Open Access
REVIEW
A review on re-emerging bacteriophage therapy in the era of XDR
1 Department of Microbiology, Shaheed Rajguru College of Applied Sciences for Women, University of Delhi, Vasundhara Enclave, New Delhi, 110096, India
2 Lab-209, Cell & Developmental biology lab, Center of Research for Development, Parul University, Vadodara, 391760, Gujarat, India
3 Instituto de Conservacióny Mejora de la Agrodiversidad Valenciana, Universitat Politècnica de València, Valencia, 46022, Spain
* Corresponding Author: Prashant Kaushik,
BIOCELL 2023, 47(9), 1915-1930. https://doi.org/10.32604/biocell.2023.029564
Received 26 February 2023; Accepted 01 June 2023; Issue published 28 September 2023
Abstract
In the present medicine world antibiotic resistance is one of the key threats to universal health coverage. Researchers continue to work hard to combat this global health concern. Phage therapy, an age-old practice during the early twentieth century, was outshined by the discovery of antibiotics. With the advent of widespread antibiotic resistance, phage therapy has again redeemed itself as a potential alternative owing to its adeptness to target bacteria precisely. Limited side effects, the ability to migrate to different body organs, a distinct mode of action, and proliferation at the infection site, make phages a profitable candidate to replace conventional antibiotics. The progressive outcome of numerous in vitro studies and case reports has validated the clinical efficacy of phage therapy. The bright perspective of using phages to treat bacterial infections has fueled enormous medical research to exploit their potential as therapeutics. The gaps in the information about phages and the lack of consent for clinical trials is major hurdle for consideration of phage therapy. Crafting phage therapy as a reality in medicine requires a coordinated effort from different fraternities. With this review, we aim to emphasize the importance of phage therapy in modern medicine. This review explains their historical journey, basic phage biology, cross-talk with the host immunity, obstacles with phage therapy, and their possible remedies. Comprehensive data on the various significant clinical trials of phage therapy has been presented. We evaluated the efficacy of antibiotics and phage therapy in part and in combination, along with recent progress and future perspectives of phage therapy.Graphical Abstract
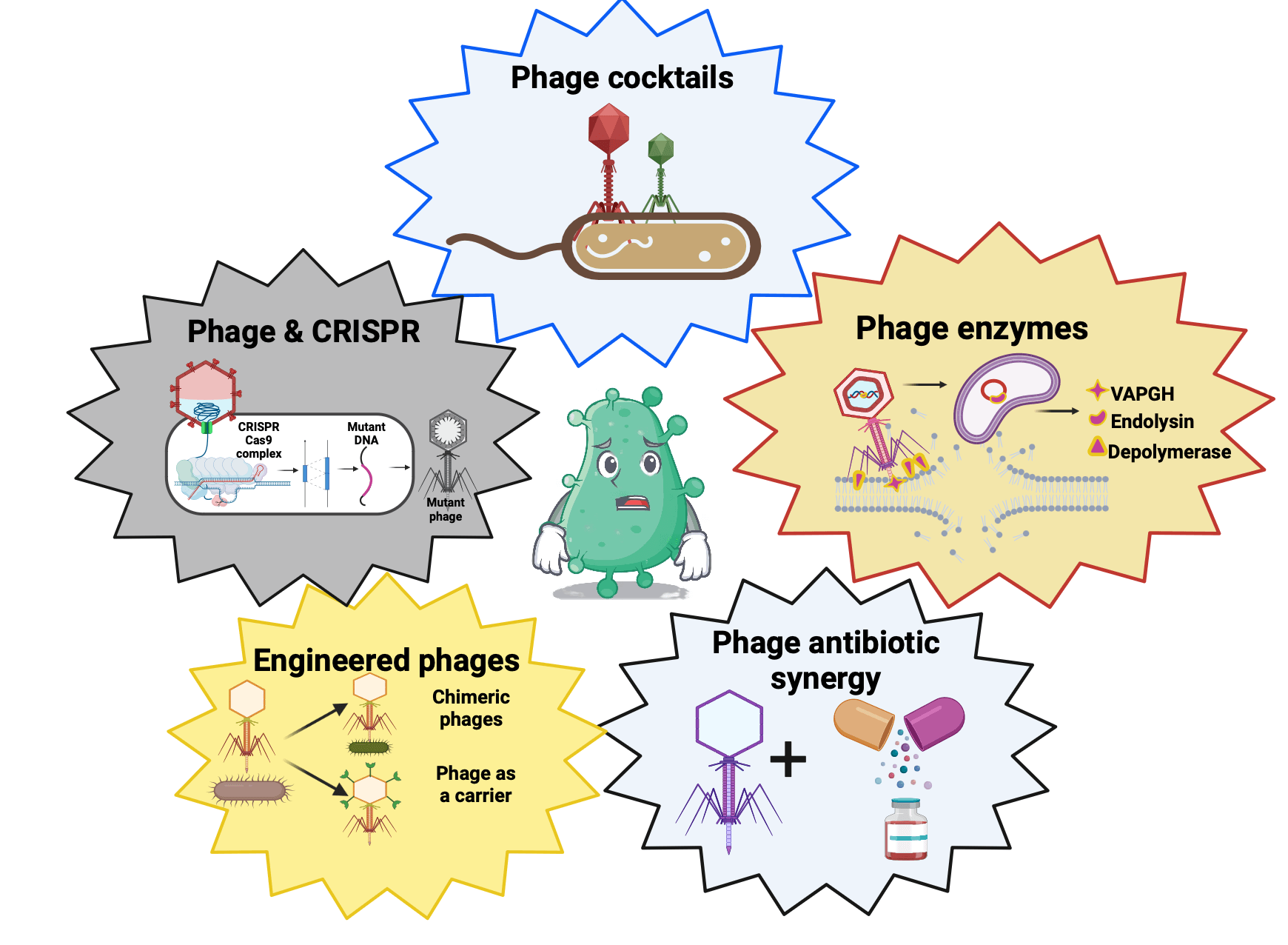
Keywords
Antibiotic-resistant bacterial strains have become a major problem in contemporary medicine. Since antibiotics have established themselves as effective antibacterial medicines, they have adopted multiple forms. Consequently, the constant antibiotic pressure has led to the emergence of antibiotic-resistant bacteria, some of which are MDR (Multi Drug Resistant)-bacteria unable to be treated with multiple antibacterial drugs. Such circumstances have brought forth the post-antibiotic era, which began in the late 2000s (Loganathan et al., 2021). A group of the most common multidrug-resistant bacteria has been abbreviated ESKAPE, an acronym for Enterococcus faecium, Staphylococcus aureus, Klebsiella pneumoniae, Acinetobacter baumannii, Pseudomonas aeruginosa, and Enterobacter species (Rice, 2008). The multidrug-resistant (MDR) strains of Mycobacterium tuberculosis, the causative agent of MDR-TB (Multidrug-resistant tuberculosis), a major public health issue around the world, is of grave concern (Palomino and Martin, 2014). These Mycobacterium strains are mostly resistant to rifampicin and isoniazid, two important drugs used to treat the disease. Since 2006, the presence of even more resistant strains of M. tuberculosis known as extensively drug-resistant (XDR)-TB has been recognized (Gandhi et al., 2006). Even more recently, a troubling situation has emerged with the characterization of M. tuberculosis strains found to be resistant to all antibiotics tested, a situation known as totally drug-resistant (TDR)-TB (Velayati et al., 2009; Udwadia et al., 2012).
One of the effective therapeutic options for use against highly resistant and incurable bacterial infections is bacteriophage-therapy. The perspective of bacteriophage usage for therapeutics has regained interest in view of widespread antibiotic resistance and enhanced understanding of the significance of the human microbiome. The antibacterial nature of the phage promises chances of a successful post-antibiotic era (Cisek et al., 2017).
Bacteriophages were discovered over a century ago by two scientists, William Twort and Felix d’Herelle, independently. A bacteriophage is a common virus that feeds on bacteria (host) and may kill it in due course of time, serving as a means for releasing progeny phage. This discovery sparked controversy and excitement due to their unknown biology and potential for treating bacterial infections, respectively. Bacteriophages have since been known to be ‘bacterial viruses’ that infect specific bacterial strains and inhibit their growth, thus acting as a curative agent for bacterial diseases (Moelling et al., 2018). A decade before the discovery of penicillin in 1928, bacteriophages were used to treat various bacterial infections. Physicians in countries like Georgia, Poland, and Russia continue to use phages as therapeutic agents and generate valuable practical experience from them (Kutateladze and Adamia, 2010). Myelnikov has elaborately explained in his publishing the adoption and survival of bacteriophage therapy (Myelnikov, 2018). In the United States, the incident of Tom Patterson, who was infected in Egypt by a resistant strain of A. baumannii, was brought back to the United States in critical condition and then cured by phages at the University of California, San Diego. This incident has spawned a number of articles and even a book in its praise. Several clinical trials are currently underway or will begin in the coming months. The number of scientific studies on the subject has increased significantly (Brives and Pourraz, 2020). Promising human trials against Mycobacteria, E. faecalis infections and urinary tract infections (UTIs), etc., are being carried out presently, providing the medicine world with hope for a better future in the regimen of antibacterial agents.
In this review, we highlight the significance of phage therapy in modern medicine, providing their history, mode of action as therapeutic agents, along with interaction with the host immune system. We have also delved into the obstacles with phage therapy and its solution, as provided by researchers. The review gives comprehensive data on the various significant clinical trials that have been done using the approach and the trials under progress. We evaluated the efficacy of antibiotics and phage therapy in part and in conjunction. We also tried to highlight the recent progression made in phage therapy, and its future perspectives. The review also highlights the current scenario of phage therapy in India, battling with a multitude of cases of antibacterial resistance.
