Open Access
ARTICLE
Inhibition of H2O2-induced TM3 cell apoptosis by oxidative stress by lentinan functionalized selenium nanoparticles through JAK2/STAT-3 and P53 pathways
1 Department of Andrology, Guangzhou Women and Children’s Medical Center, Guangzhou Medical University, Guangzhou, 510120, China
2 Center Laboratory, Guangzhou Women and Children’s Medical Center, Guangzhou Medical University, Guangzhou, 510120, China
* Corresponding Authors: YINGHUA LI. Email: ; LIANDONG ZUO. Email:
BIOCELL 2023, 47(6), 1397-1405. https://doi.org/10.32604/biocell.2023.027971
Received 24 November 2022; Accepted 10 February 2023; Issue published 19 May 2023
Abstract
Background: Nano-selenium has been widely used in antiviral and anticancer therapy, and has the advantages of good targeting and low toxicity. For the first time, we combined male reproduction with nano-selenium to investigate its antioxidant effect. This study investigated the protective effect of lentinan functionalized selenium nanoparticles on oxidative stress injury of the hydrogen peroxide (H2O2)-induced Leydig cell line, TM3. Methods: The suitable concentration of nano-selenium treatment to promote cell proliferation was also discussed. The concentration of 4 μM could significantly promote the growth of TM3 cells. Oxidative stress damage was caused using an 800 μM concentration of hydrogen peroxide. The cells were divided into four groups: normal control group, oxidative stress treatment group, H2O2+SeNPs@LNT group, and SeNPs@LNT group. The H2O2+SeNPs@LNT group was pretreated with 4 μM of SeNPs@LNT for 12 h, followed by 800 μM of H2O2 for 8 h. Results: Nano-selenium could significantly promote the proliferation and viability of TM3 cells. SeNPs@LNT treatment increased the level of mitochondrial membrane potential in normal cells and slowed down the decline in mitochondrial membrane potential level caused by oxidative stress injury. In addition, the increase in reactive oxygen species caused by oxidative stress was inhibited by SeNPs@LNT treatment. The apoptosis of TM3 cells was detected, and SeNPs@LNT alleviated the necrosis and apoptosis of TM3 cells induced by H2O2. Nano-selenium plays a protective role against oxidative H2O2-induced stress injury in TM3 cells through the changes in the Janus kinase 2/signal transducer and activator of transcription 3 signaling pathway and P53 pathway, and the expression levels of other related proteins, protein kinase B (AKT) and C3. Conclusion: SeNPs@LNT exhibited good biological activity and antioxidant effect and can thus be used to protect the male reproductive system from oxidative stress.Keywords
Selenium, as an essential trace element, plays an important role in maintaining the normal reproductive structure and function of human beings and animals. It is involved in the synthesis of at least 25 human selenoproteins and essential dietary components containing selenocysteine in the body (Wadhwani et al., 2016). In nature, selenium can be categorized as organic selenium, inorganic selenium, and nano-selenium (Hosnedlova et al., 2018). Three forms of selenium (inorganic selenium, such as sodium selenite and sodium selenate; organic selenium, such as selenocysteine and selenium-enriched yeast; functionalized and non-functionalized nano-selenium) are used in anti-cancer therapy, dietary supplements, as antibacterial and antiviral agents, clinically or in combination with other antioxidants (Labunskyy et al., 2014; Qazi et al., 2019). Inorganic selenium is strictly controlled due to its high biological toxicity. Biological organic selenium has high safety and high selenium supplementation efficiency and is involved in cell repair, free radical scavenging, reducing the physiological toxicity of chemotherapy drugs (such as cisplatin, etc.), and resisting the accumulation of heavy metals, etc. (Maiorino et al., 2018).
As a new type of nanotechnology product, nano-selenium has improved the effectiveness and safety of traditional selenium products and has been used in targeted drug carriers, diabetes treatment, and the treatment of Alzheimer’s disease (Michaelis et al., 2014; Rayman, 2020). Nano-selenium is synthesized in three ways: physical synthesis, chemical synthesis, and biosynthesis. The physical synthesis method cannot be widely used in the production of nano-selenium due to the limitation of synthesis conditions (high temperature and high pressure, catalyst) and low efficiency, and the use of plant extracts, fungi, and bacteria to synthesize bio-derived nano-selenium overcomes the shortcomings (easy to agglomerate and unstable) of particles made by the chemical synthesis, and their special functionalization can further reduce the cytotoxicity or improve the application functionality (Andersen et al., 2003; Boitani and Puglisi, 2008; Hariharan and Dharmaraj, 2020). Throughout the research history of nano-selenium, selenium has been regarded as a toxic substance and even a carcinogen due to its narrow safety range. Until the 20th century, people gradually found that selenium is beneficial to humans and animals; it has antioxidant, anti-cancer, immunity enhancing, and other functions, can treat Keshan disease, cardiovascular diseases, diabetes, cancer, etc., and is an indispensable essential trace element for physiological activities.
