Open Access
REVIEW
Differential mRNA expression in peripheral blood is associated with oral squamous cell carcinoma: Recent advances and future challenges
1 Department of Stomatology, Zunyi Medical University, Zunyi, 563000, China
2 Department of Oral and Maxillofacial Surgery, Peking University Shenzhen Hospital Stomatology Center, Shenzhen, 518000, China
3 Department of Nursing, Henan University, Kaifeng, 475000, China
* Corresponding Author: HONGYU YANG. Email:
# These authors contributed equally to this study
(This article belongs to the Special Issue: Recent Advancement in Cancer Molecular Signaling)
BIOCELL 2023, 47(7), 1449-1458. https://doi.org/10.32604/biocell.2023.026704
Received 21 September 2022; Accepted 16 January 2023; Issue published 21 June 2023
Abstract
Oral squamous cell carcinoma (OSCC) is a malignant tumor triggered by the accumulation of multiple gene mutations in oral epithelial cells. Different OSCC-related biomarkers have been reported in circulation in the peripheral blood that support the occurrence and development of OSCC. Recent advances in high-throughput and highly sensitive detection methods have overcome the limitation of the low concentration of most peripheral blood biomarkers. Hence, blood biomarker detection has become an efficient screening tool for the early diagnosis of OSCC. The growing data available in public cancer and gene databases have provided new foundations for OSCC research. In particular, the identification of OSCC biomarkers using bioinformatic tools has shed new light on the underlying mechanisms as well as on the genetic landscape of OSCC. More recently, mRNA targeting therapies have emerged as valuable anticancer treatment strategies, as they allow for the regulation of the expression of certain functional proteins to reverse genetic abnormalities or induce tissue repair. Thus, mRNA-targeting therapies can be used to regulate the expression of antigens, antibodies, or cellular receptors by immune cells. Particularly, anti-cancer cellular immunotherapy carrying specific mRNAs has attracted significant attention in OSCC treatment. Here, we review the present knowledge on the role of peripheral blood mRNAs in the diagnosis, treatment, development, and prognosis of OSCC. Moreover, we address future research prospects of mRNAs in the peripheral blood in OSCC and the opportunities and challenges that may arise in future clinical therapeutic applications.Keywords
Oral squamous cell carcinoma (OSCC) is a common malignant tumor with a survival rate of less than 65% after 5 years of resection (Chi et al., 2015). OSCC is caused by gene mutations associated with chewing betel quid, smoking, and drinking. OSCC also results from human papillomavirus infection. To date, several pathogenic proteins have been shown to be associated with OSCC, and the involvement of RNAs, such as micro, circular, and long non-coding RNAs, have also added to the increased complexity of OSCC diagnosis and treatment. Treatments for OSCC have progressed from surgery, radiotherapy, and chemotherapy to immune targeted therapy. For example, lipid nanoemulsion particles loaded with curcumin have shown anticancer properties with effects against OSCC (Liu et al., 2022). Hyperthermia therapy in combination with traditional treatment methods was also shown to represent a new treatment option with enhanced efficacy (Ju et al., 2018). However, hyperthermia therapy is still under investigation and undergoing clinical trials, so it is not widely available. Moreover, a ‘magic’ approach involves improving malignancy treatment, including regulating the pro-resolution pathway, as its resolvin D2 was shown to reduce the levels of cancer-derived cytokines/chemokines, such as tumor necrosis factor-α (TNF-α), interleukin (IL)-6, chemokine-10 (CXCL10), and monocyte chemoattractant protein-1 (MCP-1), as well as of populations of cancer mediator-induced CD11b+Ly6G myeloid cells and nociception (Song et al., 2020).
Using fluids for early diagnostics has advantages associated with their relatively non-invasive collection process. Particularly, mRNA in the blood, semen, urine, and saliva has been established to represent a new resource that can replace traditional disease diagnostic methods (Faria et al., 2010). Changes in mRNA expression in the peripheral blood can promote OSCC occurrence and development, and thus can be used for early diagnosis of OSCC (Faria et al., 2010; Srivastava and Ghosh, 2015). Indeed, programmed cell death receptor 1 (PD1), arginase 1 (ARG1), ornithine decarboxylase (ODC), prospero homeobox 1 (PROX1), and other coding genes were identified in patients with OSCC and associated with tumor occurrence and progression via the epithelial–mesenchymal transition (EMT), phosphatidylinositol 3-kinase (PI3K)/protein kinase B (AKT), and Notch signaling pathways. The Notch signaling pathway enhances the proliferative signal via neural proliferation, and its activity is inhibited by the Numb protein to promote neural differentiation (Musfee et al., 2020; Wang et al., 2021). In addition, the role of inflammation and the tumor microenvironment (TME) have also been investigated in the context of OSCC. Ubiquitin-conjugating enzyme E2C (UBE2C) was related to the pathogenesis and TME of OSCC, which might be a potential diagnostic and therapeutic biomarker (Huang et al., 2021). Particularly, the chronic states of leukoplakia and oral submucous fibrosis gradually develop into OSCC (Nissinen et al., 2016).
Nevertheless, although many studies have identified differentially expressed genes in OSCC, neither research aided by public databases nor experimental evidence has led to actual improvements in OSCC survival rates. Therefore, specific biomarkers for tumor diagnosis are still warranted, and patient-individual differences must be considered. Many scientists have focused on the relationship between bloodborne mRNA levels and OSCC; however, specific diagnostic molecular blood markers have not yet been identified for the inchoate stage diagnosis of OSCC. Therefore, we have reviewed the present knowledge on the role of peripheral blood mRNA biomarkers, identified using bioinformatic tools, in the diagnosis, treatment, and prognosis of OSCC. In addition, we address the opportunities and challenges that may arise in the future application of mRNA biomarkers for OSCC clinical treatment.