To begin with, bacteriologist Ernest Hanbury Hankin, in the year 1896, published in French in The Annals of the Pasteur Institute about a ‘biological principle’ capable of destroying the cultures of Vibrio cholerae. The ‘principle’ could pass through the Millipore filters that retained bacteria. He discovered them while he was appointed as the Chemical Examiner and Bacteriologist in the Central Provinces of India and found that they were present in the waters of the Ganga and Yamuna rivers in India (Fig. 1) (Wittebole et al., 2014). In the year 1915, Frederick Twort, a British microbiologist, while trying to grow Vaccinia virus on agar media, observed a “glassy and transparent” transformation on agar plates where bacteria would not grow even when sub-cultured. This transformant could pass through a fine porcelain filter that would trap bacteria and could also break down the bacterial culture into granules. Before Twort could conduct further experiments, however, the First World War interrupted his career. In 1917, independent of Twort’s research, Felix d’Herelle, who was a French-Canadian microbiologist, published a short paper about the research he carried out on the enteric bacteria of dysentery patients at the Pasteur Institute, describing the lysis of bacteria over multiple propagations. He coined the term bacteriophage (a bacteria-eater), justifying that there was some “invisible microbe that is antagonistic to the dysentery bacillus.” Following this discovery, phages were used to cure cases of dysentery and clinical trials were expanded to experiment efficacy of phages on other bacterial infections like bubonic plague, cholera, and other skin infections. A major break-through occurred in 1939, when Ellis and Delbruck proposed the one-step growth curve for phage replication (Fig. 1) (Cann, 2016). However, this golden period did not last long, as a major antibacterial compound penicillin was accidentally discovered by Alexander Fleming in 1928 and by the mid-to-late 1940s, it was made widely available for the general public. During the period of 1930s–40s, phage therapy saw a rapid decline (Fig. 1). Sooner, penicillin-resistant Staphylococci was also identified, but the discovery of many other antibiotic compounds continued. The development of sulfonamides (which were potent antibacterial agents) in Germany in the 1930s and the large-scale production and use of antibiotics in the United States from the 1940s onward coincided with the nearly complete departure of phage therapy in the following years (Myelnikov, 2018) More than 40 antibiotics were developed and made available for clinical use during the “golden period” of antibiotics, which lasted from the 1940s to the 1970s (Fig. 1).
Figure 1: A timeline of notable developments in the history of phage research and phage therapy. The important milestones in the field of phage research and phage therapy are illustrated in the boxes. The high and low in the timeline represent the increase and decrease of research interest in phage therapy.
During this period (1940–1970s), antibiotic resistance was viewed with minimal concern due to the rapid development of newer compounds, which fueled the cycle of antibiotic discovery. These discoveries somehow side-lined phage therapy and its trials. The second half of the 20th century was thus marked by a dramatic reduction in infectious diseases. It made antibiotics one of the greatest accomplishments in the history of medicine (Brives and Pourraz, 2020). However, beginning in the 1990s, a steady decline in the use of novel antibiotics made the effects of this cycle more obvious. The majority of newly released antibiotics seemed to be either modified or combined versions of previously recognized compounds, a phenomenon known as a “dry pipeline” in antibiotic research and development. The medicine world soon took another turn when bacteria started to become resistant to antibacterial compounds, and it became difficult to treat many mild to severe infections with common antibiotics. In 1956, the first MDR (multi-drug resistant) Mycobacterium was identified, and in 1987, the last antibiotic, Daptomycin of the class of lipopeptides, was discovered for the cure of serious gram-positive bacterial infections. Pathogens that were multidrug-resistant (MDR), extensively drug-resistant (XDR), and even pan-drug-resistant (PDR)-resistant to all current antibacterial agents were developed as a result of the continuous accumulation of antibiotic resistance traits by the host. The post-antibiotic era began with the emergence of ESKAPE pathogens. Persistent antibiotic usage has resulted in the emergence of MDR and XDR bacteria, rendering even the most effective drugs ineffective. This situation, described by the World Health Organization (WHO) as being a critical priority for research and development, calls for immediate development, standardization, and application of novel treatment options against infectious diseases, and phage therapy is once again gaining recognition. With the start of a new century, phage therapy got another chance to rekindle interest among scientists. Several attempts to develop phage-based biotechnologies in human health, animal health, or biocontrol in the agri-food sector occurred in the late 1990s and early 2000s (Fig. 1) (Kuchment, 2011). Phage therapy has been used occasionally in France and Belgium to treat patients in cases of therapeutic failure (Patey et al., 2018). Over the past years, phage therapy has been under development again in laboratories and hospitals. Many successful trials have already been conducted and are still going on, which promise a high potential of phage therapy.
As a measure to combat multidrug-resistant infections, phage therapy is believed to be a potential adjunct to the existing conventional antibiotic treatments. The life cycle of a bacteriophage explains its antibacterial mode of action. It can infect its host chiefly in two ways-lytic and lysogenic (Fig. 2).
Figure 2: Bacteriophage life phases-Following the initial attachment of a bacteriophage to the host-cell receptor, the genetic material of the phage is introduced into the host cell, where it multiplies to produce many copies of the virus before lysing the cell wall and releasing progeny viruses to continue the cycle. This is known as the cycle of lytic replication. Alternatively, the lysogenic cycle can also be established by bacteriophages. In this case, the phage genome integrates into the host’s genome and multiplies along with it rather than giving rise to virus particles. While some lysogenic bacteriophages can be induced to enter the lytic cycle, others continue to be lysogenic.
The distinction between lytic and lysogenic bacteriophages is based on how the replication cycle of a phage proceeds (Cisek et al., 2017). Phages bind to specific receptors on the bacterial cell surface and infuse their genetic material into the host cell. Following this, they can proceed with two mechanisms, the first one being lysogeny which involves the integration of the phage genome into the bacterial genome, and then, it re-produces vertically from mother to daughter cell. Besides lysogeny, a phage can undergo a lytic cycle, wherein it invades the host replication machinery to produce progeny phages and eventually lyse the cell. For therapeutic purposes, lytic phages are inevitably chosen. Some of these lytic bacteriophages use antimicrobial peptides-Amurins to suppress the synthesis of peptidoglycan, an important component of the bacterial cell wall (Woźnica et al., 2015). However, the majority of them use two sets of proteins to lyse the bacterial cell. These are Holins and Endolysins, which work synergistically to cause lysis, collectively called the Holin-lysin system (Fig. 3) (Loessner, 2005; Drulis-Kawa et al., 2015).
Figure 3: Overview of Holin-Endolysin mechanism of lysis: The figure depicts the mechanism of lysis of a bacterial cell. Two major proteins are involved in the process-Holin and Endolysin. Holins perforate the cytoplasmic membrane of the host and allow the endolysins to ultimately break down the peptidoglycan and lyse the cell.
Amurin peptides are lytic antimicrobial peptides that are also known as DLAs (Direct Lytic Agents). Recently, both engineered and native DLAs with potent bactericidal activity against a variety of Gram-negative pathogens, including MDR and XDR P. aeruginosa, K. pneumoniae, and Acinetobacter baumannii, have been identified. In addition to their effectiveness, Amurins have been shown to clear biofilms and work synergistically with a variety of standard-of-care antibiotics in vitro (Schuch et al., 2022). Holins are involved in the process of triggering host cell lysis. Their function is to perforate the cytoplasmic membrane of the host (Fig. 3). This provides endolysins access to bacterial peptidoglycan. Hence, holins determine the time of bacterial lysis. They act at a specific time point, control bacterial murein accessibility for phage endolysin, and synchronize the activity of the holin-lysin system (Dewey et al., 2010; Shi et al., 2012). Enzymatic proteins called phage endolysins have the function of breaking down cell walls (Wang et al., 2000). They are utilized by bacteriophages to hydrolyze the peptidoglycan of infected bacteria (Fig. 3) (Schmelcher et al., 2012).
Endolysins carry out endopeptidase, amidase, glycosidase, or lytic trans glycosylase activities to degrade murein and destroy bacterial cells (Donovan and Foster-Frey, 2008; Nelson et al., 2012). They induce the release of progeny virions by acting at the end of the phage replication cycle (Fig. 3). Endolysins that target gram-positive bacteria have different structures than those targeting gram-negative bacteria, reflecting differences in enzyme targets’ cell wall architecture. Gram-positive bacteria have hydrolytic enzymes with two functional domains- the cell wall binding domains (CBDs) and the enzymatically active domains (EADs). While EAD is the main catalytic site actually fueling the breaking of bonds in the peptidoglycan, CBD is in place to avoid any collateral damage. Cell wall debris is tightly bound to it after the cell lysis to keep it from diffusing and destroying the cells that are not yet infected by phages (Borysowski et al., 2006). Gram-negative bacteria, on the other hand, have an outer membrane in place that limits access to the peptidoglycan and prevents such collateral damage. Therefore, enzymes directed against them appear to be small globular proteins with only one domain, known as the enzymatically active domain (EAD). The holin-lysin system terminates the cycle of phage infection at a specific time point. The ‘dual-start model’ explains how the effect of endolysins on the bacterial cell wall is coordinated. According to it, the proportion of holin and its antagonist-antiholin, determines the time of bacterial lysis. The holin-antiholin ratio is maintained through the regulation of their translation. Loss of plasma membrane integrity occurs when there is an increase in the holin-antiholin ratio, which enables endolysins to enter the periplasm and eventually break down the host peptidoglycan, eventually lysing the bacterial cell (To and Young, 2014).
Bacteriophage receptors on the host cell surface
Phage-cell interactions are seen as a process with numerous sequential stages: phage adsorption on the host cell surface and entry of phage genome into the cell, intracellular synthesis of viral components and assembly of virions, bacterial cell lysis, and phage re-lease (Rakhuba et al., 2010). A particular bacteriophage strain is believed to be capable of infecting a narrow host range or microbial species. Such specificity in the interaction of the phage with the host cell is determined by the specificity of adsorption, which is in turn determined by the nature and the structural characteristics of receptors on the bacterial cell surface of both gram-positive and gram-negative bacteria (Fig. 4). The outer membrane of gram-negative bacteria is structurally distinct from the inner membrane and plasma membrane of gram-positive bacteria. There are two distinguishing characteristics- its high permeability, which is caused by increased levels of integral proteins forming transport channels (up to 20,000 per cell) (Nikaido, 2003), and the presence of a unique glycolipid lipopolysaccharide (LPS) in the external lipid layer, which is typically found only in gram-negative bacteria. Bacteriophage receptors could be proteins found in the membrane and at various LPS sites. In many cases, phages require both types of molecules for adsorption (Lindberg, 1973).
Figure 4: Schematic depiction of bacteriophage receptors on the surface of Gram-positive (left) and Gram-negative (right) bacteria. In the figure, Gram-positive and Gram-negative bacteria cell surface-associated virulent components that serve as phage receptors have been depicted. On the left, the receptors for Gram-positive bacteria are shown, which include surface-exposed LTA (lipoteichoic acid), PIP (phage infection proteins), and CWPS (cell wall polysaccharide). LPS (lipopolysaccharide) and CPS (capsular polysaccharide) are two surface-associated glycopolymers that Gram-negative bacteria (shown on the right) possess as some of their primary receptors for phages. A number of outer membrane proteins, including the porins-OmpC, OmpF, and TolC, have also been discovered to serve as the secondary receptor for the phages of Escherichia, Salmonella, and Vibrio.
