Open Access
ARTICLE
Design and Development of Composite Plywood that Integrates Fire Resistance, Water Resistance and Wear Resistance
1
College of Materials Science and Engineering, Nanjing Forestry University, Nanjing, 210037, China
2
College of Civil Engineering, Nanjing Forestry University, Nanjing, 210037, China
3
Jiangsu Shancong Wood Co., Ltd., Lianyungang, 222505, China
* Corresponding Author: Zheng Wang. Email:
(This article belongs to the Special Issue: Bio-Composite Materials and Structures-2021)
Journal of Renewable Materials 2023, 11(5), 2333-2344. https://doi.org/10.32604/jrm.2023.026137
Received 18 August 2022; Accepted 09 September 2022; Issue published 13 February 2023
Abstract
In order to improve the fire resistance, water resistance and wear resistance of ordinary plywood products in the wood processing industry, three composite structures of plywood products S1, S2 and S3 were designed in this paper, and a reasonable production process was proposed. Through the physical and mechanical properties and fire resistance testing and technical and economic analysis, the applicability of composite plywood was evaluated. The results of the study showed that the physical mechanics of the three kinds of composite structure plywood met the standard requirements, and their fire resistance was far better than that of ordinary plywood. Among them, the S1 structural board had the best overall physical and mechanical properties. The S3 structural board showed the best fire resistance, which was about 1.9 times more than that of ordinary plywood, and the added cost was the lowest. The thin cork board added to the S2 structural board had poor fire performance since the air in the cork board cavities had a certain combustion-supporting effect, which inhibited the fire resistance of high-pressure laminate (HPL) layer. Moreover, the additional cost of the S2 board was the highest, and its comprehensive performance was the worst. The S3 structural plywood product composed of HPL fireproof board with a thickness of about 1 mm in the surface layer and ordinary plywood with a thickness of about 12 mm in the core layer was the most cost-effective product, which could meet the needs of various fields such as construction, home furnishing, decoration and transportation.Keywords
Plywood is one of the commonly used materials for furniture. It is one of the three major panels of wood-based panels. It can also be used for aircraft, ships, trains, automobiles, buildings, packaging boxes, and indoor and outdoor decoration. Plywood can improve the utilization rate of wood and is one of the main ways to consume relatively less wood than other wooden materials [1,2]. In China, plywood is the most widely used wood-based panel product with the fastest output growth, which has reached an average annual growth rate of 15% in the past decade. In 2019, Chinese plywood production reached 180 million m3, accounting for more than 60% of global plywood production [3,4]. At the same time, plywood consumption and international trade also ranked first in the world [5,6]. Compared with solid wood, plywood is characterized by a stable structure, good physical and mechanical properties, and small variability [7–9]. There are many dynamic and static methods used in the inspection of plywood [10–12].
However, it is difficult for ordinary plywood products to ensure flatness, high fire, water, and wear resistance at the same time, which greatly restricts the use and market sales of the board. The main reason is that the wood material on the surface of such a board shrinks, swells and burns very easily if exposed to water and fire. In addition, the use of urea-formaldehyde resin glue makes its water-proof performance poor, resulting in dimensional deformation of the board, decay and mildew, and even direct damage. In the production and application of solid wood panels, the method of impregnating chemicals is often used to modify and reduce the dry shrinkage and wet expansion of wood [13–15]. However, due to the existence of glue joints in the plywood, the simple dipping treatment cannot meet the expected requirements. Even if the plywood products are veneered in the later stage to increase their fire resistance, wear resistance and surface flatness, the service life of the plywood will be reduced due to poor surface bonding, low bonding strength, and high labour costs. Generally, conventional flame-retardant plywood achieves its fire resistance through the process of impregnating flame retardants, which is relatively complicated and expensive [16–18]. In recent years, researchers have been able to effectively enhance the fire resistance of plywood by mixing flame retardants with adhesives to form a new flame-retardant system, or by applying fire retardant coatings to the surface of the plywood [19–22]. This method can also be used in veneer plywood to enhance its fire resistance, which affects its surface finishing performance to a certain extent and increase production investment. The surface wear resistance of plywood is usually achieved by attaching cladding materials or resin coating on the surface [23–26]. Due to changes in the processing conditions of plywood products (such as hot-pressing parameters, substrate types, etc.), sometimes it is easy to reduce the wear resistance of the surface layer, thereby affecting its service life [27,28].
