Open Access
ARTICLE
Exploration of the oxidative-inflammatory potential targets of Coicis Semen in osteoarthritis: Data mining and systematic pharmacology
1 Department of Rheumatism Immunity, The First Affiliated Hospital, Anhui University of Chinese Medicine, Hefei, 230031, China
2 Institute of Rheumatism Prevention and Treatment of Traditional Chinese Medicine, Anhui Academy of Chinese Medicine Sciences, Hefei, 230031, China
3 Graduate School, Anhui University of Chinese Medicine, Hefei, 230012, China
4 Department of Rheumatism Immunity, The Second Affiliated Hospital, Anhui University of Chinese Medicine, Hefei, 230061, China
* Corresponding Author: Jian Liu,
BIOCELL 2023, 47(7), 1623-1643. https://doi.org/10.32604/biocell.2023.028331
Received 14 December 2022; Accepted 20 March 2023; Issue published 21 June 2023
Abstract
Objective: On the basis of data mining, systematic pharmacology, molecular docking, and experiment validation, the oxidative-inflammatory molecular targets of Coicis Semen in the therapy of osteoarthritis (OA) were explored. Methods: The association rule analysis was effectively applied to highlight the correlation between Coicis Semen and oxidative inflammation indices. The random walk model was subsequently used to evaluate the clinical efficacy of Coicis Semen. Network pharmacology was used to predict network targets. The binding affinity of the active ingredient in Coicis Semen to the key target of OA was also successfully predicted. Results: Coicis Semen showed a significant reduction in oxidative-inflammatory indicators of OA. A total of 108 promising targets were predicted for the 24 bioactive compounds in Coicis Semen. Eight target genes were considered core target genes. The enrichment analysis predicts that Coicis Semen may activate the interleukin (IL)-17, mitogen-activated protein kinase (MAPK), and nuclear factor kappa B (NF-kappa B) signaling pathways. Molecular docking demonstrated that stigmasterol, 2-monoolein, sitosterol, and sitosterol alpha1 had free binding energies to oxidative and inflammatory targets (MAPK1, Estrogen Receptor 1 [ESR1], and Peroxisome Proliferator-Activated Receptor Alpha [PPARA]). Both clinical trials and in vitro cell experiments revealed that Coicis Semen could increase ESR1 and PPAR-α levels while decreasing MAPK1 levels. Conclusions: Coicis Semen has a remarkable anti-OA effect. Precisely, the major components of Coicis Semen, including stigmasterol, sitosterol alpha1, sitosterol, and 2-monoolein, specifically inhibit MAPK1, ESR1, and PPARA to reduce the inflammatory response and oxidative damage in OA.Keywords
Supplementary Material
Supplementary Material FileGlobally, osteoarthritis (OA) affects a large portion of the elderly due to chronic degenerative changes of the cartilage tissue in the joints (Hodgkinson et al., 2022). With a higher aging rate in China, OA has increasingly become a major problem interfering with the living quality of middle-aged and elderly people, causing a large economic burden to patients, their families, and society (El-Tawil et al., 2016). Current OA treatments are primarily palliative, aiming to alleviate symptoms while also including exercise, analgesics, weight control, and intra-articular drug injection (Ringdahl and Pandit, 2011; van Spil et al., 2019). Complementary and alternative drugs assume even greater urgency in the selection of drug therapies. Chinese herbal medicine (CHM) has a long history and is unique for OA, which has certain advantages for the overall management of the course. As a result of the application of evidence-based medicine, numerous randomized controlled trials, as well as systematic reviews, have assessed the safety and effectiveness of CHM for treating osteoarthritis (Yang et al., 2021; Si et al., 2018).
There is a large volume of ancient Chinese book materials describing the role of CHM in the treatment of arthritis, with slight side effects (Cao et al., 2021; Chen et al., 2016; Wang et al., 2020a). The source of Coicis Semen is the ripe, dry seed of Coicis Semen. L, which is widely consumed as food or medicine in Asian countries. The main effects of Coicis Semen include detoxification, dampness, and the removal of arthralgia. Coicis Semen and herbal prescriptions containing Coicis Semen have anti-inflammatory functions (e.g., inflammatory cytokines IL-1, tumor necrosis factor [TNF], matrix metalloproteinase-3 [MMP-3], MMP-13, to name a few) and joint-protective effects (Guo et al., 2012; Shen et al., 2019; Xu et al., 2013).
Network pharmacology integrates the CHM component network with an organism’s biological target network, and shows the traits of the multi-target, multi-component, and multi-signal pathway of CHM through the “molecule target” network characteristic map (Tang et al., 2020; Wang et al., 2021). The field of data mining in medical research has been at the forefront of research as it has demonstrated excellent performance in analyzing data and assisting clinical decisions (Wu et al., 2021). Based on the strengths of data mining, this research was designed to probe the association rules and the clinical efficacy role of Coicis Semen in OA. Furthermore, we attempted to elucidate the bioactive components and targets of Coicis Semen acting on OA. To reveal the potentially useful components and molecular mechanism of Coicis Semen in OA, molecular docking technology was employed for the enrichment study between the drug-active compounds and target protein molecules. To additionally verify the mechanism of action, the confirmed efficacy of Coicis Semen on OA was also demonstrated experimentally.
Acquisition of clinical data and analysis of association rules
Detailed data on patients with osteoarthritis admitted to the Department of Rheumatology and Immunology of the First Affiliated Hospital of the Anhui University of Chinese Medicine was collected and analyzed, over the period from September 2009 to May 2022. The herbal prescriptions and disease-associated laboratory indices, such as the inflammatory markers: C-reactive protein (CRP) and erythrocyte sedimentation rate (ESR), the immune indices: immunoglobulin A (IgA), immunoglobulin M (IgM), immunoglobulin G (IgG), complement component 3 (C3) and complement component 4 (C4), and the oxidation index: superoxide dismutase (SOD) recorded in electronic medical record systems were routinely abstracted for participants. The research protocol and procedures have been approved by the Ethics Committee of the First Affiliated Hospital of Anhui University of Chinese Medicine (Review No. 2022MCZQ01). This study is a retrospective data mining study based on hospital information systems. Because the system uses secondary level identification to identify information, patient informed consent was not required. In total, 12,842 patients with OA were examined, including 12,226 who received herbal remedies.
Patients were divided into an experimental group (including Coicis Semen in herbal prescriptions) and a control group (not including Coicis Semen in herbal prescriptions). The control group had 3,992 cases, separately, and the experimental group had 8,234 cases.
Association Rule. The compatibility rules of the Coicis Semen/indicators were extracted by SPSS Clementine v.11.1 (IBM Corp., Armonk, NY, USA). Nominations for the application of herbs or indicators were assigned 1, while nominations for non-use are assigned 0. An association rule between X and Y has three degrees of support, confidence, and lift, respectively (Ding et al., 2020). The specific calculation formula of association rules was as documented in previous studies (Fang et al., 2022b; Zhou et al., 2021).