In male reproduction, the key role is mediated by two selenoproteins, phospholipid hydrogen peroxide (H2O2) glutathione peroxidase (PHGPx/GPX4) and selenoprotein P (Liu et al., 2019). GPX4, as an oxidation-inactivated protein, constitutes more than half of the mitochondrial envelope in the middle of mature spermatozoa, ensuring the stability and viability of spermatozoa (Zi et al., 2018). GPX4 is thought to protect developing spermatozoa from oxidative stress-induced DNA damage early in the spermatogenesis process (Liu et al., 2020). Strong expression of GPX4 protein in seminiferous tubules and immune signals were also observed in the epididymis and ejaculated sperm (Yan et al., 2021). Selenophosphorin P, as a selenium transporter, is expressed in vesicle-like structures in the basal region of Sertoli cells for selenium uptake (Novak and Mollen, 2015). Its function is closely related to the expression of ApoE receptor 2 (ApoER2). ApoER2 is a receptor highly expressed on the membrane surface of neurons and the initial segment of the epididymis. Its role in the brain is to affect the migration of neurons to the appropriate location in the developing brain, and its role in male reproduction is to transport selenoprotein P to the Sertoli cells of the testis for uptake and utilization. It affects the function and expression of aggretin protein and GPX4 (Liu et al., 2017). Other proteins, GPX1 and GPX3, are also expressed in male reproductive tissues and secretions located in the epididymal epithelium to protect epididymis and mature spermatozoa from oxidative stress (Banerjee et al., 2020). Selenium is the oxidation–reduction (Redox) center of these enzymes and is essential for their biochemical activities (Zorov et al., 2014).
Lentinan is a polysaccharide with a β-1,3-linked glucose backbone and a β-1,6-linked glucose branch chain (Zorov et al., 2014). Lentinan possesses a large number of bioactive groups. As an antioxidant component, lentinan can significantly reduce the malondialdehyde content of neurons and prevent lipid peroxidation in neurons. At the same time, Lentinus edodes polysaccharide can increase SOD activity in animal blood and promote lymphocyte proliferation. The antioxidant mechanism may involve a hydrogen migration scheme, which transfers hydrogen atoms to other molecules, terminates the chain reaction of free radicals, and avoids cell membrane damage and oxidative degradation of nucleic acids by removing superoxide anions (Xu et al., 2019). Past studies have shown that in lentinan-functionalized nano-selenium particles (SeNPs-LNT), the three spiral single-stranded glucan is dispersed in the water medium, proving the interaction between the hydroxyl and nano-selenium, improving the dispersion stability in water and adjust the redox reaction rate or the content of SeNPs-LNT, synthesis of selenium nanoparticles of different size. Compared with simple nano-selenium, Lentinus edodes functionalized nano-selenium has greater stability, stronger biological targeting effect, and better biocompatibility and effectiveness than inorganic and organic compounds (Xu et al., 2019; Tavoosi et al., 2020).
Aiming at the physiological and biochemical effects of selenium in male reproduction and the application of nanomaterials in antioxidants, this study evaluated the cytoprotective effects and the mechanism involved in SeNPs-LNT on H2O2-induced oxidative stress injury in mouse mesenchymal cells, TM3. The study showed that it can significantly reduce the ROS level, reduce the apoptosis of cells, act on mitochondria, change the mitochondrial membrane potential, and regulate the physiological function of cells through Janus kinase 2/signal transducer and activator of transcription 3 (JAk2/STAT-3) pathway and P53 pathway, and C3 and AKT-related proteins are involved.
Cell Counting Kit-8 (CCK8) (C0038) and BCA protein detection kit (P0009), Reactive Oxygen Species (ROS) Assay Kit (S0033), and Annexin V-fluorescein isothiocyanate (FITC) Apoptosis Assay Kit (C1062) were purchased from Beyotime Biotechnology (Shanghai, China). Anti-STAT-3, anti-JAK2, anti-P-P53, anti-AKT, anti-C3, anti-β-actin, anti-rabbit lgG secondary antibody, and anti-mouse IgG secondary antibodies for western blotting were from Cell Signaling Technology (Boston, USA). Fetal bovine serum (FBS) (10099) and Dulbecco’s modified Eagle’s medium (DMEM) (11965092) were from Gibco (New York, USA). Mitochondrial membrane potential Assay Kit (JC-1) (MAK160) was purchased from Sigma (MO, USA).
Preparation and characterization of SeNPs
SeNPs@LNT was prepared by Professor Chen Tianfeng team at Jinan University. Data for the synthesis and characterization of nano-selenium can be obtained from an earlier publication (Zorov et al., 2014).
The Leydig cell line TM3 was isolated from mouse stromal cells and obtained from American Type Culture Collection. Cells were maintained in DMEM supplemented with 10% FBS, streptomycin (100 μg/mL), and penicillin (100 U/mL). The cells were treated with 0, 125, 250, 500, 600, 700, 800, and 1000 μM H2O2 for 8 h to observe the cell viability and screen out the appropriate concentration of oxidative stress. The concentration of 800 μM of H2O2 was selected for the treatment according to the standard of IC50, which caused a decline in cell viability but did not cause complete necrosis. The Leydig cell line TM3 was treated with SeNPs@LNT at 1, 2, 4, 8, 16 and 32 μM for 24 h to observe the cytotoxicity of selenium. The cell viability was the strongest at 4 μM of SeNPs@LNT, and the cells were treated with 4 μM intervention concentration. TM3 cells in the logarithmic growth phase were cultured for 12 h, and the solution was changed after observing adherence. TM3 cells were randomly divided into the control group, H2O2 group, SeNPs group, and H2O2+SeNPs group. For the H2O2+SeNPs group, TM3 cells were pretreated with SeNPs@LNT 4 μM for 12 h, followed by H2O2 800 μM for 8 h (Pi et al., 2013).