In this regard, there is an urgent need for a veneer composite plywood that simultaneously considers fire resistance, wear resistance and surface flatness to better meet the urgent market demand for multi-functional plywood and to improve the economic benefits of enterprises and society. Therefore, in this paper, three kinds of composite plywood that were fireproof, waterproof and wear-resistant were designed, their manufacturing process and performance were evaluated, and the obtained results were analyzed and discussed.
2 Structural Design and Production Process of Composite Plywood
In this study, three kinds of composite plywood products integrating fireproof, waterproof and wear-resistant functions were designed, and their specifications were approximately 2440 mm × 1220 mm × 16 mm. The specimens were labelled as S1, S2 and S3 (Table 1). The structure of each product consisted of a core layer, a levelling layer and a surface layer, as shown in Fig. 1. The core layer was ordinary plywood with a thickness of about 12 mm. Ordinary plywood refers to plywood other than special plywood and structural plywood, including four types: Class I, Class II, Class III and Class IV [29]. The levelling layer was medium-density fiberboard (MDF) with a thickness of about 1 mm, which played the role of veneer levelling. The fireproof layer was a Portuguese cork board with a thickness of about 2 mm, which was responsible for fire protection and heat preservation. The surface layer was a thermosetting resin-impregnated paper high-pressure laminate (HPL) with a thickness of about 1 mm, which had high flame resistance and wear resistance. The adhesive between layers was a one-component polyurethane adhesive, which compared to other amino-based adhesives was characterized by strong adhesion, good water resistance and no formaldehyde emission.
Figure 1: Structural diagram of three kinds of fireproof, waterproof and wear-resistant composite plywood
The core board of the fireproof, waterproof, and wear-resistant composite plywood designed in this paper was Populus deltoids plywood, with a moisture content of 8% to 12% and an air-dry density of 589.8 kg/m3 (Produced by Jiangsu Shancong Wood Co., Ltd., located in Lianyungang, China). Plywood was prepared by rotary cutting of logs into veneers, multi-layer veneer orthogonal gluing, cold pressing, hot pressing, curing and sawing. Its thickness usually constitutes 8–25 mm, while the length is not limited. Since the plywood was made of orthogonal group blanks (the textures of adjacent layers were perpendicular to each other), its material was characterized by in-plane isotropy.
The levelling layer was MDF, with a moisture content of 8% to 11% and a density of 547 kg/m3, produced by Wujiang Shihu Wood Industry, located in Suzhou, China; the fireproof layer was a Portuguese cork board, obtained from the bark of cork oak. Its structure was composed of many tetra decahedral dead cells, which were filled with air, and the outside of the cell wall was surrounded by a lot of suberins. The plate was produced by Portugal AMRIM ICB company, its thickness and density constituted about 2 mm and 118 kg/m3, respectively. The board had excellent characteristics compared to other wood-based panels, such as thermal conductivity of 0.04 W⋅m−1⋅K−1, specific heat of ≥ 2.0 J⋅g−1⋅°C−1, and the ability to handle temperature ranges of −80°C–140°C. The performance of the board remains stable under high temperature or freezing conditions, and the weather resistance is more than 60 years [30].
The panel layer was an HPL fireproof board, which was composed of wear-resistant paper, decorative paper and kraft paper. It was first impregnated with resin and dried to form film paper and then cured by cross-linking reaction under high temperature and high pressure. It was produced by Shanghai Fumei Decoration Material, located in Shanghai, China.
Ordinary plywood was produced of thin layers of wood and pressed together with phenolic resin adhesive. The ratio of phenolic resin and flour was 4:1, which was provided by Wuhan Carnoss Technology Company, located in Wuhan, China. The core layer, the levelling layer, and the face layer were all cold-pressed with a one-component polyurethane adhesive, which had no formaldehyde release. It was produced by Fuller Adhesive Company (China).
As shown in Table 2, the main equipment used for ordinary plywood included a grinding machine, gluing machine, lengthening machine, cold pressing machine, hot pressing machine, sawing machine and sanding machine.
The cold pressing equipment used was CGYJ-150D cold press, with a total pressure of 150 t, the maximum distance between the upper and lower worktables was 1000 mm, and the power of the oil pump was 7.5 kW. It was produced by Shijiazhuang Cangao Huaan Power Electronic Technology company located in Shijiazhuang, China.
