Open Access
ARTICLE
Effect of Oils on SBS Modified Asphalt: Rheological Characteristics and Oxidation Aging
1 Jiangxi Transportation Institute Co., Ltd., Nanchang, 330200, China
2 School of Civil Engineering and Architecture, East China Jiaotong University, Nanchang, 330013, China
3 School of Highway, Chang’an University, Xi’an, 710064, China
* Corresponding Author: Jing Xu. Email:
Journal of Renewable Materials 2023, 11(3), 1339-1351. https://doi.org/10.32604/jrm.2022.023270
Received 18 April 2022; Accepted 08 June 2022; Issue published 31 October 2022
Abstract
This study focuses on the effect of oils on rheology and oxidation aging of Styrene-Butadiene-Styrene modified asphalt (SBSMA) in the long term, after reducing one low-temperature Performance Grade (PG) of SBSMA by incorporating oils. Two oils, including corn-based bio-oil and re-refined engine oil bottom (REOB), were selected to enhance the low-temperature performance of SBSMA. All samples were subjected to Rolling Thin Film Oven (RTFO) aging and 20-h as well as 40-h Pressure Aging Vessel (PAV20 and PAV40) aging, prior to multiple stress creep recovery (MSCR), frequency sweep and Flourier transform infrared spectroscopy (FTIR) scanning. A good high-temperature performance of oil/SBS modified asphalt blends was reflected in MSCR and PG results, meanwhile non-recoverable creep compliance (Jnr) and recovery (R) were found to share a highly correlated relationship during aging progress. In addition, Glover–Rowe (G–R) parameter and phase angle master curves suggest that the improvement of cracking property mainly came from the softening effect of oils. Adding oils into SBSMA was observed to increase oxidation kinetics, but the blends with oils still exhibited better anti-oxidation aging than the base binder, mainly due to the SBS addition. Bio-oil exhibited an effect of relieving age hardening susceptibility of SBSMA.Keywords
Due to its excellent performance, Styrene-Butadiene-Styrene (SBS) modification technology could be one of the most wide-application and successful modification methods for asphalt binders in the paving industry [1]. Also, high content SBS modified asphalt is popular to be used in open-graded friction course (OGFC) to construct permeable asphalt pavement since its high viscosity can provide extra strength [2,3]. Nevertheless, a number of researches have proved that SBS modified asphalt (SBSMA) is prone to oxidative aging, with rheological response greatly changed and SBS degradation [4,5]. Even, short-term aging could cause an unneglected impact on performance [6]. The aging effect is typically regarded as benefiting the resistance to rutting of binders because of the increasing modulus [7]. Yet, the research found that aging of a certain level could exert a detrimental influence on high-temperature performance of SBSMA (increase of non-recoverable creep compliance, Jnr) [3]. This phenomenon could be explained that the reducing elasticity caused by SBS degradation is dominant at a certain aging level, instead of the age hardening of binders.
Cracking could be one of the main distresses facing asphalt pavement during the long-term service period. Thermal cracking can be considered as a series of cracking at various widths and lengths, which is often transverse to the roadway central line. The cracking leads to deterioration of structural capacity and penetration of water into the pavement, which further results in secondary impairment of the asphalt pavements [8]. It has been well accepted that low-temperature cracking of asphalt binders is predominantly dependent on stiffness and internal stress relaxation (the slope of the logarithm of stiffness and logarithm of time, m-value) [9,10]. Historically, the low-temperature performance of asphalt binders was once controlled by setting limiting stiffness.