Peripheral Blood mRNA and OSCC
Tissue and saliva mRNA and peripheral blood gene expression in patients with OSCC are currently being investigated to identify biomarkers of OSCC (Bu et al., 2015). In 2010, a study group analyzed the peripheral blood of 27 patients with OSCC and 25 healthy controls. They revealed that peripheral blood plays an important role in the diagnosis and treatment of OSCC. Annexin A1 (ANXA1), which was reported to be downregulated at the mRNA level in OSCC, was the first blood-based biomarker identified and represents a complementary non-invasive approach for diagnosing OSCC because it is present in the blood at significantly low levels, depending on the age, sex, and tumor anatomy of each patient (Faria et al., 2010). Lymphocytes are the main components of peripheral blood in patients with tumors. The ANXA1 pathogenic mechanism remains unclear but may refer to the “eat me” signals in tumor cells (Faria et al., 2010). Serum levels of IL-17F, which is secreted by peripheral blood mononuclear cells during tumor development, can be used for OSCC diagnosis (Ding et al., 2015). Moreover, the number of CD3+ and CD4+ T cells, which are critical components of the immune system, affects IL-17F and the IL-17F:vascular endothelial growth factor (VEGF) ratio (Hu et al., 2016). Tumor biomarkers in the blood or other body fluids as well as in tissues represent signs of either a normal or abnormal process and/or a specific situation or disease; thus, examining several biomarkers together can promote more accurate results and reliable cancer diagnosis (Hu et al., 2016). In addition, low c-myc expression results in poor prognosis of OSCC (Vora et al., 2007). ARG1 and ODC levels were found to be increased in the peripheral blood of patients with head and neck squamous cell carcinoma (HNSCC) by immunocytochemistry, semiquantitative reverse transcription and quantitative real-time polymerase chain reactions, and the expression of ARG1, ARG2, and ODC was markedly increased in tumor tissues as compared with adjacent tissues (Srivastava and Ghosh, 2015; Zhang et al., 2013).
The disease-free survival rate of patients with reduced post–operative cytokeratin (CK) 17 mRNA expression was found to be significantly increased compared with that of patients with elevated CK17; thus, CK17 is a candidate tumor marker of OSCC, and, of the CK-family, it might be the most suitable marker for diagnosis of OSCC due to its significant upregulation and the strong overexpression in peripheral blood (Kitamura et al., 2017). Indeed, it was reported that the overexpression of CK17 and CK20 is significantly associated with metastases in neck lymph nodes and that CK19 is significantly overexpressed in T3 and T4 OSCC in stages III and IV patients and in poorly differentiated OSCC. Nevertheless, determined by relative quantification, the mean value of CK17 overexpression was significantly higher than that of the other CKs and significantly associated with T1 and T2 OSCC as well as with stages I and II patients (Toyoshima et al., 2007). VEGF levels were reported to be enriched in OSCC cases as compared with healthy controls (Nabavi et al., 2019). Serum CK20 is relevant for the prognosis of OSCC, as it indicates disseminated tumor cells in the peripheral blood of postoperative patients with OSCC (Kitamura et al., 2017). In addition, Fas apoptotic inhibitory molecule 2 (FAIM2) was found to be downregulated in OSCC tissues, with KRT13, FAIM2, and CYP2W1 expression in OSCC showing some tendency to be associated with some clinical-pathological parameters and survival (Hartanto et al., 2015). TaqMan low-density array data showed that low-density arrays of selected blood genes can indeed be used to provide insights into cancer progression pathways, and FAIM2 may represent an early biomarker for monitoring tobacco-induced head and neck squamous cell (HNSC) neoplasms (Hasan et al., 2020).
OSCC markers identified from tissues have paved the way for the research on additional biomarkers in the peripheral blood. The crosstalk between myeloid-derived suppressor cells (MDSCs) and cancer cells promotes the malignant processes of OSCC and the immune-suppressive properties of MDSCs, which provides an opportunity for the targeted treatment of tumor-associated immunosuppressive cells (Pang et al., 2020). Analysis of immunocytes in the peripheral blood showed that high IL-18 levels were associated with more CD19+ B cells, where serum IL-37 seemed to be associated with reduced percentage of CD3+CD8+ T cells, indicating that their balance could change the adaptive immune response. We first revealed the different functions of the IL-18/IL-37 ratio in serum and tumor tissues (Ding et al., 2020). High IL-18 expression in cancer tissues of low lymph node metastasis and tumor stages, which is not consistent with the pro-cancerogenic role of serum, and the IL-18:IL-37 ratio can affect B cells (CD19+) and T cells (CD3+ and CD8+) in OSCC, suggesting it to be an efficient biomarker of OSCC. In OSCCs, the transforming growth factors (TGF)-β1/IL-17A stimulate neutrophils and notably induce various cell processes, including migration, proliferation, invasion, stemness, and EMT (Yu et al., 2021). Moreover, IL-17A can promote angiogenesis in several tumors, including OSCC (Ding et al., 2015). Prognostic factors for OSCC include carbonic anhydrase IX that is a potential target for treatment; therefore, it should not be ignored (Eckert et al., 2019).
In just a few years, microarray chip technology, transcription analysis, RNA sequencing, and other high-throughput genetic detection technologies have become affordable and increasingly accurate. In tumors, bioinformatic analysis is performed to identify differential expression and functional enrichment of unknown genes; thus, RNA sequencing has been applied to predict relevant clinical outcomes and is poised to be increasingly used for personalized diagnosis and treatment. In addition, the RankComp algorithm revealed six pairs of relative expression orderings, which have important implications for gene signatures in OSCC (Ou and Wu, 2021). Thus, more tumor biomarkers in the peripheral blood of OSCC patients will be detected in the future by bioinformation analysis. Relevant tumor markers in the peripheral blood of OSCC patients as shown in Fig. 1 and Table 1.
Figure 1: The pathological mechanisms of biomarkers and OSCC blood.
Tumor development is associated with gene mutations in local sites and throughout circulation, such as blood circulation and immune response. Owing to the complex processes between local tissues and body circulation, genes found in tissues may be indicators for the development of OSCC in circulation. Recently, PD1 in peripheral blood has been a hot topic; new biomarkers have been found and previous biomarkers have been tested in different studies; such studies aim to identify genes to guide blood analyses. Among them, the immune response plays a major role in the development of OSCC. Immune tolerance is mediated by inhibitory signaling pathways, so-called immune checkpoints, of which PD1 is one of the most relevant pathways. PD1 overexpression can indicate a decreased immune response, which can be due to T-cell exhaustion and the promotion of immune tolerance (Wehrhan et al., 2021). Indeed, PD-L1 and PD-L2 are ligands of PD1; the interaction between them represents the majority of local immunosuppression mediation in oral cancer (Wehrhan et al., 2021). PD-L1 binds to PD1, a cell surface receptor expressed by activated T cells and macrophages, and induces apoptosis or the formation of regulatory T cells in these immune cells. Thus, PD-L1 overexpression leads to immunosuppression (Weber et al., 2017). Targeting the PD1/PD-L1 pathway with specific antibodies leads to significantly improved overall survival and quality of life (Weber et al., 2017). The association between lymph node metastases and high PD-L1 expression in the peripheral blood of patients with OSCC indicates that the PD-L1 expression of immune cells might be of high biological relevance.
