Open Access
REVIEW
Development of micro/nanostructured‒based biomaterials with biomedical applications
Department of Clinical Laboratory Sciences, College of Applied Medical Sciences, Taif University, Taif, 21944, Saudi Arabia
* Corresponding Author: AFAF ALHARTHI. Email:
(This article belongs to the Special Issue: Recent Advances in Nanomaterials in Plant Biostimulation)
BIOCELL 2023, 47(8), 1743-1755. https://doi.org/10.32604/biocell.2023.027154
Received 17 October 2022; Accepted 10 February 2023; Issue published 28 August 2023
Abstract
Natural biomaterials are now frequently used to build biocarrier systems, which can carry medications and biomolecules to a target region and achieve a desired therapeutic effect. Biomaterials and polymers are of great importance in the synthesis of nanomaterials. The recent studies have tended to use these materials because they are easily obtained from natural sources such as fungi, algae, bacteria, and medicinal plants. They are also biodegradable, compatible with neighborhoods, and non-toxic. Natural biomaterials and polymers are chemically changed when they are linked by cross linking agents with other polymers to create scaffolds, matrices, composites, and interpenetrating polymer networks employing microtechnology and nanotechnology. This review highlights how microengineered and nanoengineered biomaterials are utilized to produce efficient drug-delivery systems and biomedical and biological therapies and how innovative sources of biomaterials have been identified.Keywords
Synthesized microdevices and nanodevices have proved invaluable in biotechnology and biomedicine. Since 2012, biocompatible and biodegradable microparticles, of 0.1 to 1 µm size, have gained much attention as ideal drug carriers. Formulations using microparticles have a wide range of applications, such as oral vehicles for drug delivery, subcutaneous injections, intramuscular injections, and site-specific delivery vehicles. Furthermore, polymeric particulate-based materials have been widely used in the delivery of medications. Some of the critical factors for choosing a suitable polymer are the physicochemical properties, thermosensitivity, and pH-responsivity to the surrounding environment (Bao et al., 2016; Onck et al., 2005; Yilmaz et al., 2016).
Nanobiotechnology is a multidisciplinary field that combines chemistry, biology, engineering, and medicine and has been the foundation of efforts for developing drug-delivery devices and methods. Site-specific targeting and the controlled release of conventional medicines, recombinant proteins, vaccines, and nucleic acids have been made possible with novel materials and formulations (Anderson et al., 2004). Nanoscale drug-delivery devices can be developed to manage the biodistribution of drugs, optimize release kinetics, and reduce toxic side effects, thus increasing the therapeutic index of a drug (Kayser et al., 2005).
Although current drug-delivery systems are efficient at producing high local concentrations of medications through controlled drug release, they are only able to target tissues rather than specific cells (Qiu and Bae, 2006). Since the mid-twentieth century, various metallic nanoparticles (NPs) have been biosynthesized by microorganisms through genetic modification, and extracts of these microorganisms could form metallic NPs. These biometallic NPs exhibit higher catalytic activity, are more stable and are less toxic than chemically produced metallic NPs. Furthermore, biometallic NPs can be generated by culturing microorganisms or cell-free extracts with dissolved metal ions for hours or days utilizing green and environmentally acceptable methods. Some of the metals that have been used in this type of synthesis to form NPs are silver, gold, cadmium sulfide, cadmium selenide, copper, copper oxide, gadolinium oxide, ferrimagnetic magnetite, lead sulfide, palladium, antimony trioxide, titanium dioxide, and zirconium dioxide (Kato and Suzuki, 2020; Sharaf et al., 2022).
Numerous characteristics of nanoscale drug-delivery systems can be modified for particular uses in terms of their capabilities of drug release (physical interaction between drug and carrier, chemical cleavage of covalent spacer), drug encapsulation (physical interaction between drug and carrier), biodistribution (molecular weight, addition of targeting group), biocompatibility (electrical charge, addition of bioinert functionality), and biodegradability (backbone, spacer), as well as their shape (materials and chemistry employed) (Qiu and Bae, 2006; Rozan et al., 2022). Their unique size-dependent properties make these materials superior to other delivery systems and indispensable in many areas of human activity (Salata, 2004). This brief review attempts to summarize the most recent developments in the field of applied nanomaterials, particularly, their applications in biology and medicine, and discussed their prospects for commercialization.
Microtechnology and nanotechnology: A preamble
Microtechnology and nanotechnology are used for the fabrication of materials of micron and submicron scales, respectively. When utilizing materials in a nanoform, the field of nanotechnology blends science and technology. It focuses on the synthesis of materials at scales ranging from 1 to 100 nm with applications in various industries, including agriculture, medicine, and the pharmaceutical industry; environmental applications; and uses in other fields (Elegbede and Lateef, 2020; Lateef et al., 2018). This technology is developing rapidly, and scientists and researchers are working hard to incorporate new ideas that involve synthesis or new applications (Lateef et al., 2021). Ongoing studies are focusing on the composition, structure, morphology, and other aspects of nanoscale formulations, and further development of such characterizations is required (Bai et al., 2022). Nanomaterials’ physical, chemical, and biological characteristics are fundamentally distinct from those of individual atoms, molecules, and bulk materials at the nanoscale (Khan et al., 2022). Their greater surface area and quantum effects set them apart from other materials in a significant way. Their larger surface area often results in more chemically reactive qualities and also impacts the mechanical or electrical properties of the materials (Findik, 2021).