Oxidative aging on asphalt binders inevitably leads to an obvious change to viscoelastic behavior and a progressive increase in complex modulus as well as a reduction in phase angle [11,12]. Complex modulus could be considered as corresponding to stiffness and phase angle to m-value [13]. The stiffer asphalt binder in the pavement would accumulate higher internal stress during heating and cooling cycles of day and night, while binders with lower m-value are not capable of dissipating the increasing internal stress, both of which make pavement prone to cracking. Similar to the aging effect, the application of Reclaimed Asphalt Pavement (RAP) and Reclaimed Asphalt Shingles (RAS) would also result in higher stiffness and brittleness of asphalt binders [14–17]. Improving cracking property always is the main challenge and target during the recycling process. Softer grade binder and recycling oils (rejuvenators) are often-used solutions to offset the increased stiffness and weakened m-value caused by aged binders. Nowadays, various types of oils have been used as recycling agents to recover the deteriorated performance of aged asphalt binders, and have been assessed from the perspectives of rheology, microscale property, and chemistry. A number of studies showed that the bio-based oils exhibited superior properties as a recycling agent regarding improving internal stress relaxation ability, reducing stiffness, long-term rejuvenating effectiveness, etc. [18–21]. Another challenge concerning the use of recycling oils is the oil dosage determination. The optimum oil dosage is normally determined based on the performance-recovery tests of asphalt binders with the extracted RAP/RAS binders, prior to the mixture test, and there are several methods to calculate the dosage: restore HT PG (high-temperature PG) to the target (e.g., HT PG of virgin binder); restore LT PG (low-temperature PG) to the target and checking HT PG; achieving −5°C for ΔTc; balanced performance design [22–25]. Notice that the performance-improving effect of adding oil mostly follows the linear relationship with the oil content, which can help give some insights into enhancing the performance of virgin binders when applying oils.
To improve the cracking performance of virgin binders can also turn to oils. Zhang et al. investigated adding oils to adjust asphalt binders to cold climate regions and found that oils could dramatically increase the fracture energy of binders and reduced the fracture temperature for asphalt mixtures, with low-temperature cracking property greatly enhanced [26]. Kilger et al. [27] evaluated the long-term-improving effect of oil in the polymer modified asphalt binders, and found that the rheological characteristics benefit from oils would maintain after double standard Pressure Aging Vessel aging, and then diminished. Additionally, Sadek confirmed the positive effect of improving anti-cracking performance by adding oils by conducting Semi-Circular Bending Illinois Flexibility Index test, meanwhile it also revealed that the cracking performance between mixtures and binders share certain correlating relationships [28].
However, the modifying effect among different oils and polymers could significantly vary, leading to distinctly different binder performance. The attempts to combine bio-based or REOB with SBS polymers to reach both improved high- and low- temperature performance are worthwhile. Further, the oil effect on rheological characteristics and oxidation during the aging process also needs to be well estimated. So, this study measured aging characteristics and rheological evolution of oil/SBS modified asphalt binders, with the employment of Flourier transform infrared spectroscopy (FTIR) scanning, multiple stress creep, and recovery test (MSCR), and frequency sweep. From there, indices of Glover–Rowe parameter (G–R), carbonyl area (CA), and PAV aging time to damage curves were obtained, and oils effect was further compared and analyzed. The specific objectives in this work are to:
1. Characterize the effect of oils on the rheological behavior of SBSMA,
2. Assess the effect of oils on the oxidation kinetics of aging on SBSMA and
3. Identify the effect of oils on the age hardening susceptibility of SBSMA.
2.1 Raw Materials and Preparation
In this work, one bio-oil, sourced from corn, and one re-refined engine oil bottom (REOB) were selected to reduce one low-temperature PG and improve resistance to cracking of SBSMA. These two selected oils are commercially available. The density of bio-oil and REOB is 1.03 and 0.96 g/cm3, respectively, while the corresponding viscosity at 60°C is 27.5 and 305 mPa·s.
The selected base binder PG58-28 is produced by SK company, Korea, meanwhile two SBS polymers of LG (made in Korea) and Dushanzi (made in China) were used to prepare SBS modified asphalt. In the paper, LG and Dushanzi SBS polymers are reported as SBS-1 and SBS-2, respectively. Both SBS-1 and 2 share the linear molecular structure, and the styrene-butadiene ratio of these two polymer modifiers is also identical (30/70). The molecular weight of SBS 1 and 2 is 10000 and 12000, respectively.
The specific preparation progress of oil/SBS modified asphalt is as follows: first, the base binder and SBS polymers were blended at high-shear (5000 RPM) for one and half hours at 180°C; then the elemental sulfurs (cross-linker) were added and additional low-speed shear (about 2000 RPM) blending continued for one hour at 180°C; last, the oil at the suitable content was added and the blends were mixed using a low-speed mixing of 700 RPM at 170°C for 10 min.
2.2 Materials Composition Determination
To achieve the purpose of reducing one low-temperature PG, the oil content for each sample was determined. Notice that the oil content for samples varies when SBS and oil are different. Table 1 presents the exact materials composition and continuous PG as well as descriptions for each sample. From Table 1, it can be known that adding oils at the determined content could successfully reduce one low-temperature PG for SBSMA, except Blend 1-REOB, though high-temperature PG was also decreased (from 82°C to 76°C).
