Open Access
ARTICLE
Enhancing the Decomposition of Paper Cups Using Galleria Mellonella and Eisenia Fetida
1 Civil Engineering Department, The University of Jordan, Amman, 11942, Jordan
2 Department of Chemistry, Technical University of Munich, Munich, Germany
3 Plant Production, Jordan University of Science and Technology, Irbid, 22110, Jordan
4 Basic Medical Veterinary Sciences, Jordan University of Science and Technology, Irbid, 22110, Jordan
* Corresponding Author: Shadi Moqbel. Email:
Journal of Renewable Materials 2024, 12(2), 349-367. https://doi.org/10.32604/jrm.2023.046369
Received 28 September 2023; Accepted 06 December 2023; Issue published 11 March 2024
Abstract
The composition of paper cups creates a challenge for the recycling industry, as the paperboard–plastic film composite is hard to separate. Therefore, paper cups are sent to landfills or waste incinerators. This study explores the combined use of red worms (Eisenia fetida) and Greater wax moth (Galleria mellonella) in the biodegradation of paper cups. The study investigates the conditions and combinations that promote using Eisenia fetida and Galleria mellonella for degrading paper cups. The study considered the influence of environmental temperature, the presence of food waste, varying the number of Eisenia fetida worms, and the presence of a Galleria mellonella growth-slowing agent on the degradation process. To achieve the study objectives, the study followed a quantitative approach. The study monitored the degradation of paper cup cuts that were placed in jars containing different combinations of Eisenia fetida worms, Galleria mellonella larvae, food waste, bedding material, and Galleria mellonella growth-slowing agents. The study found that the best operating temperature is 30oC. The study found that using food waste improves the performance of Eisenia fetida worms and Galleria mellonella larvae significantly. The study found that adding a Galleria mellonella growth-slowing agent slightly enhances the degradation of the paper cup. Finally, a numerical model was obtained to simulate the paper cup degradation efficiency.Graphical Abstract
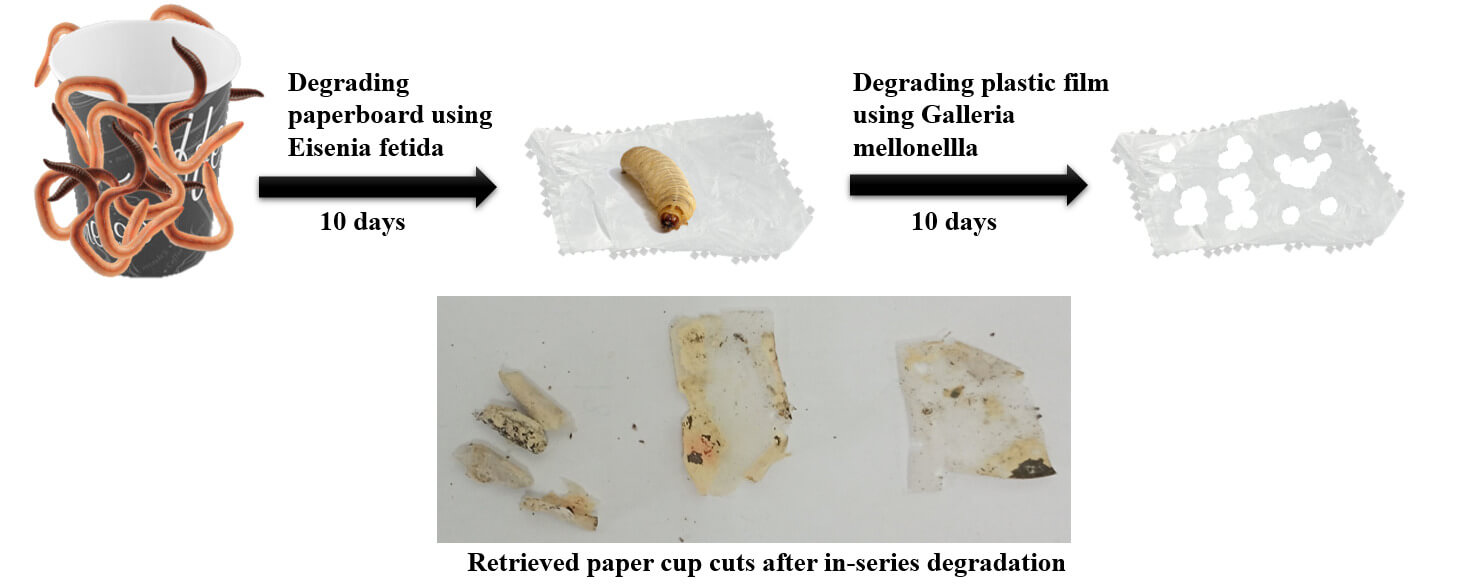
Keywords
The convenience provided by cheap disposable cups has favored their use among people compared to reusable cups. Disposable paper cups provide a wide range of usage versatility in addition to the ability to be used for hot and cold beverages. Furthermore, because of the hygienic benefits, such as in pandemics, they have become the preferred choice and their production is massive. The global production of paper cups is estimated at 263.8 Billion Units in 2022 with a growth rate of 1.11% per year and it is projected to reach 283.2 Billion Units by 2028 [1]. In 2018, the EPA estimated that 26 million tons of nondurable paper and paperboard products were generated; 1.4 million tons of these products were paper plates and cups [2].
The disposable paper cup consists of a paperboard that provides structural support with a base weight of 150–350 g/m2 [3]. This paperboard structure is laminated with an insulating plastic film, commonly light-density polyethylene, with a base weight of 8–20 g/m2 [3]. Although this composite structure provides a wide range of uses, it is the reason paper cups are not recyclable in most cases. The strong bond between the paper fibers and the plastic laminating material makes it hard to separate and recycle [4]. The growing demand for on-the-go hot beverages necessitated the addition of more layers to insulate the cup for people’s safety and convenience. As a result, the structure of paper cups is evolving into a more complex form. Recyclers prefer handling single material rather than hard-to-separate composite material. As a result, if not incinerated, paper cups are destined for landfills, where they will remain for an indefinite period. Unless serious measures are taken to intervene in the disposable cups production and disposal, the scale of the problem will continue to increase. Currently, there is no indication of a decrease in the production of these cups, nor is there any willingness among producers and related authorities to reduce production.