A recent subfield of nanotechnology, nanobiotechnology, has created particles with specific forms and functions by integrating biological methods with physical and chemical processes. Nowadays, metal and metal oxide NPs formed using silver, platinum, selenium, iron, and gold, as well as copper oxide, zinc oxide, and titanium dioxide, are quite popular because of their numerous beneficial features in a variety of application domains (Aygün et al., 2020; El-Batal et al., 2020; Elfeky et al., 2020). Many of the damaging impacts of physical and chemical methods, such as a long processing time and the use of toxic ethylene glycol and organic reagents, can be avoided by green procedures employing diverse biological entities. Nanomaterial-based green assemblies are widely used for synthesis; they mostly rely on plants, bacteria, algae, fungi, actinomycetes, and arthropod metabolites to produce nanomaterials free of contaminants (Fouda et al., 2020; Lotha et al., 2019). These living things, notably fungal cells, are capable of producing molecules and active substances that stabilize and reduce the formation of nanomaterials with a variety of forms, physiochemical properties, and compositional features (Feroze et al., 2020; Salem et al., 2021).
Microbial synthesis of nanomaterials
Microbial nanotechnology, which combines microbiology with nanotechnology, uses microbes as nanostructures. Microorganisms are regarded as the perfect sources of nanostructures for microbial nanotechnology (Rehman et al., 2020a; Shobha et al., 2020) because of their extensive physiological variety, tiny size, and genetic malleability. Fig. 1 shows the relationship between microbiology and nanotechnology for the development of nanobiotechnology-based microbial structures. It refers to a wide world encompassing bacterial, mycobacterial, viral, immunological, parasitological, protozoological, phycological, and nematological efforts (Saba et al., 2021).
Figure 1: Relationship between microbiology and nanotechnology for the development of nanobiotechnology-based microbial (Ter-Zakaryan and Zhukov, 2021).
Green synthesis of nanoparticles by microorganisms
Various techniques have been employed for NP synthesis, including physical, chemical, and biological ones. Physicochemical approaches for NP synthesis have several drawbacks, including the use of costly machinery, the high generation of heat from the use of organic solutions that can be toxic, the limited capacity for solubility of substances with high melting points (Modan and Plăiașu, 2020), and the high energy consumption, as well as the limited yield of NPs (Gahlawat and Choudhury, 2019; Soni et al., 2018).
The usage of hazardous chemicals, which can lead to environmental issues, is the fundamental disadvantage of these techniques (Pal et al., 2019). There is now a demand for an ecologically benign method of producing NPs, with the current focus being on green synthesis methods using biological resources, such as plants, microbes, enzymes, polysaccharides, and degradable polymers (Roychoudhury, 2020). Due to its simplicity and cost-effectiveness and the fact that it does not require the use of hazardous or ecologically harmful substances, green synthesis is more advantageous than conventional physical and chemical procedures. Thus, it has become increasingly important in recent years (Pal et al., 2019).
One of two approaches, generally referred to as the top-down and bottom-up approaches, is used to synthesize NPs. In the top-down approach, bulk materials are broken down into nanosized particles by various physical and chemical processes (Gahlawat and Choudhury, 2019; Prasad, 2019). The primary flaw of this method is that the NPs created have an irregular surface texture. This method is not ideal for large-scale manufacturing processes because it is a costly and time-consuming approach (Prasad, 2019). Bottom-up methods develop NPs by allowing atomic and molecular structures to self-assemble, giving them a more exact size, shape, and molecular makeup (Gahlawat and Choudhury, 2019). Fig. 2 illustrates how this technique uses both chemical and biological manufacturing methods.
Figure 2: Synthesis of NPs either top-down or down-top approach.
Approaches and mechanisms of biosynthesis of nanoparticles by microorganisms
Most microorganisms have the potential to act as biofactories for the synthesis of eco-friendly and inexpensive NPs. Of these, the metals gold, silver, copper, zinc, and titanium, palladium, and nickel, are widely used. They can produce NPs with a predetermined form, size, content, and particle monodispersity (Fouda et al., 2018; Kato and Suzuki, 2020; Khan et al., 2018). The biosynthesis of NPs with microorganisms can be achieved by capturing target metal ions from their surroundings and converting them enzymatically into an elemental form via a reduction mechanism (Fouda et al., 2018).
NPs are formed along metabolic or biosynthetic pathways and/or by cellular enzymes not present in all species; each microbe has a different metabolic process and enzymatic activity (Ramos et al., 2020). Microorganisms must be able to tolerate heavy metals to produce NPs. High metal stress and several other parameters are important in determining the rate of production, yield, and morphologic features of NPs, including their temperature, pH, the concentration of metal precursor, and reaction time (Saxena et al., 2016). These parameters also affect a variety of microbial processes. Certain bacteria are capable of reducing metal ions to a suitable metallic material while under stress (Husseiny et al., 2015). In most cases, microorganisms that live in metal-rich ecosystems are extremely resistant to those metals because of the absorption and chelation of those metals by intracellular and extracellular proteins. Therefore, techniques based on imitating the natural biomineralization process may be advantageous for the synthesis of NPs (Rabeea et al., 2020).
Fig. 3 is a schematic representation of the intracellular and extracellular pathways involved in the production of NPs. Through electrostatic interactions, metallic ions (positively charged) are deposited in cell walls (negatively charged). Ions are reduced through metabolic processes after being transported into the microorganism’s cells to produce NPs, controlled by enzymes such as nitrate reductase. Subsequently, the NPs gathered in the periplasmic region might pass through the cell wall (Khan et al., 2018).
Figure 3: Mechanism of biosynthesis of nanoparticles by microorganisms.
Nitrate reductase is involved in the extracellular production of NPs. Reductase enzymes are found in the cell wall or released from the cell into the growth medium, and they create the NPs. The nitrate reductase converts metal ions to their metallic forms during this process. Microorganisms have various components critical to the reduction of NPs, including proteins, enzymes, and other biological molecules (Mohd Yusof et al., 2019).