The samples investigated in this study were subjected to Rolling Thin Film Oven (RTFO) aging in accordance with the AASHTO T240 to simulate the short-term aging during the mixing and compaction process. 20 and 40 h Pressure Aging Vessel (named as PAV20 and PAV40, respectively) aging were employed on the samples to simulate the long-term aging that occurred in service life, following AASHTO R28.
2.4 Master Curves and Glover–Rowe Parameter
Based on the time-temperature superposition principle (TTSP), frequency sweeps at various temperatures can be used to construct rheology mater curves. The frequency sweeps of each sample between 1 and 30 rad/s were carried out at 0°C, 10°C, 15°C, 25°C, 35°C, 50°C, and 65°C. The small parallel plate (8 mm) and 2 mm gap were used at frequency sweep when temperature ranged from 0°C to 35°C, meanwhile 25 mm parallel plate and 1 mm gap were employed when the temperature was 50°C and 65°C.
Before frequency sweep, strain sweeps were performed to determine the linear viscoelastic region for asphalt binders at different temperatures and aging states, where complex shear modulus remains above 95% of its initial value [29]. Then, the frequency sweep was conducted within the strain limit to ensure the linear viscoelastic response for all samples at each aging state.
Rheology master curves were constructed by horizontally shifting the complex shear modulus and phase angle at all combination temperatures to the reference temperature of 25°C, following the Christensen-Anderson-Marasteanu (CAM) model [30]. The shifting factor, obtained by CAM model, to construct complex modulus and phase angle master curves is same.
Glover–Rowe parameter (G–R) was found to be highly correlated with the ductility of asphalt binders, and has been regarded as a good indicator of cracking resistance. The asphalt binder ductility values of 3 and 5 cm could correspond to two binder failure types: cracking onset and significant cracking, meanwhile these ductility thresholds are highly correlated with G–R parameter equal to 180 and 600 kPa, respectively [9]. So, master curves at the reference temperature of 15°C (seen in Fig. 1) were also developed to calculate the G–R parameter, according to Eq. (1).
Figure 1: Representative shifted master curves of complex shear modulus and phase angle at the reference temperature of 15°C
where complex shear modulus (G*) and phase angle (δ) were selected from the corresponding master curves at 15°C and 0.005 rad/s.
2.5 Multiple Stress Creep and Recovery Test (MSCR)
MSCR test has a good simulation of the load of moving vehicles on asphalt pavement, meanwhile the indices obtained from MSCR have been proved to have a good correlation with the high-temperature anti-rutting performance of asphalt binders [31]. The MSCR tests were performed at 64°C following AASHTO T 350. Two replicates of each sample were tested. From there, indices of non-recoverable creep compliance (Jnr) and recovery (R) were obtained. In this paper, Jnr and R at 3.2 KPa were used to further characterize the high-temperature performance (reported as Jnr3.2 and R3.2, respectively), since the standard of AASHTO MP 19 categorizes high-temperature performance based on Jnr3.2.
The asphalt binder in pavement inevitably gets oxidatively aged during the service period, with some oxygen-containing function groups generated. The amount of carbonyl has been proved to be linearly correlated with asphalt aging time and regarded as an indicator of binder oxidation level [32]. In this study, a Bruker TENSOR was used to collect the spectra of the samples with Attenuated Total Reflection (ATR) Fourier Transform infrared spectroscopy (ATR-FTIR) method. Each spectrum was obtained by an average of 32 spectra at 2 cm-1 resolution with the wavenumber range of 400–4000 cm−1. Before quantitative analysis on the spectrum, baseline correction and band normalization were conducted at Thermo Scientific OMNICTM software. As depicted in Fig. 2, carbonyl area (CA) is defined as the area, surrounded by the spectrum and the drawn baseline (highlighted in red in the figure) at the fixed wavelength range (from 1660 to 1753 cm−1), and the change of CA to indicate the oxidation level of asphalt binders [14]. The detailed calculation of CA was carried out with MATLAB software for the sake of avoiding manual errors.
