbic system. Neuroscience 115: 213–227. DOI 10.1016/S0306-4522(02)00375-5. [Google Scholar] [CrossRef]
Benson D (2000). Making memories stick: Cell-adhesion molecules in synaptic plasticity. Trends in Cell Biology 10: 473–482. DOI 10.1016/S0962-8924(00)01838-9. [Google Scholar] [CrossRef]
Berger NA, Besson VC, Boulares AH, Bürkle A, Chiarugi A et al. (2018). Opportunities for the repurposing of PARP inhibitors for the therapy of non-oncological diseases: PARP inhibitors for repurposing. British Journal of Pharmacology 175: 192–222. DOI 10.1111/bph.13748. [Google Scholar] [CrossRef]
Bozdagi O, Valcin M, Poskanzer K, Tanaka H, Benson DL (2004). Temporally distinct demands for classic cadherins in synapse formation and maturation. Molecular and Cellular Neuroscience 27: 509–521. DOI 10.1016/j.mcn.2004.08.008. [Google Scholar] [CrossRef]
Bucher M, Fanutza T, Mikhaylova M (2020). Cytoskeletal makeup of the synapse: Shaft versus spine. Cytoskeleton 77: 55–64. DOI 10.1002/cm.21583. [Google Scholar] [CrossRef]
Cerboni B, Di Stefano A, Micheli V, Morozzi G, Pompucci G, Sestini S (2010). PARP activity and NAD concentration in PMC from patients affected by systemic sclerosis and lupus erythematosus. Nucleosides, Nucleotides & Nucleic Acids 29: 471–475. DOI 10.1080/15257771003741471. [Google Scholar] [CrossRef]
Chambon P, Weill JD, Mandel P (1963). Nicotinamide mononucleotide activation of new DNA-dependent polyadenylic acid synthesizing nuclear enzyme. Biochemical and Biophysical Research Communications 11: 39–43. DOI 10.1016/0006-291x(63)90024-x. [Google Scholar] [CrossRef]
Chi NW, Lodish HF (2000). Tankyrase is a Golgi-associated mitogen-activated protein kinase substrate that interacts with IRAP in GLUT4 vesicles. Journal of Biological Chemistry 275: 38437–38444. DOI 10.1074/jbc.M007635200. [Google Scholar] [CrossRef]
Cohen-Armon M (2004). Long-term memory requires PolyADP-ribosylation. Science 304: 1820–1822. DOI 10.1126/science.1096775. [Google Scholar] [CrossRef]
Coll JL, Ben-Ze’ev A, Ezzell RM, Rodriguez Fernandez JL, Baribault H, Oshima RG, Adamson ED (1995). Targeted disruption of vinculin genes in F9 and embryonic stem cells changes cell morphology, adhesion, and locomotion. Proceedings of the National Academy of Sciences 92: 9161–9165. DOI 10.1073/pnas.92.20.9161. [Google Scholar] [CrossRef]
Domke LM, Rickelt S, Dörflinger Y, Kuhn C, Winter-Simanowski S, Zimbelmann R, Rosin-Arbesfeld R, Heid H, Franke WW (2014). The cell-cell junctions of mammalian testes: I. The adhering junctions of the seminiferous epithelium represent special differentiation structures. Cell and Tissue Research 357: 645–665. DOI 10.1007/s00441-014-1906-9. [Google Scholar] [CrossRef]
Fannon AM, Sherman DL, Ilyina-Gragerova G, Brophy PJ, Friedrich VL, Colman DR (1995). Novel E-cadherin-mediated adhesion in peripheral nerve: Schwann cell architecture is stabilized by autotypic adherens junctions. Journal of Cell Biology 129: 189–202. DOI 10.1083/jcb.129.1.189. [Google Scholar] [CrossRef]
Fields RD, Itoh K (1996). Neural cell adhesion molecules in activity-dependent development and synaptic plasticity. Trends in Neurosciences 19: 473–480. DOI 10.1016/S0166-2236(96)30013-1. [Google Scholar] [CrossRef]
Flachs P, Darasova A, Hozak P (2019). Nuclear vinculin involvement in mouse spermatogenesis. Biopolymers and Cell 35: 177–178. DOI 10.7124/bc.0009B4. [Google Scholar] [CrossRef]