Propensity score matches. The propensity score for each individual was calculated based on factors such as age, sex, body mass index (BMI), length of hospital stays (LOS), and the underlying disease to reduce the bias in treatment selection. The patients in the two groups were precisely matched using the method of propensity score as elucidated by Hanaya Raad and Victoria Cornelius (Raad et al., 2020).
Random walking. The lab-indexed random walk model was evaluated with the ORACLE 10 g tool. The random walk model had long-range correlations, which clearly implies that immuno-inflammatory indicators were valid. The principle and formula of random walking were first proposed by Karl Pearson (Pearson, 1905). Subsequently, it has also been flexibly used in the evaluation of medical clinical data (Fang et al., 2022a; Li et al., 2022; Zhou et al., 2021). The entailed detailed calculation formula is based on previous research (Zhou et al., 2021).
Screening active compounds and corresponding targets
The Traditional Chinese Medicine Systems Pharmacology Database served as the source for the collection of all the composite parts of Coicis Semen. To draw additional conclusions, only compounds that met the criteria suggested by the TCMSP database, such as oral bioavailability (OB) ≥ 30 and drug-likelihood (DL) ≥ 0.18, were chosen as candidate compounds (Chen, 2011). Further, among the compounds with OB < 30 or DL < 0.18, which were explored with “compound (name)” and “arthritis” [all fields] in PubMed databases to discover relevant research, the compounds in purified form centered on anti-OA mechanisms were also regarded as bioactive compounds and included for further study. The chemical structures were drawn by ChemDraw (version 14.0). The underlying target genes of Coicis Semen were then aggregated via the TCMSP platform and selected for the human species. The Coicis Semen target was obtained from the UniProt database.
Screening of predictive targets for OA and co-disease targets
Information on OA-associated target genes was screened and surveyed by the following electronic databases: GeneCards, PHARMGKB, OMIM, TTD, DrugBank, DisGeNET, and MalaCards. We used the UniProt database to perform target-human gene name conversion for disease targets and delete duplicate targets, which were searched using the keyword “osteoarthritis.” The results of the seven databases analysis were summarized, integrated, and deduplicated to obtain OA-related targets. Venn plots were drawn using the Venny online tool to identify the active ingredient targets and the disease targets for OA. Subsequently, as a result of the intersection of Coicis Semen and OA, the latent target set of Coicis Semen for treating OA was identified.
Construction of the Coicis Semen active ingredients and disease target network
To set up the “Coicis Semen active component-disease target network” diagram, the screening results were inputted into Cytoscape software (version 3.7.2) (Piñero et al., 2021). Cytoscape can analyze and construct network graphs, and it can set essential parameters including volume, color, shape, etc. The connections between nodes in the network reflect the interactions between them. The higher the degree value, the more nodes are connected to it (Huang et al., 2009).
Protein-protein interaction network construction and analysis
Protein-protein interaction (PPI) relates to the physical binding of two or more proteins in response to different disturbances and circumstances (Watanabe et al., 2021). A protein interaction network diagram was created with a species that qualified as “Homo sapiens”, at a confidence level of 0.400, and hidden disconnected nodes in the network. Our next step was to evaluate the topological properties of the nodes in the interaction network using the CytoNCA plug-in (Tang et al., 2015): “degree centrality (DC),” “closeness centrality (CC),” “betweenness centrality (BC),” “network centrality (NC),” “eigenvector centrality (EC),” and “local average connectivity (LAC).” We gained the core objectives of the network by measuring the value and nature of nodes, which are measured by the six above parameters (Wood et al., 2008). The higher a node is, the greater its importance.
Gene ontology enrichment analysis and kyoto encyclopedia of genes and genomes pathway analysis
We used R (Rx64, 3.6.3), and the biological molecular functional annotation system DAVID (https://david.ncifcrf.gov/), to have access to the Coicis Semen treatment of osteoarthritis target set enrichment analysis. Statistical significance was determined by Fisher’s test using p values < 0.05 and q values < 0.05.
Molecular docking verification and clinical experimental validation
The PDB database was searched by limiting the organism to “Homo sapiens only” to retrieve 3D structures of osteoarthritis target genes. The 2D structure of the Coicis Semen active component was retrieved, and the structure was optimized using Chem3D. AutoDock Tools, a visualization software, was applied to molecular docking. Eventually, the binding model and 3D visual analysis of the docking results were performed using the PyMOL software. LigPlot+ software demonstrated the 2D structure of protein-ligand molecular interactions (Schöning-Stierand et al., 2020).
A random sample of 30 patients with OA from the Department of Rheumatology and Immunology at the Anhui Provincial Hospital of Traditional Chinese Medicine was assigned to the experimental group (including Coicis Semen in herbal prescriptions) or the control group (not including Coicis Semen in herbal prescriptions). A total of 15 healthy donors were included in the healthy controls (HC) from the physical examination center. Details of the clinical samples are presented in Suppl. Table S1. The study protocol was approved by the Ethics Committee of the First Affiliated Hospital of Anhui University of Chinese Medicine (Review No. 2022MCZQ01). Informed consent was signed by all participants. Fasting blood was collected in the morning, cell culture supernatants were collected, centrifuged (1000 g, 4°C, 10 min) to remove cell debris, and then stored at −80°C until further quantification. Commercially available ELISA kits for PPAR-α (Genmei Technology Co., Ltd., Wuhan, China), ESR1 (Genmei Technology Co., Ltd., Wuhan, China) and MAPK1 (Genmei Technology Co., Ltd., Wuhan, China) were used to detect the expression according to the instructions in the kit.
Coicis Semen (100 g, S27205, MedChemExpress, Shanghai, China) was dissolved in 100 mM dimethyl sulfoxide (DMSO, Gibco, California, USA) to make a stock concentration. For the experiments, the required concentration of Coicis Semen was obtained by an additional dilution of the concentrated stock solution with culture media. The human chondrocytes C28/I2 (Haodi Huatuo Biotechnology Co., Ltd., Shenzhen, China) were cultured in complete Roswell Parker Memorial Institute (RPMI)-1640 medium (Gibco, California, USA) containing 10% fetal bovine serum (FBS, Gibco, California, USA) at 37°C with 5% CO2. Lipopolysaccharide (LPS, 10 µg/mL, Sigma-Aldrich, St. Louis, Missouri, USA) was utilized to stimulate C28/I2 cells to establish the model of OA in vitro (Jia and Wei, 2021). LPS has been documented as an initial factor in the regulation of inflammatory response during the pathogenesis of OA (Scotece et al., 2018).
The treatment in the study was divided into the normal control group (NC, normal cultured C28/I2 cells), model group (MC, treated with 10 μg/mL LPS for 12 h), Coicis Semen low-dose group (Coicis Semen-L, treated with 10 μg/mL LPS for 12 h, then treated with 25 μmol/L Coicis Semen for 24 h), Coicis Semen medium-dose group (Coicis Semen-M, treated with 10 μg/mL LPS for 12 h, treated with 50 μmol/L Coicis Semen for 24 h), and Coicis Semen high-dose group (Coicis Semen-H, treated with 10 μg/mL LPS for 12 h, treated with 100 μmol/L Coicis Semen for 24 h). The dosage of Coicis Semen was referred to as the in vitro experimental drug dosage on the MedChemExpress (MCE, Shanghai, China) official website and related literature studies (Du et al., 2021; Zhang et al., 2019a).
