Open Access
ARTICLE
An Evaluation to the Performance of Evacuated Tube Solar Heaters for Arid and Hot Areas
1 Department of Chemistry, College of Science, University of Thi-Qar, Nasiriyah, Iraq
2 Centre for Sustainable Cooling, School of Chemical Engineering, University of Birmingham, Birmingham, UK
* Corresponding Author: Hameed B. Mahood. Email:
Energy Engineering 2023, 120(3), 587-596. https://doi.org/10.32604/ee.2023.025407
Received 09 July 2022; Accepted 02 December 2022; Issue published 03 January 2023
Abstract
Solar energy applications could be the best alternative to the conventional fuels for the purposes of domestic, water and space heating and some industries in the sunny, arid, and hot areas. In the present study, the performance of an evacuated tube solar heater for water heating for months of February and March was experimentally investigated. This was performed in a hot and arid area (Nasiriya City, South of Iraq). A solar heater with ten evacuated tube solar collectors with a capacity of 100 liter was used in the experiments. Each evacuated tube had a length of 1.8 m with an outside diameter of 8 cm. It was observed that for the two selected months, water temperature of the solar heater reached a maximum more than 70°C during sunny days with no heat extraction from the tank of the solar heater. Moreover, heat was extracted from the solar collector with four different flowrates 0.5, 0.75, 1, and 1.25 l/min, respectively. The results showed that temperature of the solar heater behaved differently from the static situation. When the heat extraction begun, there was a gradual and noticeable decrease in the water temperature of the heater. The observed decrease was slight with the lowest flowrate (0.25 l/m) and becomes sharp with the highest flowrate (1.25 l/min). However, water temperature of the solar heater remained higher than 40°C for the investigated flowrates except the case of 1.25 l/min. The results showed that evacuated tube solar heater can work efficiently in arid and hot areas in winter and spring seasons when the conditions of solar radiation are suitable.Keywords
It is certainly true that the suitable alternatives to the conventional sources of energy are renewable energies. These energies are sustainable, clean and also free gas emission. Solar energy is one of the most important types of renewables, it has been used widely around the world [1]. The significant component of all solar systems is the solar collector. This part of the system absorbs the incident solar radiation and simultaneously converts it into multiple useful applications. These applications can vary from generating electricity to providing thermal heat to many chemical and physical processes. The processes can include many industrial and domestic applications such as desalination, dairy industry, space heating, thermal distillation, domestic heating, and crop drying [2–6].
The used solar collectors to heat water for domestic and other purposes are mainly flat plate and evacuated tube solar collectors. Evacuated tube solar heaters or collectors are light and can easily be fixed on roofs; they are lighter than flat plates solar collectors, and they are advanced and highly efficient solar heaters. In other words, they are more efficient than flat plate solar heaters. These collectors are designed with low or no heat loss to the atmosphere, and this was performed due to vacuum tubes. Consequently, thermal heat would be captured internally, and this would increase the efficiency of the collector. The prevention of overall heat loss is ensured by surrounding the absorber with an evacuated annular space, this is performed by using two concentric glass tubes with a vacuum in between. Evacuated tubes are lighter and cheaper than flat plate solar collectors [1,7]. Zhang et al. [8] claimed that coating of solar absorbers and particularly evacuated tube solar heaters which made of Aluminum-Nitride is costly with a reflectivity higher than of the black body coating. Therefore, more studies are required to improve the performance of solar heaters and decreasing the reflectivity of incident solar radiation.
A large number of previous studies investigated the use of Carbon nanotube thin films to convert the incident solar radiation energy into other energy forms. Moreover, many other studies dealt with the use of phase change materials (PCMs) in the solar energy applications. These were particularly investigated their applications in solar storages and mostly focused on PCMs with melting points lower than 50°C [9–13]. Ali et al. [14] claimed that the use of PCM for the latent heat thermal energy is a practical method to sore thermal energy. However, they implied that the low thermal conductivity of PCM affects the charge-discharge process of the thermal storage. Therefore, they suggested a new design to overcome this difficulty. In their new design, a helical coil was used to maximize the contact surface between the heat transfer fluid (HTF) and the PCM. The helical coil was filled with PCM supported with porous media. The results showed that using porous media enhanced the performance of the investigated system. It was claimed that porous media can remarkably reduce the charge/discharge time of PCM up to 57%.