The massive production and accumulation of paper cups in landfills intrigued researchers to seek environmentally friendly solutions. Converting disposable paper cups into other products such as graphene sheets with Fe2+ as a catalyst [5], paper plastic composites [2], pulping new PA-coated paper cups [6], production of hydrocarbon liquid by pyrolysis [7], and synthesizing carboxymethylcellulose from disposable paper cups [8] was one approach to solving the paper cup problem. Although these solutions can keep some paper cups out of landfills, they are only temporary and do not provide complete destruction of the paper cup materials. Exploring new degradation or biodegradation methods is another approach that could provide a long-term solution and complete destruction. Paper cup biodegradation can be achieved by micro-organisms or macro-organisms capable of degrading the paper structure, the plastic film, or both materials. Furthermore, combining multiple species yields better results [9]. Composting was previously suggested as a possible solution. Ghorpade et al. [10] demonstrated the feasibility of composting paper cups with yard waste, but they also demonstrated that the fast chemical hydrolysis associated with paper composting may suppress the activity of compost microorganisms. Later, vermicomposting was considered for paper waste degradation. Arumugam et al. [11] explored vermicomposting using Eisenia fetida to degrade the paper of the paper cups. They found that a ratio of 1:1 of paper to cow dung is the best combination for the degradation of aliphatic, aromatic, carboxylic, and phenolic groups in the paper cup. Muthunarayanan et al. [12] used Eudrilus eugenia to compost paper cups and temple waste in order to reduce solid waste pollution. Sharma et al. [13] found that composting paper waste with earthworms is more efficient when mixed with rice straw and cow dung. Arumugam et al. [14] showed that adding bacterial consortia reduces the degradation period of paper cup waste using Eudrilus eugeniae significantly. Nevertheless, when subject to paper cups as the sole organic source, earthworms suffer oxidative stress, DNA damage, and tissue damage [11,15]. Although these studies have proved the ability of earthworms to degrade paper cups, little is known about their degradation behavior and kinetics. Furthermore, earthworms are still unable to cut through the plastic film on paper cups. As a result, it was necessary to investigate the degradation behavior of earthworms and incorporate another macroorganism capable of degrading plastics in paper cups.
Although Singh et al. [16] were the first to observe insects consuming plastic in the 1970s, it was recently that attention was drawn to insects’ ability to consume plastic. Recent studies have confirmed that several insects can degrade different types of plastic [17]. It was also confirmed that these insects can grow, mature, and reproduce while only eating plastic [18]. The work of Bombelli et al. [19] may be pivotal in bringing this kind of focus [20]. It showed the capability of Galleria mellonella to chew through plastic. Weber et al. [20] methodologically challenged the findings of Bombelli et al. [19], who could not rule out Galleria mellonella’s ability to degrade polyethylene. Several studies were conducted later and provided additional evidence confirming the ability of Galleria mellonella to degrade plastics [21,22]. Furthermore, Galleria mellonella was found to be capable of eating different types of plastic such as rigid polyurethane, polystyrene, and acrylonitrile-butadiene-styrene [23] and polyethylene film [24]. The structural similarity between honeycomb and plastics is believed to be the key to Galleria mellonella’s ability to feed on plastic [21].
The mechanism by which Galleria mellonella is capable of consuming plastics is still under study and a full understanding has not been achieved yet [17]. Determining the mechanism of plastic degradation by Galleria mellonella extends to deciding whether the degradation occurs in vivo or in vitro. In vitro studies showed that wax moths use a metabolic approach of wax-degradation strategies for plastic molecule polyethylene biodegradation without using intestinal microbiota [25]. On the other hand, several in vivo studies showed the ability of wax moth larvae to degrade plastic inside their intestines. Cassone et al. [22] demonstrated the role of the genus Acinetobacter in the intestinal microbiome of Galleria mellonella as in vivo biodegradation of polyethylene. Zhu et al. [23] discovered that Enterobacter is involved in the degradation of rigid polyurethane, whereas Enterococcus is involved in the degradation of acrylonitrile-butadiene-styrene and polystyrene. Zhang et al. [26] identified a fungal strain (Aspergillus flavus strain PEDX3) as the most efficient PE-degrading strain that can degrade both LDPE and HDPE in the gut of Galleria mellonella. In addition, they determined two LMCO enzymes as potential PE-degrading enzymes. Recently, Billen et al. [24] confirmed the ability of both greater wax moth larvae (Galleria mellonella) and mealworms larvae (Tenebrio molitor) to cut through plastic but concluded that the mechanism of relatively rapid biodegradation must include more than the gut microbiome alone.
Quantitative research on the Galleria mellonella degradation of plastic has shown two distinct outcomes. The first is that each Galleria mellonella is capable of consuming an average of 250 μg/day of LDPE [21] and a total of 35% of the mass of rigid polyurethane [23]. The second outcome is that this consumption decreases with time [21] and Galleria mellonella cannot feed on plastic as the only diet [24]. Adding diet supplements was found to be effective in increasing the biodegradation of plastics by Galleria mellonella [27].
The impact of plastics on the healthiness of Galleria mellonella is still under investigation [28–30]. Noël et al. [31] concluded that using light-density polyethylene does not influence the microbiota community in the guts of Galleria mellonella. While plastics can reduce the immune response for Galleria mellonella [28], survival rate, mobility, weight gain, and melanization processes in Galleria mellonella have been found unchanged when fed light-density polyethylene as the primary food [29,30]. Accordingly, Galleria mellonella can be a good candidate for degrading plastic film in paper cups. Currently, the concept of using Eisenia fetida and Galleria mellonella as degrading organisms working together has not been explored.
The purpose of this study is to investigate the combined use of red worm (Eisenia fetida) and Greater wax moth (Galleria mellonella) in the degradation of paper cups. These organisms are used in this study to degrade the two components creating paper cups (paperboard and plastic film). The objectives of the study are to investigate the conditions and combinations that optimize using Eisenia fetida and Galleria mellonella for degrading paper cups, to investigate the degradation behavior of Eisenia fetida and Galleria mellonella, and to investigate the influence of environment temperature and growth-slowing agent, and working order on the degradation process.
2.1 Red Worms and Wax Moths Collection
Red worms (Eisenia fetida) were obtained from a local supplier who imported them for composting purposes. For the wax moth, 5 hive-frames of wax moth-infested hives were collected from a local honey farmer. Wax moth larvae were collected from these frames and tested to confirm their identity as Galleria mellonella.
A larva and an adult wax moth were frozen for nearly 10 min at 4°C before the extraction step. Then, 25 mg of homogenized worm and (wing and leg) adult tissues were placed in 1.5 ml microcentrifuge tubes and extracted using DNeasy Blood & Tissue Kit (Qiagen, Germany) according to manufactural instructions. At the end of the extraction, 50 µL of elution buffer was used instead of 200 µL to increase the final DNA yield.