Many tissue biomarkers may be explored in peripheral blood in the future. However, the blood contains tumor regulation factors, mediators, or inflammation factors of other tumor types. Integrin may represent a potential candidate biomarker for oral cancer. The absence of NOD-like receptor family pyrin-domain-containing protein 3 (NLRP3) inflammasomes enhances the anti-tumor effect of fluorouracil, which allows integrin-signaling for regulating different functions, including cell migration, invasion, proliferation, and movement; thus, integrin may represent a potential candidate biomarker for oral cancer (Russell et al., 2013).
To date, only a few studies have explored the different pathways involved in OSCC development within local tumor tissues and circulating peripheral cells. A previous study found that PD-L1 upregulation in the peripheral blood of OSCC patients was associated with poor overall survival (Weber et al., 2019). Zhao et al. (2022) reported that the knockdown of ST2 downregulated constitutive PD-L1 expression, whereas ST2 overexpression enhanced PD-L1 levels via IL-33 signals. Indeed, the IL-33/ST2 mechanism activates the JAK2/STAT3 pathway to directly promote PD-L1 expression, but it also promotes MyD88/NF-κB signals that indirectly further support IFN-γ-induced PD-L1 expression (Zhao et al., 2022). The selectivity of mogamulizumab towards regulatory T cells among the peripheral CCR4+CD8+ cell population may be increased by adjusting the trametinib concentration in the blood, as CD8+ T-cell susceptibility to mogamulizumab is lower than that of regulatory T cells in vitro (Ono et al., 2022). In addition, OSCC cells induce MDSC differentiation with an immune suppressive phenotype from peripheral bone marrow cells, enhance the secretion of ARG1, and induce nitric oxide synthase by MDSCs (Pang et al., 2020). The expression of survivin has been reported in OSCC, where it plays a role in reducing apoptotic cell death and increasing the proliferative activity of cancer cells in the peripheral blood. Calixarene 0118 causes the early induction of the mitogen-activated protein kinase pathway via the immediate response gene FOS, a subunit of the activator protein-1 complex, which affects the progression of early tumors (Greer et al., 2022). Glaucocalyxin A (GLA) disrupts redox homeostasis and activates the mitochondrial apoptotic pathway, whereas cell apoptosis is completely prevented by the synthetic peptide Z-VAD(OMe)-FMK and N-acetylcysteine (Wang et al., 2022). Simultaneously, the expression state of ChaC glutathione-specific gamma-glutamylcyclotransferase 1 (CHAC1) significantly affects GLA-mediated apoptosis.
Liquid biopsies (LBs) are currently regarded as clinically essential and non-invasive diagnostic tools for OSCC because they could provide precision and individualized medicine to design tailored treatments (Adeola et al., 2022). Indeed, some peripheral blood serum biomarkers were reported to be related to tumor size and staging, thereby representing valuable tools for early OSCC diagnosis (Payehghadr et al., 2018). Hence, peripheral blood gene expression profiling may be used for the early detection of pre-malignant lesions. In particular, genes in the peripheral blood may be used as surrogates for providing insights into cancer progression pathways and, possibly, as early biomarkers for monitoring tobacco-induced head and neck squamous cell carcinomas (HNSCC), including OSCC (Hasan et al., 2020). A previous study revealed similar alterations in tissue samples and the peripheral blood of tobacco-consuming HNSCC patients concerning polycyclic aromatic hydrocarbon-metabolizing enzymes and associated transcription factors, signaling molecules, DNA repair genes, oncogenes, EMT pathway genes, and cell adhesion/motility genes (Hasan et al., 2020).
As most clinical biochemical analyses involve the use of blood plasma or serum, LBs have several advantages including easy accessibility, reliability, reproducibility, and the possibility of early detection of diseases (Hasan et al., 2020). In addition, LBs may be clinically useful, as they provide a more representative picture of circulating tumor cells (CTCs), circulating tumor DNA and RNA, and tumor-derived exosomes obtained among all types of body fluids (Hasan et al., 2020). Cancer cells have an inherent tendency to detach from the tumor and enter the circulating system, which will support their high proliferation and low cohesiveness. In addition, during apoptosis and necrosis, tumor cells shed their genetic materials and protein content into the bloodstream. Similarly, such substances are also secreted during metastasis; thus, they can be exploited as markers. These properties of cancer cells have been used for early diagnosis of tumors, therapeutic guidance, and recurrence monitoring. For example, increased levels of CD44s mRNA in the peripheral circulation were related to tumor progression and metastasis. The CD44 expressed on tumor cells binds weakly to CD62 on endothelial cells to mediate the rolling of tumor cells for extravasation, which further leads to metastasis. Signal transduction induced by CD44 generally occurs via the Wnt, PI3K/AKT, and ERK pathways. A recent study on MDA-MB-468 cells showed that CD44-HA binding initiates cellular signals through c-Jun, which ultimately upregulates apoptosis inhibitors and supports tumor cell survival (Singh et al., 2022a). In addition, CYP1A1 expression in peripheral blood lymphocytes has been reported to be similar to that observed in surgically resected tissue. Notably, an increased CYP1A1 mRNA expression in peripheral blood lymphocytes was found to be associated with CYP1A1 polymorphisms, as well as with tumor invasion and metastasis (Singh et al., 2022b).
Although researchers have determined many biomarkers in the peripheral blood, no specific markers have been reported to detect early OSCC. Therefore, mRNA expression in local tissues should be studied to elucidate the relationship with peripheral blood. The expression of CK13, FAIM2, and cytochrome P450 family 2 subfamily W member 1 (CYP2W1) in OSCC is correlated with selected clinicopathological parameters and the survival rate of patients. These genes were downregulated in OSCC samples compared with adjacent samples.