The improvement of evacuated tube collectors (ETCs) by enhancing the absorptive layer was investigated by Sayer et al. [1]. A multifunctional absorber was suggested utilizing carbon-based materials. Carbon Nano tube sheet coatings were used to enhance the absorption of incident solar radiation. Simultaneously, Phase change materials (PCMs) were also utilized to increase heat accumulation. The used PCM (Octadecane paraffin) had a melting point of 28°C and it is classified as non-toxic material with long term chemical stability. It was claimed [2] that the new approach enables the solar collectors for a more constant output even on a cloudy weather and can significantly prolong the output of heat at night. Consequently, their conclusion supported the enhancement of the performance of the evacuated tube solar collector. PCMs with melting points less than 50°C were also investigated for the applications of solar energy. Kürklü et al. [15] tried to develop a new type of solar collector with a short term investigation to its thermal performance. In their trial, a PCM (paraffin wax) with a melting range of 45°C–50°C was used. It was found that the inclusion of PCM was much advantageous over the conventional thermal storage with only water for the heat collection. Seeniraj et al. [16] studied numerically the performance improvement of latent heat thermal storage using multiple PCMs. The findings clearly showed appreciable enhancement in the melting of PCM and output temperature of the used heat transfer fluid.
Nano technology including fluids and materials were recently used in the applications of solar energy. Sheikholeslami et al. [17] investigated the use of Al2O3-water as an operating fluid to improve the thermal treatment of Linear Fresnel Reflector (LFR). Finite volume numerical method was applied for the prediction of the distribution of velocity, temperature and entropy, in addition to the measurements of thermal performance. The results demonstrated that the use of nano-powders of Al2O3 significantly improved the performance of the investigated solar application. To analyze the melting process of Paraffin in a heat storage, honeycomb configuration has been utilized by Sheikholeslami [18] to build a solid structure to enhance the thermal storage. The constructed body consisted of stainless steel and aluminum. The holes in the solid body were filled with a mixture of paraffin (RT82) and Al2O3 nanoparticles. A significant decrease in the melting time of paraffin by around 27.9% was obtained. Furthermore, a numerical study on the use of nanoparticles in the solar energy applications was performed by Sheikholeslami et al. [19]. In this study, a mixture of oil and CuO nanoparticles were applied to enhance the performance of a parabolic solar system. It was found that there is a noticeable increase in the outlet temperature and heat transfer coefficient of the investigated system. In the same direction, Sheikholeslami [20] has investigated the effect of ZnO nanoparticles mixed with paraffin on the performance of an air conditioning unit. It was concluded that the utility of the mixture of paraffin and ZnO nanoparticles led to an increase in the level of conduction and consequently decrease the time require for the melting of paraffin by 5.22%. Furthermore, dispersing hybrid nano-powders were tested numerically by Sheikholeslami [21] to improve a solar system included twenty mirrors to concentrate solar radiation to the absorber pipe of the solar system. In this study, fins with horseshoe shape were connected to the bottom of absorber and this was to enhance the performance of the tested solar system. Apparent increases in the thermal performance and convective factor around 0.18% and 31.98% respectively were achieved.
The performance of a flat-plate solar collector with a mixture of water and Al2O3 and CuO nanofluids as working fluids was investigated by Tong et al. [22]. The obtained experimental results demonstrated that thermal efficiency of the flat-plate solar collector could be improved by the use of Al2O3 and CuO nanofluids comparing with conventional solar collector with the use of water. The performance characteristics of a flat plate solar collector were investigated experimentally by the application of various nanofluids in the solar collector and comparing the sensitivity of its performance under different operating conditions [23]. The obtained experimental results illustrated that the efficiency of the flat plate solar collector reached the highest (87%) when a multi-walled carbon nanotube (MWCNT) nanofluid was applied and declined to the lowest (62%) when basefluid (water) was applied. Additionally, it was concluded that when nanofluids were used in the flat plate solar collector, high efficiency was observed, and the sensitivity of performance under the various operating parameters was found to be lower than in the case of water.