Outer membrane proteins are classified into five types: 1) structural proteins that interact with the peptidoglycan layer, 2) specific and non-specific porins that form membrane channels, 3) enzymes, 4) high-affinity substrate receptors, and 5) transport proteins that are involved in secretion. Bacteriophage binds with protein-LPS complex, and precipitation of this complex with Mg+2 leads to irreversible phage attachment (Datta et al., 1977).
The host specificity of phages varies greatly; some phages have demonstrated the potential to infect a wide variety of bacterial strains and even genera, while others are highly strain-specific (Koskella and Meaden, 2013). For example, some of the phages specific to Clostridium include-Susfortuna, CPS1, CPS2, phiCP39O, and phiCP26F, among others (Venhorst et al., 2022). According to Paolozzi and Ghelardini (2006), bacteriophage Mu can infect species of Escherichia coli, Citrobacter freundii, Shigella sonnei, Enterobacter, and Erwinia; the staphylococcal phage 812 infects 95% of the 782 strains of S. aureus and 43% of the other Staphylococcus species tested. Meanwhile, bacteriophage P-27/HP infects 60% of the 28 S. aureus isolates examined by Gupta and Prasad (2010). They are all characterized as polyvalent or having a wide host range. The majority of phages are exclusively infectious to bacteria that carry their complementary receptor, restricting the host range of a lytic phage. Typical lytic phages associated with human pathogens and the gut microbiome belong to the newly assigned class caudoviricetes (earlier in order caudovirales), which include all “tailed phages” of bacteria and archaea having double-stranded DNA genomes and Microviridae, which have single-stranded DNA genomes and are tailless (Turner et al., 2023). Traditionally, phage therapy has relied on lytic phages, which obligately kill their bacterial host. For treatment, lytic phages are combined into “phage cocktails,” which consist of various phages that have been shown to be effective against the target pathogen in vitro. To date, no serious adverse effects from phage therapy have been identified, justifying their use against bacterial infections. However, it is important to mention here that phages used in therapy should not contain any genes encoding virulence factors or protein toxins. The presence of such factors/genes can benefit the bacterial host by encoding virulence factors (such as diphtheria toxin, shiga toxin, and botulinum toxin), metabolic genes, and antibiotic resistance genes (such as β-lactamases). Toxin and virulence factor production is undesirable (Lin et al., 2017). Therefore, it is important to identify the most suitable phage for the treatment of an infection. Preclinical studies, both in vitro and in vivo, should prove that the phage meets all requirements for it to be developed into a pharmaceutical product (Manohar et al., 2019). There is considerable evidence that bacteriophages are part of the healthy human microbiota/virobiota, and numerous studies are being conducted to evaluate their role in the microbiome, which may imply that the human immune system does not perceive bacteriophages as a threat (Navarro and Muniesa, 2017; Minot et al., 2011).
Phage against Clinically Significant Pathogens
The first known therapeutic use of phages occurred in 1919 when d’Herelle and several hospital interns ingested a phage cocktail (later designated as Intesti phage) to check its safety. Later, they gave it to a 12-year-old boy with severe dysentery. With just a single dosage, the boy’s symptoms subsided, and he recovered completely within a few days. However, D’Herelle did not publish his conclusions until 1931. Time and again, phage therapy has been explored in studies against a variety of clinically significant pathogens utilizing various animal models. Human trials for bacteriophage therapy have also been conducted, mostly at institutes in Eastern Europe. The most well-known of these institutes are the Eliava Institute of Bacteriophage in Georgia and the Institute of Immunology and Experimental Therapy in Wroclaw, Poland. The Eliava Institute has significantly used phages in the preclinical and clinical treatment of common bacterial pathogens such as E. coli, S. aureus, P. aeruginosa, S. dysenteriae, Streptococcus spp., Salmonella spp., Enterococcus spp., and Proteus spp. (Kutateladze and Adamia, 2008). Some of the important promising clinical trials on humans and animal models against bacterial pathogens with their outcomes are listed in Table 1 in their chronological order, along with some ongoing trials. Clinical trials using various phage-bacteria combinations offer heterogeneous results. Results vary from some patients experiencing complete bacterial clearance while others remain without any impact (Vandenheuvel et al., 2015). The probable reasons behind some of the failures during phage treatment are discussed in different reports of randomized and double-blind clinical trials specifically carried out for this purpose. The enlisted reasons include-Ambiguity of intestinal bacterial infections caused by other co-infecting bacteria, interference by antibiotic treatments or too low titers of phages or target bacteria (Rose et al., 2014; Jault et al., 2019).
Immune System Response to Phage-therapy
Research and analyses on the interactions between bacteriophages and the immune system are crucial for the appropriate use of phage therapy as it carries a risk of immunological reactions. The location of the bacterial infection and the therapeutic phage administration site influences the immune response towards the phage (Reyes et al., 2012). Antibodies are known to be induced during oral administration of phages during therapy for bacterial infections caused by Escherichia, Klebsiella, Proteus, Pseudomonas, and Staphylococcus (Dabrowska et al., 2005). Anti-phage antibodies have been reported in the serum of many species (e.g., humans). These are indicative of the natural interaction of animals/humans with different types of phages (Smith et al., 1987). Cellular immunity, in addition to the humoral immune response, is crucial in the defense against phages. Since phages consist mostly of proteins and nucleic acids, they are inherently non-toxic. Additionally, the topical applications of phages during clinical studies have not shown any side effects as far as studies have been carried out (Wright et al., 2009), and so far, any immunological complications have not been reported after the administration of phages (Sarker et al., 2012). Overall findings point to the importance of testing each phage’s immune response, especially if intravenous therapy is being considered. A recent comparative study on the immunogenicity of two therapeutic bacteriophages-A3R and 676Z, active against S. aureus, has been carried out in murine models. When compared for their overall ability to induce specific antibodies, these two phages were able to produce IgM and IgG. Further, there were no enhanced specific immune responses to the proteins studied four phage proteins in the virions, with the emphasis on the tail sheath (TmpH) or external capsid head (Mcp) (Kaźmierczak et al., 2022). These phages are routinely applied in patients at the Phage Therapy Unit, Poland for the treatment of S. aureus infections. In contrast to oral administration, a different trend is observed in the bloodstream and other internal organs, which are not native habitats for phages. Both adaptive and innate immunity are significantly stimulated when phages are administered intravenously. Phagocytes quickly eliminate certain phages from the blood and internal organs if there are no host bacteria for them. They are internalized and eradicated by reticuloendothelial system cells of the liver and spleen. It is interesting to note that specialized macrophages found in the liver, Kupffer cells can phagocyte phages four times greater than macrophages in the spleen. This increases the possibility that phages that are arrested in the spleen could trigger lymphocytes to produce antibodies, which can combat infections more effectively. Pathogenic bacteria are often eliminated by innate immunity, which is regarded as the first line of defense before the adaptive immune response is activated. According to studies, patients who received phage therapy showed a decline in the number of mature neutrophils in their peripheral blood, suggesting that an active innate immune response has been activated by the phages (Weber-Dąbrowska et al., 2002). Phages are also capable of inducing certain antibodies (neutralizing antibodies) against them during phage therapy, which typically reduces its ability to kill the target bacteria in vivo. The factors which might affect the concentration of neutralizing antibodies include (a) The path of phage administration—topical and oral administration induce a slight increase in antibodies, and (b) and the dose regimen. Studies have indicated that anti-phage neutralizing antibodies are likely one of the most important factors responsible for the functional limitation of phage therapy (Smith et al., 1987). It has been hypothesized that the production of neutralizing antibodies should not pose a major problem during the preliminary cure of acute infections. This is because the phage action dynamics are quicker than the production of neutralizing antibodies by the host (Sulakvelidze and Alavidze, 2001). However, if anti-phage antibodies are still present when the second round of treatment is given, they could be a concern. One such immune response of a phage cocktail was observed on an 81-year-old immunocompromised patient with bronchiectasis and persistent Mycobacterium abscessus lung illness. The patient was given a six-month treatment with a three-phage combination active against the strain. Intravenous injection of phage to minimize infectious burden was safe and lowered sputum load tenfold within one month in this case study. However, M. abscessus counts re-emerged after two months as the patient generated a powerful IgM- and IgG-mediated neutralizing antibody response to the phages, which was later linked with poor therapeutic efficacy (Dedrick et al., 2021).
This issue can be resolved in three different ways. It may be considered to (a) administer the phage again, (b) increase the phage concentration, or (c) administer other phages because resistance varies from one phage to another. Along with the development of anti-phage neutralizing antibodies, the level of non-neutralizing antibodies IgM and later IgG also increases, further strengthening the response of the immune system following subsequent jabs of certain types of phages (Biswas et al., 2002; Capparelli et al., 2010).
Until now, phage therapy is not a chosen alternative for therapeutic treatments against bacterial infections, and its efficacy is still debatable (Sulakvelidze and Alavidze, 2001). Some of these challenges were recognized as early as the 1930s. It was recognized that the prior determination of phage specificity against bacterial strains is indispensable; there was a doubt if the efficacy of phage treatment was sustained over time or not as some of the pharmacokinetic experiments revealed that the body rapidly removed the phages via spleen. Studies by Luria and Delbruck in 1943 exhibited that bacteria could evolve to develop resistance against phages (Kortright et al., 2019); moreover, some earlier studies demonstrated that laboratory experiments of phages with bacteria did not coincide with in vivo results. Hence, the experiment suggested that the in vitro efficacy of phage therapy was good, but not in vivo. Most of these doubts were resolved by Smith and Huggins pioneering experiments with Phage R in 1982, moreover, they also proved phage therapy to be more effective than antibiotic therapy. Using mice as their experimental model, they showed that a single dose of phage R was equivalent to eight doses of streptomycin against K1+ E. coli (Smith and Huggins, 1982). However, there are still some unresolved challenges to be addressed, such as:
Selection of the ‘appropriate’ phage
The phages that can be used as therapeutic tools have to be carefully selected such that they are efficient in killing the target bacteria without altering much the environments where they are used (Merabishvili et al., 2009). The following phage characteristics are generally ensured- the phages should obligately follow the lytic cycle of infection, there should not be storage problems associated with them, i.e., they should be easy to store, there should be carefully studied data about its efficacy and safety beforehand, and in the most ideal situations, the genome should be completely sequenced to ensure that there are no undesirable genes present that code for any toxins (Gill and Hyman, 2010). Moreover, we have to be careful enough not to select transducing phages for therapy (Abedon and Thomas-Abedon, 2010). Transduction is the phenomenon of the transfer of genetic material from one bacterium to another via a bacteriophage. If we use a lytic bacteriophage for therapy that has a transducing potential, there would be chances of it delivering antimicrobial genes from one bacterium to another and various other clinical complexities. One should therefore be cognizant of the transducing potential of a phage before using it for therapy (Doub, 2021). Some of the additional characteristics that may as well be accounted for are the morphology of the virion and its protein profiles (Skurnik and Strauch, 2006).