Anti-Cancer Cellular Immunotherapy and OSCC Blood
PD-L1 expression in OSCC may contribute to an immunosuppressive local TME. A systemic state of immune activation (indicated by a low checkpoint expression in the peripheral blood) or a state of immune tolerance (indicated by a high checkpoint expression) can help determine tumor progression in OSCC (Weber et al., 2019). Indeed, a cutoff point (COP) can be determined to allow for the differentiation of OSCC patients from controls based on PD-L1 expression in the tumor tissue or peripheral blood (Weber et al., 2017). Notably, an increased malignant behavior (higher tumor grade, positive nodal status) may be associated with PD-L1-mediated systemic immune tolerance (Weber et al., 2017). Moreover, PD-L1 expression in the peripheral blood can indicate the existence of metastatic disease (N+) in OSCC. The role of the absolute lymphocyte count in peripheral blood as a predictive biomarker has been validated in metastatic melanoma patients treated with ipilimumab (Darvin et al., 2018). Cytometry by time-of-flight-based immune profiling of peripheral blood samples collected from anti-CTLA-4 and anti-PD1-treated melanoma patients showed a distinct set of biomarkers in response to therapy (Darvin et al., 2018). Apart from circulating CD8+ T cells, CD8+ effector memory type-1 T cells have also been reported as predictive biomarkers for ipilimumab-treated stage IV melanoma (Darvin et al., 2018). Interestingly, evaluation of non-small-cell lung cancer patients showed that PD-L1+ CTCs were more frequent than PD-L1+ cells in the TME (83% vs. 41%); however, no correlation was observed between PD-L1 expression in tumors and CTCs (Darvin et al., 2018). Therefore, further investigation is warranted to establish PD-L1 expression on CTCs as a cancer-predictive biomarker (Darvin et al., 2018). In patients with advanced-stage OSCC, surgery along with adjuvant radiotherapy remain the preferred treatments, which are performed with or without chemotherapy (Coletta et al., 2020).
Ganoderma lucidum polysaccharides (GLPS) treatment changes tumor cell morphology and granularity, delays migration, decreases colony formation, and impairs sphere formation, thereby leading to non-invasive and less proliferative behavior in OSCC cells (de Camargo et al., 2022). Moreover, as FOXO3a activation inhibits CDK4/6 and cyclin D1 expression and upregulates p27 and Bim expression of OSCC cells, FOXO3 may be a potent therapeutic target for OSCC (Fang et al., 2011). Genomic studies have dissected the genetic characteristics of the immune compartment to characterize cancer subtypes of immune activity and immune failure. This approach is expected to provide information on the immune status of patients with OSCC and guide new therapies to improve immune-therapy responses (Chai et al., 2020). FOXD1-overexpression leads to stronger tumorigenic abilities by promoting the occurrence and development of tumors (Li et al., 2020). Lycorine hydrochloride induces the mitochondrial apoptotic pathway through ROS mediated by the JNK signaling pathway leading to HSC-3 cell apoptosis, showing that lycorine hydrochloride is a potential drug candidate for OSCC therapy (Li et al., 2021). During the process of treating OSCC, anlotinib targets FGFR3 and inhibits tumor cancer cell proliferation and promotes apoptosis by the FGFR3/AKT/mTOR signaling pathway. Berberine has anti-CSC effects as an adjuvant to usual chemotherapy to improve treatment effects in oral cancer (Lin et al., 2017). Anthocyanin limits the feasibility, transfer, and invasion in OSCC cells. Various genes, including APP, EHMT1, ACACB, PCNA, PLAU, FST, HMGA2, LAMC2, and SPP1, were associated with patient survival based on data from The Cancer Genome Atlas (TCGA) (Dai et al., 2020; Yadav et al., 2021). Downregulation of ANO1 by cinobufagin showed the potential anticancer effect of cinobufagin in OSCC tissues. Baicalein inhibited the expression of Sp1, p65, and p50 by reduction of their relative mRNA levels, and it reduced NF-κB activity in OSCC cells. Public data, clinical tissues, and transfected cells were screened for the molecular mechanism of indirect targeting between GLA and CHAC1, and the results demonstrated that GLA significantly inhibits cell proliferation and induces apoptosis. In addition, GLA-upregulated CHAC1 expression participates in ATF4/CHOP signaling during endoplasmic reticulum stress (Wang et al., 2022). High CHAC1 expression also indicates positive survival, as CHAC1 alternatively regulates the number of tumor cells (Wang et al., 2022). GLA has potential tumor therapeutic effects that trigger the ROS-mediated ATF4/CHOP/CHAC1 axis in patients with tumors (Wang et al., 2022). Therefore, HPRT1 is also a potential prognostic biomarker and therapeutic target in OSCC (Wu et al., 2022).
Autophagy is a mechanism by which cellular material is delivered to lysosomes for degradation, allowing basal turnover of cell components and providing energy and macromolecular precursors. Autophagy plays opposing, context-dependent roles in cancer; thus, interventions to both stimulate and inhibit autophagy have been proposed as cancer therapies. In a control group, it was found to suppress tumor expression by the digestion of mutated proteins and impacts further cell mutations and cancer processes (Khan et al., 2020). Lack of nutrients and oxygen (methanolic stress) in the cell environment leads to autophagy, which is typically upregulated in cancer cells and provides the amino acids, free fatty acids, and glucose necessary for tumor survival and proliferation (Khan et al., 2020). Low differentiation and p38 activation are associated with poor prognosis in OSCC. Resveratrol may prevent drug resistance as it leads to cell cycle arrest and, subsequently, to apoptosis and autophagy of cancer cells (Cheng et al., 2022). Genipin deters cell proliferation and stimulates apoptosis, and it was recently shown to induce OSCC cell autophagy via PI3K/AKT/mTOR pathway inhibition (Wei et al., 2020). Interferon-gamma-induced autophagy is a cellular protective process and blocking autophagy flux can promote interferon-gamma-mediated OSCC cell apoptosis. Thus, the combination of interferon-gamma and autophagy inhibitors represents a novel strategy for OSCC therapy (Zhou et al., 2021).