Cui et al. [24] aimed to improve and compare the thermal efficiency of evacuated tube solar collector by applying water, Fe3O4 nanofluid and Fe3O4 hybrid nanofluid as the working fluids. The maximum efficiency was shown when the hybrid nanofluid was used as the working fluid with an increase of 26.4% higher than cases of the water. Moreover, the results showed the thermal efficiency of evacuated tube solar collector was remarkably improved by using the hybrid nanofluids as working fluid comparing to the water and Fe3O4 nanofluids.
Solar energy applications were also used in agriculture [25–27]. Hassanien et al. [28] investigated the performance and viability of using an evacuated tube solar collector as a solar water heater assisted an electric heat pump to provide heat to a greenhouse. The study was performed in Kunming, China; two greenhouses were used in the investigation. Thermal energy required for the greenhouse was calculated beside the analysis of energy consumption. The results showed that temperature in the heated greenhouse was higher than the unheated one by 2°C–3°C with a reduction in the relative humidity by 10% in the heated greenhouse.
It is beneficial to evaluate the performance of solar collectors in areas have the suitable weather conditions for solar applications. Bargach et al. [29] studied the benefits of the utilization of solar flat plate collectors for heating agricultural greenhouses. In their study, they examined and introduced thermal results focusing on the effect of the heater system on the microclimate greenhouse with a comparison with an unheated greenhouse, it was observed that the heated greenhouse performed effectively than the unheated greenhouse.
It was observed that there are no sufficient studies tackling the evaluation of the performance of evacuated tube solar collectors in the area of the study. To this end, the present study aims to evaluate the performance of an evacuated tube solar collector in winter season in an area with arid weather conditions. This comes with the increasing interest in the use of this type of solar collectors for different heating applications.
To achieve this study, an evacuated tube solar heater with a capacity of 100 liter was installed in Nasiriya City (Latitude: 31.05799°, Longitude: 46.25726°) at the Department of Chemistry, College of Science, University of Thi-Qar, Iraq. The used evacuated solar heater consists of 10 evacuated tubes connected to a tank with a capacity of 100 liter. The experimental unit is shown in Fig. 1.
Figure 1: The used evacuated tube solar collector, the experiments were performed in a hot and arid area in the City of Nasiriya, South of Iraq
Each cylindrical evacuated tube of the investigated solar heater used in the experiments and shown in Fig. 1 has a length of 1.8 m. The height of the tank from the ground was 1.27 m, and the inclination angle of the evacuated tube was 45 degrees. The used evacuated tube comprises two concentric tubes; the external glass tube which had an outside diameter of 8 cm and a thickness of 0.15 cm while the internal tube (solar absorber) had an outside diameter of 5 cm. After the installation of the evacuated tube solar heater for experiments, experimental work was achieved in months of February and March; these months are in winter and spring seasons in the area of the study and it would be beneficial to examine the performance of this heater in this cold weather conditions of the year. To perform the experiments, the tank of the solar heater was filled with tap water before the sunshine. Then, the evacuated tube solar heater was left to 9 am for the thermal energy accumulation from the incident solar radiation. At 9 am, morning, water temperature of the solar collector was measured. After the temperature measurement, the tank of the solar heater was evacuated and re-filled. Then water temperature of the tank was monitored from 10 am to 5 pm (sunset) without heat extraction. To examine the effect of heat extraction on the temperature of the storage tank of the evacuated tube solar heater, four different heat extraction flowrates were selected for four days. They were respectively 0.5, 0.75, 1 and 1.25 l/min. The temperature measurements were recorded.
To investigate the profile of the temperature of the heater (evacuated tube solar heater), water temperature of the heater was monitored for many days at the area of the study (Nasiriya City, South of Iraq). Two days in February and two days in March were selected to do the experiments and to examine the behavior of water temperature of the selected solar heater. As it was mentioned in Section 2, the temperature of the water in the tank was firstly measured when the tank of the solar heater was full with tap water at 9 am in the morning. After the first measurements, the tank of the solar heater was evacuated, and filled again with tap water with temperatures between 16°C–20°C (depending on tap water temperature of the day of the experiment). The temperature increase of the water of the solar heater with the time progress was monitored for the selected days. The results are shown in Fig. 2 for the selected four days.