Figure 2: Typical FTIR spectra of asphalt binders and the corresponding carbonyl area
3.1 High-Temperature Rheological Performance
Based on high-temperature PG (Table 1), it still remains at a high level of high-temperature performance of SBSMA with oils. Moreover, the resistance to permanent deformation at high temperature was further measured by R3.2 and Jnr3.2 of MSCR tests, with results shown in Fig. 3, meanwhile the values of R3.2 and Jnr3.2 of blends at RTFO aging state are highlighted in the figure, on which AASHTO MP 19-10 determines binders’ PG plus grade.
Figure 3: MSCR R3.2 and Jnr3.2 results at 64°C for oil/SBS modified asphalt. (a): R3.2 and (b): Jnr3.2
Clearly, the softening effect of adding bio-oil or REOB was reflected by both reduced R3.2 and increased Jnr3.2. This softening effect by adding oils seems greater on Blend 1 than on Blend 2, since more changes occurred on R3.2 and Jnr3.2 of Blend 1, before and after adding oils. Notice that, except Blend 2-BO (Jnr3.2 of 0.57), Jnr3.2 of all blends at RTFO would not exceed 0.5 kPa−1, suggesting those blends can be classified into the highest level of “E” (MSCR PG64 E) based on AASHTO MP 19-10, revealing that adding oils are capable of improving low-temperature PG without compromising much high-temperature performance of binders. In addition, increasing R3.2 and reducing Jnr3.2 as aging also confirmed that aging could benefit the high-temperature performance of all blends.
From Fig. 4, it was found that Jnr3.2 vs. R3.2 could be plotted into a curve combining all data points at all aging conditions. The logarithmic trend line superimposed for the curve shows that R3.2 and Jnr3.2 are highly correlated (R2 = 0.91~0.98), and also means the evolution of R3.2 and Jnr3.2 is highly consistent during the aging process. This also indicates that the specific trendline of R3.2 and Jnr3.2 (fitting equation) may mostly depend on the type of modifier while adding oils and the aging effect only change the point location at the curve.
Figure 4: The correlating curves for Jnr3.2 vs. R3.2 at 64°C
3.2 Phase Angle Master Curves and Black Space Diagrams
Asphalt binder is a typical viscoelastic material, whose strain normally lags behind the applied strain. This strain-stress characteristic can be depicted by phase angle, ranging from 0° to 90°. So, oils’ effect on the rheology of SBSMA in the long term was investigated with the employment phase angle master curves, with results shown in Fig. 5.
Figure 5: The representative phase angle master curves for oil/SBS modified asphalt binders at each aging state at the reference temperature of 25°C, (a) Unaged, (b) RTFO, (c) PAV20 and (d) PAV40
From Fig. 5a, it is easily acknowledged that the greatest gap among phase angle master curves is the difference between base binder and blends. The base binder exhibited an obviously higher curve than blends at the low-frequency range (high temperatures), which mainly is attributed to the SBS introduction that ensures the higher elastic response and provides better anti-rutting performance. On the other hand, oils’ effect on the rheology of SBSMA is not that obvious, since the curves of blends with and without oils nearly overlapped, especially Blend 2 and Blend 2-REOB. The phase angle master curve of Blend 2-BO is slightly higher than the other two blends, and this is because of the better softening effect of bio-oil than it of REOB. As a result, at the point of the phase angle master curve, adding oils would not greatly influence the physical cross-linking of polystyrene blocks and polymer elastic networks, so the viscoelastic response of SBSMA would not be influenced significantly.
Figs. 5b–5d depict the phase angle master curves of blends at various aging states. Research have proved that SBS polymers in binder degrade as aging time [3]. So, the polymer phase domination, where phase angle remains relatively stable, came to disappear as aging severity. Meanwhile adding oils into SBSMA would not influence this trend, and the curves for blends remained very close to each other during the whole aging process.
To clearly identify the effect of oils on phase angle master curves of blends, the master curves were plotted separately according to the binder type, shown in Fig. 6. It also confirms that oxidation aging not only had an effect of reducing phase angle but could reshape the curve. The phase angle plateau at low frequencies of blends started to come smaller after RTFO aging and disappeared at PAV40. Adding oils into SBSMA would not change this trend, and all blends shared a very similar shape for their phase angle master curves.