Cell treatment with radioimmunoprecipitation assay (RIPA) buffer (Biosharp, Beijing, China) containing protease and phosphatase inhibitors (Biosharp, Beijing, China) was performed to extract total proteins from C28/I2 cells. The primary antibodies used in this study included anti-β-actin (1:1000 dilution, Zs-BIO, Beijing, China), anti-ESR1 (1:1000 dilution, Affinity, Wuhan, China), anti-MAPK1 (1: 2000 dilution, Abcam, Cambridge, England), and anti-PPAR-α (1:2000 dilution, Affinity, Wuhan, China). The secondary antibodies included goat anti-rabbit IgG (1:5000 dilution, Zs-BIO, Beijing, China), anti-β-actin (1:10000 dilution, Zs-BIO, Beijing, China), anti-ESR1 (1:5000 dilution, Affinity, Wuhan, China), anti-MAPK1 (1:5000 dilution, Abcam, Cambridge, England), and anti-PPAR-α (1:5000 dilution, Affinity, Wuhan, China). The relevant instructions were referred to and finally the ECL hypersensitive luminescence kit (Beyotime, Shanghai, China) was used to detect each protein.
The statistical analyses were carried out with SPSS (version 22.0; IBM, NY, USA). Continuous variables were presented as the mean ± standard deviation, or medians and interquartile ranges (IQR). The nonparametric statistical analyses between the two groups were performed using the Wilcoxon signed-rank and Mann–Whitney U-test for paired and unpaired data, respectively. The Student’s t-test was used to compare data that was typically distributed. Categorical variables were expressed as numbers (%) and were compared using the χ2 test. Variations were recognized statistically as significant at p < 0.05. To analyze the variances and construct the images, GraphPad Prism 9.0 software (GraphPad, CA, USA) was used.
Analysis of the association rules between Coicis Semen and oxidative-inflammatory indices
Based on the analysis of the apriori module, each item is ranked with the highest level of confidence, with a minimum support of 60% and a minimum confidence level of 80%. The relationship between oxidative-inflammatory index optimization and Coicis Semen was analyzed using the association rules. We conclude that Coicis Semen with improved CRP has a confidence level of 89.80%. The improvement in confidence on the ESR was 85.73%, and on the SOD, it was 83.82%. At the same time, all lifts are greater than 1. The OA oxidative-inflammatory index showed substantial improvement with Coicis Semen treatment, as shown in Table 1.
Changes in oxidative-inflammatory indices after treatment with Coicis Semen
The baseline features for each group are compared before and after propensity score matching in Table 2. We used propensity score analysis to adjust for potential differences in the socio-demographics of patients in the two groups, including age, gender, and BMI. In the analysis of the two groups, comorbidities of interest (coronary heart disease, cerebral infarction, hypertension, chronic gastritis, hyperuricemia, osteoporosis, fatty liver) were significantly different, but that was comparable to that of the matched control group and the matched experimental group. The oxidative-inflammatory indices (ESR, IgA, SOD, CRP, C3, IgM, C4, IgG) were markedly greater in the experimental group than the control group (p < 0.05) but were comparable after propensity-score matching. Subsequently, it could be concluded that ESR, C3, IgA, CRP, IgG, and C4 were considerably reduced after treatment compared to pre-treatment. Compared to the control group, the experimental group had a significant reduction in ESR, C3, IgA, IgG, C4, CRP, and SOD after treatment (Table 3).
Evaluation of the oxidative-inflammatory index by a random walking model after Coicis Semen treatment
The random walking model showed that there was a positive correlation between Coicis Semen treatment and ESR levels, CRP levels, IgA levels, IgG levels, C3 levels, SOD levels, and C4 levels. It is not difficult to find from Table 4 that the experimental group also performs better than the control group in the walking evaluation of different laboratory indicators (ESR, CRP, IGA, IGG, SOD, C3, C4). Fig. 1 visually displays the walking trend of the oxidative-inflammatory index for both groups.
Figure 1: Random walking model of oxidative-inflammatory indices in OA patients after treatment with Coicis Semen.
Note: The green line indicates the experimental group. Then, the blue line indicates the control group. Walking steps are measured by the length of a horizontal line. Intervention effectiveness and response are measured by the height of the vertical line. ESR: erythrocyte sedimentation rate; CRP: C-reactive protein; IgA: immunoglobulin A; IgG: immunoglobulin G; C3: complement C3; C4: complement C4; SOD: superoxide dismutase.
Screening for active components of Coicis Semen
By January 2022, a total of 38 compounds were recognized in Coicis Semen. All of the identified compounds were filtered through Absorption, Distribution, Metabolism, and Excretion (ADME) screening, and a total of 24 active compounds met the criteria, accounting for 63.16% of all 38 compounds. The properties of the compounds are demonstrated in Table 5.
Prediction of target genes of osteoarthritis and their intersections with Coicis Semen
By searching GeneCards, OMIM, TTD, PharmGkb, Disgenet, Drugbank, and Malacards disease databases, we collected 1835, 6, 32, 9, 1827, 150, and 63 target genes related to OA, respectively (Fig. 2A, Suppl. Table S2). On an average, 2924 OA-related targets were obtained after removing duplicated genes. Overall, a Venn graph intersection of identified targets for Coicis Semen and OA (Fig. 2B) identified 61 target genes.
Figure 2: A Venn graph illustrating target genes that are osteoarthritis (OA)-related (A) and a Venn diagram illustrating the intersection between identified targets of screened compounds and OA (B).
“Herb-ingredient-target” network diagram construction
A “herb-ingredient-target” network diagram intuitively revealed the link between the ingredients of Coicis Semen and target genes related to OA-related genes (Fig. 3). The network comprised 130 nodes (108 active compounds and 21 targets) and 224 edges (degree > 1). As shown in Fig. 3, the red triangles represent Coicis Semen, the green rectangle represents the active chemical composition of Coicis Semen, the blue circles represent the target genes of OA, and the lines between them represent the relationship between chemical composition and OA target genes.
Figure 3: The “herb-ingredient-target” network of Coicis Semen in the treatment of OA.
Protein-protein interaction network and target protein interaction network analysis
An interaction network of 58 nodes and 190 edges (Fig. 4A) was constructed using CytoNCA based on DC, BC, CC, EC, NC, and LAC parameters. The thresholds for the first screening were LAC > 1.714, degree > 5, closeness > 0.137, eigenvector > 0.0626, betweenness > 15.638, and network > 3.05, and the results showed 22 nodes and 111 edges (Fig. 4B). Twenty-two targets were then screened further away. Next, the second screening threshold was degree > 10, eigenvector > 0.214, LAC > 5.8, betweenness > 8.746, closeness > 0.656, and network > 6.654. We obtained an EP300, JUN, ACTB, RELA, MYC, MAPK1, ESR1, and PPARA core PPI network with 8 nodes and 26 edges (Fig. 4C).