Figure 2: Temperature development with the time progress for the selected four days; the selected days were 24th and 28th of February and 4th, and 9th of March 2021. The experiments were performed in a hot and arid area in the City of Nasiriya, South of Iraq. Zero value on X-axes represents 9 am
It is apparent from Fig. 2 that temperature in the morning (9 am) was high for all considered days. This is expected and it is because the water received about two hours of insolation without heat extraction (water withdraw), and this allowed thermal energy to accumulate in the water of the tank of the evacuated tube solar heater triggering a temperature increase. Then, when the tank of the heater (evacuated tube solar heater) was filled again, the inlet temperatures of tap water were mostly between 16°C and 21°C. For the selected days, it was observed that there was a gradual increase in the temperature of the water of the solar heater in the tank of the evacuated tube solar heater. For the examined days, water temperature reached the maximum mostly around 2 pm (Fig. 2). The behaviour of the temperature might be as a consequence of the incident solar radiation which increases gradually from the morning time to reach the maximum around the midday. For more clarification, the hourly incident solar radiation for the investigated days in the area of the study (Nasiriya City, South of Iraq) is shown in Fig. 3 [30].
Figure 3: The incident solar radiation development with the time progress for the selected four days; the selected days were 24th and 28th of February and 4th, and 9th of March 2021. The experiments were performed in a hot and arid area in the City of Nasiriya, South of Iraq [30]
It is apparent from Fig. 3 that for all selected days, solar radiation increases from the morning time (9 am) and reaches the maximum at midday. Then, there is a continuous and regular decrease (Fig. 3) in the incident solar radiation to reach the minimum at 5 pm. Consequently, the heat accumulated in water of the evacuated tube solar heater triggered an increase in water temperature of the solar heater to reach the maximum at 2 pm.
Another interesting observation can be seen from Fig. 2 that temperatures became higher in days which were selected in March than the other days. This might be attributed to the increase in the incident solar radiation for these days as shown in Fig. 3 when moving from February to March. As demonstrated in Fig. 3, values of incident solar radiation in March are higher than values recorded in February. Fig. 2 shows clearly that the highest water temperature of the evacuated tube solar heater was in the 9th of March. However, the behaviour was identical for all considered days in February and March of the present study. Water temperature of the solar heater reached the highest in the day of 9th of March; it was about 65°C. The recorded temperatures introduce an indication that in the area of the study (Nasiriya City, South of Iraq), and in this period of the year (February and March), temperature can reach more than 60°C which is sufficient for the water heating for domestic and some industrial applications require low-rate of temperature. In the area of the study, the two selected months are approximately in winter and spring seasons. Behaviours shown in Fig. 2 are when there is no heat extraction from the tank of the evacuated tube solar heater. In other words, there was no water withdrawal from the tank of the solar heater. To clearly investigate the behaviour after the re-filling of the tank, temperatures of water when the thank of the evacuated tube solar heater was re-filled are shown in Fig. 4.
Figure 4: Temperature development with the time progress of the solar collector after the re-filling of the solar tank for the selected days; the selected days were 24th and 28th of February and 4th, and 9th of March 2021. The experiments were performed in a hot and arid area in the City of Nasiriya, South of Iraq. Zero value on X-axes represents 9 am
Fig. 4 illustrates that around 10 am in the morning time (1 on X-axes) when the tank of the solar collector was re-filled, the temperature was the lowest (16°C–21°C), and this was the temperature of the tap water when it received no insolation. Moreover, it is apparent from the figure that for all considered days, after the tank was re-filled with tap water, there was a gradual increase in the temperature from 10 am to 2 pm. This was as a result of thermal energy accumulation in water when insolation was absorbed. At 2 pm water temperature reaches the maximum, and the behaviour was identical for all days (Fig. 4). After 2 pm, the temperature remains approximately close to the maximum for about 2 h. Then there was a decrease in the water temperature of solar heater when the time is close to sunset (5 pm). This was anticipated due to the gradual decrease in the incident solar radiation on the evacuated tube solar heater. However, water remained hot with temperatures above 40°C.