Studies have demonstrated that the synthesis of Mt NP is carried out by NADH-dependent enzymes. By using NADH-dependent reductases as the electron carriers, the reduction pathways appear to start by transferring an electron from NADH (Jain et al., 2011). Additionally, microbes can release proteins that function largely as stabilizing agents, ensuring colloidal stability and inhibiting Mt NP clumping (Mohd Yusof et al., 2019).
Effects of microbiologic synthesis factors of nanoparticles
There are some factors that can impact NP production, including the pH and temperature and the presence of specific enzymes; and the microbial biomass, precursor concentrations, and time of contact (Fig. 4). In order to maximize the production of silver NPs, zinc oxide NPs, and gold NPs from Trichoderma spp., Sclerotinia sclerotiorum, Aspergillus terreus, Aspergillus niger, and Cladosporium cladosporioides, researchers have looked at the temperature, inoculum of the fungal biomass, pH, and precursor concentration (Fouda et al., 2018; Ramos et al., 2020; Saxena et al., 2016). They demonstrated how improving the aforementioned variables could considerably boost the production of these three NPs, and their mycosynthesis. Additionally, the size of the silver NPs produced by Fusarium oxysporum during mycosynthesis was significantly affected by the optimization of both the physical and cultural circumstances (Husseiny et al., 2015).
Figure 4: Factors affecting biosynthesizing of nanoparticles by microbiology.
Myconanotechnology is a field of science that combines mycology and nanotechnology (Rabeea et al., 2020). In this developing field, fungi may be used to synthesize NPs or nanostructures with desired shapes and sizes (Fouda et al., 2018; Subramaniyan et al., 2018). A simple technique for rapidly generating stable biological NPs is mycosynthesis or mycofabrication. The effective and inexpensive synthesis of NPs can be done by simply cultivating fungi because they contain substantial metabolites (carbohydrates, proteins, and lipids) with better bioaccumulation capabilities and simple downstream handling (Fouda et al., 2019).
Advantages of using fungi in nanoparticle synthesis
Fungi-mediated green NP generation has several advantages, such as simple and straightforward scaling up, simple processing, economic viability, good biomass processing, and good recovery of significant surface distances with the optimal outgrowth of mycelia (Abdelhakim et al., 2020; Salem et al., 2021). From an economic standpoint, the utilization of a fungal biomass filtrate containing various metabolites as a green way of producing metallic NPs was superior to previous biological techniques (Mohamed et al., 2019). Additionally, different kinds of fungi grow swiftly and produce vast masses of fungal cells, and they can easily be kept safe in a laboratory (Elegbede et al., 2020). As a result, extracellular synthesis of NPs is preferable to intracellular synthesis and is suitable for large-scale production. Research on intracellular manufacturing is possible for theoretical academic research. The employment of basidiomycetes for the creation of NPs has yet to be substantially researched, whereas syntheses utilizing lesser fungi and bacteria have been studied. The fungal biological system has environmentally benign and energy-saving regeneration characteristics. The most well-known species of mushrooms are found in the division Basidiomycota, order Polyporales. Over 1800 species of fungi are found in this division. Academic theoretic investigations on intracellular manufacturing are possible (Rehman et al., 2020b).
Basidiomycetes are advantageous for the green production of nanoscale particles because they are nonpathogenic and non-toxic and can be grown in pure cultures (Rehman et al., 2019). Due to their abilities to produce large amounts of protein and high yields of particles, their low residual toxicity, and ease of handling, fungi are appealing as reducing and stabilizing agents in the biogenic manufacture of silver NPs (Rehman et al., 2019). Due to its many advantages, including its low cost, ease of use and control, and exceptional qualities, F. oxysporum is used to manufacture NPs. It should receive greater publicity because it might be a practical solution for the industrial production of NPs (Zielonka and Klimek-Ochab, 2017). As shown in Table 1, several fungal species can be potentially used for preparations.
Advantages of using bacteria in nanoparticle synthesis
Due to their high yield and ease of handling, bacteria are desirable for use in biotechnological applications such as the manufacture of NPs. Bacteria have a built-in defense mechanism called CRISPR Cas, which enables them to deal with challenges such as changes in pH and hazardous environments brought on by concentrations of metal ions, among other things (Dauthal and Mukhopadhyay, 2016). There are certain disadvantages, too, such as a sluggish rate of synthesis, the difficulty of controlling the size and form of the NPs, and sterile conditions. As a result, employing plants and their components as an alternative for producing NPs is an option. Because plants are non-toxic, are naturally capable of capping ends, contain reducing metal ions, and have the capacity to collect heavy metals in their cells, they have attracted the interest of scientists all over the world (Marooufpour et al., 2019).
As bionanofactories, several metallic NPs, including those of silver, gold, copper, selenium, and iron, as well as metal oxide NPs, including silver oxide, copper oxide, zinc oxide, and titanium dioxide, have been produced. It is important to control the conditions of the production process since the pH, the material utilized, the presence of light, the concentration of metal ions, and the temperature all have the potential to impact the shape and size of metallic NPs, as well as the efficiency of manufacturing them. Therefore, metallic NP biosynthesis is particularly significant when extremophilic bacteria are used that have naturally evolved to survive under severe environmental circumstances (e.g., high concentrations of metals, high and low temperatures, an acidic or alkaline pH, high pressure, high levels of salt, and high levels of radiation) (Atalah et al., 2022).