As a local tumor of the head and neck, OSCC has great metastasis and invasiveness potential. Therefore, mRNA diagnostic markers identified in the blood of patients with OSCC are ideal for its early diagnosis and treatment. The occurrence and development of early OSCC, as well as the malignant transformation of potentially malignant disorders, are complex processes that rely on host immunity, TME, and inflammation. Cancer-associated inflammation is considered the seventh marker of cancer, with cancer cells producing pro-inflammatory mediators. Moreover, tumor occurrence and development are related to genetic aberrations in oncogenes, tumor suppressors, and other elements of the TME. Hurdles in the use of biomarkers include developing a means of finding reliable biomarkers and realizing their precise clinical applications. In the future, more biomarkers and their mechanisms will be explored for the treatment of cancer. Currently, data available in the TCGA and GEO public databases greatly contribute to a better understanding of the etiological nature of OSCC. Moreover, body fluids hold considerable research value for the early diagnosis of OSCC. However, how regulation of peripheral blood-circulating biomarkers will affect the outcome of the primary tumor and metastasis remains to be clarified.
Funding Statement: This review was funded by the Key Project of Basic Research of Shenzhen Science and Technology Innovation Commission (Grant Number JCYJ20200109140208058) and the Guangdong Provincial High Level Clinical Key Specialty (Grant Number SZGSP008).
Author Contributions: Xia Mu design and draft the article, Yu-Bing Hu, Dan-Dan Wu and Hong-Yu Yang review the manuscript. All authors reviewed the results and approved the final version of the manuscript.
Availability of Data and Materials: Data sharing not applicable to this article as no datasets were generated or analyzed during the current study.
Ethics Approval: Not applicable.
Conflicts of Interest: The authors declare that they have no conflicts of interest to report regarding the present study.
References
Adeola HA, Bello IO, Aruleba RT, Francisco NM, Adekiya TA, Adefuye AO, Ikwegbue PC, Musaigwa F (2022). The practicality of the use of liquid biopsy in early diagnosis and treatment monitoring of oral cancer in resource-limited settings. Cancers 14: 1139–1153. https://doi.org/10.3390/cancers14051139 [Google Scholar] [PubMed] [CrossRef]
Bu JQ, Bu X, Liu B, Chen F, Chen P (2015). Increased expression of tissue/salivary transgelin mRNA predicts poor prognosis in patients with oral squamous cell carcinoma (OSCC). Medical Science Monitor 21: 2275–2281. https://doi.org/10.12659/MSM.893925 [Google Scholar] [PubMed] [CrossRef]
Chai AWY, Lim KP, Cheong SC (2020). Translational genomics and recent advances in oral squamous cell carcinoma. Seminars in Cancer Biology 61: 71–83. https://doi.org/10.1016/j.semcancer.2019.09.011 [Google Scholar] [PubMed] [CrossRef]
Cheng Y, Chen J, Shi Y, Fang X, Tang Z (2022). MAPK signaling pathway in oral squamous cell carcinoma: Biological function and targeted therapy. Cancers 14: 4625–4629. https://doi.org/10.3390/cancers14194625 [Google Scholar] [PubMed] [CrossRef]
Chi AC, Day TA, Neville BW (2015). Oral cavity and oropharyngeal squamous cell carcinoma—an update. CA: A Cancer Journal for Clinicians 65: 401–421. https://doi.org/10.3322/caac.21293 [Google Scholar] [PubMed] [CrossRef]
Coletta RD, Yeudall WA, Salo T (2020). Grand challenges in oral cancers. Frontiers in Oral Health 1: 1–3. https://doi.org/10.3389/froh.2020.00003 [Google Scholar] [PubMed] [CrossRef]
Dai J, Su Y, Zhong S, Cong L, Liu B, Yang J, Tao Y, He Z, Chen C, Jiang Y (2020). Exosomes: Key players in cancer and potential therapeutic strategy. Signal Transduction and Targeted Therapy 5: 1–10. https://doi.org/10.1038/s41392-020-00261-0 [Google Scholar] [PubMed] [CrossRef]
Darvin P, Toor SM, Sasidharan Nair V, Elkord E (2018). Immune checkpoint inhibitors: Recent progress and potential biomarkers. Experimental, Molecular Medicine 50: 165–176. https://doi.org/10.1038/s12276-018-0191-1 [Google Scholar] [PubMed] [CrossRef]
de Camargo MR, Frazon TF, Inacio KK, Smiderle FR, Amôr NG, Dionísio TJ, Santos CF, Rodini CO, Lara VS (2022). Ganoderma lucidum polysaccharides inhibit in vitro tumorigenesis. cancer stem cell properties and epithelial-mesenchymal transition in oral squamous cell carcinoma. Journal of Ethnopharmacology 286: 114891. https://doi.org/10.1016/j.jep.2021.114891 [Google Scholar] [PubMed] [CrossRef]
Ding L, Hu EL, Xu YJ, Huang XF, Zhang DY, Li B, Hu QG, Ni YH, Hou YY (2015). Serum IL-17F combined with VEGF as potential diagnostic biomarkers for oral squamous cell carcinoma. Tumor Biology 36: 2523–2529. https://doi.org/10.1007/s13277-014-2867-z [Google Scholar] [PubMed] [CrossRef]
Ding L, Zhao X, Zhu N, Zhao M, Hu Q, Ni Y (2020). The balance of serum IL-18/IL-37 levels is disrupted during the development of oral squamous cell carcinoma. Surgical Oncology 32: 99–107. https://doi.org/10.1016/j.suronc.2019.12.001 [Google Scholar] [PubMed] [CrossRef]