For more elucidation and investigation to the behaviour of water temperature of the selected solar heater, the temperature development with the time progress was investigated when heat was extracted from the solar heater. This point is a practical and crucial point for the viability of the evaluated solar heater in the area of the study (Nasiriya City, South of Iraq). To perform this part of the experiments, four different heat extraction flowrates were selected; they were 0.5, 0.75, 1 and 1.25 l/min. The temperature development with the time progress was monitored for each case. Since the heat extraction processes were performed in four different days (15, 17, 19 and 22 of March), the difference in the incident solar radiation on the solar heater was neglected in the comparisons among temperature behaviours with the flow-rate variation. Temperature measurements of the selected days are shown in Fig. 5.
Figure 5: Temperature development with the time progress when heat was extracted from the solar tank, four different flow rates were selected; the heat extraction was performed in days of 16th, 17th, 22nd, and 23rd of March. The experiments were performed in a hot and arid area in the City of Nasiriya, South of Iraq
It can be seen from Fig. 5 that when heat was extracted from the solar heater, there is a gradual and continuous decrease in the water temperature with the continuous heat extraction for all flowrates. This can be explained that the used hot water (water taken from the tank) was substituted with tap water; this water has a temperature range of 17°C–20°C for the investigated days in the present study. As illustrated in the figure (Fig. 5) that when the heat was extracted, there was a sharp decrease in the temperature. This can be attributed to the cold water used for the substitution. This decrease became slighter due to the solar radiation absorption by the solar collectors and consequently thermal energy accumulated in the water of the assessed evacuated solar heater. However, with the flowrate of 0.5 l/min (the lowest flowrate), the reduction in the temperature was approximately small comparing to the noticeable decrease with the flowrate of 1.25 l/min (the highest). For the flowrate of 0.5 l/min and for eight hours continuous heat extraction, the temperature remains above forty degrees as clearly shown in Fig. 5. With the flowrate 0.75 l/min, the figure shows that temperature remains 6 h above forty. These observations are interesting since temperatures above forty are applicable and deployable for a wide range of applications. It is significant to emphasize that for the flowrate of 0.7 l/min, the initial temperature of the water in the tank was around 50°C while it was around 65°C for the rest flowrates of the present study, this because the weather was very cloudy in this day and consequently the incident solar radiation was negatively affected and that reflected on the temperature of water in the tank of solar heater. This gives an indication that in the cloudy weather, a decrease in the water temperature of the evacuated solar heater is expected in the area of the study. However, it remains around 35°C as demonstrated in Fig. 5 with the continuous flowrate of 0.75 l/min for eight hours.
This paper investigated the performance of an evacuated tube solar heater as a device to heat water in hot and arid areas. The investigation was carried out in Nasiriya City, South of Iraq (Latitude: 31.05799°, Longitude: 46.25726°) in months of February and March. To perform the investigation a 100-liter evacuated tube solar heater was used. This heater comprises ten evacuated tubes; each tube had a total length of 1.8 m with an outside diameter of 8 cm. These tubes are connected to a 100-liter tank.
• It was found that for the selected days, water temperature of the solar heater reached more than 70°C when there is no heat extraction from the heater.
• When heat was extracted from the solar heater with four heat extraction flowrates (0.5, 0.75, 1 and 1.25 l/min), it was observed that there was a decrease in the water temperature of the solar heater with the time progress. However, the temperature remained around 40°C with 0.5 and 0.75 l/min flowrates after eight hours of heat extraction.
• The decline in water temperature of the solar heater was the fastest whit the highest flowrate (1.25 l/min) compared to other investigated flowrates (0.5, 0.75, and 1).
• The results showed that the investigated evacuated tube solar heater can be utilised effectively and efficiently in hot and arid areas for the water heating purposes or other applications in winter and spring seasons.
• Studies on evaluation of solar energy applications and particularly evacuated tube and flat plate solar heaters in the area of the study and globally will be useful to increase the availability of practical and theoretical findings.
Acknowledgement: Authors acknowledge the cooperation with the Department of Chemistry, College of Science, University of Thi-Qar, Iraq.