Similar characteristics of other bacteria enable the control of the NPs’ sizes. For instance, the thermophilic strain GWE1 of Geobacillus wiegelii, isolated from a drying oven, could create nonmetallic NPs. The microorganism could make elemental tellurium NPs when exposed to the sodium tellurite salt, and it could convert the selenium ion Se4+ to elemental selenium (SeO) and produce selenium NPs both within and outside of cells when exposed to sodium selenite. The pH and temperature have been demonstrated to affect the size and shape of selenium NPs generated by this microorganism (Atalah et al., 2022). Adding 5% polyvinylpyrrolidone to the culture medium caused the NPs to shrink by 52% (da Silva et al., 2019).
Advantages of using algae in nanoparticle synthesis
Using algae for the biosynthesis of NPs is becoming increasingly popular (Table 2). Phyconanotechnology is a very young area of nanoscience that deals with the use of algae to create NPs. Algae are employed to make NPs because they can grow at low temperatures, have a high capacity to absorb metal, are simple to handle and maintain, and are less harmful to the environment than some other entities (Negi and Singh, 2018) .
Algae are frequently used these days for the production of NPs because of their remarkable ability to absorb metals and decrease metal ions, relatively inexpensive cost when used in manufacturing, and, most importantly, their ability to be used for the large-scale synthesis of NPs (Rajkumar et al., 2021; Singh and Singh, 2019).
Their greater capacity to withstand extreme climatic conditions than other microbes is an additional intriguing characteristic (Khan et al., 2019). An algal biomass may be utilized to create both alive and dead NPs, which is why these organisms are referred to as bionanofactories (Omar et al., 2017). An additional benefit of employing algae is the duration of time necessary for the manufacture of silver NPs. Compared with other microorganisms, algae-mediated synthesis is faster. Synthesis of silver NPs from Escherichia coli takes around 60 h (Baltazar-Encarnación et al., 2019).
Typically, an algal species produces an NP by first accumulating and then decreasing cations. The extracellular or intracellular processes can be used to produce them from the algal biomass (Fig. 5). In contrast to the intracellular process, which involves enzyme activity inside the cell wall and cell membrane, the bioreduction of a metal ion to its NP occurs on the surface of the algal cell in the extracellular pathway (Negi and Singh, 2018). Algae play an important role in the production of silver NPs under a variety of physical, chemical, and environmental circumstances. The extract or biomass content, pH, incubation period, lighting, precursor concentration, and temperature are a few of the crucial variables.
Figure 5: Method of nanoparticles biosynthesized by algae.
Advantages of using medicinal plants in nanoparticle synthesis
Reports of microbe-based synthesis procedures date back to at least 1989. In contrast, the biological creation of NPs from plants did not start until 2002 (Singh et al., 2020). The primary limitations of aseptic conditions and complex procedures, such as microbial isolation, growth optimization, and maintenance, which demand trained personnel and slow reaction times, increase the scaling-up cost (Herlekar et al., 2014). They are the major drawbacks of microbe-mediated NP synthesis. On the other hand, depending on the kinds of plants employed and the concentration of phytochemicals, plant-mediated NP production can be finished in a few minutes or hours (Ameta et al., 2018). Plant-mediated synthesis of metallic NPs is gaining in popularity due to its ease of use, the speed of producing NPs with a variety of morphologic characteristics, lack of need for labor-intensive cell culture maintenance, and environmental friendliness (Velmurugan et al., 2016). Plant extracts are employed in various ways to manufacture NPs, as shown in Fig. 6, and different types of NPs are produced by biosynthesis, as shown in Table 3. These methods save money, are environmentally beneficial, and do not require complicated procedures (Celik et al., 2020; Koca et al., 2020).
Figure 6: Techniques are used for the synthesis of nanoparticles via plant extracts and its antibacterial mechanism.
However, the green synthesis of NPs using plant materials has more advantages, such as environmentally friendly behavior, less toxicity, lower cost, more biocompatibility, better profile for controlling the size, and biocompatibility (Bhardwaj et al., 2020). The metal NPs derived from plant extracts have been shown to be biocompatible and also nontoxic, and have therefore, recently received much attention. These NPs have antiviral, antibacterial, and anticancer properties (Mittal et al., 2013; Rajan et al., 2015), offering a great potential for targeted drug delivery (Table 4).
NPs and microparticles derived from molecular substrates such as algae, fungi, bacteria, and medicinal plants have been widely employed for several biological applications. The primary goal of nanotechnology is to develop reliable stream-lined production methods that regulate the chemical composition, morphologic features, and monodispersing systems in the large-scale production of nanomaterials. NPs offer good possibilities for the delivery of medications, imaging, and biosensing because of their tiny size, which allows them to permeate tissues and be ingested by cells. The most recent developments in the use of NPs for diverse therapeutic purposes have been highlighted in a number of studies cited in this review. Numerous eco-friendly designs for the synthesis of NPs from plants, bacteria, and fungi (green synthesis) have been mentioned and recommended because of their environmentally friendly attributes, low toxicity, low cost, good biocompatibility, good profile for controlling the size of NPs, and biocompatibility. The use of NPs for antimicrobial and cancer therapies is one area that has attracted much interest. The designs show significant promise for delivering medicines directly to tumors while limiting side effects related to hazardous chemotherapies. The benefits are due to the tiny size of these particles, their tendency to collect in tumors, and their ability to attach to the outer membranes of bacteria. The use of NPs and microparticles for immunological therapies has also garnered significant interest.
Acknowledgement: The researchers would like to acknowledge the Deanship of Scientific Research, Taif University for funding this work.
Funding Statement: The author received no specific funding for this study.
Author Contributions: Afaf Alharthi confirmed contribution to the paper as follows: study conception and design, data collection, analysis and interpretation, draft manuscript preparation. All author approved the final version of the manuscript.