A fragment of Cytochrome Oxidase I gene (cox1) was amplified using the following pair primer: forward primer LCO1490 (5′-GGTCAACAAATCATAAAGATATTGG-3′) and reverse primer HCO2198 (5′-TAAACTTCAGGGTGACCAAAAAATCA-3′) (Folmer et al., 1994). In a 12.5 µL total volume, 6.25 µL of GoTaq® Green Master Mix (Promega, USA) 0.5 µL of forward and reverse primers (10 µM), 3 µL of DNA sample were added. Nuclease-free water was used to complete the volume instead of DNA in the negative control. PCR program consisted of 1 cycle of 94°C for 2 min, followed by 35 cycles of 94°C for 30 s, 55°C for 30 s, and 72°C for 30 s, with a further extension step at 72°C for 5 min then cooled at 4°C as a final step. A 709 bp PCR product was detected on a 1.5% agarose gel after running a 5 µL amplicon of each PCR sample. The remaining volume was used for the sequencing step. PCR products were sent for Sanger sequencing with purification and sequencing service at Jordan University of Science and Technology. Samples were purified using ExoSAP-IT™ PCR Product Cleanup (Applied Biosystems™) and according to kit recommendations, then prepared for sequencing by following BrilliantDye TM Terminator v1.1 Cycle Sequencing Kit protocol. The sequencing service employed primer pairs that were used to amplify the cox1 fragment and then carried out and run in an automated sequencer (SeqStudio™ Genetic Analyzer System with SmartStart). The obtained chromatograms were visually checked with Ugene software to identify the homology of the sequenced samples. Each sequence was uploaded and searched through the NCBI database, using the BLASTn application.
2.3.1 Galleria Mellonella Rearing
Several Galleria mellonella larvae were collected from the beehive frames infested with the wax moth. These larvae were transferred into several 1 L glass containers covered with pierced metal lids. Each metal lid had several 1-mm punctures to allow airflow and prevent pressure buildup inside the container. The containers were previously furnished with a rearing diet including a mix of (corn meal, yeast, soy flour, powder milk, honey, glycerol, and beeswax blocks) as described by Jorjão et al. [32]. Later, rearing Galleria mellonella was continued following mostly the procedure described by Pereira et al. [33]. The procedure consisted of placing every 4–5 wax moth larvae in an empty 1-L glass container. The container is closed with a pierced metal lid. The container is placed upside down with filter paper placed inside the lid. The filter paper is meant to collect eggs. Every 2–3 days, the filter paper is removed and replaced with a new one. The collected filter paper is then placed in another glass 1-L container with around 1–2 cm layer of rearing diet. The filter paper is placed above the diet media until the larvae are seen crawling inside the medial and on the container wall. The process is then repeated when the new moths are developed inside the container. All of the rearing containers were placed in an incubator at 30°C.
The worms were placed in several 15-L containers filled with locally produced peat moss. Food waste was added to the containers every 4–5 days. As the number of worms noticeably increased, around 20 adult worms were transferred to a new 15-L container filled with peat moss. The containers were kept at room temperature in a shaded area.
2.4 Testing Medium and Components
The tests of paper cup degradation were performed in 300 ml jars with perforated metal lids. Each jar was washed with deionized water and dried before testing. Then, each jar was furnished with 15 g of peat moss and watered with 10 ml of deionized water to keep the moisture content at 60%–70%. Paper cups were cut into small rectangles about 2–3 cm long with an average weight of 0.167 g. One rectangular paper cup cut was added to each jar. Food waste was added with an average weight of 4.5 g. Food waste consisted of kitchen food waste. Due to the limited setup size, small pieces of raw leftover tomato were used as food waste. During the placement of the food waste in the jar, the food waste was not put into contact with the paper cup cuts.
Each jar assigned for testing with worms included 10 adult Eisenia fetida worms with a weight range of 0.9–1.0 g. Each jar assigned for wax moth larvae included 10 Galleria mellonella larvae with a weight range of 0.16–0.18 g. The selection of Galleria mellonella and Eisenia fetida for experimental work was on reaching 2 cm for larvae as recommended by Pereira et al. [33] while for Eisenia fetida worms it was having 10–12 cm adult worms.
2.5 Paper Cup Biodegradation Tests
The study examined the biodegradation potential of paper cups by Galleria mellonella and Eisenia fetida under different conditions. The test setup is based on cutting paper cups into small pieces and placing these cuts in small jars that include Galleria mellonella, Eisenia fetida, or both with supporting materials at different conditions. The tested conditions were different combinations of temperature, food waste, and working order. Accordingly, five combinations were used in addition to a control sample. The test jar content was prepared according to the matrix presented in Table 1.
To cover potential variability, each jar combination was tested in a group of 5 jars. The weight of paper cup cuts was recorded and visual inspection was observed at each stage. After each stage, paper cup cuts were left in a dry area for 24 h to evaporate the excess moisture absorbed during the incubation period. Later, paper cup cuts were cleaned of attached peat moss using a fine brush and then weighed.
As the biological activities of Galleria mellonella and Eisenia fetida are influenced by temperature, the study combinations were tested at different operating temperatures to determine the optimum temperature. Tests were conducted at temperatures 20°C, 25°C, 30°C, and 35°C.
As the optimum temperature was determined, the tests were repeated at the optimum temperature for Eisenia fetida and Galleria mellonella to work in series. In this setup, paper cup cuts were exposed firstly to Eisenia fetida and then to Galleria mellonella. The setup preparation was similar to the one used in the previous part. The bedding material, paper cup rectangles, and food waste were placed in a 300 mL jar. Then 10 adult Eisenia fetida worms were added to the jars and incubated for 10 days at 30°C. After 10 days, the paper cup cuts were extracted, dried, and weighed. After weighing, the paper cup cuts were added to jars that included 10 Galleria mellonella larvae and incubated again for 10 days. After the second incubation, the remaining paper cup cuts were retrieved and weighed. Upon completion of the test, visual observations were recorded and removal percentage was determined.
2.6 Enhancing Degradation Techniques
2.6.1 Applying Galleria Mellonella Growth-Delaying Agents
Optimizing the degradation process and increasing the degradation ratio were studied in the form of prolonging the larval stage for Galleria mellonella using plant extract as mentioned in Zaitoun [34]. Galleria mellonella larvae can rush their development to the pupation stage when subjected to stresses such as suddenly changing their diet [33]. Delaying the pupation can occur using juvenile hormones or by sudden cooling, but such conditions affect their eating progress and their weight gain [29]. Some plant seeds’ extracts were found to suppress the pupation safely [34]. Plantago psyllium as a suppressing agent was chosen due to its local availability. The extract was prepared using cold percolation with 95% ethanol as described by Zaitoun [34]. The extract of plant seeds was applied to paper cups and the degradation of paper cups was monitored at 20°C, 25°C, 30°C, and 35°C. At the time of application, the paper cup cut pieces were dipped in the extract oil before being placed in test jars. Ten Galleria mellonella larvae were added to the test jars. Then, the jars were incubated at the specified temperature for ten days.