Untreated TM3 cells were plated in 96-well plates at a concentration of 5 × 104/mL. TM3 cells in 96-well plates were incubated overnight at 37°C. TM3 cells in each group, as described above, were treated with 100 μL CCK-8 solution in a 96-well plate and incubated for 1 h. The absorbance at 470 nm was read with a microplate reader (Varioskan Flash, Thermo, Waltham, USA). Cells from four different experiments were analyzed for each treatment.
Detection of mitochondrial membrane potential (∆Ψm)
TM3 cells were cultured in a 6-well microtiter plate with a cell density of 5 × 104/mL for 24 h according to different treatment groups. After adding 1 mL of cell culture medium, 1 mL of JC-1 staining working solution was added, and the mixture was fully mixed. The cells were incubated at 37°C for 20 min; then, the supernatant was removed by aspiration and washed twice with JC-1 staining buffer. Cell culture medium, which may contain serum and phenol red, was added, and the mitochondrial membrane potential was examined under a fluorescence microscope (Leica, Wetzlar, German).
Intracellular active oxygen levels
TM3 cells were cultured on a 96-well droplet plate at a density of 5 × 104/mL for 24 h. For the nano-selenium and H2O2 treatment group: TM3 cells were pretreated with 4 μM nano-selenium for 12 h and treated with 800 μM H2O2 for 8 h. Then, the cells were stained with 10 μM DCFH-DA for 0.5 h. Two techniques, including microscopy and fluorometry, were used to measure intracellular ROS levels. Images were collected by fluorescence microscope (Leica, Wetzlar, German) in each group at the same exposure time. Fluorescence intensity of 488 nm excitation and 525 nm emission was measured with a fluorescence plate reader (Thermo Fisher Scientific, Waltham, USA).
Apoptosis detection by annexin V-fluorescein isothiocyanate
TM3 cells were cultured in 6-well plates at a concentration of 5 × 104/mL. After oxidative stress induction and drug treatment, the culture medium was aspirated and washed once with PBS. For in situ fluorescence detection of adherent cells, 195 μL Annexin V-FITC binding solution was added and mixed gently. Then, 10 μL propidium iodide staining solution was added, mixed well, and incubated in the dark for 10–20 min at room temperature (20°C–25°C) in an ice bath. Aluminum foil can be used to protect from light. Cell assays were performed as soon as possible after staining, usually within 1 h.
TM3 cells were cultured on a 12 cm cell culture dish at a density of 8 × 104/mL according to different treatments for 24 h. TM3 cells were treated in different ways, as mentioned above, and placed in a lysis buffer to obtain cellular proteins. Protein concentration was determined by the BCA method. For loading, 40 μg of the sample was resolved by SDS-PAGE and transferred to a nitrocellulose membrane under the appropriate voltage. After that, TBSE buffer containing Tween-20, TrIS-Base, and 10× TBS (pH 7.4) was sealed with skim milk for 1 h. The primary antibody was diluted at 1:1000, and an appropriate amount of primary antibody was added to drown the PVDF membrane, and the reaction was conducted overnight at 4°C. After that, it was incubated with a secondary antibody for 1–2 h. Enhanced chemiluminescence reagents were used for X-ray development (Clinx, Shanghai, China).
Construction of oxidative stress model
TM3 cells were treated with concentration gradients of 0, 125, 250, 500, 600, 700, 800, and 1000 μM H2O2 for 8 h. After the induction of oxidative stress, cell viability after treatment with each concentration was detected by CCK-8, and the results are shown in Fig. 1A. With the increase in the concentration of H2O2, the cell viability gradually decreased. When the concentration was 800 μM, the cell viability was 58.81% (p < 0.0001), and the difference was statistically significant. The concentration of 800 μM was selected as the concentration of the H2O2 treatment according to the standard of IC50, which caused cell viability decline but not complete necrosis.
Figure 1: Effect of lentinan-functionalized nano-selenium particles (SeNPs@LNT) on H2O2-induced TM3 cells. (A) TM3 cells were exposed to increased concentrations of H2O2 (from 0 to 1000 μM) for 8 h. (B) TM3 cells were exposed to increased concentrations of SeNPs@LNT (from 0 to 32 μM) for 12 h. (C) TM3 cells were treated with 4 μM SeNPs for 12 h before being challenged by 800 μM H2O2 for 8 h. Cell viability was determined by CCK-8. (D) The TM3 cell was detected under a fluorescent microscope as a bright field. All data were presented as mean ± SD of three separate experiments. *p < 0.05, **p < 0.01, ***p < 0.001, ****p < 0.0001. The results were obtained from three repeated experiments.