Figure 6: The typical evolution of phase angle master curve between base binder and blends as aging. Blend 2 series are used to represent all blend samples
To further analyze the long-term effect of oils on resistance to cracking, Fig. 7 shows the G–R results at black space. There are two damage curves in red representing cracking onset and significant cracking, respectively (G–R parameter equals 180 and 600 kPa). From the black space diagram with damage curves, the reducing complex modulus and growing phase angle could help data points move away from the damage curves. Both bio-oil and REOB have a good effect of decreasing the complex modulus on Blend 1 and Blend 2. Yet, from the black diagrams, it seems that only the phase angle of Blend 1-BO was obviously increased, while other samples did not witness an obvious change after adding oils.
Figure 7: Glover-Rower results for oil/SBS modified asphalt binders in black space diagrams. Four data points in each curve represent four aging conditions: unaged, RFTO, PAV20, and PAV40
On the other hand, aging had an opposite effect on resistance to cracking, as compared with oil inclusion. The curves of all samples moved towards the top-left corner (toward the damage curve) as aging severity increased. After 40-h PAV aging, Blend 1-BO and Blend 1-REOB reached the cracking onset damage curve, and even Blend 1 reached the significant cracking damage curve. The samples of Blend 2 series had a relatively better cracking performance, only Bend 2 has reached the cracking onset damage curve. Overall, blends with oils perform better in terms of resistance to cracking at intermediate temperature, especially adding bio-oils. It also should be noted that oils (low-temperature modifiers) cannot restore the G-R parameter of SBSMA to the base binder’s level.
A strong linear correlation relationship between CA and PAV aging time can be seen in Fig. 8 (R2: 0.96–1). And, it should be noted that a small difference in slope of CA vs. PAV aging time may reveal a distinct gap in the oxidation kinetics among asphalt binders since the CA change upon the whole aging process is also very limited.
Figure 8: CA results of blends with and without oils at various aging levels. 0 PAV aging hour corresponds to RTFO aging state
All binders, including blends with and without oils as well as the base binder, kept stable oxidation kinetics during the long-term aging, with oxidation products with carbonyl bonds continuously generating. It also can be known that adding oils into SBSMA could impact the increase rate of CA during aging process (slope of the curve). Bio-oil was observed to have an effect of increase oxidation kinetics, since the slope of both Blend 1-BO and Blend 2-BO increased, compared to blends without oils (from 0.0211 to 0.0267 and from 0.0233 to 0.0263, respectively). Additionally, Blend 1-BO and Blend 2-BO remained at a higher position than other samples. This is due to the addition of bio-oils since the chemical composition of bio-oils has been proven to contain abundant Aliphatic esters (carbonyl bonds). The addition of bio-oils into binders also means introducing extra carbonyl bonds which are not generated by oxidation aging. As a result, CA values of blends with bio-oils are higher than blends without bio-oils and base binder. Whereas, the effect of adding REOB on oxidation kinetics is uncertain, which also depends on the type of SBS polymer, due to the opposite effect of adding REOB on Blend 1 (slope went down) and Blend 2 (slope went up). Additionally, it should be noticed that all blends, regardless of adding oil or not, exhibited a smaller slope than the base binder, which may indicate that blends share a better anti-aging performance, from the perspective of oxidation.
Oxidation in asphalt binders results in oxygen-containing substance accumulation and the resulting age hardening. Fig. 9 presents curves of the logarithm of the G-R parameter vs. CA of each sample, and strong linear correlations have been witnessed (R2 ranging from 0.86 to 0.97). The average change rate of the logarithm of the G-R parameter vs. CA (the slope of the curve) has been defined as age hardening susceptibility parameter [14]. This work also turns to the hardening susceptibility parameter to characterize the effect of oxidative aging on binder rheological properties and compare the effect of adding oils.
Figure 9: The correlating relationship between G–R and CA parameters as PAV aging
For both Blend 1 and Blend 2, adding oils would not greatly change hardening susceptibility, with the slope slightly growing and reducing. Nevertheless, it needs to be recognized that REOB and bio-oil have an opposite impact regarding influencing hardening susceptibility. REOB has the effect of increasing the slope, while bio-oil can slow down the age-hardening rate (the increase rate of Log G–R vs. CA). Additionally, all blends perform better than the base binder in terms of hardening susceptibility, with a lower slope than it of base binder, though there is an obvious gap in the G-R parameter between blends and the base binder.
To further evaluate the resistance to cracking as aging, PAV aging times to reach G–R damage curves for each sample have been calculated, based on oxidation kinetics (Fig. 8) and the relationship between CA and G–R (hardening susceptibility, Fig. 9).