2.6.2 Number of Eisenia Fetida Worms
Optimizing paperboard degradation was sought by varying the number of Eisenia fetida worms in the testing jar. Accordingly, degradation tests were conducted at optimum degradation temperature (30°C) with food waste. The study examined the degradation when adding 5, 10, and 20 Eisenia fetida worms to the testing jars. One paper cup cut was placed in each testing jar. The paper cup cuts in the testing jars were almost the same weight. The test for each count of worms was repeated 3 times. The testing jars were placed in an incubator for 10 days. By the end of the incubation, the paper cup cuts were extracted and dried. Upon drying, the average weight loss for each group was determined.
2.7 Paperboard Degradation Rate Determination
The previous tests have shown that the paper cup cuts absorbed moisture while being in the jar. Therefore, measuring paperboard loss necessitated extracting, drying, and weighing the paper cup cuts to determine the mass loss percentage. As a result, it was hard to measure the weight and then return them after the drying period. Therefore, measuring the paperboard degradation was performed in a set of 15 testing jars. The testing jars were prepared in a similar manner to the previous tests where 300 ml jars with perforated metal lids were used. Each jar was washed with deionized water and dried before testing. The jars were furnished with 15 g of peat moss and watered with 10 ml of deionized water to achieve the required moisture content. Paper cups were cut into rectangles about 2–3 cm long. A single rectangle of the paper cup cuts was added to each jar. Each jar assigned for testing with worms included 10 adult Eisenia fetida worms. All 15 jars were prepared and placed in an incubator at 30°C (optimum degradation temperature). Three jars were taken out of the incubator every two days and the paper cup cut would be placed for drying and then weighing. The test spanned over 10 days and the paperboard loss was determined over the entire period.
In this part of the experiment, the impact of food waste on the degradation rate was also explored. Hence, two sets of testing jars were prepared: test with food waste, and test without food waste. Food waste was added to jars with an average weight of 4.5 g. The paperboard loss was determined for these two sets.
The wax moth that was collected from the field was identified by doing PCR on the cox1 gene for the insect larva and adult then sequenced. It matched Galleria mellonella with a 99.56 % sequence identity similarity to NCBI-deposited isolate MH416635.1.
During the rearing process, several observations were recorded confirming the degradation of paper cups and the potential use of Eisenia fetida and Galleria mellonella as the main biodegradation agents for future use. Two paper cups were placed in a 15 L container containing peat moss only and two paper cups were placed in a 15 L container used for rearing Eisenia fetida. The paper cups were retrieved after one month of placement. A picture of retrieved cups is shown in Fig. 1. Fig. 1 shows that Eisenia fetida worm degraded the accessible paper of the paper cups completely. Because the cup rim is made by curving the cup edge to make the smooth rim, the paper on the cup rim was not degraded due to inaccessibility. Worms were also unable to access the sealing lines on the sides and bottom. On the other hand, the paper cups in the peat moss alone had little degradation. Fig. 1 shows the inability of Eisenia fetida to penetrate and chew the plastic film.
Figure 1: Degradation of paper of the paper cups using Eisenia fetida. Cups to the left were subject to Eisenia fetida degradation. Cups to the right were not subjected to Eisenia fetida degradation
Degradation of paper cups using Galleria mellonella was also noticed when placing a paper cup in a rearing jar containing Galleria mellonella larvae. Also, an LDPE film was extracted from a paper by dissolving the paperboard in an acid. The plastic film was washed thoroughly with distilled water and then placed in another rearing jar. The larvae were able to penetrate the paper cup wall and the plastic film, causing several holes as shown in Figs. 2 and 3. These figures demonstrate Galleria mellonella’s ability to feed on plastic film.
Figure 2: Holes in the paper cup wall created by Galleria mellonella larvae
Figure 3: Plastic film after degradation by Galleria mellonella
The degradation tests of paper cups using Eisenia fetida and Galleria mellonella at different conditions showed wide variations in terms of the degree of degradation and the final product. The percentages of degradation for each combination and test temperature are shown in Table 2. In all conditions, the control samples lost less than 0.5% of their weight over the course of the tests. As a result, the procedure used had no significant effect on the test results. The study results show that the degradation tends to increase with temperature increases; however, in samples containing Eisenia fetida worms, degradation dropped after 30°C while it continued to rise in Galleria mellonella larvae samples. This drop is also noticed in the combination with worms. The drop in the combinations that include Eisenia fetida worms can be attributed to the impact of heat on Eisenia fetida. Therefore, it can be inferred that the overall performance is sensitive to temperature change due to its impact on Eisenia fetida. Although the literature has shown that Eisenia fetida can tolerate a wide range of ambient temperatures with an optimum temperature of 25°C [35,36], the optimum performance for Eisenia fetida was noted at 30°C. The increase in temperature, on the other hand, created a favorable condition for Galleria mellonella larvae to consume more of the paper cup material. The results show that as the temperature rises, the degradation of paper cups by Galleria mellonella larvae increases from 1% to 13.4%. Although Galleria mellonella exhibited low degradation percentages compared to Eisenia fetida, plastic film degradation was only observed in the combinations that included their larvae.
The combination of worms and food waste showed a significant increase in paper cup degradation compared to worms alone. Adding food waste increased the degradation by about 21%, 23%, 12%, and 11% for temperatures of 20°C, 25°C, 30°C, and 35°C, respectively. The increase was higher at 20°C and 25°C and lower at 30°C and 35°C. The results of this combination provide a solution to Arumugam et al. [11] reported body loss and tissue damage when using paper cups as the sole organic food source of Eisenia fetida.
The combination of Eisenia fetida and Galleria mellonella showed unexpected behavior. Compared to Eisenia fetida alone, the degradation was higher at 20°C and 35°C and slightly lower when the temperature was 25°C and 30°C, which are favorable temperatures for Eisenia fetida. A justification for increased degradation outside the favorable temperature range can not be deducted. This observation may be answered by future research.
Adding food waste to the combination of Galleria mellonella and Eisenia fetida improved the degradation drastically. The study results show that using a combination of Eisenia fetida, Galleria mellonella, and food waste resulted in the greatest degradation at all temperature values tested. At 20°C, the degradation improved by 28% compared to Eisenia fetida alone and by 7% compared to Eisenia fetida and food waste. At 25°C, the improvement was less than what was noticed at 20°C, where degradation increased by 24% compared to Eisenia fetida alone and by about 1.5% compared to Eisenia fetida and food waste. As the temperature increased, the improvement became less. The best performance for this combination was 75.7% degradation at 30°C.