Funding Statement: The authors received no specific funding for this study.
Conflicts of Interest: The authors declare that they have no conflicts of interest to report regarding the present study.
References
1. Sayer, A. H., Al-Hussaini, H., Campbell, A. N. (2016). New theoretical modelling of heat transfer in solar ponds. Solar Energy, 125(3), 207–218. DOI 10.1016/j.solener.2015.12.015. [Google Scholar] [CrossRef]
2. Sobhansarbandi, S., Martinez, P. M., Papadimitratos, A., Zakhidov, A., Hassanipour, F. (2017). Evacuated tube solar collector with multifunctional absorber layers. Solar Energy, 146, 342–350. DOI 10.1016/j.solener.2017.02.038. [Google Scholar] [CrossRef]
3. Papadimitratos, A., Sobhansarbandi, S., Pozdin, V., Zakhidov, A., Hassanipour, F. (2016). Evacuated tube solar collectors integrated with phase change materials. Solar Energy, 129, 10–19. DOI 10.1016/j.solener.2015.12.040. [Google Scholar] [CrossRef]
4. Sobhansarbandi, S., Atikol, U. (2015). Performance of flat-plate and compound parabolic concentrating solar collectors in underfloor heating systems. Journal of Solar Energy Engneering, 137(3), 034501. DOI 10.1115/1.4029229. [Google Scholar] [CrossRef]
5. Baqir, A. S., Mahood, H. B., Sayer, A. H. (2018). Temperature distribution measurements and modelling of a liquid-liquid-vapour spray column direct contact heat exchanger. Applied Thermal Engineering, 139(1), 542–551. DOI 10.1016/j.applthermaleng.2018.04.128. [Google Scholar] [CrossRef]
6. Sayer, A. H., Mahood, H. B. (2020). Improved thermal efficiency of salinity gradient solar pond by suppressing surface evaporation using an air layer. Energy Engineering, 117(6), 367–379. DOI 10.32604/EE.2020.011156. [Google Scholar] [CrossRef]
7. Sayer, A. H. (2017). An experimental and theoretical investigation of novel configurations of solar ponds for use in Iraq (PhD Dissertation). University of Surrey, Guildford (UK). [Google Scholar]
8. Zhang, M., Fang, S., Zakhidov, A. A., Lee, S. B., Aliev, A. E. et al. (2005). Strong, transparent, multifunctional, carbon nanotube sheets. Science, 309(5738), 1215–1219. DOI 10.1126/science.1115311. [Google Scholar] [CrossRef]
9. Seeniraj, R., Narasimhan, N. L. (2008). Performance enhancement of a solar dynamic LHTS module having both fins and multiple PCMs. Solar Energy, 82(6), 535–542. DOI 10.1016/j.solener.2007.11.001. [Google Scholar] [CrossRef]
10. Kürklü, A., Özmerzi, A., Bilgin, S. (2002). Thermal performance of a water-phase change material solar collector. Renewable Energy, 26(3), 391–399. DOI 10.1016/S0960-1481(01)00130-6. [Google Scholar] [CrossRef]
11. Wei, J., Jia, Y., Shu, Q., Gu, Z., Wang, K. et al. (2007). Double-walled carbon nanotube solar cells. Nano Letters, 7(8), 2317–2321. DOI 10.1021/nl070961c. [Google Scholar] [CrossRef]
12. Klinger, C., Patel, Y., Postma, H. W. C. (2012). Carbon nanotube solar cells. PLoS One, 7(5), e37806. DOI 10.1371/journal.pone.0037806. [Google Scholar] [CrossRef]
13. Sarı, A., Karaipekli, A. (2007). Thermal conductivity and latent heat thermal energy storage characteristics of paraffin/expanded graphite composite as phase change material. Applied Thermal Engineering, 27(8–9), 1271–1277. DOI 10.1016/j.applthermaleng.2006.11.004. [Google Scholar] [CrossRef]
14. Ali, R. K., Siavashi, M., Tahmasbi, M., Norouzi, A. M. (2022). Experimental analysis to improve charge/discharge of thermal energy storage in phase change materials using helical coil and porous metal foam. Journal of Energy Storage, 55, 105759. [Google Scholar]