Bacteriophages show high specificity against the bacterial strains that it infects and kills (Sybesma et al., 2018). This specificity, although advantageous with respect to its mode of action, narrows the range of hosts that it can act against and limits the treatment only against those bacterial strains whose susceptibility to specific phages is known priorly.
Phage therapy is an ‘unfamiliar’ concept
One of the greatest challenges to phage therapy is it being an unfamiliar and new concept in the Western Medical Establishment. Lesser clinical trials are responsible for the Western countries to be reluctant in using phage therapy (Sybesma et al., 2018). The preclinical research data has shown that phage therapy can be used effectively against a wide range of bacterial infections, but clinical trials are yet to confirm and establish this compared to the fewer experimental proofs that we have. Therefore, the insufficiency of knowledge about using the phages in therapy stands as a barrier to be used by scientists in treatment protocols currently. More trials and studies in the future will help know about phage therapy better with all the pros and cons established.
Bacteria evolving to be phage-resistant
One of the major challenges to phage therapy is the possible evolution of bacteria to become resistant to phages forming bacteriophage-insensitive mutants (BIMs) (Pires et al., 2020). It has been observed in the course of studies over the last few years that phage-resistant mutants emerge almost frequently, and it is unavoidable (Oechslin, 2018). Bacteria have evolved a wide range of strategies to prevent lytic phage infection, and phages have developed an equally diverse range of ways to overcome this resistance. For bacteria, it includes modification or loss of receptors, in addition to the incorporation of phage DNA into the clustered regularly interspaced palindromic repeats/CRISPR associated system (CRISPR/Cas) system (Labrie et al., 2010; Bernheim and Sorek, 2020). On the other hand, phages have developed to become capable of identifying new or altered receptors as well as possessing anti-CRISPR genes (Koskella and Brockhurst, 2014). BIMs have been observed in some in vitro studies (Fu et al., 2010; Le et al., 2014). In a study by McGee et al. (2023), Escherichia coli cultures were infected with phage strains that were not previously encountered in order to determine bacterial resistance to phage across several strains. It was discovered that phage-resistant E. coli mutants that developed resistance to a particular phage strain were also resistant to phages with similar adsorption strategies. Mutations that confer phage-resistant phenotypes are usually those involved in LPS structure/synthesis (McGee et al., 2023). However, some studies have shown that the in vitro observations of resistance do not always coincide with in vivo observations (Oechslin et al., 2017).
Key to Challenges of Phage Therapy
• Bacterial resistance is a critical challenge in the effective development of phage therapies. Research conducted by Yehl et al. (2019) identified host-range-determining regions (HRDRs) in the T3 phage tail fiber protein through natural evolution and structural modeling and devised a high-throughput approach for genetically engineering these regions using site-directed mutagenesis. This method, inspired by antibody specificity engineering, creates deep functional diversity while minimizing disturbances to the overall tail fibre structure, resulting in synthetic “phage-bodies.” It was demonstrated that altering HRDRs results in phage-bodies with different host ranges, and that certain phage-bodies may limit bacterial growth in vitro for a long period of time by inhibiting bacterial resistance. This strategy seems as a potential approach for the development of next-generation antimicrobials that reduce resistance development and can be applied to various viral scaffolds for a wide range of applications Yehl et al. (2019).
Dedrick et al. published a study that described the case of an adolescent with cystic fibrosis and a widespread Mycobacterium abscessus infection which was treated with a three-phage cocktail post bilateral lung transplantation. Genome engineering and forward genetics were used to develop effective lytic phage variants that eliminated the infectious M. abscessus strain. Intravenous phage treatment was well-tolerated and linked to objective clinical improvements such as sternal wound healing, improved liver function, and significant clearance of infected skin nodules.
• Using phage cocktails in place of a single phage for therapy (Pirnay et al., 2011). The goal is similar to antiviral/cancer combination therapy: to target numerous, distinct mechanisms used by bacteria for infection, such as to improve bacterial killing and minimize/delay resistance mechanisms of bacteria (Altamirano and Barr 2021). Post-Coronavirus Disease of 2019 (COVID-19), gut dysbiosis was observed as a common side effect where bacteriophage cocktails proved to be an efficient way for restoring the normal balance of the microbiota. A study was carried out by Zurabov et al. for the rehabilitation of 30 patients following COVID-19, wherein two complex phage cocktails targeting multiple bacterial species were used, The real-time efficacy of the bacteriophages was determined against the clinical strain of K. pneumoniae. The use of phage cocktails for two weeks demonstrated safety and the absence of adverse effects. This study serves as a foundation for a larger and more extensive investigation of the usage of phage cocktails and their effects on the different parts of the microbiome.
• Using a different phage against the bacteria in place of the phage against which the resistance has been developed- this is simple with phages owing to their ubiquitous nature and abundance (Rohde et al., 2018).
• Using phages and antibiotics together (Tagliaferri et al., 2019).
Weighing pros and cons of antibiotics and phage therapy
Bacteriophages, as well as antibiotics, are antibacterial entities/substances that disrupt/inhibit the growth of bacteria by different modes of action. Bacteriophages employ their lytic cycle to lyse the bacterial cells, whereas antibiotics disrupt certain physiological processes of bacteria, such as cell wall or protein synthesis. Antibiotic resistance is a grave concern in the healthcare system, prompting researchers to seek new or modified medications. However, developing a new antibiotic is a challenging task that can take years, and the likelihood of bacteria developing resistance to newly discovered antibiotics will always be an unanswered question (Venkatesan, 2021). Recent studies for finding alternatives to antibiotics have sparked interest in phage therapy. Bacteriophages have once again become the center of attention as a potential therapeutic approach for antibiotic-resistant bacterial infections (Pirnay et al., 2018). Phage therapy and antibiotic therapy share certain characteristics, such as- both have to be administered at neutral pH environments, the success of therapy in each case is dependent on the time of initiation of treatment, the immune system of the patient plays an important role in treatment in both the cases, and there are chances that bacteria may become resistant to both phages and antibiotics (Loc-Carrillo and Abedon, 2011). Despite the shared purpose, there are certain striking differentiating features between the two.
In contrast to antibiotics, phages are highly specific in terms of infecting only particular species and strains of bacteria. This specificity of phages could be both positive as well as negative. On the positive side, being specific against bacteria, the incidents of collateral damage are greatly minimized. They only lyse the target bacteria and do not perturbate the natural microflora. Antibiotics, on the other hand, have been reported to have notorious secondary outcomes such as C. difficile infection and antibiotic-associated diarrhea. Moreover, their disruption in the gut microbial community can also lead to increased chances of diabetes, asthma, and obesity. Even with various advantages, this specificity limits phage therapy in certain ways. For instance, this therapy will not be useful in incidents where more than one strain of bacteria has to be targeted, as in the case of burn wounds. Moreover, phages will only have a narrow spectrum of action in contrast to broad-spectrum antibiotics (Elbreki et al., 2014).
The wide use of antibiotics over the years has also helped to document the various adverse effects that they might have caused. This data helps us to determine the safety of a drug in the treatment of a bacterial disease. Some of the complications related to antibiotic treatment are nephrotoxicity, anaphylaxis, hepatotoxicity, cardiotoxicity, and neurotoxicity, as well as a number of gastrointestinal and hematological complications (Granowitz and Brown, 2008). In contrast, phage therapy has only lately achieved recognition, and there is little evidence related to its safety. However, administering phages orally is generally considered safe (Bruttin and Brüssow, 2005). Despite this, there are some studies that demonstrate that phages can become a part of blood circulation after their translocation across the intestinal epithelium (Górski et al., 2006). Such an immunological response implies the potential to cause a harmful reaction in people with compromised immunity (Borysowski and Górski, 2008). However, other researchers doubt and say that it is unlikely for phages to generate such a reaction.
Another point that gives phage therapy an advantage over antibiotic therapy is the capability of phages to degrade extracellular polymeric substances (EPS) and diffuse the bacterial biofilms and hence giving them access to the bacteria. The phage disperses the biofilm with the aid of the enzyme EPS depolymerase that is present on the outer surface of its capsid. Antibiotics, on the other hand, are not equipped to treat biofilm-based bacterial infections. Higher doses are required in order to penetrate the dense biofilms, even then it does not guarantee complete eradication. The formation of biofilms on medical devices such as catheters, prostheses, and lenses by P. aeruginosa, L. monocytogenes, and Staphylococcus epidermidis can now be eliminated by phage treatment (Lin et al., 2017). Additionally, an alternative to broaden the lytic spectrum of phages can be combining phages with other antimicrobial treatments or other phages as well, as seen in phage cocktails (Kutter et al., 2010). An interesting point of advantage of phage therapy over antibiotic therapy is that even a broad-range phage cocktail will have greater specificity when it comes to its mode of action in comparison to that of the narrow spectrum antibiotics (Goodridge, 2010).
Recent Developments in Phage Therapy
Bacteriophages were utilized to treat patients suffering from various bacterial infections in Russia, Poland, and Georgia primarily during the time period of the 1920s–50s, soon after their discovery by D’Herelle in 1917. Lately, more countries joined, including Switzerland, Belgium, France, and the USA (Reardon, 2014). Our enhanced knowledge about genetics, pharmacology, immunology, and phage biology has upheld this re-established interest in phage therapy. The minimum suggested regulatory prerequisite for the therapeutic use of phages includes phages having a stringently lytic cycle, proven antimicrobial effect against the desired pathogen, and exclusion of endotoxins and contaminated bacterial debris. These major aspects involved in phage therapy have now been standardized to maximize treatment efficacy (Young and Gill, 2015). The Phage Therapy Centre of Hirszfeld Institute of Immunology and Experimental Therapy in Wroclaw, Poland, provides experimental antibacterial phage therapies to its patients against several pathogenic microbes including Acinetobacter, Burkholderia, Escherichia, Klebsiella, Proteus, Enterobacter, Enterococcus, Pseudomonas, Shigella, Staphylococcus, and Salmonella, among others. According to the Centre’s data, 35%–50% of patients treated in the center had favorable therapy outcomes. Each therapy is a combination of a few bacteriophage strains to boost antibacterial action and reduce the likelihood of developing phage resistance (Viertel et al., 2014). The potential of phages in the treatment of several human infections is still being studied at various levels. The US National Institutes of Health (NIH) has initiated a phase I/II trial with study no. -NCT04287478 intended to evaluate bacteriophage therapy in patients suffering from urinary tract infections in December 2020 with around 150 participants. This study is estimated to be completed by 2023.