The degradation of paper cups using Galleria mellonella alone achieved the lowest degradation percentages. In the samples with Galleria mellonella alone, the degradation was as low as 1% at 20°C then increased with temperature rise. Although the optimum temperature for Galleria mellonella has been reported at 30°C [37], the degradation efficiency kept increasing with temperature rise to 13.4% at 35°C. This result indicates that wax moth larvae favor a temperature range higher than Eisenia fetida. Concurrently, the low degradation results show that it is inadvisable to use Galleria mellonella as a sole biodegradation agent. Nevertheless, it is worth noting that the degradation of the laminating polyethylene was only observed in the sample containing Galleria mellonella regardless of the setup. Fig. 4 shows the degradation of paper cup cuts using Galleria mellonella alone. Also, it was observed that some of the Galleria mellonella larvae would isolate themselves from the beginning of the test to build a cocoon and pupate inside.
Figure 4: Paper cup cut retrieved after using Galleria mellonella alone
When comparing the separate degradation of Eisenia fetida and Galleria mellonella and their work combined, it becomes clear that their work together is relatively better than working alone. Their combined work was better at temperatures of 20°C and 35°C than working alone, while their work together was a little less than the work of Eisenia fetida alone. Such a result might be explained that in the temperature range of 25°C–30°C the two species become very active and the activity of one specie affect the other.
The results show that the combinations with food waste had the highest degradation percentage. The combination of Eisenia fetida with food waste had a 10%–23% higher degradation than Eisenia fetida alone. Also, the combination with food waste, larvae, and worms had a 6%–23% increase in degradation compared to degradation with worms and larvae. This condition can be explained by the fact that food waste offered additional ingredients for Eisenia fetida and Galleria mellonella survival. Therefore, Eisenia fetida and Galleria mellonella were capable of eating more of the paper cup material.
The degradation ratio for the Eisenia fetida and Galleria mellonella larvae after a 10-day incubation period was calculated at each test temperature. The calculation results are shown in Fig. 5. The results show that the Eisenia fetida has a relatively higher degradation ratio than Galleria mellonella except for temperatures higher than 30°C. Although the degradation ratio seems higher for the Eisenia fetida, it must be noted that the Eisenia fetida degrades the paper part only while the wax moth larvae degrade both parts of the paper cup. The degradation ratio profile with temperature for Eisenia fetida shows an increase when the temperature rises to 30°C then it decreases as the temperature goes beyond 30°C. Interestingly, the degradation ratio for the wax moth larvae beyond 30°C is much higher than for Eisenia fetida.
Figure 5: Degradation ratio for the red worms and wax moth larvae at each temperature
The degradation rate at which paper cup cuts were degraded was calculated based on the amount of consumption of paper cups per insect per incubation period. According to these calculations, Eisenia fetida has a consumption rate of 1.83 × 10−4, 2.68 × 10−4, 1.25 × 10−3, and 4.43 × 10−4 g paper cup/(g Eisenia fetida. day) at temperatures 20°C, 25°C, 30°C, and 35°C, respectively. Galleria mellonella larvae have a consumption rate of 1.1 × 10−4, 2.16 × 10−4, 7.18 × 10−4, and 1.56 × 10−3 g paper cup/(g Galleria mellonella. day) at temperatures of 20°C, 25°C, 30°C, and 35°C, respectively. For the combined work of Eisenia fetida and Galleria mellonella, the degradation rate of paper cups was calculated without considering their weight. Accordingly, the degradation rate was calculated as 6.68 × 10−3, 7.3 × 10−3, 1.787 × 10−2, and 6.96 × 10−3 g paper cup/day at temperatures of 20, 25, 30, and 35 oC, respectively.
Following the performance of the Eisenia fetida and Galleria mellonella larvae in degrading the paper cup cuts at different temperatures. The test was repeated at optimum temperature (30°C) where the degradation was split into two steps (in-series degradation). The paper cup cuts were degraded initially by Eisenia fetida worms in the presence of food waste for 10 days then Galleria mellonella larvae for 10 days. During the first 10 days, the Eisenia fetida degraded an average of 77.7% of the paper cup cuts. In the second 10 days, the Galleria mellonella larvae degraded an average of 15.2% of the material left by the Eisenia fetida. The test showed two significant outcomes. First, the in-series configuration increased the overall degradation of paper cup cuts to more than 80% by weight, which is greater than the previous test combinations. Second, the remaining of several paper cup cuts were plastic films with a very small amount of paperboard material. Once these films were placed for wax moth degradation, wax moth larvae fed on them. Some of the paper cup cuts were fragmented by the wax moth larvae into small pieces. In one jar, the Galleria mellonella larvae used some of these small pieces in building their cocoon near the end of the test period. Some larvae, on the other hand, merely chewed the edges of the paper cup cuts and made several small holes in them. Fig. 6 shows retrieved paper cup cuts after the in-series process. In Fig. 6, two of the films show high degradation and loss in the plastic film and one fragmented film used to build a cocoon. The fragmented film in Fig. 6 contains two cocoons. The estimation of over 80% degradation was done conservatively as some of the small plastic pieces became attached to the cocoon material. It is worth noting that the fragmentation of the plastic film was only noticed in the in-series setup. No fragmentation was noticed in any of the previous combination tests. Hence, it is clear from the test that the in-series degradation of Eisenia fetida and Galleria mellonella larvae is much better than placing them together.
Figure 6: Retrieved paper cup cuts after in-series process
The degradation rate in this optimized setup as the combined consumption of paper cups per day was calculated to be 4.17 × 10−2 g paper cup/day at a temperature of 30°C. The degradation rate during the first phase, Eisenia fetida degradation, was estimated to be 3.46 × 10−3 g paper cup/(g Eisenia fetida. day). The degradation rate during the second phase, Galleria mellonella degradation, was estimated to be 1.17 × 10−3 g paper cup/(g Galleria mellonella. day).
3.5 Enhancing Degradation Tests
3.5.1 Degradation Using Growth-Delaying Agents
Adding plant extract to the paper cups was intended to delay the pupation stage and extend the larva stage. In this test, wax moth larvae were added in a similar manner to the previous tests but with paper cup cuts covered with a plant extract (Plantago psyllium). The resulting mass losses were 1.6%, 4.0%, 7.6%, and 10.4% at 20°C, 25°C, 30°C, and 35°C, respectively. The results show that the plant extract caused a slight increase in degradation percentage at temperatures 20°C, 25°C, and 30°C and a slight decrease in degradation at 35°C. The increase in degradation rate may be attributed to the suppressing effect of the extract and probably increasing the larva’s appetite to eat more.
3.5.2 Number of Eisenia Fetida Worms
The results from the paperboard degradation with different numbers of worms showed a different behavior than what might be projected. The paperboard loss percentage showed an increase as the number of worms increased from 5 to 10; however, the loss percentage decreased as the number increased from 10 to 20 worms. The average weight loss percentages with 5, 10, and 20 worms were 41.4%, 70.3%, and 46.6%, respectively. The results show that increasing the number of worms improves degradation, but only up to a point where having too many worms reduces degradation. These results are in agreement with Mohapatra et al. [38]. For the test setup used in this experiment, the optimum degradation was obtained with 10 worms. The reason behind this observation might be the high competition among the worms. The high competition appears to drive the worms away from available food, resulting in less paperboard consumption. Using larger volume setups or adding higher amounts of paper cups might give a different ratio than the results obtained in this experiment. Further study is needed to determine the optimum number for larger-scale setups.