Characteristics and cytotoxicity of SeNPs@LNT
The synthesis and characterization data of nano-selenium can be referred to in this article (Zorov et al., 2014). The results of treating cells with different concentration gradients of nano-selenium for 12 h are shown in Fig. 1B. In the set concentration gradient range, the cell viability gained a certain degree of peak after nano-selenium treatment. When the concentration was 4 μM, the cell viability was 154.00% (p < 0.001), and the difference was statistically significant. This concentration was chosen as the concentration of drug treatment. The cell viability did not increase significantly when the concentration was increased after treatment with 4 μM of SeNPs@LNT, and the cell density under a microscope was consistent with Fig. 1B. Compared to the inorganic and organic selenium as controls, nano-selenium had less cytotoxicity.
Antioxidant and cell proliferation effects of nano-selenium in vitro
The cell viability of the normal control group and oxidative stress group after 12 h of nano-selenium pretreatment was shown in Fig. 1C. The cell viability of the oxidative stress group was 62.71%, which was significantly different from the control group (p < 0.0001). The cell viability of the H2O2+SeNPs group was 97.79%, which was statistically significant compared to the H2O2 group (p < 0.0001). The cell viability of the SeNPs group was 108.56%, which was statistically significant with the oxidative stress group and the H2O2+SeNPs group. The microscopic results of the cells are shown in Fig. 1D. In the oxidative stress group, the refractive ability of the cells increased, the shape of the cells became round, and some cells showed apoptosis and necrosis.
SeNPs@LNT inhibited the decrease of mitochondrial membrane potential induced by hydrogen peroxide
JC-1 kit was used to detect the mitochondrial membrane potential level of each treatment group, and the results are shown in Fig. 2. In the nano-selenium treatment group, the membrane potential was higher, JC-1 aggregated in the mitochondrial matrix, forming polymers, and the red fluorescence level was stronger. However, the level of JC-1 monomer in the oxidative stress treatment group (H2O2 group) was higher, and green fluorescence was detected by fluorescence microscopy.
Figure 2: The mitochondrial membrane potential of the TM3 cell was detected with JC-1 fluorescent probe under a fluorescent microscope. The ratio of JC-1 monomer and polymer (red and green fluorescence) in different treatment groups was obvious. The results were obtained from three repeated experiments.
Selenium nanoparticles can be targeted to mitochondria to play their biological role. Mitochondria are key organelles of energy metabolism and ROS production, which trigger the induction of mitochondrial permeability transition pore (mPTP). This triggering phenomenon is the response of mitochondria to the amplification of oxidative stress ROS signal. The plasticity of mitochondrial structure and function is important in maintaining cell homeostasis. Damage to their function causes the accumulation of ROS and triggers inflammation (Liochev, 2013; Zhang et al., 2016). The subsequent detection of ROS revealed that oxidative stress caused the accumulation of ROS. According to relevant reports, selenium nanoparticles (SeNPs) synthesized by Lactobacillus casei ATCC 393 can alleviate H2O2-induced intestinal epithelial barrier dysfunction, reduce ROS production, and reduce mitochondrial membrane potential through the mitochondrial pathway (Yan et al., 2021). Liu et al. (2017) showed that PC-SeNPs could significantly improve mitochondrial fragmentation in their study on the reversal of palmitate-induced apoptotic uptake by phycocyanin functionalized selenium nanoparticles in pancreatic β-cells (Liu et al., 2017).
The production of reactive oxygen species was inhibited by SeNPs@LNT
The quantitative and fluorescence results of detecting intracellular ROS levels are shown in Figs. 3A and 3B. The intracellular ROS level in the oxidative stress group was 162.59% (p < 0.0001), and the intracellular ROS level in the H2O2+SeNPs group was 65.00% (p < 0.0001). There was no significant increase in ROS in the control group and nano-selenium treatment group.
Figure 3: Effect of lentinan-functionalized nano-selenium particles (SeNPs@LNT) on H2O2-induced TM3 cells on the production of reactive oxygen species (ROS). TM3 cells were treated with 4 μM SeNPs@LNT for 12 h before being challenged by 800 μM H2O2 for 8 h. ****p < 0.0001. The results were obtained from three repeated experiments.
The results of fluorescence data showed that green fluorescence increased in the oxidative stress group, and nano-selenium treatment could reduce the increase of ROS level caused by H2O2.