A French company, Pherecydes Pharma, dedicated to precision phage therapy for the cure of resistant and/or complex bacterial infections, has announced the enrollment of the first patient in the PhagoDAIR Phase II clinical trial in June 2022. This is the world’s first initiative of phage therapy for osteoarticular infections of prosthetic devices infected by S. aureus. The study will also be conducted in European countries and intends to include 64 patients having infected hip joint or knee with S. aureus. The patients will be divided into two groups: control group and phage therapy. Patient’s receiving phage therapy will be given anti-S aureus phages active on their strain accompanied by suppressive antibiotic therapy. The evaluation process will start 12 weeks after the administration of phages, and will continue for 2 years. By the end of 2023, the preliminary results are anticipated, and the evaluation process will continue until the first half of 2025. Based on the initial outcomes of the Phase II trial, Pherecydes Pharma is preparing for a Phase III trial, which could start in 2024. Advancements in the gene-editing tool CRISPR/Cas have led to the achievement of new possibilities in phage therapy. One such example is a bioengineered phage able to deliver a CRISPR/Cas Programme to disrupt the gene for antibiotic resistance and can also eliminate antibiotic-resistant plasmids (Yosef et al., 2015). These phages can be sprayed onto hospital surfaces to minimize the incidence and dissemination of antibiotic resistance genes. Although the domain of bio-engineered phages is still nascent, it will definitely spawn many valuable innovations like the one mentioned above.
The concept of phage therapy in India is in its juvenile stage and will take some more years of research and development to establish itself as a medicinal procedure. However, the country has been encountering antimicrobial resistance for decades, and there seems to be no promising alternative to tackle it. After the COVID-19 pandemic, the crisis has amplified, with many more bacteria augmenting the list of MDRs. In 2018, over 500,000 new cases of rifampicin-resistant tuberculosis were reported worldwide. Seventy-eight percent of these patients had multidrug-resistant tuberculosis. According to the Global Tuberculosis Report 2019, India accounted for 27% of the total global burden in 2018. A recent study on terminally ill hospitalized COVID-19 patients in India reports the emergence of various gram-negative bacteria to be resistant to various broad-spectrum antibiotics, including Carbapenem, during secondary infections (Vijay et al., 2021). Carbapenem is a broad-spectrum antibiotic and is considered to be an unfailing drug treating bacterial infections. Antibiotic research has reached a roadblock where bacteriophage therapy seems to be an alternative treatment. The three experimental studies initiated by Banaras Hindu University, India, on clinical trials of phage therapy, showed the effectiveness of topical phage preparations in chronic wound healing. Gupta et al. (2019) conducted a clinical study that demonstrated the importance of bacteriophage therapy in chronic wounds caused by antibiotic-resistant bacteria. The study included twenty patients who had chronic non-healing ulcers for more than six weeks. Within a few weeks, a significant improvement in the form of complete wound epithelization could be accomplished (Gupta et al., 2019). A study by Patel et al. (2021) was carried out with forty-eight patients having at least one full-thickness wound that did not heal in 6 weeks with conventional wound treatments. Positive results were observed with significant improvement in wound healing in >82% of the patients by the end of three months of follow-up. Moreover, the study also suggested that specialized phage therapy is similarly effective whether the patient is diabetic or not. In diabetic patients, however, healing was relatively delayed (Patel et al., 2021). Both studies show that topical phage therapy resulted in complete clinical wound healing in patients who were refractory to traditional therapy. Furthermore, the antibiotic resistance status of the bacteria suspected of being involved in the wound had no impact on the outcome of the therapy. Another recently published non-randomized case-control study on the impact of bacteriophages on the healing process of infected acute traumatic wounds by Bhartiya et al. (2022) has shown impressive results. When compared to the control, i.e., conventional therapy, the average number of days needed for total granulation of wounds, achieving sterility, and time of recovery, was lessened by half (Bhartiya et al., 2022). Because phages are highly specific for their bacterial hosts, phage cocktail preparations typically ensure a broader spectrum of activity and decrease the probability of the development of bacterial mutants resistant to phages. As a result, all of these studies used a bacteriophage cocktail as therapy. GangaGen, an Indian pharmaceutical company, is testing ectolysin encoded by phage P128 against methicillin resistant S. aureus (MRSA), and they are in Phase 2 of their clinical trials. More such trials in the future will pave the way for revolutionary medicine against drug-resistant bacteria in India (Channabasappa et al., 2018). In 2021 a case report was published in which an Indian patient suffering from an inflammatory disorder of the prostate gland known as Chronic Bacterial Prostatitis (CBP) showed recuperation in his symptoms by phage preparations from the Eliava Institute Georgia. Earlier, the CBP conditions were difficult to resolve even after multiple antibacterial treatments (Johri et al., 2021). Several such clinical trials are required in India to demonstrate the safety and efficacy of data generated in preclinical experiments of phage therapy before they can be reliably converted into clinical practice.
As we are moving ahead in modern medicine to provide a better healthcare system to mankind, the escalation of antibiotic resistance is dragging our feet back into the dark ages of medicines. According to a 2014 UK Government-commissioned Review on Antimicrobial Resistance, AMR might kill 10 million people annually by 2050 (O’neill, 2014). WHO, numerous other organizations, and researchers concur that the development of AMR is a critical issue that necessitates a global, coordinated action plan to address. Bacteria that are multidrug-resistant (MDR), extensively drug-resistant (XDR), and pan-drug-resistant (PDR) are extremely lethal. The global upsurge of antibiotic resistance increases the likelihood of returning to the clinical equivalent of the pre-antibiotic era and necessitates the development of new groups of antimicrobials that could improve the efficacy of currently used drugs. The development of new antibacterial drugs that can facilitate a more strategic treatment approach without affecting the beneficial natural microbiota can be made possible by the promising but challenging approach of phage therapy. In the post-antibiotic era, phage therapy is the best natural complement. The mode of action of bacteriophages is different from that of antibiotics, which makes antibiotic-resistant bacterial strains susceptible to phage infection. This is a significant advantage of phage therapy. Numerous phage therapy clinical trials in phase I and phase II have shown that there aren't many severe safety issues with phages. The effectiveness of phage therapy depends on the concentration of phages (the dose) administered at the infection site as well as on their capacity to target and kill bacteria, inhibit bacterial development, and eradicate the infection. This has been demonstrated in animal studies. Several Gram-positive bacterial infections, including MRSA and VRE, are still incurable. Despite the availability of last-resort antibiotics, the mortality rate for these drug-resistant bacterial infections remain high. Physicians, funding bodies, governments, and policymakers must not overlook the use of phage therapy to treat such infections. The use of phage-antibiotic combinations is becoming more popular in phage research, but implementation is debilitated by a lack of proper implementation. Though there are a limited number of clinical trials which are in process, and only some are in phase II, there is enough evidence from Poland and Georgia that bacteriophages are effectual therapeutic agents. In India, clinical trials have been conducted by Banaras Hindu University (BHU), and have shown promising results. However, constraints such as a limited host range, bacterial resistance to phage, manufacturing costs, and challenging dose finding/delivery methods must be addressed. Recent advancements in synthetic genome assembly and viral genome engineering can be used to develop phage with remarkable pharmacological activities. The advancement in technology, such as next-generation sequencing and high-throughput screening, have augmented the likelihood of genome analysis and phage characterization. Many studies and clinical trials conducted worldwide have shed more light on bacteriophage therapy as an effective substitute. Though phages would not reinstate antibiotics, they are the best complement for the non-antibiotic era.
Acknowledgement: The authors PB, SK, IS, RS and RM would like to thank the management of Shaheed Rajguru College of Applied Science for Women, University of Delhi, New Delhi, India for providing facilities for carrying out the present study. MJ would like to thank Centre of research for development, Parul University, Vadodara, Gujrat, India. PK would like to thank Instituto de Conservacióny Mejora de la Agrodiversidad Valenciana, Universitat Politècnica de València, Valencia, Spain.
Funding Statement: The authors received no specific funding for this study.
Author Contributions: The authors confirm contribution to the paper as follows: study conception and design: PB, SK, IS and PK conceptualized the present study. SK and IS have contributed equally. SK, IS, RS, MJ and PB were involved in acquisition, analysis of the literature and drafting of the manuscript. PB, RS, RM, MJ and PK have contributed in drafting and critical revision of the manuscript. All authors have contributed substantially and approve the final version of the manuscript for publication.
Availability of Data and Materials: Not applicable.
Ethics Approval: Not applicable.
Conflicts of Interest: The authors declare that they have no conflicts of interest to report regarding the present study.