As shown in Fig. 2, the main processing flow of this fireproof, waterproof and wear-resistant composite plywood was as follows: poplar veneer sorting (substrate manufacturing) → veneer oblique grinding → drying → sizing → paving assembly → cold pressing and hot pressing → composite with MDF and HPL → cold pressing → trimming and edge sealing.
Figure 2: Production flow chart of fireproof, waterproof and wear-resistant composite plywood
The main production process of fireproof, waterproof and wear-resistant composite plywood developed by this research was as follows:
1) Ordinary plywood preparation process: Poplar was rotary cut into veneer units with a thickness of about 2 mm, which were subjected to oblique grinding, drying, grading, sizing, assembling (the texture between adjacent layers is vertical), cold pressing (pre-pressing).
2) Cutting process: sawing the plywood after 7∼10 days of curing and sanding to determine thickness and quality.
3) Sizing process with MDF and HPL: within 30 min, a one-component polyurethane adhesive was applied to the surface of the plywood and thin MDF; the layers were then assembled into a composite The total amount of glue constituted 110–120 g/m2.
4) Cold pressing process: cold pressing of 1.2 MPa was performed on the composite plywood after sizing; the pressing and curing times constituted 24 h and 5 days, respectively. This can be seen in Fig. 3.
5) Edge trimming and edge sealing process: As shown in Fig. 4, the edge of the board after trimming should be free of burrs; the board surface should be flat, without indentation and bending, and its diagonal difference should be within 2 mm.
Figure 3: Cold pressing process of composite plywood
Figure 4: Edge cutting process of composite plywood
The quality of the core plywood greatly affects the mechanical properties of the composite plywood and the gluing properties of the subsequent processes. When the surface of the plywood is uneven, with burrs, potholes, and other defects, the difficulty of its further finishing increases. To improve the fire resistance of composite plywood and reduce the release of harmful gases such as formaldehyde, it is very important to select the appropriate type of adhesive, amount of glue, cold pressing pressure and pressing time and other process parameters. Based on the research outcomes, the advantages of using a one-component polyurethane adhesive are suitability for cold pressing and the absence of formaldehyde emission, which reduces energy consumption and emissions of harmful gases during production. It is worth noting that the amount of adhesive should be 110–120 g/m2, and the pressure should be maintained at about 1.2 MPa for at least 24 h.
3 Physical and Mechanical Properties and Fire Resistance of Composite Plywood
In this study, the evaluation of physical and mechanical properties of composite plywood including static bending strength (MOR), elastic modulus (MOE), surface bonding strength, surface wear resistance and surface crack resistance was based on GB/T 17657-2013 “Test methods of evaluating the properties of wood-based panels and surface decorated wood-based panels” [31]. To assess the fire resistance of the composite, the method of burning through the composite plate at a fixed point using a propane fuel gun was chosen. The burn-through time was used as an index to evaluate the refractory performance [32–35]. The test pieces have been conditioned to constant mass in an atmosphere with a relative humidity of (65 ± 5)% and a temperature of (20 ± 2)°C. Constant mass is considered to be reached when the results of two successive weighing operations, carried out at an interval of 24 h, do not differ by more than 0.1% of the mass of the test piece. The schematic diagram and actual measurement of the fire resistance test are shown in Fig. 5.
Figure 5: Fire resistance test
The following physical and mechanical properties of the specimens with three design schemes were tested: static bending strength, elastic modulus, surface bonding strength, surface wear resistance and surface crack resistance. The fire resistance test adopts the method of recording the duration of fixed-point perforation with a hot-gun to determine the fire resistance of the specimen. The test results are shown in Table 3. The accuracy of the results constituted ±0.01 MPa for the mechanical test, ±0.5 mg for the surface resistance test, ±2 s for the time error range of the fire resistance test, and ±1°C for the temperature error range.