Eckert AW, Horter S, Bethmann D, Kotrba J, Kaune T et al. (2019). Investigation of the prognostic role of carbonic anhydrase 9 (CAIX) of the cellular mRNA/protein level or soluble CAIX protein in patients with oral squamous cell carcinoma. International Journal of Molecular Sciences 20: 375–395. https://doi.org/10.3390/ijms20020375 [Google Scholar] [PubMed] [CrossRef]
Fang L, Wang HM, Zhou L, Yu D (2011). Akt-FOXO3a signaling axis dysregulation in human oral squamous cell carcinoma and potent efficacy of FOXO3a-targeted gene therapy. Oral Oncology 47: 16–21. https://doi.org/10.1016/j.oraloncology.2010.10.010 [Google Scholar] [PubMed] [CrossRef]
Faria PCB, Sena AAS, Nascimento R, Carvalho WJ, Loyola AM, Silva SJ, Durighetto AF, Oliveira ADJ, Oliani SM, Goulart LR (2010). Expression of annexin A1 mRNA in peripheral blood from oral squamous cell carcinoma. Oral Oncology 46: 25–30. https://doi.org/10.1016/j.oraloncology.2009.09.003 [Google Scholar] [PubMed] [CrossRef]
Gorenjak V, Vance DR, Petrelis AM, Stathopoulou MG, Dadé S et al. (2019). Peripheral blood mononuclear cells extracts VEGF protein levels and VEGF mRNA: Associations with inflammatory molecules in a healthy population. PLoS One 14: e0220902. https://doi.org/10.1371/journal.pone.0220902 [Google Scholar] [PubMed] [CrossRef]
Greer PFC, Rich A, Coates DE (2022). Effects of galectin-1 inhibitor OTX008 on oral squamous cell carcinoma cells in vitro and the role of AP-1 and the MAPK/ERK pathway. Archives of Oral Biology 134: 105335. https://doi.org/10.1016/j.archoralbio.2021.105335 [Google Scholar] [PubMed] [CrossRef]
Hartanto FK, Karen-Ng LP, Vincent-Chong VK, Ismail SM, Mustafa WM, Abraham MT, Tay KK, Zain RB (2015). KRT13, FAIM2 and CYP2W1 mRNA expression in oral squamous cell carcinoma patients with risk habits. Asian Pacific Journal of Cancer Prevention 16: 953–958. https://doi.org/10.7314/APJCP.2015.16.3.953 [Google Scholar] [PubMed] [CrossRef]
Hasan F, Yadav V, Katiyar T, Yadav S, Pandey R, Mehrotra D, Hadi R, Singh S, Bhatt MLB, Parmar D (2020). Validation of gene expression profiles of candidate genes using low density array in peripheral blood of tobacco consuming head and neck cancer patients and auto/taxi drivers with preneoplastic lesions. Genomics 112: 513–519. https://doi.org/10.1016/j.ygeno.2019.04.003 [Google Scholar] [PubMed] [CrossRef]
Heikenwalder M, Lorentzen A (2019). The role of polarisation of circulating tumour cells in cancer metastasis. Cellular and Molecular Life Sciences 76: 3765–3781. https://doi.org/10.1007/s00018-019-03169-3 [Google Scholar] [PubMed] [CrossRef]
Honarmand MH, Farhad-Mollashahi L, Nakhaee A, Nehi M (2016). Salivary levels of ErbB2 and CEA in oral squamous cell carcinoma patients. Asian Pacific Journal of Cancer Prevention 17: 77–80. https://doi.org/10.7314/APJCP.2016.17.S3.77 [Google Scholar] [PubMed] [CrossRef]
Hong KO, Kim JH, Hong JS, Yoon HJ, Lee JI, Hong SP, Hong SD (2009). Inhibition of Akt activity induces the mesenchymal-to-epithelial reverting transition with restoring E-cadherin expression in KB and KOSCC-25B oral squamous cell carcinoma cells. Journal of Experimental and Clinical Cancer Research 28: 28. https://doi.org/10.1186/1756-9966-28-28 [Google Scholar] [PubMed] [CrossRef]
Hu P, Zhang W, Xin H, Deng G (2016). Single cell isolation and analysis. Frontiers in Cell and Developmental Biology 4: 116. https://doi.org/10.3389/fcell.2016.00116 [Google Scholar] [PubMed] [CrossRef]
Huang GZ, Chen ZQ, Wu J, Shao TR, Zou C, Ai YL, Lv XZ (2021). Pan-cancer analyses of the tumor microenvironment reveal that ubiquitin-conjugating enzyme E2C might be a potential immunotherapy target. Journal of Immunology Research 2021: 27. https://doi.org/10.1155/2021/9250207 [Google Scholar] [PubMed] [CrossRef]
Ju H, Mao L, Zhang L, Liu S, Wu Y, Ruan M, Hu J, Ren G (2018). Ultrasound hyperthermia enhances chemo-sensitivity in oral squamous cell carcinoma by TRIF-mediated pathway. Journal of Oral Pathology, Medicine 47: 964–971. https://doi.org/10.1111/jop.12774 [Google Scholar] [PubMed] [CrossRef]
Khan T, Relitti N, Brindisi M, Magnano S, Zisterer D, Gemma S, Butini S, Campiani G (2020). Autophagy modulators for the treatment of oral and esophageal squamous cell carcinomas. Medicinal Research Reviews 40: 1002–1060. https://doi.org/10.1002/med.21646 [Google Scholar] [PubMed] [CrossRef]
Khani M, Mohamadnia A, Hosseini F, Bayat P, Bahrami N (2019). Assessing VEGF mRNA and miR–494 expression in peripheral blood collected from patients suffering from oral squamous cell carcinoma. Journal of Craniomaxillofacial Research 6: 1–7. https://doi.org/10.18502/jcr.v6i1.1628 [Google Scholar] [CrossRef]
Kitamura R, Toyoshima T, Tanaka H, Kawano S, Matsubara R, Goto Y, Jinno T, Maruse Y, Oobu K, Nakamura S (2017). Cytokeratin 17 mRNA as a prognostic marker of oral squamous cell carcinoma. Oncology Letters 14: 6735–6743. https://doi.org/10.3892/ol.2017.7066 [Google Scholar] [PubMed] [CrossRef]
Li X, Bu W, Meng L, Liu X, Wang S, Jiang L, Ren M, Fan Y, Sun H (2019). CXCL12/CXCR4 pathway orchestrates CSC-like properties by CAF recruited tumor associated macrophage in OSCC. Experimental Cell Research 378: 131–138. https://doi.org/10.1016/j.yexcr.2019.03.013 [Google Scholar] [PubMed] [CrossRef]