Regulating oxidative stress can initiate different cellular responses, from signaling pathways that trigger cell protection to initiating coordinated activation of mitochondrial fission and autophagy to optimizing the clearance of abnormal mitochondria and cells to protect the spread of damage to neighboring mitochondria and cells. The redox state of the cell environment is mainly determined by the ratio of reduction/oxidation cofactors to proteins that carry a large number of redox-sensitive amino acid residues and functional groups, NAD(P)H/NAD(P), and reduced glutathione and oxidized glutathione (GSH/ GSSG), which together form a compartmentalized redox buffer in which all components are in redox equilibrium under cell homeostasis conditions. When stressed, ROS induced by H2O2 can affect metabolic pathways through glycolytic pathways, oxidative phosphorylation, and lipid metabolism (lipolysis and lipogenesis). They act on mitochondria, endoplasmic reticulum, and cytoplasmic networks of various second messengers, as well as antioxidases (superoxide dismutase SOD, glutathione peroxidase) to change the intracellular environment (Tsubata, 2020). Tavoosi et al. (2020) treated H2O2-induced pancreatic beta-cells with cerium and yttrium oxide radionuclides and nano-selenium, and their intracellular ROS levels were significantly reduced. Xu et al. (2019) used Lactococcus lactis NZ9000 to biosynthesize potan-capped selenium carriers, SeNPs, that can inhibit the increase in ROS, the decrease in adenosine triphosphate, and the decrease in mitochondrial membrane potential in H2O2-exposed IPEC-J2 cells (Xu et al., 2019). Studies on the anti-tumor effect of nano-selenium have reported that modified nano-selenium particles can localize in mitochondria and trigger changes in ROS through endocytosis. As a signaling medium involved in cell growth, progression, and death, ROS has a role in the regulation of cell physiology (Su et al., 2019). It contains superoxide radicals, hydroxyl radicals, and singlet oxygen, which can destroy cell proteins, lipids, and nucleic acids. At the same time, the generation of ROS stimulates the activation of various intracellular signaling pathways, such as the P53 pathway, the mitogen-activated kinase pathway, the phosphatidylinositol 3-kinase/protein kinase B pathway, etc.
SeNPs@LNT reduced apoptosis and necrosis induced by oxidative stress
As shown in Fig. 4, apoptotic cells were stained only with green fluorescence, while the necrotic cells were stained with red and green double stains, and normal cells did not stain with any fluorescence. Under a fluorescence microscope, the oxidative stress group showed increased necrosis and apoptotic cells, and the degree of necrosis was less after nano-selenium treatment. There was no obvious apoptosis and necrosis in the control and the nano-selenium groups.
Figure 4: Under the fluorescence microscope, Annexin V-fluorescein isothiocyanate staining shows green fluorescence in early apoptotic cells, and propidium iodide staining shows red fluorescence in necrotic cells and late apoptotic cells. Apoptosis and necrosis in different treatment groups could be observed. The results were obtained from three repeated experiments.
SeNPs@LNT prevented oxidative stress-induced cell damage through the Janus kinase 2/signal transducer and activator of transcription 3 signaling pathway and P53 signaling pathway
Proteins related to cellular pathways activated by SeNPs@LNT are shown in Fig. 5. Oxidative stress induced the decrease in STAT-3 expression and C3 protein level and increased the expression of P-P53 protein. SeNPs@LNT enhanced the expression of JAK2 and decreased the expression of AKT protein. A simulation diagrams for the relevant mechanisms is shown in Fig. 6. Oxidative stress is also an important factor in inducing programmed cell death. We observed the production of ROS, the initiation of apoptosis, and changes in JC-1. The production of mitochondrial ROS causes dysfunction of the electron transport chain and disrupts the regulation of energy production. Most apoptotic signals originate from mitochondria and are regulated in part by members of the Bcl-2 family of pro-apoptotic (Bax) and anti-apoptotic (Bcl-2, Bcl-xL) proteins, which control mitochondrial transmembrane potential. Various stimuli, including oxidative stress, can activate mitochondrial endogenous apoptotic pathways. Concurrently, we observed changes in STAT-3, JAK2, and P-P53 proteins.
Figure 5: The signaling pathways activated by lentinan-functionalized nano-selenium particles (SeNPs@LNT) treatment. The TM3 cells exposed to different treatments expressed the pro-apoptotic and anti-apoptotic protein tracked by western blotting. β-actin was used as the loading control. The results were obtained from three repeated experiments.
Figure 6: Proposed signaling pathway triggered by lentinan-functionalized nano-selenium particles (SeNPs@LNT) and H2O2 inTM3 cells.
JAK2/STAT-3 pathway is a signaling pathway related to immune regulation, cell proliferation, differentiation, apoptosis, and hematopoiesis (O’Shea and Plenge, 2012). STAT is phosphorylated by JAK; it is then dimerized, transferred to the nucleus through the nuclear membrane, where it acts on DNA, and regulates the expression of related genes (Yu et al., 2009). A variety of cytokines and growth factors transmit signals through the JAK/STAT signaling pathway, including interleukin 2–7, epidermal growth factor, platelet-derived growth factor, and interferon (Boengler et al., 2008). Tyrosine kinase-associated receptors on cell membranes to receive signals from cytokines and growth factors. To some extent, the activation of this pathway reflects the changes in DNA methylation, the degree of cellular inflammation, and the expression of other cellular mediators (Xin et al., 2020). Its downstream target genes encode transcribed cytokines and growth factors that are further involved in the physiological functions of cells.