The static bending strength and elastic modulus test results of composite plywood met the requirements of GB/T 9846-2015 (Plywood for general use). For 12 mm thick plywood, the static bending strength constituted ≥28 MPa, and the elastic modulus was ≥5000 MPa. Since the surface material of composite plywood specimens S1, S2, and S3 was HPL with high density, their surface compressive and tensile properties improved, making the MOR values higher than that of conventional plywood of 28 MPa by 65.0%, 35.4% and 30.0%, respectively. Among them, the S1 board showed the highest values of MOR since it was composed of HPL, leveling layer MDF and base plywood. In S1 specimens, the interfaces of the materials are similar in pairs, which makes the bonding performance in the interface better and the corresponding MOR value higher. S2 board was composed of HPL, leveling layer MDF, cork board and base plywood. The results showed that the majority of bending failures occurred in the cork board layer due to its low density, loose attachment, rough interface and poor gluing performance, which, in turn, was reflected in the low MOR values. The S3 board was composed of HPL and base plywood. Due to the rough surface of the plywood, there is a difference between the interfaces of HPL and plywood, resulting in weak bonding strength and a lower MOR value of the S3 panel. All boards showed significantly increased elastic modulus values than conventional plywood with MOE of 5000 MPa since the surface materials of the S1, S2, and S3 boards were all HPL, which had higher surface rigidity. It should be noted that the differences between MOE values of 3 composite plywood boards were negligible.
The surface bonding strength mainly measures the bonding quality of the facing material and the base material of the composite plywood. The surface bonding strength of S1, S2, and S3 composite plywood specimens all exceeded the standard of 0.6 MPa required by GB/T 34722-2017. Among them, the surface roughness difference between the three materials used to compose the S1 board was small, resulting in the best gluing performance. The cork layer in the S2 board was obtained from the bark of the Portuguese cork oak and had a self-adhesive surface. Although the cork board is characterized by excellent fire resistance and thermal insulation properties, it has a loose structure and many pores. Therefore, the adhesive easily permeated into the cork’s surface, resulting in a decrease in its effective gluing area. According to the results, the surface bonding strength of the S2 board reached the standard requirement of GB/T 34722-2017. For the S3 board, due to the obvious difference in the interface roughness between HPL and plywood, a certain degree of adhesive penetration was also formed, which reduced the effective gluing area and corresponding surface bonding strength. Obviously, S3 showed better bonding properties than that of S2, but was still inferior to that of S1. According to the results, similar interface properties contributed more to higher surface bonding strength. Material interface bonding properties are shown in Table 4.
As for the surface abrasion test, the surface wear resistance of the test piece mainly depends on its surface material. The surface abrasion test values of S1, S2 and S3 composite plywood were in the range of 47–56 mg/100 r, which is far lower than the 80 mg/100 r specified in GB/T 15102-2017, indicating that the surface material HPL of the three specimens had excellent wear resistance. The surface crack resistance of S1, S2 and S3 boards with HPL as the surface material was level 5, which is the highest level specified in GB/T 17657-2013.
According to the fire resistance test results, the burn-through time of ordinary plywood was only 321 s, while the fire resistance time of S1, S2, and S3 structural boards was far longer than that of ordinary plywood. Among them, the S1 structural board and the S3 structural board showed the best results, and the burn-through time was about 1.75 times and 1.94 times higher than that of the unveneered plywood, respectively. Since the MDF was composed of wood fibers, it had a certain effect on supporting combustion, and the duration of the S3 structural board was slightly longer than that of the S1 structural board. However, considering the three requirements of plate surface flatness, surface bonding strength and fire resistance, the S1 structural plate showed greater advantages. The fire resistance of the S2 structural board was higher than that of ordinary plywood, and lower than that of the S1 and S3 boards. The study results showed that due to the low thickness of the cork board in the S2 specimen, the gaps were obvious resulting in poor fire resistance. At the same time, the air in the cavities contributed to a greater combustion-supporting effect, which reduced the performance of the HPL fire board.
Figs. 6 and 7 show the time-temperature curves of the fire resistance test. In the recorded data, the time and temperature errors were ±2 s and ±1°C, respectively. For ordinary plywood, the temperature at the center of the back of the specimen did not change significantly before 100 s. After that, the temperature of the specimen increased uniformly until the back of the specimen was carbonized and blackened. The backside temperature of the three composite plywood structures did not change significantly before 160 s, but the temperature of the S2 structural board increased significantly after that. This was because the HPL on the surface of the S2 structural board was burned through, and the flame burned the cork board. Because the air in the cavity of the thin cork board played a certain role in supporting combustion, it accelerated the temperature rise of the specimen. For the S1 structural plate, the flame further burned the thin MDF, which also played a certain combustion-supporting role and accelerated its temperature rise. It can be seen from Figs. 6 and 7 that the curve corresponding to the S2 structural plate was steeper, followed by the S1 structural plate, and the S3 structural plate had a slower rate of temperature increases because there was no intermediate layer with a certain combustion-supporting effect. Obviously, the S1 and S3 structural plates have more stable heating rates, which can provide more time and opportunities for evacuation in real fire situations. The temperature-time curves obtained from these two groups of fire resistance tests showed similar trends and fire resistance limit values with a high degree of agreement and good reliability. Among them, the S3 structural board showed the best fire performance.
