Open Access
ARTICLE
Health Risks Assessment of Heavy Metal Pollution in the Soil-Crop System from an E-Waste Dismantling Area
Key Laboratory of Soil Contamination Bioremediation of Zhejiang Province, Zhejiang Agriculture and Forestry University, Hangzhou, 311300, China
* Corresponding Author: Keli Zhao. Email:
(This article belongs to the Special Issue: The Effect of Soil Quality Degradation on the Plant Growth, Quality and Food Safety in Subtropical Agroforestry Ecosystems)
Phyton-International Journal of Experimental Botany 2022, 91(12), 2669-2685. https://doi.org/10.32604/phyton.2022.022416
Received 09 March 2022; Accepted 12 April 2022; Issue published 29 August 2022
Abstract
Soil is an essential resource for agricultural production. In order to investigate the pollution situation of heavy metals in the soil-crop system in the e-waste dismantling area, the crop and soil samples (226 pairs, including leaf vegetables, solanaceous vegetables, root vegetables, and fruits) around the e-waste dismantling area in southeastern Zhejiang Province were collected. The concentrations of Cd, Cu, Pb, and Cr were determined. The average concentrations of Cd, Cu, Pb, and Cr in soils were 0.94, 107.79, 80.28, and 78.14 mg kg-1, respectively, and their corresponding concentrations in crops were 0.024, 0.7, 0.041, and 0.06 mg kg-1, respectively. The transfer capacity of leaf vegetables was significantly higher than that of non-leaf vegetables, and the accumulation of four heavy metals in crops tended to be Cd > Cu > Cr/Pb. The pollution index’s results revealed that the soil pollution degree under different land uses ranked as root vegetables soil > leaf vegetables soil > solanaceous vegetables soil > fruit soil. The carcinogenic and non-carcinogenic risks of heavy metal exposure were ranked as food intake > accidental ingestion > dermal contact > inhalation. The comprehensive non-carcinogenic risk was ranked as Cr > Cd > Pb/Cu. Our results could be used to provide useful information for further crop cultivation layout in the study area, which can guarantee the local residents’ health and food safety.
Keywords
As the basis of agricultural production and human survival, the soil plays a vital role in food and ecological security [1]. Up to now, soil has been severely contaminated by heavy metals, which have bioaccumulative and biotoxic features [2–4]. Heavy metals absorbed by the root can enter and accumulate in the plant tissues, affect food security and thus human health through the food chain [5]. Classified as pollutants of great priority by the United States Environmental Protection Agency (USEPA), Cd, Cr, Cu and Pb are the most representative and can seriously endanger the safety of human health [6,7]. For instance, high doses of Pb have the potential to harm blood enzymes and alter the central nervous system, while Cr could influence the normal function of the liver, kidneys and other organs [8–10].
With the tremendous advancement of science and technology in recent years, the lifespan of electronic and electrical products has been shorter, thus forming a large amount of electronic waste. Electronic waste (e-waste) refers to discarded electronic products, consisting of all components and consumables that are part of the electronic products when they are disposed of [11]. Notably, nowadays e-waste is becoming an increasingly prominent source of heavy metals during dismantling and incineration [12–15]. According to a recent study, 53.6 × 106 metric tons of e-waste was generated in 2019, but most of it was exported illegally to developing countries [11]. However, China is one of the major recipients of e-waste all over the world, while it has been the highest generator of e-waste in the world (7.2 Mt) since 2017. Therefore, China is now facing a severe challenge of e-waste disposal. Most of the recycling and dismantling workshops were located in Guiyu and Taizhou, where numerous house-hold workshops are engaged in the business of recycling or dismantling [16]. Informal processing and recycling of e-waste such as strong acid leaching and the open burning of dismantled components, has contributed to the release of a large number of heavy metals into the surrounding environment hazardous to human health [17,18]. Studies showed that Chinese workers located in e-waste dismantling areas were more likely to suffer from blood and kidney diseases as well as respiratory, neurological, and immune system disorders [19]. Despite the pollution control policies (PCP) that have been implemented to curb metal contamination since 2012, the concentration of heavy metals like Cd, Cu, Ni, and Zn in soils had no noticeable change, causing persistent ecological and health safety problems [7]. Thus, based on the current situation that the study site was heavily contaminated and difficult to control, it was necessary to investigate the contamination characteristics and evaluate the ecological and health risks.
Most of the related research focused on the pollution characteristics and health risks of rice and soil system surrounding the study area [20–23], but very few studies have examined vegetables and fruit. However, with the change of people’s diet structure, people’s intake of fruits is also increasing as well as vegetables. Thus, this study will indicate the pollution characteristics and health risks of the soil-crop system (vegetables and fruits) around e-waste dismantling areas. The main objectives of this study were: (1) To identify contamination characteristics and ecological risks of four heavy metals (Cd, Cr, Cu, and Pb) in the soil samples around the study area. (2) To reveal the transfer characteristics of these four heavy metals in different crops. (3) To evaluate the carcinogenic and non-carcinogenic health risks of the metals through different exposure pathways.