From Fig. 10, the PAV length to reach G–R damage curves obviously increased after adding oils. For both Blend 1 and Blend 2, adding bio-oil has a better effect than REOB, because of a higher PAV aging length to cracking. Meanwhile, SBS type could play a more important role in resistance to cracking, because the PAV aging time to G–R damage curves of Blend 2 series is obviously longer than it of Blend 1 series.
Figure 10: The PAV aging lengths for all samples to reach G–R damage onset and significant cracking curves
The main objective of this work is to investigate the long-term effect of oils on SBSMA, from the aspects of rheology, oxidation kinetics and resistance to cracking, upon which indices, including phase angle master curves, CA, G-R parameters, hardening susceptibility, and PAV aging time to cracking, were employed to analyze the effect of oils. Two typical oils, bio-oil and REOB, were selected to be added into SBSMA to prepare oil and SBS modified asphalt. The summarized conclusions are as follows:
• MSCR results confirm that adding oils at desired content would not greatly weaken the high-temperature performance of SBSMA, with all blends classified as MSCR PG64E. During the aging process, the evolutions of Jnr and R were highly related, where the specific trendline of Jnr and R was controlled by the type of SBS, while the aging effect and adding oils only change the data positions at the curve.
• From the phase angle master curves, adding oils into SBSMA would not obviously change rheological characteristics, with only phase angle values slightly changed. At the point of G–R parameters, the improvement of resistance to cracking after adding oils mainly comes from the softening effect of oils (reducing complex modulus), because the increase of phase angle is very limited.
• Adding oils was found to have a certain effect of increasing oxidation kinetics, but SBSMA containing oils still exhibited a lower slope of CA vs. aging time than the base binder. This indicates that blends perform better than the base binder in terms of anti-aging, at the point of chemical oxidation.
• The hardening susceptibility results show that the bio-oil has a relieving effect, but adding REOB is the opposite. Additionally, the index of PAV aging time to G–R damage curves results show that blends with bio-oil exhibit longer cracking life than other blends.
Funding Statement:This work is jointly found by Science and Technology Research Project of Jiangxi Provincial Department of Education (Grant Nos. GJJ210645, GJJ210623), Key R&D Projects of Xinjiang Uygur Autonomous Region (Grant No. 2021B01005) and Science and Technology Project of Jiangxi Provincial Department of Transportation (Grant Nos. 2020Z0002, 2018Q0030). The authors would also like to appreciate the financial support from China Scholarship Council and Chang’an University. The special thanks would go to Dr. Yuan Zhang and Dr. Hui Chen, both of who provide the professional training and help.
Conflicts of Interest: The authors declare that they have no conflicts of interest to report regarding the present study.
References
1. Chen, M., Geng, J., Xia, C., He, L., Liu, Z. (2021). A review of phase structure of SBS modified asphalt: Affecting factors, analytical methods, phase models and improvements. Construction and Building Materials, 294, 123610. DOI 10.1016/j.conbuildmat.2021.123610. [Google Scholar] [CrossRef]
2. Cai, J., Song, C., Zhou, B., Tian, Y., Li, R. et al. (2019). Investigation on high-viscosity asphalt binder for permeable asphalt concrete with waste materials. Journal of Cleaner Production, 228, 40–51. DOI 10.1016/j.jclepro.2019.04.010. [Google Scholar] [CrossRef]
3. Yan, C., Huang, W., Lin, P., Zhang, Y., Lv, Q. (2019). Chemical and rheological evaluation of aging properties of high content SBS polymer modified asphalt. Fuel, 252, 417–426. DOI 10.1016/j.fuel.2019.04.022. [Google Scholar] [CrossRef]