Gentile JE, Carrizales MG, Koleske AJ (2022). Control of synapse structure and function by actin and its regulators. Cells 11: 603. DOI 10.3390/cells11040603. [Google Scholar] [CrossRef]
Goldberg S, Visochek L, Giladi E, Gozes I, Cohen-Armon M (2009). PolyADP-ribosylation is required for long-term memory formation in mammals. Journal of Neurochemistry 111: 72–79. DOI 10.1111/j.1471-4159.2009.06296.x. [Google Scholar] [CrossRef]
Homburg S, Visochek L, Moran N, Dantzer F, Priel E, Asculai E, Schwartz D, Rotter V, Dekel N, Cohen-Armon M (2000). A fast signal-induced activation of poly (Adp-Ribose) polymerase. Journal of Cell Biology 150: 293–308. DOI 10.1083/jcb.150.2.293. [Google Scholar] [CrossRef]
Hottiger MO (2015). SnapShot: ADP-Ribosylation signaling. Molecular Cell 58: 1134–1134. DOI 10.1016/j.molcel.2015.06.001. [Google Scholar] [CrossRef]
Hwang JR, Chou CL, Medvar B, Knepper MA, Jung HJ (2017). Identification of β-catenin-interacting proteins in nuclear fractions of native rat collecting duct cells. American Journal of Physiology-Renal Physiology 313: F30–F46. DOI 10.1152/ajprenal.00054.2017. [Google Scholar] [CrossRef]
Kilinc D (2018). The emerging role of mechanics in synapse formation and plasticity. Frontiers in Cellular Neuroscience 12: 483. DOI 10.3389/fncel.2018.00483. [Google Scholar] [CrossRef]
Kraus WL (2015). PARPs and ADP-Ribosylation: 50 years…and counting. Molecular Cell 58: 902–910. DOI 10.1016/j.molcel.2015.06.006. [Google Scholar] [CrossRef]
Lafon Hughes LI, Romeo Cardeillac CJ, Cal Castillo KB, Vilchez Larrea SC, Sotelo Sosa JR, Folle Ungo GA, Fernández Villamil SH, Kun González AE (2017). Poly(ADP-ribosylation) is present in murine sciatic nerve fibers and is altered in a Charcot-Marie-Tooth-1E neurodegenerative model. PeerJ 5: e3318. DOI 10.7717/peerj.3318. [Google Scholar] [CrossRef]
Lafon-Hughes L, Di Tomaso MV, Méndez-Acuña L, Martínez-López W (2008). Chromatin-remodelling mechanisms in cancer. Mutation Research-Reviews in Mutation Research 658: 191–214. DOI 10.1016/j.mrrev.2008.01.008. [Google Scholar] [CrossRef]
Lafon-Hughes L, Vilchez Larrea SC, Kun A, Fernández Villamil SH (2014). VERO cells harbor a poly-ADP-ribose belt partnering their epithelial adhesion belt. PeerJ 2: e617. DOI 10.7717/peerj.617. [Google Scholar] [CrossRef]
Lavoie-Cardinal F, Bilodeau A, Lemieux M, Gardner MA, Wiesner T, Laramée G, Gagné C, de Koninck P (2020). Neuronal activity remodels the F-actin based submembrane lattice in dendrites but not axons of hippocampal neurons. Scientific Reports 10: 11960. DOI 10.1038/s41598-020-68180-2. [Google Scholar] [CrossRef]
Le Duc Q, Shi Q, Blonk I, Sonnenberg A, Wang N, Leckband D, de Rooij J (2010). Vinculin potentiates E-cadherin mechanosensing and is recruited to actin-anchored sites within adherens junctions in a myosin II-dependent manner. Journal of Cell Biology 189: 1107–1115. DOI 10.1083/jcb.201001149. [Google Scholar] [CrossRef]
Leung AKL (2014). Poly(ADP-riboseAn organizer of cellular architecture. Journal of Cell Biology 205: 613–619. DOI 10.1083/jcb.201402114. [Google Scholar] [CrossRef]
Liśkiewicz A, Marczak Ł., Przybyła M, Sługocka A, Liśkiewicz D, Nowacka-Chmielewska M, Student S, Bogus K, Małecki A, Lewin-Kowalik J (2020). Decreased hippocampal efficiency in obese rats is expressed by impaired cognition, neurogenesis and proteomic changes. Proceedings of the Nutrition Society 79: E400. DOI 10.1017/S0029665120003481. [Google Scholar] [CrossRef]