Li MH, Liao X, Li C, Wang TT, Sun YS et al. (2021). Lycorine hydrochloride induces reactive oxygen species-mediated apoptosis via the mitochondrial apoptotic pathway and the JNK signaling pathway in the oral squamous cell carcinoma HSC-3 cell line. Oncology Letters 21: 236. https://doi.org/10.3892/ol.2021.12497 [Google Scholar] [PubMed] [CrossRef]
Li Z, Yan T, Wu X, Zhang W, Li J, Wang L, Yang J (2020). Increased expression of FOXD1 is associated with cervical node metastasis and unfavorable prognosis in oral squamous cell carcinoma. Journal of Oral Pathology Medicine 49: 1030–1036. https://doi.org/10.1111/jop.13098 [Google Scholar] [PubMed] [CrossRef]
Lin CY, Hsieh PL, Liao YW, Peng CY, Lu MY, Yang CH, Yu CC, Liu CM (2017). Berberine-targeted miR-21 chemosensitizes oral carcinomas stem cells. Oncotarget 8: 80900–80908. https://doi.org/10.18632/oncotarget.20723 [Google Scholar] [PubMed] [CrossRef]
Liu W, Wang J, Zhang C, Bao Z, Wu L (2022). Curcumin nanoemulsions inhibit oral squamous cell carcinoma cell proliferation by PI3K/Akt/mTOR suppression and miR-199a upregulation: A preliminary study. Oral Diseases 18: 233. https://doi.org/10.1111/odi.14271. [Google Scholar] [PubMed] [CrossRef]
Maki M, Saitoh K, Kaneko Y, Fukayama M, Morohoshi T (2019). Expression of cytokeratin 19 (CK19and vascular endothelial growth factor (VEGFgenes in peripheral blood of patients with oral squamous cell carcinoma (OSCC). Journal of Isfahan Medical School 37: 480–484. https://doi.org/10.22122/JIMS.V37I526.11826 [Google Scholar] [CrossRef]
Musfee FI, Guo D, Pinard AC, Hostetler EM, Blue EE, Nickerson DA (2020). Rare deleterious variants of NOTCH1, GATA4, SMAD6, and ROBO4 are enriched in BAV with early onset complications but not in BAV with heritable thoracic aortic disease. Molecular Genetics and Genomic Medicine 8: e1406. https://doi.org/10.1002/mgg3.1406 [Google Scholar] [PubMed] [CrossRef]
Nabavi P, Mohamadnia A, Naseroleslami M, Bahrami N (2019). Expression of cytokeratin 19 (CK19) and vascular endothelial growth factor (VEGF) genes in peripheral blood of patients with oral squamous cell carcinoma. Journal of Isfahan Medical School 37: 480–484. https://doi.org/10.22122/JIMS.V37I526.11826 [Google Scholar] [CrossRef]
Nissinen L, Farshchian M, Riihilä P, Kähäri VM (2016). New perspectives on role of tumor microenvironment in progression of cutaneous squamous cell carcinoma. Cell and Tissue Research 365: 691–702. https://doi.org/10.1007/s00441-016-2457-z [Google Scholar] [PubMed] [CrossRef]
Ono S, Suzuki S, Kondo Y, Okubo I, Goto M et al. (2022). Trametinib improves Treg selectivity of anti-CCR4 antibody by regulating CCR4 expression in CTLs in oral squamous cell carcinoma. Scientific Reports 12: 21678. https://doi.org/10.1038/s41598-022-22773-1 [Google Scholar] [PubMed] [CrossRef]
Ou D, Wu Y (2021). The prognostic and clinical significance of IFI44L aberrant downregulation in patients with oral squamous cell carcinoma. BMC Cancer 21: 1–11. https://doi.org/10.1186/S12885-021-09058-Y/FIGURES/5 [Google Scholar] [CrossRef]
Pandruvada SN, Yuvaraj S, Liu X, Sundaram K, Shanmugarajan S, Ries WL, Norris J, London SD, Reddy SV (2010). Role of CXC chemokine ligand 13 in oral squamous cell carcinoma associated osteolysis in athymic mice. International Journal of Cancer 126: 2319–2329. https://doi.org/10.1002/IJC.24920 [Google Scholar] [PubMed] [CrossRef]
Pang X, Fan HY, Tang YL, Wang SS, Cao MX et al. (2020). Myeloid derived suppressor cells contribute to the malignant progression of oral squamous cell carcinoma. PLoS One 15: 229089. https://doi.org/10.1371/journal.pone.0229089 [Google Scholar] [PubMed] [CrossRef]
Payehghadr S, Bahrami N, Naji T, Mohamadnia A, Mohammadi F (2018). Detection of miR-21. MUC1mRNA and VEGF protein biomarkers expression changes in oral squamous cell carcinomas (OSCCin peripheral blood. Asian Pacific Journal of Cancer Biology 3. https://doi.org/10.31557/apjcb.2018.3.2.59-64 [Google Scholar] [CrossRef]
Rai R, Mahale A, Saranath D (2004). Molecular cloning, isolation and characterisation of ERK3 gene from chewing-tobacco induced oral squamous cell carcinoma. Oral Oncology 40: 705–712. https://doi.org/10.1016/J.ORALONCOLOGY.2004.01.010 [Google Scholar] [PubMed] [CrossRef]
Russell SM, Angell TE, Lechner MG, Liebertz DJ, Correa AJ, Sinha UK, Kokot N, Epstein AL (2013). Immune cell infiltration patterns and survival in head and neck squamous cell carcinoma. Head and Neck Oncology. 5: 24. [Google Scholar] [PubMed]
Singh B, Aggarwal B, Das P, Srivastava SK, Sharma SC, Das SN (2022a). Over expression of cancer stem cell marker CD44 and its clinical significance in patients with oral squamous cell carcinoma. Indian Journal of Otolaryngology and Head and Neck Surgery 52: 1–6. https://doi.org/10.1007/s12070-022-03200-3 [Google Scholar] [PubMed] [CrossRef]
Singh RD, Patel KA, Patel JB, Pandya SJ, Patel PS (2022b). Evidence of association of CYP1A1 expression in blood lymphocytes and clinicopathological variables in oral cancer. Indian Journal of Clinical Biochemistry 37: 178–184. https://doi.org/10.1007/s12291-021-00958-1 [Google Scholar] [PubMed] [CrossRef]