Figure 4: Protein-protein interaction (PPI) network construction and topology analysis (A). Screening of drug compound targets and osteoarthritis-related targets (B). The core PPI network obtained from B (C).
By inputting 61 key genes into the DAVID website, 1478 biological processes (BP), 73 cellular components (CC), and 76 molecular functions (MF) were acquired with a 0.05 threshold. These targets were primarily enriched in oxidative stress, inflammatory response, and multi-multicellular organism process in BP, as shown in Fig. 5A, including positive regulation of the inflammatory response (GO: 0050727), positive regulation of oxidative stress (GO: 0006979), regulation of multi-multicellular organism process (GO: 0044706), etc. In CC analysis, the cellular components of these key targets mainly were cytoplasmic vesicle lumen (GO: 0060205), vesicle lumen (GO: 0031983), protein-lipid complex (GO: 0032994), to name a few. The MF analysis predicted that these key targets are compactly connected with transcription coregulator binding activity (GO: 0001221), nuclear receptor activity (GO: 0004879), etc.
Figure 5: Gene Ontology (GO) analysis of targets, the top 10 significant enrichment terms in biological processes (BP), cellular components (CC), and molecular functions (MF) (A). Note: The y-axis displays the enrichment counts of these terms. The x-axis shows the enrichment terms, respectively. Top 30 Kyoto Encyclopedia of Genes and Genomes (KEGG) enrichment pathways (B). Note: The y-axis displays the top 30 significantly enriched pathways. The redder the color, the smaller is the p-value. The x-axis represents the target gene counts. Sankey bubble diagram (C).
Note: The Sankey diagram on the left represents the genes contained in each pathway. The bubble graph on the right shows the number of genes to which pathway belongs. The color of the bubbles represents the level of enrichment, and the redder the color, the more significantly enriched the gene is in the pathway.
The KEGG results revealed that the targets were abundant in 136 pathways, including Hypoxia-inducible factor (HIF-1), IL-17, cyclic adenosine monophosphate (cAMP), TNF, phosphoinositide 3-kinase (PI3K-Akt), MAPK, the NF-kappa B signaling pathway, etc. (Fig. 5B). In the enrichment analysis, it was found that Coicis Semen is capable of acting on OA through multiple pathways, primarily involved in the oxidative-inflammatory response. The Sankey bubble diagram revealed the genes contained in the first 30 pathways (Fig. 5C). The top 3 target genes with the highest frequency were MAPK1, ESR1, and PPARA (Suppl. Table S3).
A molecular docking study was performed in this study to verify the possible mutual effects between eight core target proteins and Coicis Semen active compounds. A heatmap was used to visualize docking scores, as shown in Fig. 6. Lower docking energies indicate higher stable receptor-ligand conformations (Tang et al., 2015). Target proteins were strongly bound to Coicis Semen compounds with binding energies between −4.3 and −10.8 kcal/mol, suggesting that Coicis Semen-derived compounds have a favorable affinity for eight core target proteins. Ultimately, the top four target protein macromolecules and small compound molecules with the best docking affinity were selected for visualization by Pymol (<−10 kcal/mol) (Fig. 7). Interestingly, MAPK1, ESR1, and PPARA were selected as the target proteins, which were also consistent with the enrichment results (Fig. 5C).
Figure 6: Heatmap of the docking scores. The horizontal coordinate represents the core target proteins (PDB ID), and the vertical coordinate represents the active compounds of Coicis Semen.
Figure 7: Molecular docking results of “chemical compounds and target genes.” The 3-dimensional visual structure of MOL001323 (sitosterol alpha1) to MAPK1 (7nr9) (A); MOL000359 (sitosterol) to PPARA (1k7l) (C); MOL000449 (stigmasterol) to ESR1 (7rs8) (E); MOL008121 (2-Monoolein) to PPARA (1k7l) (G). Their corresponding protein-ligand 2D interactions can be seen in B, D, F, and H, respectively. The dashed lines indicate hydrogen bonding (green) and hydrophobic interactions (light purple).
Mechanism of Coicis Semen in relation to MAPK1, ESR1 and PPAR-α expression
The expression of ESR1 and PPAR-α in OA patients was remarkably lower than that in healthy controls, but MAPK1 expression was significantly higher. Compared with pre-therapy, the expression level of ESR1 and PPAR-α increased, while that of MAPK1 decreased in both the control group and experimental group after treatment. When the difference value between the two groups before and after treatment, the expression of ESR1 and PPAR-α in the experimental group increased remarkably more than that in the control group, while MAPK1 expression decreased significantly more (Fig. 8).
Figure 8: *p < 0.05, **p < 0.01, vs. healthy group; #p < 0.05, ##p < 0.01, vs. pre-therapy; Δp < 0.05, vs. the difference value between the two groups pre-therapy and post-therapy.
Human chondrocytes C28/I2 were cultured and treated with LPS to build an in vitro model of OA. The expression of ESR1 and PPAR-α protein in the MC group was significantly lower than that in the NC group (p-ESR1 = 0.0022, p-PPAR-α = 0.0018). The expression level of ESR1 protein in the Coicis Semen group was significantly higher than that in the MC group (p-Coicis Semen-M = 0.0025, p-Coicis Semen-H = 0.0031) while the expression level of PPAR-α protein in the Coicis Semen group was significantly higher than that in the MC group (p-Coicis Semen-L = 0.0412, p-Coicis Semen-M = 0.0038, p-Coicis Semen-H = 0.0024). The protein expression levels of PPAR-α and ESR1 in the Coicis Semen-H group were higher than those in the Coicis Semen-M group (p-ESR1 = 0.0315, p-PPAR-α = 0.0221). Further, the expression of MAPK1 protein in the MC group was significantly higher than that in the NC group (p-MAPK1 = 0.0015). The expression level of MAPK1 protein in the Coicis Semen group was significantly lower than that in the MC group (p-Coicis Semen-L = 0.0032, p-Coicis Semen-M = 0.0028, p-Coicis Semen-H = 0.0034). The results showed that Coicis Semen significantly decreased the expression of MAPK1 and increased the expression of ESR1 and PPAR-α (Fig. 9).
Figure 9: Effects of Coicis Semen on Estrogen Receptor 1 (ESR1), Mitogen-activated protein kinase 1 (MAPK1), and Peroxisome proliferator-activated receptor alpha (PPAR-α) protein expression. Visual protein expression levels in C28/I2 chondrocytes from the normal control group (NC), model group (MC), Coicis Semen low-dose group (Coicis Semen-L), Coicis Semen medium-dose group (Coicis Semen-M) and Coicis Semen high-dose group (Coicis Semen-H) (A). Quantitative expression of ESR1 in each group (B). Quantitative expression of PPAR-α in each group (C). Quantitative expression of MAPK1 in each group (D). Data are represented as means ± SD. **p < 0.01, compared with the NC. #p < 0.05 and ##p < 0.01, compared with the MC. Δp < 0.05, compared with the Coicis Semen-M group. The experiments were repeated independently at least three times.