Lüscher B, Ahel I, Altmeyer M, Ashworth A, Bai P et al. (2022). ADP-ribosyltransferases, an update on function and nomenclature. The FEBS Journal, 16142. DOI 10.1111/febs.16142. [Google Scholar] [CrossRef]
Maddugoda MP, Crampton MS, Shewan AM, Yap AS (2007). Myosin VI and vinculin cooperate during the morphogenesis of cadherin cell-cell contacts in mammalian epithelial cells. Journal of Cell Biology 178: 529–540. DOI 10.1083/jcb.200612042. [Google Scholar] [CrossRef]
Mashimo M, Kita M, Uno A, Nii M, Ishihara M et al. (2022). Tankyrase regulates neurite outgrowth through Poly(ADP-ribosyl)ation-Dependent activation of β-Catenin signaling. International Journal of Molecular Sciences 23: 2834. DOI 10.3390/ijms23052834. [Google Scholar] [CrossRef]
Masutani M, Nakagama H, Sugimura T (2005). Poly(ADP-ribosyl)ation in relation to cancer and autoimmune disease. Cellular and Molecular Life Sciences 62: 769–783. DOI 10.1007/s00018-004-4509-x. [Google Scholar] [CrossRef]
Matta E, Kiribayeva A, Khassenov B, Matkarimov BT, Ishchenko AA (2020). Insight into DNA substrate specificity of PARP1-catalysed DNA poly(ADP-ribosyl)ation. Scientific Reports 10: 3699. DOI 10.1038/s41598-020-60631-0. [Google Scholar] [CrossRef]
Merkel CD, Li Y, Raza Q, Stolz DB, Kwiatkowski AV (2019). Vinculin anchors contractile actin to the cardiomyocyte adherens junction. Molecular Biology of the Cell 30: 2639–2650. DOI 10.1091/mbc.E19-04-0216. [Google Scholar] [CrossRef]
Niessen CM, Gottardi CJ (2008). Molecular components of the adherens junction. Biochimica et Biophysica Acta (BBA)-Biomembranes 1778: 562–571. DOI 10.1016/j.bbamem.2007.12.015. [Google Scholar] [CrossRef]
Pal I, Rajesh Y, Banik P, Dey G, Dey KK et al. (2019). Prevention of epithelial to mesenchymal transition in colorectal carcinoma by regulation of the E-cadherin-β-catenin-vinculin axis. Cancer Letters 452: 254–263. DOI 10.1016/j.canlet.2019.03.008. [Google Scholar] [CrossRef]
Peng X, Cuff LE, Lawton CD, DeMali KA (2010). Vinculin regulates cell-surface E-cadherin expression by binding to -catenin. Journal of Cell Science 123: 567–577. DOI 10.1242/jcs.056432. [Google Scholar] [CrossRef]
Raz A, Geiger B (1982). Altered organization of cell-substrate contacts and membrane-associated cytoskeleton in tumor cell variants exhibiting different metastatic capabilities. Cancer Research 42: 5183–5190. [Google Scholar]
Schacke M, Kumar J, Colwell N, Hermanson K, Folle G, Nechaev S, Dhasarathy A, Lafon-Hughes L (2019). PARP-1/2 inhibitor olaparib prevents or partially reverts EMT induced by TGF-β in NMuMG cells. International Journal of Molecular Sciences 20: 518. DOI 10.3390/ijms20030518. [Google Scholar] [CrossRef]
Seddiki R, Narayana GHNS, Strale P-O, Balcioglu HE, Peyret G et al. (2018). Force-dependent binding of vinculin to α-catenin regulates cell-cell contact stability and collective cell behavior. Molecular Biology of the Cell 29: 380–388. DOI 10.1091/mbc.E17-04-0231. [Google Scholar] [CrossRef]
Sheikh F, Ross RS, Chen J (2009). Cell-cell connection to cardiac disease. Trends in Cardiovascular Medicine 19: 182–190. DOI 10.1016/j.tcm.2009.12.001. [Google Scholar] [CrossRef]