Figure 6: Fire resistance test time-temperature curves (Group I)
Figure 7: Fire resistance test time-temperature curve (Group II)
In order to meet the market demand, it is necessary to carry out a cost-economic analysis of three kinds of composite structural plywood with a standard size of 2440 mm × 1220 mm, which integrates fire resistance, water resistance and wear resistance. The purchase price of a 1 mm thick HPL fireproof board is 70 CNY (China Yuan), 1 mm thick MDF is 70 CNY, 2 mm cork board is 60 CNY, and one-component polyurethane adhesive is 58 CNY/kg. Compared with ordinary plywood, the cost of each S1, S2 and S3 structural board with a size of 2440 mm × 1220 mm increased by about 370 CNY, 530 CNY and 180 CNY, respectively, while the unit price of ordinary plywood was 120 CNY. For the cost of these three structures, the additional cost input of the S3 structure was the lowest; followed by the S1 structure; and the cost input of the S2 structure board was the highest.
This paper introduces the structural design and production process of three kinds of composite plywood and tests their physical and mechanical properties and fire resistance according to national standards. The focus of the study is to evaluate the advantages and disadvantages of the three composite plywood boards in terms of fire resistance, wear resistance and water resistance. The results of the study show that the physical mechanics of the three kinds of composite structure plywood meet the standard requirements, and its fire resistance is far better than that of ordinary plywood. Among them, the overall physical and mechanical properties of the S1 structural board are the best. The S3 structural board has the best fire performance and the lowest increased production cost. Although the temperature of the S3 board changed slowly with time during the fire resistance test, the surface flatness and surface bonding strength of the board were weaker than those of the S1 structural board. The air in the cavities of the S2 board showed a certain combustion-supporting effect, which inhibited the fire resistance of HPL, and the cost of the board was the highest.
In sum, the use of common plywood as the base material and HPL fireproof board as a surface layer glued with a one-component polyurethane adhesive can greatly improve the fire resistance, water resistance and wear resistance of the composite structural plywood and meet the requirements of the surface flatness. Among them, the S3 structural board not only provides low additional production costs, but also multi-functional application of plywood, which increases the added value of the product and meets the needs of the market in fields such as construction, home furnishing, decoration, transportation, and other applications with high requirements on fire-, water- and wear resistance. The research results have high application value and market prospects.
Funding Statement: This work was supported by the 2020 Jiangsu Provincial Department of Science and Technology Policy Guidance Category (North Jiangsu Science and Technology Special SZ-L YG202014).
Conflicts of Interest: The authors declare that they have no known competing financial interests or personal relationships that could have appeared to influence the work reported in this paper.