Availability of Data and Materials: All data is available when requested.
Ethics Approval: Not Applicable.
Conflicts of Interest: The author declares that they have no conflicts of interest to report regarding the present study.
References
Abdelhakim H, El-Sayed E, Rashidi F (2020). Biosynthesis of zinc oxide nanoparticles with antimicrobial, anticancer, antioxidant and photocatalytic activities by the endophytic Alternaria tenuissima. Journal of Applied Microbiology 128: 1634–1646. https://doi.org/10.1111/jam.14581 [Google Scholar] [PubMed] [CrossRef]
Ameta RK, Shankar KR, Singh M (2018). Plant extract: An effective medium for synthesis of metal nanoparticles. SF Journal of Nanochemistry and Nanotechnology 1: 1008. [Google Scholar]
Anderson DG, Burdick JA, Langer R (2004). Smart biomaterials. Science 305: 1923–1924. https://doi.org/10.1126/science.1099987 [Google Scholar] [PubMed] [CrossRef]
Atalah J, Espina G, Blamey L, Muñoz-Ibacache SA, Blamey JM (2022). Advantages of using extremophilic bacteria for the biosynthesis of metallic nanoparticles and its potential for rare earth element recovery. Frontiers in Microbiology 13: 855077. https://doi.org/10.3389/fmicb.2022.855077 [Google Scholar] [PubMed] [CrossRef]
Aygün A, Özdemir S, Gülcan M, Cellat K, Şen F (2020). Synthesis and characterization of Reishi mushroom-mediated green synthesis of silver nanoparticles for the biochemical applications. Journal of Pharmaceutical and Biomedical Analysis 178: 112970. https://doi.org/10.1016/j.jpba.2019.112970 [Google Scholar] [PubMed] [CrossRef]
Bai L, Wang N, Li Y (2022). Controlled growth and self-assembly of multiscale organic semiconductor. Advanced Materials 34: 2102811. https://doi.org/10.1002/adma.202102811 [Google Scholar] [PubMed] [CrossRef]
Baltazar-Encarnación E, Escárcega-González CE, Vasto-Anzaldo XG, Cantú-Cárdenas ME, Morones-Ramírez JR (2019). Silver nanoparticles synthesized through green methods using Escherichia coli top 10 (Ec-Ts) growth culture medium exhibit antimicrobial properties against nongrowing bacterial strains. Journal of Nanomaterials 2019: 1–8. https://doi.org/10.1155/2019/4637325 [Google Scholar] [CrossRef]
Bao Z, Gao P, Xia G, Wang Z, Kong M, Feng C, Cheng X, Liu Y, Chen X (2016). A thermosensitive hydroxybutyl chitosan hydrogel as a potential co-delivery matrix for drugs on keloid inhibition. Journal of Materials Chemistry B 4: 3936–3944. https://doi.org/10.1039/C6TB00378H [Google Scholar] [PubMed] [CrossRef]
Bhardwaj B, Singh P, Kumar A, Kumar S, Budhwar V (2020). Eco-friendly greener synthesis of nanoparticles. Advanced Pharmaceutical Bulletin 10: 566–576. https://doi.org/10.34172/apb.2020.067 [Google Scholar] [PubMed] [CrossRef]
Celik C, Ildiz N, Ocsoy I (2020). Building block and rapid synthesis of catecholamines-inorganic nanoflowers with their peroxidase-mimicking and antimicrobial activities. Scientific Reports 10: 1–11. https://doi.org/10.1038/s41598-020-59699-5 [Google Scholar] [PubMed] [CrossRef]
da Silva BL, Abuçafy MP, Manaia EB, Junior JAO, Chiari-Andréo BG, Pietro RCR, Chiavacci LA (2019). Relationship between structure and antimicrobial activity of zinc oxide nanoparticles: An overview. International Journal of Nanomedicine 14: 9395. [Google Scholar]
Dauthal P, Mukhopadhyay M (2016). Noble metal nanoparticles: Plant-mediated synthesis, mechanistic aspects of synthesis, and applications. Industrial & Engineering Chemistry Research 55: 9557–9577. https://doi.org/10.1021/acs.iecr.6b00861 [Google Scholar] [CrossRef]
El-Batal AI, El-Sayyad GS, Mosallam FM, Fathy RM (2020). Penicillium chrysogenum-mediated mycogenic synthesis of copper oxide nanoparticles using gamma rays for in vitro antimicrobial activity against some plant pathogens. Journal of Cluster Science 31: 79–90. https://doi.org/10.1007/s10876-019-01619-3 [Google Scholar] [CrossRef]
Elegbede J, Lateef A (2020). Nanotechnology in the built environment for sustainable development. In: IOP Conference Series: Materials Science and Engineering, USA. [Google Scholar]
Elegbede J, Lateef A, Azeez M, Asafa T, Yekeen T, Oladipo I, Aina D, Beukes L, Gueguim-Kana E (2020). Biofabrication of gold nanoparticles using xylanases through valorization of corncob by Aspergillus niger and Trichoderma longibrachiatum: Antimicrobial, antioxidant, anticoagulant and thrombolytic activities. Waste and Biomass Valorization 11: 781–791. https://doi.org/10.1007/s12649-018-0540-2 [Google Scholar] [CrossRef]
Elfeky AS, Salem SS, Elzaref AS, Owda ME, Eladawy HA, Saeed AM, Awad M, Abou-Zeid R, Fouda A (2020). Multifunctional cellulose nanocrystal/metal oxide hybrid, photo-degradation, antibacterial and larvicidal activities. Carbohydrate Polymers 230: 115711. https://doi.org/10.1016/j.carbpol.2019.115711 [Google Scholar] [PubMed] [CrossRef]