OA, as we understand it, is a degenerative disease characterized by joint pain, stiffness, and limited joint movement. The theory of Traditional Chinese Medicine (TCM) considers OA a form of “arthralgia syndrome”. The team has previously shown that dampness and spleen deficiency are the two main causes of OA (Zhou et al., 2021). Contemporaneously, it has been concluded that the regularization of CHM in the treatment of OA is designed to stimulate the spleen and remove dampness, which can serve as a reference for the treatment of clinical OA. Several herbal formulas are used to deal with spleen qi deficiency syndrome, which is a fundamental TCM syndrome related to chronic inflammation, as well as other diseases (Wang et al., 2020b). Hence, the concept of developing a new therapeutic drug based on traditional remedies or ethnological medicine to combat OA is a fine one. Coicis Semen can invigorate the spleen to remove dampness, tonify deficiency, relieve pain, and so on, as documented in the Chinese medical works of “Sheng Nong’s herbal classic”. A number of pharmacological activities related to Coicis Semen have been identified, including anti-inflammatory properties, analgesic properties, antioxidant properties, and immune-enhancing properties (Yang et al., 2013).
Taken together, data mining, systematic pharmacology, molecular docking techniques, and experimental validation have all been utilized in this study to explore the clinical efficacy, potential targets, and pathways of Coicis Semen as a treatment for OA and to uncover its molecular mechanisms.
In recent years, medical big data mining has shown broad development prospects and large application value, which will bring more support to many fields such as disease research, and clinical and management decision-making. As a result of the principles for drug association analysis, the compatibility of Coicis Semen and the optimization of oxidative-inflammatory indices, such as C3, C4, CRP, ESR, IgG, and SOD (Table 1), are strongly correlated. To evaluate the efficacy of the treatment, we assembled propensity score-matched pairs of patients to minimize imbalances between patients with Coicis Semen and those without it.
Compared with the unmatched cohort, propensity score-matched groups had minimal imbalances in measured baseline characteristics, such as age, sex, LOS, BMI, and comorbidities (coronary heart disease, cerebral infarction, hypertension, osteoporosis, fatty liver, hyperuricemia, and chronic gastritis) (p > 0.05) (Table 2). Conversely, residual imbalances persist in diabetes and anemia. The statistical results show that, compared with the control group, the experimental group had improved ESR, C3, SOD, CRP, IgG, C4, and IgA profiles more effectively (Table 3). As such, the strong therapeutic efficacy of herbal prescriptions, including Coicis Semen was verified. The patient’s oxidative-inflammatory indices refer to the long-term relationship between the changes in ESR, IgA, SOD, IgG, C3, CRP, and C4 and the intervention measures the patient received. A long-term correlation exists between the comprehensive evaluation indexes and intervention measures of the two groups, and the improvement impact of the indexes of the experimental group was greater than that of the control group (Table 4; Fig. 1). Further, superoxide dismutase (SOD) is a crucial antioxidant enzyme in the body. A dysregulation of SOD expression may contribute to OA pathogenesis, along with immune inflammation and oxidative stress (Paździor et al., 2019).
We investigated Coicis semen and OA targets further using network pharmacology (Zhang et al., 2019b) and understood the mechanisms and pathways between them directly from a large amount of data, which is a professional and effective method. Our findings revealed that Coicis Semen could significantly improve the oxidative-inflammatory markers of OA and that OA treatment with Coicis semen might act on MAPK1, ESR1, and PPARA via chemical active ingredients such as stigmasterol, sitosterol alpha1, sitosterol, 2-monoolein, and so on (Figs. 2–4). Signaling by MAPK1 is crucial to the transcription and translation of inflammatory biomarkers, as well as its role in cartilage damage in OA (Chen et al., 2018; Winkler et al., 2017). Estrogen binding to ER may protect articular cartilage, thereby delaying or even preventing the development of OA (Liu et al., 2014). As a result of estrogen loss, osteocytes experience increased reactive oxygen species (ROS), which may be a common mechanism of old age on bone homeostasis, accelerating aging-related skeletal changes (Almeida et al., 2017). PPARs play a role in maintaining immune and inflammatory responses, as well as cell proliferation and differentiation (Park et al., 2022). Thus, Coicis Semen exerts a multi-component, multi-network, and multi-target effect on biological processes implicated in immune inflammation and oxidative stress.
Twenty-four bioactive compounds were retrieved, including stigmasterol, CLR, sitosterol alpha1, omaine, mandenol, monoolein, to name a few (Table 5). More recently, stigmasterol was found to effectively reduce free radical production and lipid peroxidation, inhibit apoptosis in part, down-regulate Bax and cleaved caspase-3 expression, up-regulate Bcl-X1 expression, and significantly inhibit inflammatory responses in humans (Liang et al., 2020). Furthermore, sitosterol regulates bone metabolism balance as well as antioxidation (Ahmad Khan et al., 2020). In one report, stigmasterol could efficiently restrain the degradation of pro-inflammatory factors and matrix, as well as suppress the IL-1β-induced NF-κB signaling pathway (Ahmad Khan et al., 2020). Another recent study discovered that stigmasterol reduced IL-1-induced chondrocyte injury by regulating ferroptosis via down-regulation of IL-6 and TNF-α and up-regulation of SOD and glutathione (Mo et al., 2021). The monoolein, extracted from shige sinicola, is extremely anti-inflammatory, inhibiting both the mitogen-activated protein kinase and the NF-κB pathways (Ali et al., 2017). Together, these active components exert their antirheumatic activity in a variety of ways, such as reducing bone erosion and destruction and being anti-inflammatory, antioxidant, and immunoregulatory. As a result, these findings might provide insight into the mutual effectiveness and diversity of constituents in Coicis Semen for treating OA. Oxidative damage and inflammation play a central role in OA disease (Haigis and Yankner, 2010; Minguzzi et al., 2018). Therefore, anti-inflammatory and antioxidant therapies are the main treatment strategies. The anti-inflammatory and antioxidant activity of the active components of Coicis Semen were consistent with the main mechanism of OA.
GO enrichment analysis suggested that multiple targets are connected with oxidative stress and inflammation responses, confirming their correlation with OA pathogenesis (Fig. 5A). The CC results are localized to the nucleus and endoplasmic reticulum and linked to transcription factor activity and nuclear receptor activity in MF. With the ever-increasing evidence, estrogens fulfill a vital role in sustaining the homeostasis of articular tissues and, thus, the joint itself (Roman-Blas et al., 2009). Enrichment analysis has shown that Coicis Semen is closely related to estrogen metabolism and transcriptomes. Whether or not Coicis Semen can be used as an estrogen replacement drug to treat estrogen deficiency-induced cartilage damage will be significant. According to KEGG pathway enrichment analysis, there were 136 signaling pathways firsthand linked to OA, pointing out that Coicis Semen might modulate these pathways (Figs. 5B and 5C).