Concurrently, we observed that SeNPs@LNT changed the level of P-P53 protein in TM3 cells. P-P53, as a phosphorylated form of the P53 molecule, can be induced to activate by many stress signals, such as DNA damage, oncogene activation, and nutritional deprivation. It is mainly involved in cell metabolism, antioxidant, and DNA damage repair. In the regulation of the cell cycle and cell apoptosis, P53 may induce a large number of genes involved in the execution of the signal, including executing death receptor (Fas, Dr4), apoptosis factor, and BH3 domain to promote apoptosis protein structure to activate the extrinsic apoptotic pathway, and on the outer membrane of mitochondria permeability transition induced membrane hole open, causing changes in the level of membrane potential (Chen, 2016). The serine/threonine kinase AKT, as a proto-oncogene, is activated by extracellular signals (mainly growth factors via P13K signaling) and is involved in cell survival and inhibition of apoptosis, as well as tumor progression (Zhao et al., 2017). AKT is involved in the accumulation of ROS by stimulating oxidative metabolism and promoting mitochondrial oxygen consumption. We found that nano-selenium treatment reduced the level of AKT molecule to a certain extent, but the oxidative stress treatment (H2O2 group) did not stimulate its production, and this may be related to the degree of oxidative stress. As a part of innate immunity, C3 is involved in the clearance of pathogens and damaged cells in opsonization, leading to the coating of pathogens with antibodies or complement proteins to promote the phagocytosis of debris with foreign pathogens (Copenhaver et al., 2019). The cascade activation of its products initiates three effector pathways, inflammation, phagocytosis, and membrane attack, which carry out the coordinated defense of the organism (Ricklin and Lambris, 2016). The treatment with nano-selenium up-regulated the expression of C3, and the effect of oxidative stress on the decline of cellular immune function could be seen.
The sperm characteristics, DNA integrity, chromatin quality, antioxidant enzyme levels, testosterone concentrations, and testicular histomorphology were assessed in a study of germ cell damage induced by the anti-cancer drug cisplatin with nano-selenium particles, describing the concomitant antioxidant and reproductive stimulatory effects of selenium-based preparations (Rezvanfar et al., 2013). In fact, the results of several studies are consistent with the conclusions of this study.
In this study, functionalized nano-selenium was used to regulate cell function through the JAK2/STAT-3 pathway, P53 pathway, AKT, C3 molecules, etc., acting on mitochondria, changing their membrane potential level, and alleviating the apoptosis and necrosis of TM3 cells against the elimination of ROS. The detection of cytokines and antioxidant enzymes was not carried out. The specific path of nano-selenium entering the cells could be further determined by fluorescence. The status of other organelles in the cells was not detected. As a new kind of drug, nanomaterials have attracted extensive attention in tumor immunity, antiviral and antioxidant properties, the treatment of nervous system diseases, and so on. Nanomaterials have the characteristics of strong targeting and high bioavailability. How nano-selenium drugs participate in the regulation of signaling pathway molecules and the expression of cytokines is worthy of further investigation.
Funding Statement: This study was funded by Science and Technology Projects in Guangzhou (202201020632, 202201020638, 202206010100, 202201020655, and 202102010202), the Guangdong Natural Science Foundation (2020A1515110648), the Open Project of Guangdong Key Laboratory of Marine Materia (LMM2020-7), the Open Fund of Guangdong Provincial Key Laboratory of Functional Supramolecular Coordination Materials and Applications (2020A03), and the Guangzhou Medical University Students’ Science and Technology Innovation Project (2021AEK119 and 02-408-2203-2079).
Author Contributions: Miaomiao Li and Zilin Zheng designed the study and analyzed the experimental data. Junyi Ke and Jieyi Luo drafted the manuscript. Fan Jiang and Yanxia Qu carried out the experiments. Bing Zhu, Yinghua Li, and Liandong Zuo refined the manuscript and coordinated the study, were responsible for the laboratory management, reviewed and revised articles, and confirmed the final draft. All authors read and approved the final manuscript.
Availability of Data and Materials: Data sharing is not applicable to this article as no datasets were generated or analyzed during the current study.
Ethics Approval: Not applicable.
Conflicts of Interest: The authors declare that they have no conflicts of interest to report regarding the present study.