The study area is located in Luqiao District, Taizhou City, in the southeastern part of Zhejiang Province, China (121°13’ to 121°40’E, 28°20’ to 28°38’N, Fig. 1), which has a subtropical marine monsoon climate with an average annual temperature of 17.6°C, ranging from –5.3°C to 36.8°C. The main crops in this area are rice, vegetables, and fruits.
Figure 1: Study area
The e-waste recycling industry in the region has highly developed since the 1990s, with numerous home-based e-waste disposal workshops and ubiquitous informal dismantling activities [24,25]. Previous studies reported that there were 30 villages and 384 households that were heavily involved in unauthorized e-waste handling activities, only in the Wenqiao Town of Wenling City [26]. Under the key control measurement of the government, it was listed as one of six priority control areas, meaning that it played an essential role in preventing and remediating polluted soils across China [7].
226 soil samples (0–20 cm) and their corresponding crop samples were collected based on a grid of 100 m × 100 m in this study, including 51 leaf vegetables (LV, including chard, amaranth, caper, hollow cabbage, mullein, green vegetables, lettuce, and fava leaves), 93 solanaceous vegetables (SV, including cauliflower, winter squash, red bean, cucumber, cucumber, cowpea, pepper, pumpkin, eggplant, loofah, string bean, tomato, and corn), 6 root vegetables (RV, including green onion and lotus root) and 76 fruits (orange, pear, grape, peach, and watermelon).
The samples of soils were air-dried and processed in an agate-made mortar to pass through 60 meshes sieves for available state and 100 meshes sieves for total-volume, respectively [27]; The samples of vegetables were washed away sediment and other adherents using deionized water and dried until free of water, then crushed, and mixed well for analysis [21,28,29]; The soil-water ratio of 1:2.5 was adopted to determine the soil pH [30]; The soil and vegetable samples were digested with HNO3–H2O2–HF and then determined the content of Cr, Pb, Cr, and Cu through inductively coupled plasma mass spectrometry (ICP-MS, USA) [15]. To ensure accuracy, all samples were repeated and blank samples were added to keep the relative differences within 10% and the spiked recoveries within 95%–110% [31].
2.4 Environmental and Risk Assessment
The single factor pollution index evaluation method was commonly used to evaluate the degree of soil contamination [32] (Formula-1), while the potential Ecological Risk (RI) is one of the indexes adopted by many scholars at home and abroad to assess heavy metal pollution (Formulas 2–3). Detailed description related to the formulas can be found in previous studies [32–34].
As an effective quantitative strategy to determining the human risks of multiple exposure pathways (hand-to-mouth intake, dermal contact, and inhalation), the health risk assessment model (USEPA, 2009, 2011) divides human risks into carcinogenic and non-carcinogenic risks for separate analysis, calculating ADD (chronic daily intake of heavy metals) under different exposure routes [35] (Formulas 4–8, Table S1). When there are numerous heavy metal pollutants exposed through different pathways, the risks can be added together to calculate the combined carcinogenic risk (CR) and non-carcinogenic health risk (HI). The formula for its calculation and detailed meaning can be found in these researches [36–39].
In this study, descriptive statistics, one-way ANOVA, and cluster analysis of data were performed by SPSS 21.0 software. One-way analysis of variance for total metal concentrations in soil among different crops with Duncan’s multiple comparison was performed (P < 0.05). Microsoft Office Excel 2017 software was used for the calculation of environmental risk evaluation index and health risk evaluation. All graphs were delineated by Origin 9.0 and Arc GIS 10.7 [20].
3.1 Heavy-Metal Contents in Soil
The average contents of Cd, Cu, Pb, and Cr in soils were 0.94, 107.79, 80.28, and 78.14 mg kg-1 (Table 1), respectively. The Cd and Cu contents in the soils were mostly greater than the background values of Zhejiang Province and screening values of soil pollution risk in agricultural land of China, which indicates that the Cd and Cu accumulate heavily in the soils around the e-waste dismantling area. Qing et al. [40] found that the farmland soils closed to an e-waste dismantling plant were mainly contaminated by Cd, followed by Cu, which was in line with our findings. Chen et al. [41] evaluated the soil and road dust samples from Guiyu, indicated that the concentrations of Pb and Cd were 448.73 and 0.71 mg kg-1, which were significantly higher (P ≤ 0.05) than the reference area. Shi et al. [13] also found that the paddy soils from the e-waste dismantling area were contaminated with Cd and Cu.
According to Zhao et al. [10], the coefficient of variation (CV) is commonly applied to measure global variability. Yekeen et al. [42] also reported that the variable had a weak variability, if the CV value is <10%, while it stands for an extensive variability if the value was >90%. The data (Table 1) shows that the CV of Cd in the soil is 117%, indicating that the content of Cd in the soils is strongly influenced by human activities. Meanwhile, the CV of Cu, Pb, and Cr were 72%, 71%, and 28%, belonging to moderate variability. Baltas et al. [1] showed the CV of Cr, Cu, and Pb in soils of Sinop Province in Iran was 65.74%, 39.22%, and 33.10%, respectively, reflecting that the heavy metals in this area were mainly influenced by natural factor rather than human activities. The differences between the above results are due to the different levels of anthropogenic activities, implying a higher anthropogenic input of metals in our study area because of the e-waste dismantling activities [43].