Feroze N, Arshad B, Younas M, Afridi MI, Saqib S, Ayaz A (2020). Fungal mediated synthesis of silver nanoparticles and evaluation of antibacterial activity. Microscopy Research and Technique 83: 72–80. https://doi.org/10.1002/jemt.23390 [Google Scholar] [PubMed] [CrossRef]
Findik F (2021). Nanomaterials and their applications. Periodicals of Engineering and Natural Sciences 9: 62–75. https://doi.org/10.21533/pen.v9i3.1837 [Google Scholar] [CrossRef]
Fouda A, Abdel-Maksoud G, Abdel-Rahman MA, Salem SS, Hassan SED, El-Sadany MAH (2019). Eco-friendly approach utilizing green synthesized nanoparticles for paper conservation against microbes involved in biodeterioration of archaeological manuscript. International Biodeterioration & Biodegradation 142: 160–169. https://doi.org/10.1016/j.ibiod.2019.05.012 [Google Scholar] [CrossRef]
Fouda A, Hassan SE-D, Abdo AM, El-Gamal MS (2020). Antimicrobial, antioxidant and larvicidal activities of spherical silver nanoparticles synthesized by endophytic Streptomyces spp. Biological Trace Element Research 195: 707–724. https://doi.org/10.1007/s12011-019-01883-4 [Google Scholar] [PubMed] [CrossRef]
Fouda A, Saad E, Salem SS, Shaheen TI (2018). In-vitro cytotoxicity, antibacterial, and UV protection properties of the biosynthesized Zinc oxide nanoparticles for medical textile applications. Microbial Pathogenesis 125: 252–261. https://doi.org/10.1016/j.micpath.2018.09.030 [Google Scholar] [PubMed] [CrossRef]
Gahlawat G, Choudhury AR (2019). A review on the biosynthesis of metal and metal salt nanoparticles by microbes. RSC Advances 9: 12944–12967. https://doi.org/10.1039/C8RA10483B [Google Scholar] [PubMed] [CrossRef]
Herlekar M, Barve S, Kumar R (2014). Plant-mediated green synthesis of iron nanoparticles. Journal of Nanoparticles 2014: 1–9. https://doi.org/10.1155/2014/140614 [Google Scholar] [CrossRef]
Husseiny SM, Salah TA, Anter HA (2015). Biosynthesis of size controlled silver nanoparticles by Fusarium oxysporum, their antibacterial and antitumor activities. Beni-Suef University Journal of Basic and Applied Sciences 4: 225–231. https://doi.org/10.1016/j.bjbas.2015.07.004 [Google Scholar] [CrossRef]
Jain N, Bhargava A, Majumdar S, Tarafdar J, Panwar J (2011). Extracellular biosynthesis and characterization of silver nanoparticles using Aspergillus flavus NJP08: A mechanism perspective. Nanoscale 3: 635–641. https://doi.org/10.1039/C0NR00656D [Google Scholar] [PubMed] [CrossRef]
Kato Y, Suzuki M (2020). Synthesis of metal nanoparticles by microorganisms. Crystals 10: 589. https://doi.org/10.3390/cryst10070589 [Google Scholar] [CrossRef]
Kayser O, Lemke A, Hernandez-Trejo N (2005). The impact of nanobiotechnology on the development of new drug delivery systems. Current Pharmaceutical Biotechnology 6: 3–5. https://doi.org/10.2174/1389201053167158 [Google Scholar] [PubMed] [CrossRef]
Khan AU, Khan M, Malik N, Cho MH, Khan MM (2019). Recent progress of algae and blue-green algae-assisted synthesis of gold nanoparticles for various applications. Bioprocess and Biosystems Engineering 42: 1–15. https://doi.org/10.1007/s00449-018-2012-2 [Google Scholar] [PubMed] [CrossRef]
Khan S, Sharaf M, Ahmed I, Khan TU, Shabana S, Arif M, Kazmi S, Liu C (2022). Potential utility of nano-based treatment approaches to address the risk of Helicobacter pylori. Expert Review of Anti-Infective Therapy 20: 407–424. https://doi.org/10.1080/14787210.2022.1990041 [Google Scholar] [PubMed] [CrossRef]
Khan T, Abbas S, Fariq A, Yasmin A (2018). Microbes: Nature’s cell factories of nanoparticles synthesis. Exploring the Realms of Nature for Nanosynthesis, 25–50. [Google Scholar]
Koca FD, Demirezen Yilmaz D, Ertas Onmaz N, Yilmaz E, Ocsoy I (2020). Green synthesis of allicin based hybrid nanoflowers with evaluation of their catalytic and antimicrobial activities. Biotechnology Letters 42: 1683–1690. https://doi.org/10.1007/s10529-020-02877-2 [Google Scholar] [PubMed] [CrossRef]
Lateef A, Darwesh O, Matter I (2021). Microbial nanobiotechnology: The melting pot of microbiology, microbial technology and nanotechnology. In: Microbial Nanobiotechnology, pp. 1–19. Springer Nature Switzerland. [Google Scholar]
Lateef A, Ojo SA, Elegbede JA, Akinola PO, Akanni EO (2018). Nanomedical applications of nanoparticles for blood coagulation disorders. Environmental Nanotechnology, 1: 243–277. [Google Scholar]