Additionally, molecular docking analysis was performed to examine the interactions between the eight hub genes and the active compounds complementing the key targets. The findings revealed that the target-compound pairs have high docking affinity, particularly PPARA, MAPK1, and ESR1. The docking results showed that sitosterol alpha1, stigmasterol, sitosterol, and 2-monoolein performed good binding activities with PPARA, MAPK1, and ESR1 (Figs. 6 and 7). For instance, the binding energy of molecule docking is less than −10 kcal/mol. Based on their high binding affinity, Coicis Semen is hypothesized to be effective in treating OA by modulating anti-inflammatory and antioxidant responses. Finally, the results of in clinical ELISA kit experiments showed that the Coicis Semen herbal prescription could increase the ESR1 and PPAR-α levels and decrease MAPK1 expression (Fig. 8). In vitro cell experiments additionally verified the influence of Coicis Semen on the expression levels of ESR1, PPAR-α, and MAPK1 proteins (Fig. 9), which were consistent with the results of molecular docking and clinical experiments. There are certain limitations present in this study, such as the boundedness of the database data, the corresponding analysis algorithms, the software functions of multiple platforms, the small experimental sample size, and some confounding factors in clinical trials. Nevertheless, certain observations could still be made.
Herbal prescriptions of Coicis Semen were associated with long-term improvements in the oxidative-inflammatory index in patients with OA, based on laboratory measures, propensity score methods, association rules, and random walk models. Exploring further, we tap into the major bioactive compounds and uncover the potential mechanism of action of Coicis Semen on OA, taking into account network pharmacology and molecular docking. Our study demonstrated that Coicis Semen may modulate MAPK1, ESR1, and PPARA targets through stigmasterol, sitosterol alpha1, sitosterol, and 2-monoolein, consistent with current advocacy for combination therapies. Research on this topic will undoubtedly increase our understanding of how OA is caused and help us develop different approaches to treating and preventing it. Subsequently, further in vitro experiments are necessary to verify its mechanism of action against OA.
Acknowledgement: We would like to thank the editor and the reviewers for their useful feedback that improved this paper.
Funding Statement: This work was supported by the Anhui Famous Traditional Chinese Medicine Liu Jian Studio Construction Project (Traditional Chinese Medicine Development Secret[2018] No. 11), the Ministry of Science and Technology National Key Research and Development Program Chinese Medicine Modernization Research Key Project (No. 2018YFC1705204), Anhui Province Traditional Chinese Medicine Leading Talent Project (Traditional Chinese Medicine Development Secret[2018] No. 23), the Anhui key Research and Development Program Foreign Science and Technology Cooperation Project (No. 201904b11020011) and the Anhui Provincial Education Department Project (No. 2022AH050449).
Author Contributions: The authors confirm contribution to the paper as follows: study conception and design: QIAO ZHOU and JIAN LIU; data collection and technical knowledge: LING XIN, YANYAN FANG and LEI WAN; analysis and interpretation of results: DAN HUANG and JIANTING WEN; draft manuscript preparation: QIAO ZHOU. All authors reviewed the results and approved the final version of the manuscript.
Availability of Data and Materials:: The datasets generated during and/or analyzed during the current study are available from the corresponding author on reasonable request.
Ethics Approval:: The research protocol and procedures have been approved by the Ethics Committee of the First Affiliated Hospital of Anhui University of Chinese Medicine (Review No. 2022MCZQ01).
Conflicts of Interest: The authors declare that they have no conflicts of interest to report regarding the present study.
References
Ahmad Khan M, Sarwar AHMG, Rahat R, Ahmed RS, Umar S (2020). Stigmasterol protects rats from collagen induced arthritis by inhibiting proinflammatory cytokines. International Immunopharmacology 85: 106642. https://doi.org/10.1016/j.intimp.2020.106642 [Google Scholar] [PubMed] [CrossRef]
Ali I, Manzoor Z, Koo JE, Moon SR, Byeon SH, Yoo ES, Kang HK, Hyun JW, Lee NH, Koh YS (2017). Monoolein, isolated from Ishige sinicola, inhibits lipopolysaccharide-induced inflammatory response by attenuating mitogen-activated protein kinase and NF-κB pathways. Food Science and Biotechnology 26: 507–511. https://doi.org/10.1007/s10068-017-0070-x [Google Scholar] [PubMed] [CrossRef]
Almeida M, Laurent MR, Dubois V, Claessens F, O’Brien CA, Bouillon R, Vanderschueren D, Manolagas SC (2017). Estrogens and androgens in skeletal physiology and pathophysiology. Physiological Reviews 97: 135–187. https://doi.org/10.1152/physrev.00033.2015 [Google Scholar] [PubMed] [CrossRef]
Cao J, Feng D, Wang Y, Zhang H, Zhao Y, Sun Z, Feng S, Chen Y, Zhu M (2021). Chinese herbal medicine Du-Huo-Ji-Sheng-decoction for knee osteoarthritis: A protocol for systematic review and meta-analysis. Medicine 100: e24413. https://doi.org/10.1097/MD.0000000000024413 [Google Scholar] [PubMed] [CrossRef]
Chen CYC (2011). TCM Database@Taiwan: The world’s largest traditional Chinese medicine database for drug screening in silico. PLoS One 6: e15939. https://doi.org/10.1371/journal.pone.0015939 [Google Scholar] [PubMed] [CrossRef]
Chen Y, Shou K, Gong C, Yang H, Yang Y, Bao T (2018). Anti-inflammatory effect of geniposide on osteoarthritis by suppressing the activation of p38 MAPK signaling pathway. BioMed Research International 2018: 1–11. https://doi.org/10.1155/2018/8384576 [Google Scholar] [PubMed] [CrossRef]
Chen B, Zhan H, Marszalek J, Chung M, Lin X, Zhang M, Pang J, Wang C (2016). Traditional Chinese medications for knee osteoarthritis pain: A meta-analysis of randomized controlled trials. The American Journal of Chinese Medicine 44: 677–703. https://doi.org/10.1142/S0192415X16500373 [Google Scholar] [PubMed] [CrossRef]