References
Abedon ST, Thomas-Abedon C (2010). Phage therapy pharmacology. Current Pharmaceutical Biotechnology 11: 28–47. https://doi.org/10.2174/138920110790725410 [Google Scholar] [PubMed] [CrossRef]
Altamirano FLG, Barr JJ (2021). Unlocking the next generation of phage therapy: The key is in the receptors. Current Opinion in Biotechnology 68: 115–123. https://doi.org/10.1016/j.copbio.2020.10.002 [Google Scholar] [PubMed] [CrossRef]
Babalova EG, Katsitadze KT, Sakvarelidze LA, NSh I, Sharashidze TG, Badashvili VA, Dekanosidze NG (1968). Preventive value of dried dysentery bacteriophage. Zhurnal Mikrobiologii, Epidemiologii I Immunobiologii 45: 143–145. [Google Scholar] [PubMed]
Bernheim A, Sorek R (2020). The pan-immune system of bacteria: Antiviral defence as a community resource. Nature Reviews Microbiology 18: 113–119. https://doi.org/10.1038/s41579-019-0278-2 [Google Scholar] [PubMed] [CrossRef]
Bhartiya SK, Prasad R, Sharma S, Shukla V, Nath G, Kumar R (2022). Biological therapy on infected traumatic wounds: A case-control study. The International Journal of Lower Extremity Wounds 5: 15347346211072779. https://doi.org/10.1177/15347346211072779 [Google Scholar] [PubMed] [CrossRef]
Biswas B, Adhya S, Washart P, Paul B, Trostel AN, Powell B, Merril CR (2002). Bacteriophage therapy rescues mice bacteremic from a clinical isolate of vancomycin-resistant Enterococcus faecium. Infection and Immunity 70: 204–210. https://doi.org/10.1128/IAI.70.1.204-210.2002 [Google Scholar] [PubMed] [CrossRef]
Borysowski J, Górski A (2008). Is phage therapy acceptable in the immunocompromised host? International Journal of Infectious Diseases 12: 466–471. https://doi.org/10.1016/j.ijid.2008.01.006 [Google Scholar] [PubMed] [CrossRef]
Borysowski J, Weber-Dąbrowska B, Górski A (2006). Bacteriophage endolysins as a novel class of antibacterial agents. Experimental Biology and Medicine 231: 366–377. https://doi.org/10.1177/153537020623100402 [Google Scholar] [PubMed] [CrossRef]
Brives C, Pourraz J (2020). Phage therapy as a potential solution in the fight against AMR: Obstacles and possible futures. Palgrave Communications 6: 1–11. https://doi.org/10.1057/s41599-020-0478-4 [Google Scholar] [CrossRef]
Bruttin A, Brüssow H (2005). Human volunteers receiving Escherichia coli phage T4 orally: A safety test of phage therapy. Antimicrobial Agents and Chemotherapy 49: 2874–2878. https://doi.org/10.1128/AAC.49.7.2874-2878.2005 [Google Scholar] [PubMed] [CrossRef]
Cann AJ (2016Replication, vol. 4, pp. 105–133. Principles of Molecular Virology. [Google Scholar]
Capparelli R, Nocerino N, Iannaccone M, Ercolini D, Parlato M, Chiara M, Iannelli D (2010). Bacteriophage therapy of Salmonella enterica: A fresh appraisal of bacteriophage therapy. The Journal of Infectious Diseases 201: 52–61. https://doi.org/10.1086/648478 [Google Scholar] [PubMed] [CrossRef]
Chanishvili N (2012). Phage therapy—history from twort and d'Herelle through soviet experience to current approaches. Advances in Virus Research 83: 3–40. https://doi.org/10.1016/B978-0-12-394438-2.00001-3 [Google Scholar] [PubMed] [CrossRef]
Channabasappa S, Chikkamadaiah R, Durgaiah M, Kumar S, Ramesh K, Sreekanthan A, Sriram B (2018). Efficacy of chimeric ectolysin P128 in drug-resistant Staphylococcus aureus bacteraemia in mice. Journal of Antimicrobial Chemotherapy 73: 3398–3404. https://doi.org/10.1093/jac/dky365 [Google Scholar] [PubMed] [CrossRef]
Cisek AA, Dąbrowska I, Gregorczyk KP, Wyżewski Z (2017). Phage therapy in bacterial infections treatment: One hundred years after the discovery of bacteriophages. Current Microbiology 74: 277–283. https://doi.org/10.1007/s00284-016-1166-x [Google Scholar] [PubMed] [CrossRef]
Cisło M, Dabrowski M, Weber-Dabrowska B, Woytoń A (1987). Bacteriophage treatment of suppurative skin infections. Archivum Immunologiae Etherapiae 35: 175–183. [Google Scholar]
Dabrowska K, Switała-Jelen K, Opolski A, Weber-Dabrowska B, Gorski A (2005). Bacteriophage penetration in vertebrates. Journal of Applied Microbiology 98: 7–13. [Google Scholar] [PubMed]
Datta DB, Arden BERNHARD, Henning U (1977). Major proteins of the Escherichia coli outer cell envelope membrane as bacteriophage receptors. Journal of Bacteriology 131: 821–829.4. [Google Scholar] [PubMed]
Dedrick RM, Freeman KG, Nguyen JA, Bahadirli-Talbott A, Smith BE et al. (2021). Potent antibody-mediated neutralization limits bacteriophage treatment of a pulmonary Mycobacterium abscessus infection. Nature Medicine 27: 1357–1361. https://doi.org/10.1038/s41591-021-01403-9 [Google Scholar] [PubMed] [CrossRef]
Dedrick RM, Smith BE, Cristinziano M, Freeman KG, Jacobs-Sera D et al. (2022). Phage therapy of mycobacterium infections: Compassionate-use of phages in twenty patients with drug-resistant mycobacterial disease. Clinical Infectious Diseases 76: 103–112 https://doi.org/10.1093/cid/ciac453 [Google Scholar] [PubMed] [CrossRef]
Dewey JS, Savva CG, White RL, Vitha S, Holzenburg A, Young R (2010). Micron-scale holes terminate the phage infection cycle. Proceedings of the National Academy of Sciences of the United States of America 107: 2219–2223. [Google Scholar] [PubMed]
Donovan DM, Foster-Frey J (2008). LambdaSa2 prophage endolysin requires Cpl-7-binding domains and amidase-5 domain for antimicrobial lysis of streptococci. FEMS Microbiology Letters 287: 22–33. [Google Scholar] [PubMed]
Doub JB (2021). Risk of bacteriophage therapeutics to transfer genetic material and contain contaminants beyond endotoxins with clinically relevant mitigation strategies. Infection and Drug Resistance 14: 5629–5637. https://doi.org/10.2147/IDR.S341265 [Google Scholar] [PubMed] [CrossRef]
Drulis-Kawa Z, Majkowska-Skrobek G, Maciejewska B (2015). Bacteriophages and phage-derived proteins-application approaches. Current Medicinal Chemistry 22: 1757–1773. https://doi.org/10.2174/0929867322666150209152851 [Google Scholar] [PubMed] [CrossRef]
Elbreki M, Ross RP, Hill C, O'Mahony J, McAuliffe O, Coffey A (2014). Bacteriophages and their derivatives as biotherapeutic agents in disease prevention and treatment. Journal of Viruses 2014: 1–20. https://doi.org/10.1155/2014/382539 [Google Scholar] [CrossRef]
Ferry T, Kolenda C, Batailler C, Gustave CA, Lustig S, Malatray M, Laurent F (2020). Phage therapy as adjuvant to conservative surgery and antibiotics to salvage patients with relapsing S. aureus prosthetic knee infection. Frontiers in Medicine 7: 570572. https://doi.org/10.3389/fmed.2020.570572 [Google Scholar] [PubMed] [CrossRef]
Fish R, Kutter E, Wheat G, Blasdel B, Kutateladze M, Kuhl S (2016). Bacteriophage treatment of intransigent diabetic toe ulcers: A case series. Journal of Wound Care 25: S27–S33. https://doi.org/10.12968/jowc.2016.25.7.S27 [Google Scholar] [CrossRef]
Fu W, Forster T, Mayer O, Curtin JJ, Lehman SM, Donlan RM (2010). Bacteriophage cocktail for the prevention of biofilm formation by Pseudomonas aeruginosa on catheters in an in vitro model system. Antimicrobial Agents and Chemotherapy 54: 397–404. [Google Scholar] [PubMed]
Gandhi NR, Moll A, Sturm AW, Pawinski R, Govender T, Lalloo U, Friedland G (2006). Extensively drug-resistant tuberculosis as a cause of death in patients co-infected with tuberculosis and HIV in a rural area of South Africa. The Lancet 368: 1575–1580. [Google Scholar]
Gill JJ, Hyman P (2010). Phage choice, isolation, and preparation for phage therapy. Current Pharmaceutical Biotechnology 11: 2–14. [Google Scholar] [PubMed]
Goodridge LD (2010). Designing phage therapeutics. Current Pharmaceutical Biotechnology 11: 15–27. [Google Scholar] [PubMed]
Granowitz EV, Brown RB (2008). Antibiotic adverse reactions and drug interactions. Critical Care Clinics 24: 421–442. https://doi.org/10.1016/j.ccc.2007.12.011 [Google Scholar] [PubMed] [CrossRef]
Gupta R, Prasad Y (2011). Efficacy of polyvalent bacteriophage P-27/HP to control multidrug resistant Staphylococcus aureus associated with human infections. Current Microbiology 62: 255–260. https://doi.org/10.1007/s00284-010-9699-x [Google Scholar] [PubMed] [CrossRef]
Gupta P, Singh HS, Shukla VK, Nath G, Bhartiya SK (2019). Bacteriophage therapy of chronic nonhealing wound: Clinical study. The International Journal of lower Extremity Wounds 18: 171–175. https://doi.org/10.1177/1534734619835115 [Google Scholar] [PubMed] [CrossRef]
Górski A, Ważna E, Dąbrowska BW, Dąbrowska K, Świtała-Jeleń K, Międzybrodzki R (2006). Bacteriophage translocation. FEMS Immunology & Medical Microbiology 46: 313–319. [Google Scholar]
Jault P, Leclerc T, Jennes S, Pirnay JP, Que YA, Resch G, Gabard J (2019). Efficacy and tolerability of a cocktail of bacteriophages to treat burn wounds infected by Pseudomonas aeruginosa (PhagoBurnA randomised, controlled, double-blind phase 1/2 trial. The Lancet Infectious Diseases 19: 35–45. https://doi.org/10.1016/S1473-3099(18)30482-1 [Google Scholar] [PubMed] [CrossRef]
Johri AV, Johri P, Hoyle N, Pipia L, Nadareishvili L, Nizharadze D (2021). Case report: Chronic bacterial prostatitis treated with phage therapy after multiple failed antibiotic treatments. Frontiers in Pharmacology 12: 692614. https://doi.org/10.3389/fphar.2021.692614 [Google Scholar] [PubMed] [CrossRef]