References
Andersen OM, Yeung CH, Vorum H, Wellner M, Andreassen TK et al. (2003). Essential role of the apolipoprotein E receptor-2 in sperm development. Journal of Biological Chemistry 278: 23989–23995. https://doi.org/10.1074/jbc.M302157200 [Google Scholar] [PubMed] [CrossRef]
Banerjee S, Ghosh S, Mandal A, Ghosh N, Sil PC (2020). ROS-associated immune response and metabolism: A mechanistic approach with implication of various diseases. Archives of Toxicology 94: 2293–2317. https://doi.org/10.1007/s00204-020-02801-7 [Google Scholar] [PubMed] [CrossRef]
Boengler K, Hilfiker-Kleiner D, Drexler H, Heusch G, Schulz R (2008). The myocardial JAK/STAT pathway: From protection to failure. Pharmacology & Therapeutics 120: 172–185. https://doi.org/10.1016/j.pharmthera.2008.08.002 [Google Scholar] [PubMed] [CrossRef]
Boitani C, Puglisi R (2008). Selenium, a key element in spermatogenesis and male fertility. Advances in Experimental Medicine and Biology 636: 65–73. https://doi.org/10.1007/978-0-387-09597-4 [Google Scholar] [CrossRef]
Chen J (2016). The cell-cycle arrest and apoptotic functions of p53 in tumor initiation and progression. Cold Spring Harbor Perspectives in Medicine 6: a026104. https://doi.org/10.1101/cshperspect.a026104 [Google Scholar] [PubMed] [CrossRef]
Copenhaver M, Yu CY, Hoffman RP (2019). Complement components, C3 and C4, and the metabolic syndrome. Current Diabetes Reviews 15: 44–48. https://doi.org/10.2174/1573399814666180417122030 [Google Scholar] [PubMed] [CrossRef]
Hariharan S, Dharmaraj S (2020). Selenium and selenoproteins: It’s role in regulation of inflammation. Inflammopharmacology 28: 667–695. https://doi.org/10.1007/s10787-020-00690-x [Google Scholar] [PubMed] [CrossRef]
Hosnedlova B, Kepinska M, Skalickova S, Fernandez C, Ruttkay-Nedecky B et al. (2018). Nano-selenium and its nanomedicine applications: A critical review. International Journal of Nanomedicine 13: 2107–2128. https://doi.org/10.2147/IJN [Google Scholar] [CrossRef]
Labunskyy VM, Hatfield DL, Gladyshev VN (2014). Selenoproteins: Molecular pathways and physiological roles. Physiological Reviews 94: 739–777. https://doi.org/10.1152/physrev.00039.2013 [Google Scholar] [PubMed] [CrossRef]
Liochev SI (2013). Reactive oxygen species and the free radical theory of aging. Free Radical Biology and Medicine 60: 1–4. https://doi.org/10.1016/j.freeradbiomed.2013.02.011 [Google Scholar] [PubMed] [CrossRef]
Liu C, Fu Y, Li CE, Chen T, Li X (2017). Phycocyanin-functionalized selenium nanoparticles reverse palmitic acid-Induced pancreatic beta cell apoptosis by enhancing cellular uptake and blocking reactive oxygen species (ROS)-mediated mitochondria dysfunction. Journal of Agricultural and Food Chemistry 65: 4405–4413. https://doi.org/10.1021/acs.jafc.7b00896 [Google Scholar] [PubMed] [CrossRef]
Liu HJ, Qin Y, Zhao ZH, Zhang Y, Yang JH et al. (2020). Lentinan-functionalized selenium nanoparticles target tumor cell mitochondria via TLR4/TRAF3/MFN1 pathway. Theranostics 10: 9083–9099. https://doi.org/10.7150/thno.46467 [Google Scholar] [PubMed] [CrossRef]
Liu Y, Zhao J, Zhao Y, Zong S, Tian Y et al. (2019). Therapeutic effects of lentinan on inflammatory bowel disease and colitis-associated cancer. Journal of Cellular and Molecular Medicine 23: 750–760. https://doi.org/10.1111/jcmm.13897 [Google Scholar] [PubMed] [CrossRef]
Maiorino M, Conrad M, Ursini F (2018). GPx4, lipid peroxidation, and cell death: Discoveries, rediscoveries, and open issues. Antioxidants & Redox Signaling 29: 61–74. https://doi.org/10.1089/ars.2017.7115 [Google Scholar] [PubMed] [CrossRef]
Michaelis M, Gralla O, Behrends T, Scharpf M, Endermann T, Rijntjes E, Pietschmann N, Hollenbach B, Schomburg L (2014). Selenoprotein P in seminal fluid is a novel biomarker of sperm quality. Biochemical and Biophysical Research Communications 443: 905–910. https://doi.org/10.1016/j.bbrc.2013.12.067 [Google Scholar] [PubMed] [CrossRef]
Novak EA, Mollen KP (2015). Mitochondrial dysfunction in inflammatory bowel disease. Frontiers in Cell and Developmental Biology 3: 62. https://doi.org/10.3389/fcell.2015.00062 [Google Scholar] [PubMed] [CrossRef]
O’Shea JJ, Plenge R (2012). JAK and STAT signaling molecules in immunoregulation and immune-mediated disease. Immunity 36: 542–550. https://doi.org/10.1016/j.immuni.2012.03.014 [Google Scholar] [PubMed] [CrossRef]
Pi J, Jin H, Liu R, Song B, Wu Q, Liu L, Jiang J, Yang F, Cai H, Cai J (2013). Pathway of cytotoxicity induced by folic acid modified selenium nanoparticles in MCF-7 cells. Applied Microbiology and Biotechnology 97: 1051–1062. https://doi.org/10.1007/s00253-012-4359-7 [Google Scholar] [PubMed] [CrossRef]