References
1. Tao, C. L., Zhang, J. Z., Cheng, B. D., Liu, Y. (2019). An assessment of the impact of spatial agglomeration on the quality of China’s wood processing industry products. Sustainability, 11(14), 3961. DOI 10.3390/su11143961. [Google Scholar] [CrossRef]
2. Murata, K., Nakano, M., Miyazaki, K., Yamada, N., Yokoo, Y. et al. (2021). Utilization of Chinese fast-growing trees and the effect of alternating lamination using mixed-species eucalyptus and poplar veneers. Journal of Wood Science, 67(1), 1–8. DOI 10.1186/s10086-020-01937-5. [Google Scholar] [CrossRef]
3. Zhao, L., Cao, B., Wang, F., Yazaki, Y. (1994). Chinese wattle tannin adhesives suitable for producing exterior grade plywood in China. Holz als Roh- und Werkstoff, 52(2), 113–118. DOI 10.1007/BF02615476. [Google Scholar] [CrossRef]
4. Wan, M. L., Toppinen, A., Hänninen, R. (2010). Econometric analysis of China’s plywood market. Forest Products Journal, 60(7–8), 679–687. DOI 10.13073/0015-7473-60.7.679. [Google Scholar] [CrossRef]
5. Xu, Y., Guo, L., Zhang, H., Zhai, H., Ren, H. (2019). Research status, industrial application demand and prospects of phenolic resin. RSC Advances, 9(50), 28924–28935. DOI 10.1039/C9RA06487G. [Google Scholar] [CrossRef]
6. Wang, J. F., Wang, X., Zhan, T. Y., Zhang, Y. L., Lv, C. et al. (2018). Preparation of hydro-thermal surface-densified plywood inspired by the stiffness difference in “sandwich structure” of wood. Construction and Building Materials, 177, 83–90. DOI 10.1016/j.conbuildmat.2018.05.135. [Google Scholar] [CrossRef]
7. Wang, Z. H., Ghanem, R. (2021). An extended polynomial chaos expansion for PDF characterization and varia-tion with aleatory and epistemic uncertainties. Computer Methods in Applied Mechanics and Engineering, 382, 113854. DOI 10.1016/j.cma.2021.113854. [Google Scholar] [CrossRef]
8. Peng, Y. B., Wang, Z. H., Ai, X. Q. (2018). Wind-induced fragility assessment of urban trees with structural uncertainties. Wind & Structures, 26(1), 45–56. [Google Scholar]
9. Wang, Z. H., Ghanem, R. (2022). A functional global sensitivity measure and efficient reliability sensitivity analysis with respect to statistical parameters. Computer Methods in Applied Mechanics and Engineering, 115175. DOI 10.1016/j.cma.2022.115175. [Google Scholar] [CrossRef]
10. Wang, Z., Xie, W. B., Wang, Z. H., Chao, Y. (2018). Strain method for synchronous dynamic measurement of elastic, shear modulus and poisson’s ratio of wood and wood composites. Construction & Building Materials, 182, 608–619. DOI 10.1016/j.conbuildmat.2018.06.139. [Google Scholar] [CrossRef]
11. Wang, Z. H., Gao, Z. Z., Wang, Y. L., Chao, Y., Wang, G. G. et al. (2015). A new dynamic testing method for elastic, shear modulus and poisson’s ratio of concrete. Construction and Building Materials, 100, 129–135. DOI 10.1016/j.conbuildmat.2015.09.060. [Google Scholar] [CrossRef]
12. Wang, Z., Xie, W. B., Lu, Y., Li, H. T., Wang, Z. H. et al. (2019). Dynamic and static testing methods for shear modulus of oriented strand board. Construction and Building Materials, 216, 542–551. DOI 10.1016/j.apt.2008.07.002. [Google Scholar] [CrossRef]
13. Zhang, J. W., Liu, H. H., Yang, L., Hai, T. Q., Yin, Q. (2020). Effect of moderate temperature thermal modification combined with wax impregnation on wood properties. Applied Sciences, 10(22), 8231. [Google Scholar]
14. Yang, L., Han, T. Q., Fu, Y. D. (2020). Effect of heat treatment and wax impregnation on dimensional stability of pterocarpus macrocarpus wood. Wood Research, 65(6), 963–974. [Google Scholar]
15. Yang, L., Liu, H. H. (2020). Effect of a combination of moderate-temperature heat treatment and subsequent wax impregnation on wood hygroscopicity, dimensional stability, and mechanical properties. Forests, 11(8), 920. [Google Scholar]
16. Fateh, T., Rogaume, T., Richard, F. (2014). Multi-scale modeling of the thermal decomposition of fire retardant plywood. Fire Safety Journal, 64, 36–47. [Google Scholar]
17. Lebow, S. T., Winandy, J. E. (1999). Effect of fire-retardant treatment on plywood pH and the relationship of pH to strength properties. Wood Science and Technology, 33(4), 285–298. [Google Scholar]