Song H, Lai L, Liu M, Wang X, Zhang J, Zhang S (2020). Investigating the role and mechanism of microRNA-196a in oral squamous cell carcinoma by targeting FOXO1. Experimental and Therapeutic Medicine 19: 3707–3715. https://doi.org/10.3892/etm.2020.8614 [Google Scholar] [PubMed] [CrossRef]
Srivastava S, Ghosh SK (2015). Modulation of L-arginine-arginase metabolic pathway enzymes: Immunocytochemistry and mRNA expression in peripheral blood and tissue levels in head and neck squamous cell carcinomas in North East India. Asian Pacific Journal of Cancer Prevention 16: 7031–7038. https://doi.org/10.7314/APJCP.2015.16.16.7031 [Google Scholar] [PubMed] [CrossRef]
Torres López M, Pérez Sayáns M, Chamorro Petronacci C, Barros Angueira F, Gándara Vila P, Lorenzo Pouso A, García García A (2018). Determination and diagnostic value of CA9 mRNA in peripheral blood of patients with oral leukoplakia. Journal of Enzyme Inhibition and Medicinal Chemistry 33: 951–955. https://doi.org/10.1080/14756366.2018.1466120 [Google Scholar] [PubMed] [CrossRef]
Toyoshima T, Vairaktaris E, Nkenke E, Schlegel KA, Neukam FW, Ries S (2007). Cytokeratin 17 mRNA expression has potential for diagnostic marker of oral squamous cell carcinoma. Journal of Cancer Research and Clinical Oncology 134: 515–521. https://doi.org/10.1007/s00432-007-0308-8 [Google Scholar] [PubMed] [CrossRef]
Vora HH, Shah NG, Trivedi TI, Goswami JV, Shukla SN, Shah PM (2007). Expression of C-Myc mRNA in squamous cell carcinoma of the tongue. Journal of Surgical Oncology 95: 70–78. https://doi.org/10.1002/(ISSN)1096-9098 [Google Scholar] [CrossRef]
Wang Y, Fang Y, Lu P, Wu B, Zhou B (2021). NOTCH signaling in aortic valve development and calcific aortic valve disease. Frontiers in Cardiovascular Medicine 8: 389–417. https://doi.org/10.1214/10-AOS846 [Google Scholar] [CrossRef]
Wang X, He MJ, Chen XJ, Bai YT, Zhou G (2022). Glaucocalyxin A impairs tumor growth via amplification of the ATF4/CHOP/CHAC1 cascade in human oral squamous cell carcinoma. Journal of Ethnopharmacology 290: 115100. https://doi.org/10.1016/j.jep.2022.115100 [Google Scholar] [PubMed] [CrossRef]
Weber M, Wehrhan F, Baran C, Agaimy A, Büttner-Herold M, Kesting M, Ries J (2019). Prognostic significance of PD-L2 expression in patients with oral squamous cell carcinoma-A comparison to the PD-L1 expression profile. Cancer Medicine 8: 1124–1134. https://doi.org/10.1002/cam4.1929 [Google Scholar] [PubMed] [CrossRef]
Weber M, Wehrhan F, Baran C, Agaimy A, Büttner-Herold M, Preidl R, Neukam FW, Ries J (2017). PD-L1 expression in tumor tissue and peripheral blood of patients with oral squamous cell carcinoma. Oncotarget 8: 112584–112597. https://doi.org/10.18632/oncotarget.22576 [Google Scholar] [PubMed] [CrossRef]
Wehrhan F, Weber M, Baran C, Agaimy A, Büttner-Herold M, Kesting M, Ries J (2021). PD1 expression and correlation with its ligands in oral cancer specimens and peripheral blood. Journal of Cranio-Maxillofacial Surgery 49: 118–125. https://doi.org/10.1016/j.jcms.2020.12.007 [Google Scholar] [PubMed] [CrossRef]
Wei M, Wu Y, Liu H, Xie C (2020). Genipin induces autophagy and suppresses cell growth of oral squamous cell carcinoma via PI3K/AKT/MTOR pathway. Drug Design, Development and Therapy 14: 395–405. [Google Scholar] [PubMed]
Wu T, Jiao Z, Li Y, Su X, Yao F, Peng J, Chen W, Yang A (2022). HPRT1 promotes chemoresistance in oral squamous cell carcinoma via activating MMP1/PI3K/Akt signaling pathway. Cancers 14: 855. https://doi.org/10.3390/cancers14040855 [Google Scholar] [PubMed] [CrossRef]
Yadav M, Pradhan D, Singh RP (2021). Integrated analysis and identification of nine-gene signature associated to oral squamous cell carcinoma pathogenesis. 3 Biotech 11: 1–15. https://doi.org/10.1007/s13205-021-02737-4 [Google Scholar] [PubMed] [CrossRef]
Yu T, Tang Q, Chen X, Fan W, Zhou Z, Huang W, Liang F (2021). TGF-β1 and IL-17A comediate the protumor phenotype of neutrophils to regulate the epithelial-mesenchymal transition in oral squamous cell carcinoma. Journal of Oral Pathology Medicine 50: 353–361. https://doi.org/10.1111/jop.13122 [Google Scholar] [PubMed] [CrossRef]
Zhang Y, Wang Y, Wang J, Zhang K (2013). Immune cell infiltration patterns and survival in head and neck squamous cell carcinoma. Head and Neck Oncology 5: 24. [Google Scholar]
Zhao M, He Y, Zhu N, Song Y, Hu Q, Wang Z, Ni Y (2022). IL-33/ST2 signaling promotes constitutive and inductive PD-L1 expression and immune escape in oral squamous cell carcinoma. British Journal of Cancer 394: 1915. https://doi.org/10.1038/s41416-022-02090-0 [Google Scholar] [PubMed] [CrossRef]
Zhou ZH, Zhao TC, Liang SY, Zhang ZY, Zhu DW, Ju WT, Zhong LP (2021). A therapeutic approach with combination of interferon-gamma and autophagy inhibitor for oral squamous cell carcinoma. American Journal of Cancer Research 11: 1503–1521. https://doi.org/10.1186/S12885-021-09058-Y/FIGURES/5 [Google Scholar] [CrossRef]
Cite This Article
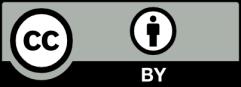