Lotha R, Shamprasad BR, Sundaramoorthy NS, Nagarajan S, Sivasubramanian A (2019). Biogenic phytochemicals (cassinopin and isoquercetin) capped copper nanoparticles (ISQ/CAS@ CuNPs) inhibits MRSA biofilms. Microbial Pathogenesis 132: 178–187. https://doi.org/10.1016/j.micpath.2019.05.005 [Google Scholar] [PubMed] [CrossRef]
Marooufpour N, Alizadeh M, Hatami M, Asgari Lajayer B (2019). Biological synthesis of nanoparticles by different groups of bacteria. In: Microbial Nanobionics, pp. 63–85. Springer Nature Switzerland. [Google Scholar]
Mittal AK, Chisti Y, Banerjee UC (2013). Synthesis of metallic nanoparticles using plant extracts. Biotechnology Advances 31: 346–356. https://doi.org/10.1016/j.biotechadv.2013.01.003 [Google Scholar] [PubMed] [CrossRef]
Modan EM, Plăiașu AG (2020). Advantages and disadvantages of chemical methods in the elaboration of nanomaterials. The Annals of “Dunarea de Jos” University of Galati. Fascicle IX, Metallurgy and Materials Science 43: 53–60. [Google Scholar]
Mohamed AA, Fouda A, Abdel-Rahman MA, Hassan SE-D, El-Gamal MS, Salem SS, Shaheen TI (2019). Fungal strain impacts the shape, bioactivity and multifunctional properties of green synthesized zinc oxide nanoparticles. Biocatalysis and Agricultural Biotechnology 19: 101103. https://doi.org/10.1016/j.bcab.2019.101103 [Google Scholar] [CrossRef]
Mohd Yusof H, Mohamad R, Zaidan UH, Abdul Rahman NA (2019). Microbial synthesis of zinc oxide nanoparticles and their potential application as an antimicrobial agent and a feed supplement in animal industry: A review. Journal of Animal Science and Biotechnology 10: 1–22. https://doi.org/10.1186/s40104-019-0368-z [Google Scholar] [PubMed] [CrossRef]
Negi S, Singh V (2018). Algae: A potential source for nanoparticle synthesis. Journal of Applied and Natural Science 10: 1134–1140. https://doi.org/10.31018/jans.v10i4.1878 [Google Scholar] [CrossRef]
Omar HH, Bahabri FS, El-Gendy AM (2017). Biopotential application of synthesis nanoparticles as antimicrobial agents by using Laurencia papillosa. International Journal of Pharmacology 13: 303–312. https://doi.org/10.3923/ijp.2017.303.312 [Google Scholar] [CrossRef]
Onck P, van Merkerk R, Raaijmakers A, de Hosson JTM (2005). Fracture of open-and closed-cell metal foams. Journal of Materials Science 40: 5821–5828. https://doi.org/10.1007/s10853-005-4996-7 [Google Scholar] [CrossRef]
Pal G, Rai P, Pandey A (2019). Green synthesis of nanoparticles: A greener approach for a cleaner future. In: Green Synthesis, Characterization and Applications of Nanoparticles, pp. 1–26. England: Elsevier. [Google Scholar]
Prasad R (2019). Microbial Nanobionics. Springer Nature Switzerland: Springer. [Google Scholar]
Qiu LY, Bae YH (2006). Polymer architecture and drug delivery. Pharmaceutical Research 23: 1–30. https://doi.org/10.1007/s11095-005-9046-2 [Google Scholar] [PubMed] [CrossRef]
Rabeea MA, Owaid MN, Aziz AA, Jameel MS, Dheyab MA (2020). Mycosynthesis of gold nanoparticles using the extract of Flammulina velutipes, Physalacriaceae, and their efficacy for decolorization of methylene blue. Journal of Environmental Chemical Engineering 8: 103841. https://doi.org/10.1016/j.jece.2020.103841 [Google Scholar] [CrossRef]
Rajan R, Chandran K, Harper SL, Yun SI, Kalaichelvan PT (2015). Plant extract synthesized silver nanoparticles: An ongoing source of novel biocompatible materials. Industrial Crops and Products 70: 356–373. https://doi.org/10.1016/j.indcrop.2015.03.015 [Google Scholar] [CrossRef]
Rajkumar R, Ezhumalai G, Gnanadesigan M (2021). A green approach for the synthesis of silver nanoparticles by Chlorella vulgaris and its application in photocatalytic dye degradation activity. Environmental Technology & Innovation 21: 101282. https://doi.org/10.1016/j.eti.2020.101282 [Google Scholar] [CrossRef]
Ramos MM, dos S Morais E, da S Sena I, Lima AL, de Oliveira FR, de Freitas CM, Fernandes CP, de Carvalho JCT, Ferreira IM (2020). Silver nanoparticle from whole cells of the fungi Trichoderma spp. isolated from Brazilian Amazon. Biotechnology Letters 42: 833–843. https://doi.org/10.1007/s10529-020-02819-y [Google Scholar] [PubMed] [CrossRef]
Rehman S, Ansari MA, Alzohairy MA, Alomary MN, Jermy BR, Shahzad R, Tashkandi N, Alsalem ZH (2019). Antibacterial and antifungal activity of novel synthesized neodymium-substituted cobalt ferrite nanoparticles for biomedical application. Processes 7: 714. https://doi.org/10.3390/pr7100714 [Google Scholar] [CrossRef]
Rehman S, Asiri SM, Khan FA, Jermy BR, Ravinayagam V, Alsalem Z, Jindan R, Qurashi A (2020a). Anticandidal and in vitro anti-proliferative activity of sonochemically synthesized indium tin oxide nanoparticles. Scientific Reports 10: 1–9. https://doi.org/10.1038/s41598-020-60295-w [Google Scholar] [PubMed] [CrossRef]