Ding L, Xie S, Zhang S, Shen H, Zhong H, Li D, Shi P, Chi L, Zhang Q (2020). Delayed comparison and apriori algorithm (DCAAA tool for discovering protein-protein interactions from time-series phosphoproteomic data. Frontiers in Molecular Biosciences 7: 606570. https://doi.org/10.3389/fmolb.2020.606570 [Google Scholar] [PubMed] [CrossRef]
Du J, Yin G, Hu Y, Shi S, Jiang J, Song X, Zhang Z, Wei Z, Tang C, Lyu H (2021). Coicis Semen protects against focal cerebral ischemia-reperfusion injury by inhibiting oxidative stress and promoting angiogenesis via the TGFβ/ALK1/Smad1/5 signaling pathway. Sedentary Life and Nutrition 13: 877–893. https://doi.org/10.18632/aging.202194 [Google Scholar] [PubMed] [CrossRef]
El-Tawil S, Arendt E, Parker D (2016). Position statement: The epidemiology, pathogenesis and risk factors of osteoarthritis of the knee. Journal of ISAKOS 1: 219–228. https://doi.org/10.1136/jisakos-2015-000002 [Google Scholar] [CrossRef]
Fang Y, Liu J, Xin L, Jiang H, Guo J, Li X, Wang F, He M, Han Q, Huang D (2022a). Radix salvia miltiorrhiza for ankylosing spondylitis: Determining potential inflammatory molecular targets and mechanism using network pharmacology. BioMed Research International 2022: 1–13. https://doi.org/10.1155/2022/3816258 [Google Scholar] [PubMed] [CrossRef]
Fang Y, Liu J, Xin L, Wen J, Guo J, Huang D, Li X (2022b). Exploration of the immuno-inflammatory potential targets of xinfeng capsule in patients with ankylosing spondylitis based on data mining, network pharmacology, and molecular docking. Evidence-Based Complementary and Alternative Medicine 2022: 1–10. https://doi.org/10.1155/2022/5382607 [Google Scholar] [PubMed] [CrossRef]
Guo Y, Chen J, Xiong X, Wu D, Zhu H, Liang Q (2012). Effect of Bizhongxiao decoction and its dismantled formulae on IL-1 and TNF levels in collagen-induced arthritis in rat synovial joints. Theoretical Biology and Medical Modelling 9: 47. https://doi.org/10.1186/1742-4682-9-47 [Google Scholar] [PubMed] [CrossRef]
Haigis MC, Yankner BA (2010). The aging stress response. Molecular Cell 40: 333–344. https://doi.org/10.1016/j.molcel.2010.10.002 [Google Scholar] [PubMed] [CrossRef]
Hodgkinson T, Kelly DC, Curtin CM, O’Brien FJ (2022). Mechanosignalling in cartilage: An emerging target for the treatment of osteoarthritis. Nature Reviews Rheumatology 18: 67–84. https://doi.org/10.1038/s41584-021-00724-w [Google Scholar] [PubMed] [CrossRef]
Huang DW, Sherman BT, Lempicki RA (2009). Bioinformatics enrichment tools: Paths toward the comprehensive functional analysis of large gene lists. Nucleic Acids Research 37: 1–13. https://doi.org/10.1093/nar/gkn923 [Google Scholar] [PubMed] [CrossRef]
Jia Z, Wei QJ (2021). CircRNA-MSR regulates LPS-induced C28/I2 chondrocyte injury through miR-643/MAP2K6 signaling pathway. Cartilage 13: 785S–795S. https://doi.org/10.1177/19476035211044826 [Google Scholar] [PubMed] [CrossRef]
Li X, Liu J, Fang Y, He M, Wang F, Han Q (2022). Mechanism of xinfeng capsule in the treatment of hypercoagulable state of ankylosing spondylitis based on data mining and network pharmacology. BioMed Research International 2022: 1–10. https://doi.org/10.1155/2022/8796980 [Google Scholar] [PubMed] [CrossRef]
Liang Q, Yang J, He J, Chen X, Zhang H, Jia M, Liu K, Jia C, Pan Y, Wei J (2020). Stigmasterol alleviates cerebral ischemia/reperfusion injury by attenuating inflammation and improving antioxidant defenses in rats. Bioscience Reports 40: BSR20192133. https://doi.org/10.1042/BSR20192133 [Google Scholar] [PubMed] [CrossRef]
Liu W, Shao FM, Yan L, Cao HX, Qiu D (2014). Polymorphisms in the gene encoding estrogen receptor alpha are associated with osteoarthritis in Han Chinese women. International Journal of Clinical and Experimental Medicine 7: 5772–5777. [Google Scholar] [PubMed]
Minguzzi M, Cetrullo S, D’Adamo S, Silvestri Y, Flamigni F, Borzì RM (2018). Emerging players at the intersection of chondrocyte loss of maturational arrest, oxidative stress, senescence and low-grade inflammation in osteoarthritis. Oxidative Medicine and Cellular Longevity 2018: 1–17. https://doi.org/10.1155/2018/3075293 [Google Scholar] [PubMed] [CrossRef]
Mo Z, Xu P, Li H (2021). Stigmasterol alleviates interleukin-1beta-induced chondrocyte injury by down-regulatingsterol regulatory element binding transcription factor 2 to regulateferroptosis. Bioengineered 12: 9332–9340. https://doi.org/10.1080/21655979.2021.2000742 [Google Scholar] [PubMed] [CrossRef]
Park S, Baek IJ, Ryu JH, Chun CH, Jin EJ (2022). PPARα−ACOT12 axis is responsible for maintaining cartilage homeostasis through modulating de novo lipogenesis. Nature Communications 13: 3. https://doi.org/10.1038/s41467-021-27738-y [Google Scholar] [PubMed] [CrossRef]
Paździor M, Kiełczykowska M, Kurzepa J, Luchowska-Kocot D, Kocot J, Musik I (2019). The oxidative stress in knee osteoarthritis patients. An attempt of evaluation of possible compensatory effects occurring in the disease development. Medicina 55: 150. https://doi.org/10.3390/medicina55050150 [Google Scholar] [PubMed] [CrossRef]
Pearson K (1905). The problem of the random walk. Nature 72: 342. https://doi.org/10.1038/072342a0 [Google Scholar] [CrossRef]
Piñero J, Saüch J, Sanz F, Furlong LI (2021). The DisGeNET cytoscape app: Exploring and visualizing disease genomics data. Computational and Structural Biotechnology Journal 19: 2960–2967. https://doi.org/10.1016/j.csbj.2021.05.015 [Google Scholar] [PubMed] [CrossRef]
Raad H, Cornelius V, Chan S, Williamson E, Cro S (2020). An evaluation of inverse probability weighting using the propensity score for baseline covariate adjustment in smaller population randomised controlled trials with a continuous outcome. BMC Medical Research Methodology 20: 70. https://doi.org/10.1186/s12874-020-00947-7 [Google Scholar] [PubMed] [CrossRef]
Ringdahl E, Pandit S (2011). Treatment of knee osteoarthritis. American Family Physician 83: 1287–1292. [Google Scholar] [PubMed]
Roman-Blas JA, Castañeda S, Largo R, Herrero-Beaumont G (2009). Osteoarthritis associated with estrogen deficiency. Arthritis Research & Therapy 11: 241. https://doi.org/10.1186/ar2791 [Google Scholar] [PubMed] [CrossRef]