Forecasting the obtained data for the use of Eisenia fetida and Galleria mellonella as degrading organisms aids in understanding their utilization and provides a rough estimate of what is required to degrade paper cups on a large scale at this stage. As a result, using an in-series process to degrade one gram of paper cup will necessitate the use of 28.9 g of Eisenia fetida for ten days. In the case of 0.9 g adult Eisenia fetida, this equates to approximately 32 worms. Based on the results presented earlier, these 32 Eisenia fetida worms are expected to express a degradation efficiency of 77%. Assuming, conservatively, that the Galleria mellonella will add 3% removal efficiency, it will translate into using 2.56 g of Galleria mellonella larvae. In the case of using 0.16 g Galleria mellonella larvae, it will mean using 16 larvae. Extending these numbers to handle disposed paper cups of 1 ton and higher will mean multiplying these numbers by three orders of magnitude and more. Furthermore, decreasing the degradation duration might mean increasing the numbers by another order of magnitude. The establishment of degradation units that handle such a number will require further studying and techno-economic assessment to determine its feasibility.
3.7 Paperboard Degradation Rate Test
The degradation rate test over a period of 10 days showed more details on the Eisenia fetida behavior while consuming the paperboard. Fig. 7 depicts the retrieved paper cup cuts over the course of the ten-day testing period with food waste. Fig. 8 depicts the percentages of degradation of paper cups over time. The degradation of the paper cup began on the second day of the experiment and progressed linearly over time. This was observed in both the test with food waste and the test without food waste. This observation may be explained by the fact that the worms take some time to acclimate to the new environment. As time progressed, worms fed on the paperboard, and the paperboard degradation increased. The results show that the mass loss percentage has a near-linear relationship with time starting from the second day. A linear function was fitted to the data obtained. The linear functions are presented for each group in Fig. 8. The results show that the degradation percentage for samples containing food waste was higher than for samples containing no food waste. Although the degradation for both cases was almost the same on day 2, the difference between the two groups grew over time, and the degradation with food waste was greater than the degradation without food waste. This result confirms the observation noted in the previous experiment.
Figure 7: Retrieved paper cup cuts along the ten days degradation using Eisenia fetida with food waste
Figure 8: Paper cup mass loss percentage vs. time
Calculating the degradation rate based on the duration for each group showed that the degradation rate varies with time. The degradation rate profiles with time for the worms with food and without food are shown in Fig. 9. The calculated degradation rate as gram paper cup per gram worm per incubation period shows that the degradation tends to increase up to a certain point and then decrease. This trend was observed in both groups (with food waste and without food waste). In the samples without food waste, the inflection point was on the sixth day. After the sixth day, the degradation rate decreases. In the samples with food waste, the inflection point was on the eighth day. It is worth noting here that the food waste disappeared in the samples with food waste on the fourth day. After the consumption of the food waste, the worms behaved in a similar trend to the samples without food waste where the degradation rate decreased, which indicates that adding the food waste postponed the drop in degradation rate. It is expected that a continuous supply of food waste will improve the degradation more and extend the improved degradation period further.
Figure 9: The degradation rate of paper cups over time
The profiles show that the degradation rate is higher for the samples with food waste than for the samples without food waste. The highest degradation rate for the samples with food waste was 0.00434 g/g-d on the eighth day while the lowest degradation rate was 0.00051 g/g-d on the second day. The highest degradation rate for the samples without food waste was 0.00259 g/g-d on the sixth day while the lowest degradation rate was 0.0003 g/g-d on the second day. It is noted that with the exclusion of the calculations on the second day, the degradation rates for the samples with food waste were entirely higher than the highest degradation rate for the samples without food waste.
The data obtained in the combination tests was used to build a numerical model to predict the efficiency of degradation. The model considered the contribution of paper cup weight, Eisenia fetida worms’ weight, Galleria mellonella larvae weight, the food waste weight, and the operating temperature. Since the weights of paper cups, Eisenia fetida worms, Galleria mellonella larvae, and food waste can be variable, the model considered the ratio of the Eisenia fetida worms, Galleria mellonella larvae, and food waste weight to the paper cup weight. The following equations were found to be the best fit that represented the data with a coefficient of determination R2 = 0.99,
where,
WP: ratio of worms’ weight to paper cup weight
MP: ratio of wax moth larvae weight to paper cup weight
FP: ratio of food waste to paper cup weight
ϴT: temperature correction factor
T: temperature in °C
3.9 Limitations and Challenges
The study acknowledges some limitations and challenges that were faced during the execution of the experimental work. First, the study measured the degradation of paper cups at the end of the incubation period relative to the initial weight. Because the paperboard absorbed some of the surrounding moisture, estimating the degradation rate during the incubation period was impossible. Therefore, weighing the paper cups requires a relatively long time to dry the paper cup cuts. Second, the study could not determine exactly how much was consumed by the Eisenia fetida worms and Galleria mellonella larvae when they were placed together. Third, the study considered an equal number of Eisenia fetida worms and Galleria mellonella larvae in each combination. Testing the performance using different measures is expected to give a better understanding of their performance. Fourth, the wax moth is considered a pest for beehives; therefore, their rearing was conducted in a controlled environment in the laboratory. It was observed that full control of the wax moth could not be achieved. Their eggs are very small and can be transported by air draft, which resulted in the escape of some of them. Also, rearing wax moths in jars with a diet and limited air circulation often resulted in fungus formation. Several colonies died during the rearing process due to fungi formation. Burd et al. [30] pointed out that fungi formation is unavoidable. Having open jars or jars with high air circulation to reduce fungi formation risks the escape of adult wax moths or some small larvae crawling out of the jars. Fifth, the study did not consider the healthiness of the Eisenia fetida worms and Galleria mellonella larvae after degrading paper cups due to resource limitations. The only available measure is the number of living worms and larvae, which did not change during the test in general. Finally, differentiating the exact degradation percentage of plastic film and the paperboard by Galleria mellonella was not possible at the time of conducting this study. Therefore, future studies might consider the degradation of these two components separately.