15. Kürklü, A., Özmerzi, A., Bilgin, S. (2002). Thermal performance of a water-phase change material solar collector. Renewable Energy, 26(3), 391–399. DOI 10.1016/S0960-1481(01)00130-6. [Google Scholar] [CrossRef]
16. Seeniraj, R., Narasimhan, N. L. (2008). Performance enhancement of a solar dynamic LHTS module having both fins and multiple PCMs. Solar Energy, 82(6), 535–542. DOI 10.1016/j.solener.2007.11.001. [Google Scholar] [CrossRef]
17. Sheikholeslami, M., Ebrahimpour, Z. (2022). Thermal improvement of linear Fresnel solar system utilizing Al2O3-water nanofluid and multi-way twisted tape. International Journal of Thermal Sciences, 176, 107505. [Google Scholar]
18. Sheikholeslami, M. (2022). Analyzing melting process of paraffin through the heat storage with honeycomb configuration utilizing nanoparticles. Journal of Energy Storage, 52, 104954. [Google Scholar]
19. Sheikholeslami, M., Zafar, S., Jafaryarb, M. (2022). Hydrothermal analysis for a parabolic solar unit with wavy absorber pipe and nanofluid. Renewable Energy, 188, 922–932. [Google Scholar]
20. Sheikholeslami, M. (2022). Modeling investigation for energy storage system including mixture of paraffin and ZnO nano-powders considering porous media. Journal of Petroleum Science and Engineering, 219, 111066. [Google Scholar]
21. Sheikholeslami, M. (2022). Numerical investigation of solar system equipped with innovative turbulator and hybrid nanofluid. Solar Energy Materials and Solar Cells, 243, 111786. [Google Scholar]
22. Tong, Y., Lee, H., Kang, W., Cho, H. (2019). Energy and exergy comparison of a flat-plate solar collector using water, Al2O3 nanofluid, and CuO nanofluid. Applied Thermal Engineering, 159, 113959. [Google Scholar]
23. Tong, Y., Chi, X., Kang, W., Cho, H. (2020). Comparative investigation of efficiency sensitivity in a flat plate solar collector according to nanofluids. Applied Thermal Engineering, 174, 115346. [Google Scholar]
24. Cui, F., Liu, F., Tong, Y., Wang, S., Guo, W. et al. (2022). Energy and exergy assessment of evacuated tube solar collector using water, Fe3O4 nanofluid and Fe3O4/MWCNT hybrid nanofluid. Process Safety and Environmental Protection, 163, 236–243. [Google Scholar]
25. Panwar, N. L., Kaushik, S. C., Kothari, S. (2011). Solar greenhouse an option for renewable and sustainable farming. Renewable Sustainable Energy Reviews, 15(8), 3934–3945. [Google Scholar]
26. Sethi, V. P., Sharma, S. K. (2007). Survey of cooling technologies for worldwide agricultural greenhouse applications. Solar Energy, 81(12), 1447–1459. [Google Scholar]
27. Hassanien, R. E., Li, M., Dong, W. (2016). Advanced applications of solar energy in agricultural greenhouses. Renewable and Sustainable Energy Reviews, 54, 989–1001. DOI 10.1016/j.rser.2015.10.095. [Google Scholar] [CrossRef]
28. Hassanien, R. E., Ming, L., Tang, Y. (2018). The evacuated tube solar collector assisted heat pump for heating greenhouses. Energy and Buildings, 169(8), 305–318. DOI 10.1016/j.enbuild.2018.03.072. [Google Scholar] [CrossRef]
29. Bargach, M. N., Dahman, A. S., Boukallouch, M. (1999). A heating system using flat plate collectors to improve the inside greenhouse microclimate in Morocco. Renewable Energy, 18(3), 367–381. DOI 10.1016/S0960-1481(98)00803-9. [Google Scholar] [CrossRef]
30. https://power.larc.nasa.gov/data-access-viewer/. [Google Scholar]
Cite This Article
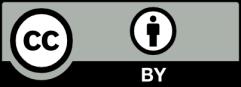