Schöning-Stierand K, Diedrich K, Fährrolfes R, Flachsenberg F, Meyder A, Nittinger E, Steinegger R, Rarey M (2020). ProteinsPlus: Interactive analysis of protein-ligand binding interfaces. Nucleic Acids Research 48: W48–W53. https://doi.org/10.1093/nar/gkaa235 [Google Scholar] [PubMed] [CrossRef]
Scotece M, Conde J, Abella V, López V, Francisco V et al. (2018). Oleocanthal inhibits catabolic and inflammatory mediators in LPS-activated human primary osteoarthritis (OA) chondrocytes through MAPKs/NF-κB pathways. Cellular Physiology and Biochemistry 49: 2414–2426. https://doi.org/10.1159/000493840 [Google Scholar] [PubMed] [CrossRef]
Shen P, Tu S, Wang H, Qin K, Chen Z (2019). Simiao pill attenuates collagen-induced arthritis in rats through suppressing the ATX-LPA and MAPK signalling pathways. Evidence-Based Complementary and Alternative Medicine 2019: 1–11. https://doi.org/10.1155/2019/7498527 [Google Scholar] [PubMed] [CrossRef]
Si YH, Ma Y, Guo Y, Pan YL, Zheng SY, Xu GH, Da WW, Xu LL (2018). Efficacy and safety of Shaoyang Xibi decoction in patients with knee osteoarthritis: A multi-center, single-blind, randomized controlled trial. Journal of Traditional Chinese Medicine 38: 733–739. https://doi.org/10.1016/S0254-6272(18)30912-9 [Google Scholar] [CrossRef]
Tang Y, Li M, Wang J, Pan Y, Wu FX (2015). CytoNCA: A cytoscape plugin for centrality analysis and evaluation of protein interaction networks. Biosystems 127: 67–72. https://doi.org/10.1016/j.biosystems.2014.11.005 [Google Scholar] [PubMed] [CrossRef]
Tang M, Xie X, Yi P, Kang J, Liao J, Li W, Li F (2020). Integrating network pharmacology with molecular docking to unravel the active compounds and potential mechanism of simiao pill treating rheumatoid arthritis. Evidence-Based Complementary and Alternative Medicine 2020: 1–16. https://doi.org/10.1155/2020/5786053 [Google Scholar] [PubMed] [CrossRef]
van Spil WE, Kubassova O, Boesen M, Bay-Jensen AC, Mobasheri A (2019). Osteoarthritis phenotypes and novel therapeutic targets. Biochemical Pharmacology 165: 41–48. https://doi.org/10.1016/j.bcp.2019.02.037 [Google Scholar] [PubMed] [CrossRef]
Wang M, Liu L, Zhang CS, Liao Z, Jing X, Fishers M, Zhao L, Xu X, Li B (2020a). Mechanism of traditional chinese medicine in treating knee osteoarthritis. Journal of Pain Research 13: 1421–1429. https://doi.org/10.2147/JPR.S247827 [Google Scholar] [PubMed] [CrossRef]
Wang X, Wu M, Lai X, Zheng J, Hu M, Li Y, Li S (2020b). Network pharmacology to uncover the biological basis of spleen Qi deficiency syndrome and herbal treatment. Oxidative Medicine and Cellular Longevity 2020: 1–20. https://doi.org/10.1155/2020/8857906 [Google Scholar] [PubMed] [CrossRef]
Wang Y, Yang H, Chen L, Jafari M, Tang J (2021). Network-based modeling of herb combinations in traditional Chinese medicine. Briefings in Bioinformatics 22: bbab106. https://doi.org/10.1093/bib/bbab106 [Google Scholar] [PubMed] [CrossRef]
Watanabe N, Ohnuki Y, Sakakibara Y (2021). Deep learning integration of molecular and interactome data for protein-compound interaction prediction. Journal of Cheminformatics 13: 36. https://doi.org/10.1186/s13321-021-00513-3 [Google Scholar] [PubMed] [CrossRef]
Winkler C, Ferdous F, Dimmick M, Scott T (2017). Lipopolysaccharide induced Interleukin-6 production is mediated through activation of ERK 1/2, p38 MAPK, MEK, and NFκB in chicken thrombocytes. Developmental & Comparative Immunology 73: 124–130. https://doi.org/10.1016/j.dci.2017.03.017 [Google Scholar] [PubMed] [CrossRef]
Wood PA, Pidcock E, Allen FH (2008). Interaction geometries and energies of hydrogen bonds to C=O and C=S acceptors: A comparative study. Acta Crystallographica B 64: 491–496. https://doi.org/10.1107/S0108768108015437 [Google Scholar] [PubMed] [CrossRef]
Wu WT, Li YJ, Feng AZ, Li L, Huang T, Xu AD, Lyu J (2021). Data mining in clinical big data: The frequently used databases, steps, and methodological models. Military Medical Research 8: 44. https://doi.org/10.1186/s40779-021-00338-z [Google Scholar] [PubMed] [CrossRef]
Xu Y, Liu Q, Liu Z, Lim L, Chen W, Lin N (2013). Treatment with SiMiaoFang, an anti-arthritis chinese herbal formula, inhibits cartilage matrix degradation in osteoarthritis rat model. Rejuvenation Research 16: 364–376. https://doi.org/10.1089/rej.2013.1439 [Google Scholar] [PubMed] [CrossRef]
Yang RS, Lu YH, Chiang W, Liu SH (2013). Osteoporosis prevention by adlay (薏苡 Yì Yĭ: The Seeds of Coix Lachryma-Jobi L. var. ma-yuen Stapf) in a mouse model. Journal of Traditional and Complementary Medicine 3: 134–138. https://doi.org/10.4103/2225-4110.110408 [Google Scholar] [PubMed] [CrossRef]
Yang L, Wu B, Ma L, Li Z, Xiong H (2021). Comparative efficacy and safety of Chinese herbal medicine for knee osteoarthritis: A protocol for systematic review and network meta-analysis. Medicine 100: e26671. https://doi.org/10.1097/MD.0000000000026671 [Google Scholar] [PubMed] [CrossRef]
Zhang C, Zhang W, Shi R, Tang B, Xie S (2019a). Coix lachryma-jobi extract ameliorates inflammation and oxidative stress in a complete Freund’s adjuvant-induced rheumatoid arthritis model. Pharmaceutical Biology 57: 792–798. https://doi.org/10.1080/13880209.2019.1687526 [Google Scholar] [PubMed] [CrossRef]
Zhang R, Zhu X, Bai H, Ning K (2019b). Network pharmacology databases for traditional Chinese medicine: Review and assessment. Frontiers in Pharmacology 10: 123. https://doi.org/10.3389/fphar.2019.00123 [Google Scholar] [PubMed] [CrossRef]
Zhou Q, Liu J, Xin L, Fang Y, Wan L, Huang D, Guo J, Wen J, Wang B (2021). Exploratory compatibility regularity of traditional Chinese medicine on osteoarthritis treatment: A data mining and random walk-based identification. Evidence-Based Complementary and Alternative Medicine 2021: 2361512. https://doi.org/10.1155/2021/2361512 [Google Scholar] [PubMed] [CrossRef]
Supplementary Materials
Table S1: The characteristics of clinical subjects in experiment validation
Table S2: The information on target genes in seven disease databases
Table S3: Information on the Sankey bubble diagram as in Fig. 5C
Cite This Article
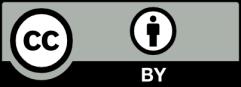