4. Sun, G., Li, B., Sun, D., Yu, F., Hu, M. (2021). Chemo-rheological and morphology evolution of polymer modified bitumens under thermal oxidative and all-weather aging. Fuel, 285, 118989. DOI 10.1016/j.fuel.2020.118989. [Google Scholar] [CrossRef]
5. Han, X., Mao, S., Xu, S., Cao, Z., Zeng, S. et al. (2022). Development of novel composite rejuvenators for efficient recycling of aged SBS modified bitumen. Fuel, 318, 123715. DOI 10.1016/j.fuel.2022.123715. [Google Scholar] [CrossRef]
6. Yan, C., Xiao, F., Huang, W. (2018). Short-term aging of high-content SBSMA. Journal of Materials in Civil Engineering, 30(8), 10. DOI 10.1061/(ASCE)MT.1943-5533.0002382. [Google Scholar] [CrossRef]
7. Lesueur, D. (2009). The colloidal structure of bitumen: Consequences on the rheology and on the mechanisms of bitumen modification. Advances in Colloid & Interface Science, 145(1–2), 42–82. DOI 10.1016/j.cis.2008.08.011. [Google Scholar] [CrossRef]
8. Zhang, J., Tan, H., Pei, J., Qu, T., Liu, W. (2019). Evaluating crack resistance of asphalt mixture based on essential fracture energy and fracture toughness. International Journal of Geomechanics, 19(4), 6019005. DOI 10.1061/(ASCE)GM.1943-5622.0001390. [Google Scholar] [CrossRef]
9. Johnson, K., Hesp, S. (2014). Effect of waste engine oil residue on quality and durability of SHRP materials reference library binders. Transportation Research Record: Journal of the Transportation Research Board, 2444(1), 102–109. DOI 10.3141/2444-12. [Google Scholar] [CrossRef]
10. Kaseer, F., Yin, F., Arámbula-Mercado, E., Martin, A. (2017). Stiffness characterization of asphalt mixtures with high recycled materials contents and recycling agents. Transportation Research Record: Journal of the Transportation Research Board, 2633(1), 58–68. DOI 10.3141/2633-08. [Google Scholar] [CrossRef]
11. Ren, S., Liu, X., Lin, P., Jing, R., Erkens, S. (2022). Toward the long-term aging influence and novel reaction kinetics models of bitumen. International Journal of Pavement Engineering, 21(9), 1–16. DOI 10.1080/10298436.2021.2024188. [Google Scholar] [CrossRef]
12. Ren, S., Liu, X., Li, M., Fan, W., Xu, J. et al. (2020). Experimental characterization of viscoelastic behaviors, microstructure and thermal stability of CR/SBS modified asphalt with TOR. Construction and Building Materials, 261, 120524. DOI 10.1016/j.conbuildmat.2020.120524. [Google Scholar] [CrossRef]
13. Moraes, R. (2014). Investigation of mineral filler effects on the aging process of asphalt mastics. (Doctoral Dissertation). The University of Wisconsin-Madison, Madison. [Google Scholar]
14. Yin, F., Kaseer, F., Arámbula-Mercado, E., Martin, A. (2017). Characterising the long-term rejuvenating effectiveness of recycling agents on asphalt blends and mixtures with high RAP and RAS contents. Road Materials Pavement Design, 18(sup4), 273–292. DOI 10.1080/14680629.2017.1389074. [Google Scholar] [CrossRef]
15. Kaseer, F., Martin, A., Arámbula-Mercado, E. (2019). Use of recycling agents in asphalt mixtures with high recycled materials contents in the United States: A literature review. Construction and Building Materials, 211, 974–987. DOI 10.1016/j.conbuildmat.2019.03.286. [Google Scholar] [CrossRef]
16. Hou, X., Xiao, F., Wang, J., Amirkhanian, S. (2018). Identification of asphalt aging characterization by spectrophotometry technique. Fuel, 226, 230–239. DOI 10.1016/j.fuel.2018.04.030. [Google Scholar] [CrossRef]
17. Cai, J., Song, C., Gong, X., Zhang, J., Pei, J. et al. (2022). Gradation of limestone-aggregate-based porous asphalt concrete under dynamic crushing test: Composition, fragmentation and stability. Construction and Building Materials, 323, 126532. DOI 10.1016/j.conbuildmat.2022.126532. [Google Scholar] [CrossRef]
18. Cai, X., Zhang, J., Xu, G., Gong, M., Chen, X. et al. (2019). Internal aging indexes to characterize the aging behavior of two bio-rejuvenated asphalts. Journal of Cleaner Production, 220, 1231–1238. DOI 10.1016/j.jclepro.2019.02.203. [Google Scholar] [CrossRef]