Rehman S, Jermy R, Asiri SM, Shah MA, Farooq R et al. (2020b). Using Fomitopsis pinicola for bioinspired synthesis of titanium dioxide and silver nanoparticles, targeting biomedical applications. RSC Advances 10: 32137–32147. https://doi.org/10.1039/D0RA02637A [Google Scholar] [PubMed] [CrossRef]
Roychoudhury A (2020). Yeast-mediated green synthesis of nanoparticles for biological applications. Indian Journal of Pharmaceutical and Biological Research 8: 26–31. [Google Scholar]
Rozan HE, Wu G, Zhou Z, Li Q, Sharaf M, Chen X (2022). The complex hydrogel based on diatom biosilica and hydroxybutyl chitosan for wound healing. Colloids and Surfaces B: Biointerfaces 216: 112523. https://doi.org/10.1016/j.colsurfb.2022.112523 [Google Scholar] [PubMed] [CrossRef]
Saba I, Wani K, Syed A, Rehman S (2021). Role of microbial nanotechnology in bioremediation of heavy metals. In: Microbial Nanotechnology: Green Synthesis and Applications, pp. 307–319. Springer Nature Switzerland: Springer. [Google Scholar]
Salata OV (2004). Applications of nanoparticles in biology and medicine. Journal of Nanobiotechnology 2: 1–6. https://doi.org/10.1186/1477-3155-2-1 [Google Scholar] [CrossRef]
Salem SS, Fouda MM, Fouda A, Awad MA, Al-Olayan EM, Allam AA, Shaheen TI (2021). Antibacterial, cytotoxicity and larvicidal activity of green synthesized selenium nanoparticles using Penicillium corylophilum. Journal of Cluster Science 32: 351–361. https://doi.org/10.1007/s10876-020-01794-8 [Google Scholar] [CrossRef]
Saxena J, Sharma PK, Sharma MM, Singh A (2016). Process optimization for green synthesis of silver nanoparticles by Sclerotinia sclerotiorum MTCC, 8785 and evaluation of its antibacterial properties. SpringerPlus 5: 1–10. https://doi.org/10.1186/s40064-016-2558-x [Google Scholar] [PubMed] [CrossRef]
Sharaf M, Sewid AH, Hamouda HI, Elharrif MG, El-Demerdash AS et al. (2022). Rhamnolipid-coated iron oxide nanoparticles as a novel multitarget candidate against major foodborne E. coli serotypes and methicillin-resistant S. aureus. Microbiology Spectrum 10: e00250-00222. https://doi.org/10.1128/spectrum.00250-22 [Google Scholar] [PubMed] [CrossRef]
Shobha B, Lakshmeesha TR, Ansari MA, Almatroudi A, Alzohairy MA, Basavaraju S, Alurappa R, Niranjana SR, Chowdappa S (2020). Mycosynthesis of ZnO nanoparticles using Trichoderma spp. isolated from rhizosphere soils and its synergistic antibacterial effect against Xanthomonas oryzae pv. oryzae. Journal of Fungi 6: 181. https://doi.org/10.3390/jof6030181 [Google Scholar] [PubMed] [CrossRef]
Singh A, Gautam PK, Verma A, Singh V, Shivapriya PM, Shivalkar S, Sahoo AK, Samanta SK (2020). Green synthesis of metallic nanoparticles as effective alternatives to treat antibiotics resistant bacterial infections: A review. Biotechnology Reports 25: e00427. https://doi.org/10.1016/j.btre.2020.e00427 [Google Scholar] [PubMed] [CrossRef]
Singh I, Singh S (2019). Study of algal mediated biosynthesis of nanoparticle: Future of green nanotechnology. Current Life Sciences 5: 7–14. [Google Scholar]
Soni M, Mehta P, Soni A, Goswami GK (2018). Green nanoparticles: Synthesis and applications. IOSR Journal of Biotechnology and Biochemistry 4: 78–83. [Google Scholar]
Subramaniyan SA, Sheet S, Vinothkannan M, Yoo DJ, Lee YS, Belal SA, Shim KS (2018). One-pot facile synthesis of Pt nanoparticles using cultural filtrate of microgravity simulated grown P. chrysogenum and their activity on bacteria and cancer cells. Journal of Nanoscience and Nanotechnology 18: 3110–3125. https://doi.org/10.1166/jnn.2018.14661 [Google Scholar] [PubMed] [CrossRef]
Ter-Zakaryan A, Zhukov A (2021). Materials Horizons: From Nature to Nanomaterials, pp. 349–377. Springer Nature Switzerland. [Google Scholar]
Velmurugan P, Cho M, Lee SM, Park JH, Lee KJ, Myung H, Oh BT (2016). Phyto-crystallization of silver and gold by Erigeron annuus (L.) Pers flower extract and catalytic potential of synthesized and commercial nano silver immobilized on sodium alginate hydrogel. Journal of Saudi Chemical Society 20: 313–320. https://doi.org/10.1016/j.jscs.2014.09.004 [Google Scholar] [CrossRef]
Yilmaz E, Yalinca Z, Yahya K, Sirotina U (2016). pH responsive graft copolymers of chitosan. International Journal of Biological Macromolecules 90: 68–74. https://doi.org/10.1016/j.ijbiomac.2015.10.003 [Google Scholar] [PubMed] [CrossRef]
Zielonka A, Klimek-Ochab M (2017). Fungal synthesis of size-defined nanoparticles. Advances in Natural Sciences: Nanoscience and Nanotechnology 8: 043001. https://doi.org/10.1088/2043-6254/aa84d4 [Google Scholar] [CrossRef]
Cite This Article
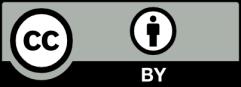