Smith S, de Lange T (1999). Cell cycle dependent localization of the telomeric PARP, tankyrase, to nuclear pore complexes and centrosomes. Journal of Cell Science 112: 3649–3656. DOI 10.1242/jcs.112.21.3649. [Google Scholar] [CrossRef]
Strosznajder JB, Czapski GA, Adamczyk A, Strosznajder RP (2012). Poly(ADP-ribose) polymerase-1 in amyloid beta toxicity and alzheimer’s disease. Molecular Neurobiology 46: 78–84. DOI 10.1007/s12035-012-8258-9. [Google Scholar] [CrossRef]
Sumida GM, Tomita TM, Shih W, Yamada S (2011). Myosin II activity dependent and independent vinculin recruitment to the sites of E-cadherin-mediated cell-cell adhesion. BMC Cell Biology 12: 48. DOI 10.1186/1471-2121-12-48. [Google Scholar] [CrossRef]
Tang L, Hung CP, Schuman EM (1998). A role for the cadherin family of cell adhesion molecules in hippocampal long-term potentiation. Neuron 20: 1165–1175. DOI 10.1016/S0896-6273(00)80497-3. [Google Scholar] [CrossRef]
Uchida N, Honjo Y, Johnson KR, Wheelock MJ, Takeichi M (1996). The catenin/cadherin adhesion system is localized in synaptic junctions bordering transmitter release zones. Journal of Cell Biology 135: 767–779. DOI 10.1083/jcb.135.3.767. [Google Scholar] [CrossRef]
Vilchez Larrea S, Valsecchi WM, Fernández Villamil SH, Lafon Hughes LI (2021). First body of evidence suggesting a role of a tankyrase-binding motif (TBM) of vinculin (VCL) in epithelial cells. PeerJ 9: e11442. DOI 10.7717/peerj.11442. [Google Scholar] [CrossRef]
Virag L (2002). The therapeutic potential of Poly(ADP-Ribose) polymerase inhibitors. Pharmacological Reviews 54: 375–429. DOI 10.1124/pr.54.3.375. [Google Scholar] [CrossRef]
Visochek L (2005). PolyADP-Ribosylation is involved in neurotrophic activity. Journal of Neuroscience 25: 7420–7428. DOI 10.1523/JNEUROSCI.0333-05.2005. [Google Scholar] [CrossRef]
Wahlberg E, Karlberg T, Kouznetsova E, Markova N, Macchiarulo A et al. (2012). Family-wide chemical profiling and structural analysis of PARP and tankyrase inhibitors. Nature Biotechnology 30: 283–288. DOI 10.1038/nbt.2121. [Google Scholar] [CrossRef]
Wang DY, Melero C, Albaraky A, Atherton P, Jansen KA, Dimitracopoulos A, Dajas-Bailador F, Reid A, Franze K, Ballestrem C (2021). Vinculin is required for neuronal mechanosensing but not for axon outgrowth. Experimental Cell Research 407: 112805. DOI 10.1016/j.yexcr.2021.112805. [Google Scholar] [CrossRef]
Xu W, Baribault H, Adamson ED (1998a). Vinculin knockout results in heart and brain defects during embryonic development. Development 125: 327–337. DOI 10.1242/dev.125.2.327. [Google Scholar] [CrossRef]
Xu W, Coll JL, Adamson ED (1998b). Rescue of the mutant phenotype by reexpression of full-length vinculin in null F9 cells; effects on cell locomotion by domain deleted vinculin. Journal of Cell Science 111: 1535–1544. DOI 10.1242/jcs.111.11.1535. [Google Scholar] [CrossRef]
Yeh TY J, Meyer TN, Schwesinger C, Tsun ZY, Lee RM, Chi NW (2006). Tankyrase recruitment to the lateral membrane in polarized epithelial cells: Regulation by cell-cell contact and protein poly(ADP-ribosyl)ation. Biochemical Journal 399: 415–425. DOI 10.1042/BJ20060713. [Google Scholar] [CrossRef]
Zhang W, Benson DL (2001). Stages of synapse development defined by dependence on F-actin. Journal of Neuroscience 21: 5169–5181. DOI 10.1523/JNEUROSCI.21-14-05169.2001. [Google Scholar] [CrossRef]
Cite This Article
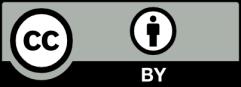