18. Liu, K. W., Jayaraman, D., Shi, Y., Harries, K., Yang, J. et al. (2022). “Bamboo: A very sustainable construction material”-2021 international online seminar summary report. Sustainable Structures, 2(1), 000015. [Google Scholar]
19. Yalinkilic, M. K., Su, W. Y., Imamyra, Y., Takahashi, M., Demirci, Z. et al. (1998). Boron effect on decay resistance of some fire-retardant coatings applied on plywood surface. Holz als Roh- und Werkstoff, 56(5), 347–353. [Google Scholar]
20. Ayrilmis, N., Korkut, S., Tanritanir, E., Winandy, J. E., Hiziroglu, S. (2006). Effect of various fire retardants on surface roughness of plywood. Building & Environment, 41(7), 887–892. [Google Scholar]
21. Chou, C. S., Lin, S. H., Wang, C. I. (2009). Preparation and characterization of the intumescent fire retardant coating with a new flame retardant. Advanced Powder Technology, 20(2), 169–176. [Google Scholar]
22. Sun, F. C., Fu, J. H., Peng, Y. X., Jiao, X. M., Liu, H. et al. (2021). Dual-functional intumescent fire-retardant/self-healing water-based plywood coatings. Progress in Organic Coatings, 154, 106187. [Google Scholar]
23. Fang, L., Chang, L., Guo, W., Chen, Y. P., Wang, Z. (2014). Influence of silane surface modification of veneer on interfacial adhesion of wood–plastic plywood. Applied Surface Science, 288, 682–689. [Google Scholar]
24. Jiang, H. L., Liu, W., Huang, H. Y., Wang, Y. Q. (2022). Parametric design of developable structure based on yoshimura origami pattern. Sustainable Structures, 2(2), 000019. [Google Scholar]
25. Olonisakin, A., He, S. P., Yang, Y. F., Wang, H. P., Li, R. et al. (2022). Influence of stacking sequence on mechanical properties and moisture absorption of epoxy-based woven flax and basalt fabric hybrid composites. Sustainable Structures, 2(2), 000016. [Google Scholar]
26. Zhang, D. Y., Gong, M., Zhang, S. J., Zhu, X. D. (2022). A review of tiny houses in North America: Market demand. Sustainable Structures, 2(1), 000012. [Google Scholar]
27. Grigoriev, S., Vereschaka, A., Milovich, F., Sitnikov, N., Andreev, N. et al. (2021). Investigation of the properties of the Cr, Mo-(Cr, Mo, Zr, Nb) N-(Cr, Mo, Zr, Nb, Al) N multilayer composite multicomponent coating with nanostructured wear-resistant layer. Wear, 468, 203597. [Google Scholar]
28. Xu, J. F., Li, X. Y., Liu, R., Shang, Z., Long, L. et al. (2020). Dialdehyde modified cellulose nanofibers enhanced the physical properties of decorative paper impregnated by aldehyde-free adhesive. Carbohydrate Polymers, 250, 116941. [Google Scholar]
29. GB/T 9846-2015 (2015). Plywood and general use. China: China Quality and Standards Publishing and Media Co., Ltd. [Google Scholar]
30. Zhan, J., Xie, W. B., Wang, Z., Liu, Z. Y., Zhao, T. C. (2018). Fire resistance of column in cork composite structure building. Journal of Southwest Forestry University, 38(4), 161–166. [Google Scholar]
31. GB/T 17657-2013 (2013). Test methods of evaluating the properties of wood-based panels and surface decorated wood-based panels. China: China Quality and Standards Publishing and Media Co., Ltd. [Google Scholar]
32. GB/T 8626-2007 (2007). Test method of flammability for building materials. China: China Quality and Standards Publishing and Media Co., Ltd. [Google Scholar]
33. Dehane, B., Madrigal, J., Hernando, C., Bouhraoua, R., Guijarro, M. (2015). New bench-scale protocols for characterizing bark flammability and fire resistance in trees: Application to Algerian cork. Journal of Fire Sciences, 33(3), 202–217. DOI 10.1177/0734904114568858. [Google Scholar] [CrossRef]
34. Ferreira, R., Pereira, D., Gago, A., Proença, J. (2016). Experimental characterisation of cork agglomerate core sandwich panels for wall assemblies in buildings. Journal of Building Engineering, 5, 194–210. DOI 10.1016/j.jobe.2016.01.003. [Google Scholar] [CrossRef]
35. Catry, F. X., Moreira, F., Pausas, J. G., Fernandes, P. M., Rego, F. (2012). Cork oak vulnerability to fire: The role of bark harvesting, tree characteristics and abiotic factors. PLoS One, 7(6), e39810. DOI 10.1371/journal.pone.0039810. [Google Scholar] [CrossRef]
Cite This Article
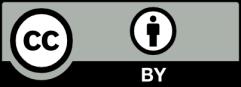