Qazi IH, Angel C, Yang H, Zoidis E, Pan B et al. (2019). Role of selenium and selenoproteins in male reproductive function: A review of past and present evidences. Antioxidants 8: 268. https://doi.org/10.3390/antiox8080268 [Google Scholar] [PubMed] [CrossRef]
Rayman MP (2020). Selenium intake, status, and health: A complex relationship. Hormones 19: 9–14. https://doi.org/10.1007/s42000-019-00125-5 [Google Scholar] [PubMed] [CrossRef]
Rezvanfar MA, Rezvanfar MA, Shahverdi AR, Ahmadi A, Baeeri M, Mohammadirad A, Abdollahi M (2013). Protection of cisplatin-induced spermatotoxicity, DNA damage and chromatin abnormality by selenium nano-particles. Toxicology and Applied Pharmacology 266: 356–365. https://doi.org/10.1016/j.taap.2012.11.025 [Google Scholar] [PubMed] [CrossRef]
Ricklin D, Lambris JD (2016). Preformed mediators of defense-gatekeepers enter the spotlight. Immunological Reviews 274: 5–8. https://doi.org/10.1111/imr.12497 [Google Scholar] [PubMed] [CrossRef]
Su LJ, Zhang JH, Gomez H, Murugan R, Hong X, Xu D, Jiang F, Peng ZY (2019). Reactive oxygen species-induced lipid peroxidation in apoptosis, autophagy, and ferroptosis. Oxidative Medicine and Cellular Longevity 2019: 5080843. https://doi.org/10.1155/2019/5080843 [Google Scholar] [PubMed] [CrossRef]
Tavoosi S, Baghsheikhi AH, Shetab-Boushehri SV, Navaei-Nigjeh M, Sarvestani NN, Karimi MY, Ranjbar A, Ebadollahi-Natanzi A, Hosseini A (2020). Cerium and yttrium oxide nanoparticles and nano-selenium produce protective effects against H2O2-induced oxidative stress in pancreatic beta cells by modulating mitochondrial dysfunction. Pharmaceutical Nanotechnology 8: 63–75. https://doi.org/10.2174/2211738507666191002154659 [Google Scholar] [PubMed] [CrossRef]
Tsubata T (2020). Involvement of reactive oxygen species (ROS) in BCR signaling as a second messenger. Advances in Experimental Medicine and Biology 1254: 37–46. https://doi.org/10.1007/978-981-15-3532-1 [Google Scholar] [CrossRef]
Wadhwani SA, Shedbalkar UU, Singh R, Chopade BA (2016). Biogenic selenium nanoparticles: Current status and future prospects. Applied Microbiology and Biotechnology 100: 2555–2566. https://doi.org/10.1007/s00253-016-7300-7 [Google Scholar] [PubMed] [CrossRef]
Xin P, Xu X, Deng C, Liu S, Wang Y, Zhou X, Ma H, Wei D, Sun S (2020). The role of JAK/STAT signaling pathway and its inhibitors in diseases. International Immunopharmacology 80: 106210. https://doi.org/10.1016/j.intimp.2020.106210 [Google Scholar] [PubMed] [CrossRef]
Xu C, Qiao L, Ma L, Yan S, Guo Y, Dou X, Zhang B, Roman A (2019). Biosynthesis of polysaccharides-capped selenium nanoparticles using lactococcus lactis NZ9000 and their antioxidant and anti-inflammatory activities. Frontiers in Microbiology 10: 1632. https://doi.org/10.3389/fmicb.2019.01632 [Google Scholar] [PubMed] [CrossRef]
Yan S, Qiao L, Dou X, Song X, Chen Y, Zhang B, Xu C (2021). Biogenic selenium nanoparticles by Lactobacillus casei ATCC 393 alleviate the intestinal permeability, mitochondrial dysfunction and mitophagy induced by oxidative stress. Food & Function 12: 7068–7080. https://doi.org/10.1039/D0FO03141K [Google Scholar] [PubMed] [CrossRef]
Yu H, Pardoll D, Jove R (2009). STATs in cancer inflammation and immunity: A leading role for STAT3. Nature Reviews Cancer 9: 798–809. https://doi.org/10.1038/nrc2734 [Google Scholar] [PubMed] [CrossRef]
Zhang J, Wang X, Vikash V, Ye Q, Wu D, Liu Y, Dong W (2016). ROS and ROS-mediated cellular signaling. Oxidative Medicine and Cellular Longevity 2016: 4350965. https://doi.org/10.1155/2016/4350965 [Google Scholar] [PubMed] [CrossRef]
Zhao Y, Hu X, Liu Y, Dong S, Wen Z, He W, Zhang S, Huang Q, Shi M (2017). ROS signaling under metabolic stress: Cross-talk between AMPK and AKT pathway. Molecular Cancer 16: 79. https://doi.org/10.1186/s12943-017-0648-1 [Google Scholar] [PubMed] [CrossRef]
Zi Y, Zhang B, Jiang B, Yang X, Liang Z, Liu W, He C, Liu L (2018). Antioxidant action and protective and reparative effects of lentinan on oxidative damage in HaCaT cells. Journal of Cosmetic Dermatology 17: 1108–1114. https://doi.org/10.1111/jocd.12488 [Google Scholar] [PubMed] [CrossRef]
Zorov DB, Juhaszova M, Sollott SJ (2014). Mitochondrial reactive oxygen species (ROS) and ROS-induced ROS release. Physiological Reviews 94: 909–950. https://doi.org/10.1152/physrev.00026.2013 [Google Scholar] [CrossRef]
Cite This Article
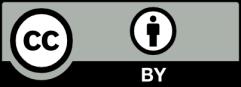