Jun JW, Shin TH, Kim JH, Shin SP, Han JE, Heo GJ, Park SC (2014). Bacteriophage therapy of a Vibrio parahaemolyticus infection caused by a multiple-antibiotic-resistant O3: K6 pandemic clinical strain. The Journal of Infectious Diseases 210: 72–78. https://doi.org/10.1093/infdis/jiu059 [Google Scholar] [PubMed] [CrossRef]
Kaźmierczak N, Grygorcewicz B, Roszak M, Bochentyn B, Piechowicz L (2022). Comparative assessment of bacteriophage and antibiotic activity against multidrug-resistant Staphylococcus aureus biofilms. International Journal of Molecular Sciences 23: 1274. https://doi.org/10.3390/ijms23031274 [Google Scholar] [PubMed] [CrossRef]
Kortright KE, Chan BK, Koff JL, Turner PE (2019). Phage therapy: a renewed approach to combat antibiotic-resistant bacteria. Cell Host & Microbe 25: 219–232. [Google Scholar]
Koskella B, Brockhurst MA (2014). Bacteria-phage coevolution as a driver of ecological and evolutionary processes in microbial communities. FEMS Microbiology Reviews 38: 916–931. https://doi.org/10.1111/1574-6976.12072 [Google Scholar] [PubMed] [CrossRef]
Koskella B, Meaden S (2013). Understanding bacteriophage specificity in natural microbial communities. Viruses 5: 806–823. https://doi.org/10.3390/v5030806 [Google Scholar] [PubMed] [CrossRef]
Kuchment A (2011). The forgotten cure: The past and future of phage therapy. Springer Science & Business Media. Springer: New York, NY. https://doi.org/10.1007/978-1-4614-0251-0 [Google Scholar] [CrossRef]
Kutateladze Á., Adamia R (2008). Phage therapy experience at the Eliava Institute. Medecine et Maladies Infectieuses 38: 426–430. https://doi.org/10.1016/j.medmal.2008.06.023 [Google Scholar] [PubMed] [CrossRef]
Kutateladze M, Adamia R (2010). Bacteriophages as potential new therapeutics to replace or supplement antibiotics. Trends in Biotechnology 28: 591–595. https://doi.org/10.1016/j.tibtech.2010.08.001 [Google Scholar] [PubMed] [CrossRef]
Kutter E, de Vos D, Gvasalia G, Alavidze Z, Gogokhia L, Kuhl S, Abedon ST (2010). Phage therapy in clinical practice: Treatment of human infections. Current Pharmaceutical Biotechnology 11: 69–86. https://doi.org/10.2174/138920110790725401 [Google Scholar] [PubMed] [CrossRef]
Labrie SJ, Samson JE, Moineau S (2010). Bacteriophage resistance mechanisms. Nature Reviews Microbiology 8: 317–327. https://doi.org/10.1038/nrmicro2315 [Google Scholar] [PubMed] [CrossRef]
Leitner L, Ujmajuridze A, Chanishvili N, Goderdzishvili M, Chkonia I et al. (2021). Intravesical bacteriophages for treating urinary tract infections in patients undergoing transurethral resection of the prostate: A randomised, placebo-controlled, double-blind clinical trial. The Lancet Infectious Diseases 21: 427–436. https://doi.org/10.1016/s1473-3099(20)30330-3 [Google Scholar] [PubMed] [CrossRef]
Le S, Yao X, Lu S, Tan Y, Rao X, Li M, Hu F (2014). Chromosomal DNA deletion confers phage resistance to Pseudomonas aeruginosa. Scientific Reports 4: 4738. https://doi.org/10.1038/srep04738 [Google Scholar] [PubMed] [CrossRef]
Lin DM, Koskella B, Lin HC (2017). Phage therapy: An alternative to antibiotics in the age of multi-drug resistance. World Journal of Gastrointestinal Pharmacology and Therapeutics 8: 162. https://doi.org/10.4292/wjgpt.v8.i3.162 [Google Scholar] [PubMed] [CrossRef]
Lindberg AA (1973). Bacteriophage receptors. Annual Reviews in Microbiology 27: 205–241. https://doi.org/10.1146/annurev.mi.27.100173.001225 [Google Scholar] [PubMed] [CrossRef]
Loc-Carrillo C, Abedon ST (2011). Pros and cons of phage therapy. Bacteriophage 1: 111–114. https://doi.org/10.4161/bact.1.2.14590 [Google Scholar] [PubMed] [CrossRef]
Loessner MJ (2005). Bacteriophage endolysins—current state of research and applications. Current Opinion in Microbiology 8: 480–487. https://doi.org/10.1016/j.mib.2005.06.002 [Google Scholar] [PubMed] [CrossRef]
Loganathan A, Manohar P, Eniyan K, VinodKumar CS, Leptihn S, Nachimuthu R (2021). Phage therapy as a revolutionary medicine against Gram-positive bacterial infections. Beni-Suef University Journal of Basic and Applied Sciences 10: 1–15. https://doi.org/10.1186/s43088-021-00141-8 [Google Scholar] [PubMed] [CrossRef]
Manohar P, Tamhankar AJ, Leptihn S, Ramesh N (2019). Pharmacological and immunological aspects of phage therapy. Infectious Microbes & Diseases 1: 34–42. https://doi.org/10.1097/IM9.0000000000000013 [Google Scholar] [CrossRef]
McGee LW, Barhoush Y, Shima R, Hennessy M (2023). Phage-resistant mutations impact bacteria susceptibility to future phage infections and antibiotic response. Ecology and Evolution 13: e9712. https://doi.org/10.1002/2Fece3.9712 [Google Scholar] [CrossRef]
Mendes BG, Duan Y, Schnabl B (2022). Immune response of an oral enterococcus faecalis phage cocktail in a mouse model of ethanol-induced liver disease. Viruses 14: 490. https://doi.org/10.3390/v14030490 [Google Scholar] [PubMed] [CrossRef]
Merabishvili M, Pirnay JP, Verbeken G, Chanishvili N, Tediashvili M, Lashkhi N, Vaneechoutte M (2009). Quality-controlled small-scale production of a well-defined bacteriophage cocktail for use in human clinical trials. PLoS One 4: e4944. https://doi.org/10.1371/journal.pone.0004944 [Google Scholar] [PubMed] [CrossRef]
Minot S, Sinha R, Chen J, Li H, Keilbaugh SA, Wu GD, Bushman FD (2011). The human gut virome: Inter-individual variation and dynamic response to diet. Genome Research 21: 1616–1625. https://doi.org/10.1101/gr.122705.111 [Google Scholar] [PubMed] [CrossRef]
Moelling K, Broecker F, Willy C (2018). A wake-up call: We need phage therapy now. Viruses 10: 688. https://doi.org/10.3390/v10120688 [Google Scholar] [PubMed] [CrossRef]
Myelnikov D (2018). An alternative cure: The adoption and survival of bacteriophage therapy in the USSR, 1922-1955. Journal of the History of Medicine and Allied Sciences 73: 385–411. https://doi.org/10.1093/jhmas/jry024 [Google Scholar] [PubMed] [CrossRef]
Navarro F, Muniesa M (2017). Phages in the human body. Frontiers in Microbiology 8: 566. https://doi.org/10.3389/fmicb.2017.00566 [Google Scholar] [PubMed] [CrossRef]
Nelson DC, Schmelcher M, Rodriguez-Rubio L, Klumpp J, Pritchard DG, Dong S, Donovan DM (2012). Endolysins as antimicrobials. Advances in Virus Research 83: 299–365. [Google Scholar] [PubMed]
Nikaido H (2003). Molecular basis of bacterial outer membrane permeability revisited. Microbiology and Molecular Biology Reviews 67: 593–656. [Google Scholar] [PubMed]
O’neill J (2014). Antimicrobial resistance: Tackling a crisis for the health and wealth of nations. [Google Scholar]
Oechslin F (2018). Resistance development to bacteriophages occurring during bacteriophage therapy. Viruses 10: 351. [Google Scholar] [PubMed]
Oechslin F, Piccardi P, Mancini S, Gabard J, Moreillon P, Entenza JM, Que YA (2017). Synergistic interaction between phage therapy and antibiotics clears Pseudomonas aeruginosa infection in endocarditis and reduces virulence. The Journal of Infectious Diseases 215: 703–712. [Google Scholar] [PubMed]
Palomino JC, Martin A (2014). Drug resistance mechanisms in Mycobacterium tuberculosis. Antibiotics 3: 317–340. [Google Scholar] [PubMed]
Paolozzi L, Ghelardini P (2006). The bacteriophage Mu. In: Calendar R, The Bacteriophages, pp. 469–496 New York: Oxford University Press. [Google Scholar]
Patel DR, Bhartiya SK, Kumar R, Shukla VK, Nath G (2021). Use of customized bacteriophages in the treatment of chronic nonhealing wounds: A prospective study. The International Journal of Lower Extremity Wounds 20: 37–46. https://doi.org/10.1177/1534734619881076 [Google Scholar] [PubMed] [CrossRef]
Patey O, McCallin S, Mazure H, Liddle M, Smithyman A, Dublanchet A (2018). Clinical indications and compassionate use of phage therapy: Personal experience and literature review with a focus on osteoarticular infections. Viruses 11: 18. https://doi.org/10.3390/v11010018 [Google Scholar] [PubMed] [CrossRef]
Pires DP, Costa AR, Pinto G, Meneses L, Azeredo J (2020). Current challenges and future opportunities of phage therapy. FEMS Microbiology Reviews 44: 684–700. https://doi.org/10.1093/femsre/fuaa017 [Google Scholar] [PubMed] [CrossRef]
Pirnay JP, de Vos D, Verbeken G, Merabishvili M, Chanishvili N, Vaneechoutte M, Adamia R (2011). The phage therapy paradigm: Prêt-à-porter or sur-mesure? Pharmaceutical Research 28: 934–937. https://doi.org/10.1007/s11095-010-0313-5 [Google Scholar] [PubMed] [CrossRef]
Pirnay JP, Verbeken G, Ceyssens PJ, Huys I, de Vos D, Ameloot C, Fauconnier A (2018). The magistral phage. Viruses 10: 64. https://doi.org/10.3390/v10020064 [Google Scholar] [PubMed] [CrossRef]
Pouillot F, Chomton M, Blois H, Courroux C, Noelig J, Bidet P, Bonacorsi S (2012). Efficacy of bacteriophage therapy in experimental sepsis and meningitis caused by a clone O25b: H4-ST131 Escherichia coli strain producing CTX-M-15. Antimicrobial Agents and Chemotherapy 56: 3568–3575. https://doi.org/10.1128/AAC.06330-11 [Google Scholar] [PubMed] [CrossRef]