This study aimed to explore the combined use of red worm (Eisenia fetida) and Greater wax moth (Galleria mellonella) in the degradation of paper cups. The study used a quantitative approach to evaluate the degradation potential of these macro-organisms. The study has confirmed the potential use of Eisenia fetida and Galleria mellonella as degrading organisms of paper cups. Such results can help divert part of the organic waste from going to the landfill and introduce a new approach to the disposal of disposable paper cups. In this study, these organisms were used together and in series to degrade the two components creating paper cups. The study examined different combinations for degrading paper cups and the results show that adding food waste to a mix of Eisenia fetida worms and Galleria mellonella larvae can help achieve about 75% degradation at 30°C within 10 days. The study found that the work of Eisenia fetida worms and Galleria mellonella larvae in series can achieve more than 80% degradation within 20 days with significant decomposition of the LDPE film. The study confirmed the ability of Galleria mellonella larvae to consume LDPE and paperboard but at a slower rate than the Eisenia fetida worms. The study found that using Eisenia fetida worms as a degrading agent requires optimization of the number of worms relative to the paperboard as a high number of worms might decrease the degradation process due to the food competition among the worms. Furthermore, achieving a high degradation percentage using Galleria mellonella requires using a massive amount of larvae, which might raise concerns in agricultural areas. The effect of temperature was found to be critical in controlling the degradation process. The results from this study show that the best operating temperature is 30°C. The study shows that using food waste improves the performance of Eisenia fetida worms and Galleria mellonella larvae significantly. Furthermore, the study found that adding some plant extract as a growth-slowing agent can slightly enhance the degradation of the paper cup using Galleria mellonella larvae. The results obtained show that the degradation by Eisenia fetida has an optimum degradation rate on the sixth day while adding food waste helps increase this rate and extend the activity duration. In this study, a numerical model was obtained to simulate the paper cups’ degradation efficiency. Finally, the study found that experimental techniques for measuring the degradation in composite material are needed and recommends further studies on Eisenia fetida and Galleria mellonella degradation behavior.
Acknowledgement: The authors would like to thank the Deanship of Scientific Research at the University of Jordan for supporting this study.
Funding Statement: This study was funded by the Deanship of Scientific Research at the University of Jordan. The fund was awarded to S. M., A. A., and R. M.
Author Contributions: The authors confirm their contribution to the paper as follows: study conception and design: S. M., A. A., and R. M., data collection: H. A., and S. M.; analysis and interpretation of results: S. M., A. A., and R. M.; draft manuscript preparation: S. M., A. A., and R. M. All authors reviewed the results and approved the final version of the manuscript.
Availability of Data and Materials: The data that support the findings of this study are available from the corresponding author, S. M., upon reasonable request.
Ethics Approval: The study was approved by the Scientific Research Board at the University of Jordan (Ref # 2020-2019/74).
Conflicts of Interest: The authors declare that they have no conflicts of interest to report regarding the present study.
References
1. Imarc (2022). Paper cups market: Global industry trends, share, size, growth, opportunity and forecast 2023–2028. https://www.imarcgroup.com/paper-cups-manufacturing-plant#:~:text=The%20global%20paper%20cups%20market,1.11%25%20during%202023%2D2028 (accessed on 08/02/2023). [Google Scholar]
2. EPA (2018). Nondurable goods: Product-specific data. https://www.epa.gov/facts-and-figures-about-materials-waste-and-recycling/nondurable-goods-product-specific-data#tab-7 (accessed on 08/02/2023). [Google Scholar]
3. Triantafillopoulos, N., Koukoulas, A. A. (2020). The future of single-use paper coffee cups: Current progress and outlook. BioResources, 15(3), 7260–7287. [Google Scholar]
4. Mitchell, J., Vandeperre, L., Dvorak, R., Kosior, E., Tarverdi, K. et al. (2014). Recycling disposable cups into paper plastic composites. Waste Management, 34, 2113–2119. [Google Scholar] [PubMed]
5. Zhao, H., Zhao, T. S. (2013). Graphene sheets fabricated from disposable paper cups as a catalyst support material for fuel cells. Journal of Material Chemistry A, 1(2), 183–187. [Google Scholar]
6. Lee, T., Yoon, C., Ryu, J. Y. (2017). A new potential paper resource; recyclability of paper cups coated with water-soluble polyacrylate-based polymer. Nordic Pulp & Paper Research Journal, 32(1), 155–161. [Google Scholar]
7. Biswal, B., Kumar, S., Singh, R. K. (2013). Production of hydrocarbon liquid by thermal pyrolysis of paper cup waste. Journal of Waste Management, 2013, 1–7. [Google Scholar]
8. Chen, J., Li, H., Fang, C., Cheng, Y., Tan, T. et al. (2020). Synthesis and structure of carboxymethylcellulose with a high degree of substitution derived from waste disposable paper cups. Carbohydrate Polymers, 237, 116040. [Google Scholar] [PubMed]
9. Khalid, H., Ikhlaq, A., Pervaiz, U., Wie, Y. M., Lee, E. J. et al. (2023). MunicipalWaste degradation by vermicomposting using a combination of eisenia fetida and lumbricus rubellus species. Agronomy, 13, 1370. https://doi.org/10.3390/agronomy13051370 [Google Scholar] [CrossRef]
10. Ghorpade, V. M., Gennadios, A., Hanna, M. A. (2001). Laboratory composting of extruded poly(lactic acid) sheets. Bioresources Technology, 76, 57–61. [Google Scholar]
11. Arumugam, K., Ganesan, S., Muthunarayanan, V., Vivek, S., Sugumar, S. et al. (2015). Potentiality of Eisenia fetida to degrade disposable paper cups–An ecofriendly solution to solid waste pollution. Environmental Science and Pollution Research International, 22(4), 2868–2876. [Google Scholar] [PubMed]
12. Muthunarayanan, V., Arumugam, K., Duraisam, T. (2016). Possibility of eco friendly post consumer waste management. Procedia Environmental Sciences, 35, 416–424. [Google Scholar]
13. Sharma, K., Garg, V. (2017). Comparative analysis of vermicompost quality produced from rice straw and paper waste employing earthworm Eisenia fetida (Sav.). Bioresources Technology, 250, 708–715. [Google Scholar]