19. Zadshir, M., Oldham, D., Hosseinnezhad, S., Fini, E. (2018). Investigating bio rejuvenation mechanisms in asphalt binder via laboratory experiments and molecular dynamics simulation. Construction and Building Materials, 190, 392–402. DOI 10.1016/j.conbuildmat.2018.09.137. [Google Scholar] [CrossRef]
20. Ren, S., Liu, X., Lin, P., Erkens, S., Gao, Y. (2022). Chemical characterizations and molecular dynamics simulations on different rejuvenators for aged bitumen recycling. Fuel, 324, 124550. DOI 10.1016/j.fuel.2022.124550. [Google Scholar] [CrossRef]
21. Zhang, Y., Bahia, H. (2020). Effects of recycling agents (RAs) on rutting resistance and moisture susceptibility of mixtures with high RAP/RAS content. Construction and Building Materials, 270, 121369. DOI 10.1016/j.conbuildmat.2020.121369. [Google Scholar] [CrossRef]
22. Mensching, D., Andriescu, A., DeCarlo, C., Li, X., Youtcheff, J. (2017). Effect of extended aging on asphalt materials containing re-refined engine oil bottoms. Transportation Research Record: Journal of the Transportation Research Board, 2632(1), 60–69. DOI 10.3141/2632-07. [Google Scholar] [CrossRef]
23. Jacobs, G., Margaritis, A., Hernando, D., He, L., Bergh, W. (2021). Influence of soft binder and rejuvenator on the mechanical and chemical properties of bituminous binders. Journal of Cleaner Production, 287, 125596. DOI 10.1016/j.jclepro.2020.125596. [Google Scholar] [CrossRef]
24. Tran, N., Taylor, A., Turner, P., Holmes, C., Porot, L. (2017). Effect of rejuvenator on performance characteristics of high RAP mixture. Road Materials and Pavement Design, 18(sup1), 183–208. DOI 10.1080/14680629.2016.1266757. [Google Scholar] [CrossRef]
25. Zaumanis, M., Mallick, R., Frank, R. (2014). Determining optimum rejuvenator dose for asphalt recycling based on Superpave performance grade specifications. Construction and Building Materials, 69, 159–166. DOI 10.1016/j.conbuildmat.2014.07.035. [Google Scholar] [CrossRef]
26. Zhang, L., Bahia, H., Tan, Y. (2015). Effect of bio-based and refined waste oil modifiers on low temperature performance of asphalt binders. Construction and Building Materials, 86, 95–100. DOI 10.1016/j.conbuildmat.2015.03.106. [Google Scholar] [CrossRef]
27. Kilger, A., Swiert, D., Bahia, H. (2019). Long-term aging performance analysis of oil modified asphalt binders. Transportation Research Record: Journal of the Transportation Research Board, 2673(12), 404–412. DOI 10.1177/0361198119847982. [Google Scholar] [CrossRef]
28. Sadek, H., Rahaman, M., Lemke, Z., Bahia, H., Swiertz, D. (2020). Effect of low-temperature modifiers on HMA mixture aging and cracking resistance. Construction and Building Materials, 237, 117456. DOI 10.1016/j.conbuildmat.2019.117456. [Google Scholar] [CrossRef]
29. Yang, K., Li, R., Castorena, C., Underwood, B. (2022). Correlation of asphalt binder linear viscoelasticity (LVE) parameters and the ranking consistency related to fatigue cracking resistance. Construction and Building Materials, 322, 126450. DOI 10.1016/j.conbuildmat.2022.126450. [Google Scholar] [CrossRef]
30. Ling, M., Luo, X., Gu, F., Lytton, R. (2017). Time-temperature-aging-depth shift functions for dynamic modulus master curves of asphalt mixtures. Construction and Building Materials, 157, 943–951. DOI 10.1016/j.conbuildmat.2017.09.156. [Google Scholar] [CrossRef]
31. Yan, C., Zhang, Y., Bahia, H. (2022). Predicting rutting performance of asphalt mixture from binder properties and mixture design variables. Road Materials and Pavement Design, 23(1), 62–79. DOI 10.1080/14680629.2020.1820890. [Google Scholar] [CrossRef]
32. Menapa, I., Yiming, W., Masad, E. (2017). Chemical analysis of surface and bulk of asphalt binders aged with accelerated weathering tester and standard aging methods. Fuel, 202, 366–379. DOI 10.1016/j.fuel.2017.04.042. [Google Scholar] [CrossRef]
Cite This Article
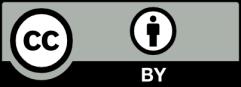