Rakhuba DV, Kolomiets EI, Dey ES, Novik GI (2010). Bacteriophage receptors, mechanisms of phage adsorption and penetration into host cell. Polish Journal of Microbiology 59: 161–165. [Google Scholar]
Reardon S (2014). Phage therapy gets revitalized: The rise of antibiotic resistance rekindles interest in a century-old virus treatment. Nature 510: 15–17. https://doi.org/10.1038/510015a [Google Scholar] [PubMed] [CrossRef]
Reyes A, Semenkovich NP, Whiteson K, Rohwer F, Gordon JI (2012). Going viral: Next-generation sequencing applied to phage populations in the human gut. Nature Reviews Microbiology 10: 607–617. https://doi.org/10.1038/nrmicro2853 [Google Scholar] [PubMed] [CrossRef]
Rice LB (2008). Federal funding for the study of antimicrobial resistance in nosocomial pathogens: No ESKAPE. The Journal of Infectious Diseases 197: 1079–1081. https://doi.org/10.1086/533452 [Google Scholar] [PubMed] [CrossRef]
Rohde C, Wittmann J, Kutter E (2018). Bacteriophages: A therapy concept against multi-drug-resistant bacteria. Surgical Infections 19: 737–744. https://doi.org/10.1089/sur.2018.184 [Google Scholar] [PubMed] [CrossRef]
Rose T, Verbeken G, de Vos D, Merabishvili M, Vaneechoutte M, Lavigne R, Pirnay JP (2014). Experimental phage therapy of burn wound infection: Difficult first steps. International Journal of Burns and Trauma 4: 66–73. [Google Scholar] [PubMed]
Sarker SA, McCallin S, Barretto C, Berger B, Pittet AC, Sultana S, Brüssow H (2012). Oral T4-like phage cocktail application to healthy adult volunteers from Bangladesh. Virology 434: 222–232. https://doi.org/10.1016/j.virol.2012.09.002 [Google Scholar] [PubMed] [CrossRef]
Schmelcher M, Donovan DM, Loessner MJ (2012). Bacteriophage endolysins as novel antimicrobials. Future Microbiology 7: 1147–1171. https://doi.org/10.2217/fmb.12.97 [Google Scholar] [PubMed] [CrossRef]
Schuch R, Cassino C, Vila-Farres X (2022). Direct lytic agents: Novel, rapidly acting potential antimicrobial treatment modalities for systemic use in the era of rising antibiotic resistance. Frontiers in Microbiology 400: 284. https://doi.org/10.3389/fmicb.2022.841905 [Google Scholar] [PubMed] [CrossRef]
Shi Y, Yan Y, Ji W, Du B, Meng X, Wang H, Sun J (2012). Characterization and determination of holin protein of Streptococcus suis bacteriophage SMP in heterologous host. Virology Journal 9: 1–11. https://doi.org/10.1186/1743-422X-9-70 [Google Scholar] [PubMed] [CrossRef]
Skurnik M, Strauch E (2006). Phage therapy: Facts and fiction. International Journal of Medical Microbiology 296: 5–14. https://doi.org/10.1016/j.ijmm.2005.09.002 [Google Scholar] [PubMed] [CrossRef]
Slopek S, Weber-Dabrowska B, Dabrowski M, Kucharewicz-Krukowska A (1987). Results of bacteriophage treatment of suppurative bacterial infections in the years 1981–1986. Archivum Immunologiae et Therapiae Experimentalis 35: 569–583. [Google Scholar] [PubMed]
Smith HW, Huggins MB (1982). Successful treatment of experimental Escherichia coli infections in mice using phage: Its general superiority over antibiotics. Microbiology 128: 307–318. https://doi.org/10.1099/00221287-128-2-307 [Google Scholar] [PubMed] [CrossRef]
Smith HW, Huggins MB, Shaw KM (1987). Factors influencing the survival and multiplication of bacteriophages in calves and in their environment. Microbiology 133: 1127–1135. https://doi.org/10.1099/00221287-133-5-1127 [Google Scholar] [PubMed] [CrossRef]
Sulakvelidze A, Alavidze Z (2001). Morris Jr JG. Bacteriophage therapy. Antimicrobe Agents Chemother 45: 649–659. https://doi.org/10.1128/AAC.45.3.649-659.2001 [Google Scholar] [PubMed] [CrossRef]
Sybesma W, Rohde C, Bardy P, Pirnay JP, Cooper I et al. (2018). Silk route to the acceptance and re-implementation of bacteriophage therapy–part II. Antibiotics 7: 35. https://doi.org/10.3390/antibiotics7020035 [Google Scholar] [PubMed] [CrossRef]
Tagliaferri TL, Jansen M, Horz HP (2019). Fighting pathogenic bacteria on two fronts: Phages and antibiotics as combined strategy. Frontiers in Cellular and Infection Microbiology 9: 22. https://doi.org/10.3389/fcimb.2019.00022 [Google Scholar] [PubMed] [CrossRef]
To KH, Young R (2014). Probing the structure of the S105 hole. Journal of Bacteriology 196: 3683–3689. https://doi.org/10.1128/JB.01673-14 [Google Scholar] [PubMed] [CrossRef]
Torabi LR, Naghavi NS, Doudi M, Monajemi R (2021). Efficacious antibacterial potency of novel bacteriophages against ESBL-producing Klebsiella pneumoniae isolated from burn wound infections. Iranian Journal of Microbiology 13: 678–690. [Google Scholar] [PubMed]
Turner D, Shkoporov AN, Lood C, Millard AD, Dutilh BE et al. (2023). Abolishment of morphology-based taxa and change to binomial species names: 2022 taxonomy update of the ICTV bacterial viruses subcommittee. Archives of Virology 168: 74. https://doi.org/10.1007/s00705-022-05694-2 [Google Scholar] [PubMed] [CrossRef]
Udwadia ZF, Amale RA, Ajbani KK, Rodrigues C (2012). Totally drug-resistant tuberculosis in India. Clinical Infectious Diseases 54: 579–581. https://doi.org/10.1093/cid/cir889 [Google Scholar] [PubMed] [CrossRef]
Vandenheuvel D, Lavigne R, Brüssow H (2015). Bacteriophage therapy: Advances in formulation strategies and human clinical trials. Annual Review of Virology 2: 599–618. https://doi.org/10.1146/annurev-virology-100114-054915 [Google Scholar] [PubMed] [CrossRef]
Velayati AA, Masjedi MR, Farnia P, Tabarsi P, Ghanavi J, ZiaZarifi AH, Hoffner SE (2009). Emergence of new forms of totally drug-resistant tuberculosis bacilli: Super extensively drug-resistant tuberculosis or totally drug-resistant strains in Iran. Chest 136: 420–425. https://doi.org/10.1378/chest.08-2427 [Google Scholar] [PubMed] [CrossRef]
Venhorst J, van der Vossen JM, Agamennone V (2022). Battling Enteropathogenic Clostridia: Phage Therapy for Clostridioides difficile and Clostridium perfringens. Frontiers in Microbiology 13: 1674. https://doi.org/10.3389/fmicb.2022.891790 [Google Scholar] [PubMed] [CrossRef]
Venkatesan P (2021). WHO 2020 report on the antibacterial production and development pipeline. The Lancet Microbe 2: e239. https://doi.org/10.1016/S2666-5247(21)00124-5 [Google Scholar] [PubMed] [CrossRef]
Viertel TM, Ritter K, Horz HP (2014). Viruses versus bacteria—novel approaches to phage therapy as a tool against multidrug-resistant pathogens. Journal of Antimicrobial Chemotherapy 69: 2326–2336. https://doi.org/10.1093/jac/dku173 [Google Scholar] [PubMed] [CrossRef]
Vijay S, Bansal N, Rao BK, Veeraraghavan B, Rodrigues C, Wattal C, Walia K (2021). Secondary infections in hospitalized COVID-19 patients: Indian experience. Infection and Drug Resistance 14: 1893–1903. https://doi.org/10.2147/IDR.S299774 [Google Scholar] [PubMed] [CrossRef]
Wang IN, Smith DL, Young R (2000). Holins: The protein clocks of bacteriophage infections. Annual Reviews in Microbiology 54: 799–825. https://doi.org/10.1146/annurev.micro.54.1.799 [Google Scholar] [PubMed] [CrossRef]
Watanabe R, Matsumoto T, Sano G, Ishii Y, Tateda K, Sumiyama Y, Yamaguchi K (2007). Efficacy of bacteriophage therapy against gut-derived sepsis caused by Pseudomonas aeruginosa in mice. Antimicrobial Agents and Chemotherapy 51: 446–452. https://doi.org/10.1128/AAC.00635-06 [Google Scholar] [PubMed] [CrossRef]
Weber-Dąbrowska B, Zimecki M, Mulczyk M, Górski A (2002). Effect of phage therapy on the turnover and function of peripheral neutrophils. FEMS Immunology & Medical Microbiology 34: 135–138. https://doi.org/10.1111/j.1574-695X.2002.tb00614.x [Google Scholar] [PubMed] [CrossRef]
Wittebole X, de Roock S, Opal SM (2014). A historical overview of bacteriophage therapy as an alternative to antibiotics for the treatment of bacterial pathogens. Virulence 5: 226–235. https://doi.org/10.4161/viru.25991 [Google Scholar] [PubMed] [CrossRef]
Woźnica WM, Bigos J, Łobocka MB (2015). Lysis of bacterial cells in the process of bacteriophage release--canonical and newly discovered mechanisms. Postepy Higieny I Medycyny Doswiadczalnej 69: 114–126. [Google Scholar] [PubMed]
Wright A, Hawkins CH, Änggård EE, Harper DR (2009). A controlled clinical trial of a therapeutic bacteriophage preparation in chronic otitis due to antibiotic-resistant Pseudomonas aeruginosa; A preliminary report of efficacy. Clinical Otolaryngology 34: 349–357. https://doi.org/10.1111/j.1749-4486.2009.01973.x [Google Scholar] [PubMed] [CrossRef]
Yehl K, Lemire S, Yang AC, Ando H, Mimee M, Torres MDT, de la Fuente-Nunez C, Lu TK (2019). Engineering phage host-range and suppressing bacterial resistance through phage tail fiber mutagenesis. Cell 179: 459–469. https://doi.org/10.1016/j.cell.2019.09.015 [Google Scholar] [PubMed] [CrossRef]
Yosef I, Manor M, Kiro R, Qimron U (2015). Temperate and lytic bacteriophages programmed to sensitize and kill antibiotic-resistant bacteria. Proceedings of the National Academy of Sciences of the United States of America 112: 7267–7272. https://doi.org/10.1073/pnas.1500107112 [Google Scholar] [PubMed] [CrossRef]
Young R, Gill JJ (2015). Phage therapy redux—What is to be done? Science 350: 1163–1164. https://doi.org/10.1126/science.aad6791 [Google Scholar] [PubMed] [CrossRef]
Cite This Article
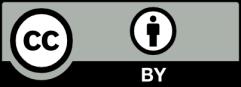