14. Arumugam, K., Renganathan, S., Babalola, O. O., Muthunarayana, V. (2018). Investigation on paper cup waste degradation by bacterial consortium and Eudrillus eugeinea through vermicomposting. Waste Management, 74, 185–193. [Google Scholar] [PubMed]
15. Arumugam, K., Renganathan, S., Kasimani, R., Babalola, O. O., Muthunarayanan, V. (2017). The role of eudrillus eugenia in the degradation of paper cup waste and the morphological, physiological and histological changes in the organism. In: Prashanthi, M., Jeyaseelan, S., Kaliannan, T. (Eds.Bioremediation and sustainable technologies for cleaner environment, pp. 65–76. Cham: Springer International Publishing. [Google Scholar]
16. Singh, P., Jerram, E. M. (1976). Plastic damage by insects. New Zealand Entomologist, 6(2), 188. [Google Scholar]
17. Ali, S. S., Elsamahy, T., Al-Tohamy, R., Zhu, D., Mahmoud, Y. A. G. et al. (2021). Plastic wastes biodegradation: Mechanisms, challenges and future prospects. Science of the Total Environment, 780, 146590. [Google Scholar] [PubMed]
18. Folmer, O., Black, M., Hoeh, W., Lutz, R., Vrijenhoek, R. (1994). DNA primers for amplification of mitochondrial cytochrome c oxidase subunit I from diverse metazoan invertebrates. Molecular Marine Biology and Biotechnology, 3(5), 294–299. [Google Scholar] [PubMed]
19. Bombelli, P., Howe, C., Bertocchini, F. (2017). Polyethylene bio-degradation by caterpillars of the wax moth Galleria mellonella. Current Biology, 27, R283–R293. [Google Scholar]
20. Weber, C., Pusch, S., Opatz, T. (2017). Polyethylene bio-degradation by caterpillars? Current Biology, 27(15), R744–R745. [Google Scholar] [PubMed]
21. LeMoine, C., Grove, H., Smith, C., Cassone, B. (2020). A very hungry caterpillar: Polyethylene metabolism and lipid homeostasis in larvae of the greater wax moth (Galleria mellonella). Environmental Science & Technology, 54(22), 14706–14715. [Google Scholar]
22. Cassone, B. J., Grove, H., Elebute, O., Villanueva, S., LeMoine, C. (2020). Role of the intestinal microbiome in low-density polyethylene degradation by caterpillar larvae of the greater wax moth, Galleria mellonella. Proceedings of the Royal Society B, 287(1922), 20200112. [Google Scholar] [PubMed]
23. Zhu, P., Pan, X., Li, X., Liu, X., Liu, Q. et al. (2021). Biodegradation of plastics from waste electrical and electronic equipment by greater wax moth larvae (Galleria mellonella). Journal of Cleaner Production, 310, 127346. [Google Scholar]
24. Billen, P., Khalifa, L., van Gerven, F., Tavernier, S., Spatari, S. (2020). Technological application potential of polyethylene and polystyrene biodegradation by macro-organisms such as mealworms and wax moth larvae. Science of the Total Environment, 735, 139521. [Google Scholar] [PubMed]
25. Kong, H., Kim, H., Chung, J., Jun, J., Lee, S. et al. (2019). The Galleria mellonella hologenome supports microbiota-independent metabolism of long-chain hydrocarbon beeswax. Cell Reports, 26(9), 2451–2464. [Google Scholar] [PubMed]
26. Zhang, J., Gao, D., Li, Q., Zhao, Y., Li, L. et al. (2020). Biodegradation of polyethylene microplastic particles by the fungus Aspergillus flavus from the guts of wax moth Galleria mellonella. Science of the Total Environment, 704, 135931. [Google Scholar] [PubMed]
27. Lou, Y., Ekaterina, P., Yang, S., Lu, B., Liu, B. et al. (2020). Biodegradation of polyethylene and polystyrene by greater wax moth larvae (Galleria mellonella L.) and the effect of co-diet supplementation on the core gut microbiome. Environmental Science & Technology, 54(5), 2821–2831. [Google Scholar]
28. Elmekawy, A., Elshehaby, M., Saber, S., Ayaad, T. (2023). Evaluation of Galleria mellonella immune response as a key step toward plastic degradation. The Journal of Basic and Applied Zoology, 84, 27. https://doi.org/10.1186/s41936-023-00349-3 [Google Scholar] [CrossRef]
29. Vasileva, A. V., Medvedeva, I. V., Kostyukova, N. M., Nikitin, O., Latypova, V. et al. (2020). Comparative analysis of biodamage to various types of polyethylene by Galleria mellonella (Insecta, Lepidoptera, Pyralidae) larvae. Biology Bulletin, 47, 1254–1259. https://doi.org/10.1134/S1062359020100258 [Google Scholar] [CrossRef]
30. Burd, B. S., Mussagy, C. U., de Lacorte Singulani, J., Tanaka, J., Scontri, M. et al. (2023). Galleria mellonella larvae as an alternative to low-density polyethylene and polystyrene biodegradation. Journal of Polymeras and the Environment, 31, 1232–1241. https://doi.org/10.1007/s10924-022-02696-8 [Google Scholar] [CrossRef]
31. Noël, G., Serteyn, L., Sare, A. R., Massart, S., Delvigne, F. et al. (2023). Co-diet supplementation of low density polyethylene and honeybee wax did not influence the core gut bacteria and associated enzymes of Galleria mellonella larvae (Lepidoptera: Pyralidae). International Microbiology, 26, 397–409. https://doi.org/10.1007/s10123-022-00303-3 [Google Scholar] [PubMed] [CrossRef]
32. Jorjão, A., Oliveira, L., Scorzoni, L., Figueiredo-Godoi, L., Prata, M. et al. (2018). From moths to caterpillars: Ideal conditions for Galleria mellonella rearing for in vivo microbiological studies. Virulence, 9(1), 383–389. [Google Scholar]
33. Pereira, M., Rossi, C. (2020). Overview of rearing and testing conditions and a guide for optimizing Galleria mellonella breeding and use in the laboratory for scientific purposes. Journal of Pathology, Microbiology and Immunology, 128, 607–620. [Google Scholar]
34. Zaitoun, S. (2007). The effect of different Mediterranean plant extracts on the development of the great wax moth Galleria mellonella L. (Lepidoptera: Pyralidae) and their toxicity to worker honeybees Apis mellifera L. (Hymenoptera: Apidae) under laboratory conditions. Journal of Food, Agriculture & Environment, 5(2), 289–294. [Google Scholar]
35. Edwards, C. A. (1988). Breakdown of animal, vegetable and industrial organic wastes by earthworms. In: Edwards, C. A., Neuhauser, E. F. (Eds.Earthworms in waste and environmental management, pp. 21–31. The Hague: SPB Academic Publishing. [Google Scholar]
36. Garg, V. K., Gupta, R. (2011). Effect of temperature variations on vermicomposting of household solid waste and fecundity of Eisenia fetida. Bioremediation Journal, 15(3), 165–172. [Google Scholar]
37. Marston, N., Campbell, B., Boldt, P. (1975). Mass producing eggs of the greater wax moth. Washington DC: US Department of Agriculture. [Google Scholar]
38. Mohapatra, D., Sahoo, K., Sannigrahi (2019). Impact of Eisenia fetida populations on bio-conversion of paper mill solid waste. International Journal of Recycling of Organic Wastes in Agriculture, 8(Suppl 1), S189–S193. [Google Scholar]
Cite This Article
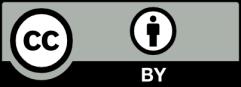