3.2 Heavy-Metal Contents in Crops
The average contents (mg kg-1) of Cd, Pb, and Cu of different crops were followed as: leaf vegetables > root vegetables > solanaceous vegetables > fruits (Table 2). The contents of Cd, Pb and Cu in leaf and solanaceous vegetables were significantly higher than in other crops, which was consistent with the results reported by Liu et al. for the vegetables from an e-waste site [32]. Notably, concentrations of Cr in root vegetables were higher than in other crops due to their massive roots and strong active transport capacity. The contents of Cd surpassed the safety limit in most vegetables, while the contents of Pb in portions of the vegetables were exceeded, compared to China’s food safety limit standard (except for Cu, which has no standard). These results implied that local residents could be exposed to food safety concerns, which deserve further investigation. The heavy metal contents in different crops (Table 2) are not consistent with the corresponding contents in soil (Table 1). For example, the mean content of Cd in root vegetable soils was 17.3% higher than that in leaf vegetable soils, while the Cd content in root vegetables was 47.6% lower than that in leaf vegetable crops. These differences were correlated with the bioavailability of the metal and the transfer capacity of different crops [44,45].
3.3 Comparison of the Transfer Capacity of Heavy Metals among Crops
The transfer factor (TF) is the ratio of heavy metal content in crops to the corresponding heavy metal content in the soil, which reflects the absorption and transfer capacity of different crops for heavy metals [46]. The data (Table 3) showed the transfer capacity of different crops for the four heavy metals are ranked as follows: Cd > Cu > Pb/Cr, suggesting that the crops had larger transfer capacity for Cd, which was in line with the results of Fan et al. [21]. The TF of different crops for Cd are as follows: leaf vegetables (0.0955) > root vegetables (0.0496) > solanaceous vegetables (0.0252) > fruits (0.0120). In general, leaf vegetables have higher transfer capacity for heavy metals, compared with other crops. Studies indicated that the differences in metal accumulation capacity and soil characteristics may cause heavy metal transfer in different plant tissues. [23,47]. According to Salah et al. [48], transpiration plays an essential role in the transfer of heavy metals inside. Yu et al. [49] reported that heavy metal transport capacity of soil crop systems is synergistically influenced by crop genotypes and environmental factors, among which the soil physico-chemical properties play an essential role.
For transfer capacities of Cd, the differences among leaf vegetables, root vegetables, and fruits were significant (P < 0.05), but there was no significant difference between root vegetables and fruits (P > 0.05). For Cu, leaf vegetables were significantly higher than others. For the Pb, leaf vegetables and root vegetables were significantly different while solanaceous vegetables and fruits were not significantly different. For the Cr, leaf vegetables and root vegetables were significantly higher than solanaceous vegetables and fruits. In general, the transfer capacity of leaf vegetables was significantly higher compared to other crops for different heavy metals, while there was no significant difference between solanaceous vegetables and fruits.
Fig. 3 showed the transfer capacities of different types of crops for heavy metals. Combined with the mean values of TF of different crops for different heavy metals (Fig. 2), solanaceous vegetables and fruits have the lowest transfer capacity for Cd and Cu, root vegetables have medium transfer capacity, and leaf vegetables have the highest transfer capacity. For Pb, leaf vegetables have higher transfer capacity, and solanaceous vegetable fruit and root vegetables have lower transfer capacity. For Cr, the first group consisted of solanaceous vegetables, while the second group of root vegetables and the third group of leaf crops were high transfer crops. Leaf vegetables have a higher transfer capacity, which is likely due to a closer distance from the leaf to its root. Therefore, once the root absorbs the heavy metals, they can fast transport to the leaves.
Figure 2: The difference of transfer ability for heavy metals of crops
Figure 3: Cluster analysis on transfer ability for heavy metals of different crops
3.4 Environmental Risk Assessment
Based on the background value of soil elements in Zhejiang Province, the single-factor pollution index indicated that the soils in the study area have been contaminated by different heavy metals to varying degrees. As shown in Table 4, Cd is heavily accumulated (7.22). Cu is moderately accumulated (3.53). Pb is lightly accumulated (2.64). Based on the risk screening value of soil elements, the results showed that PCd, PCu, PPb, and PCr were 3.13, 2.16, 0.89, and 0.52, which indicated that the Cd and Cu caused moderate and light pollution in the study area.
The PCd of crops was greater than 3.0, which belonged to moderate pollution and above (Fig. 4). The PCu of crops was greater than 2.0 (total percentage > 70%), which was light pollution and above. The PPb of crops was greater than 2.0 (total percentage > 50%), which was light pollution and above. The PCr of crops was less than 1.0 (total percentage > 67%). Therefore, Cd caused the most serious contamination of the soil in the study area, followed by Cu and Pb.
Figure 4: Single-factor pollution index ratio of heavy metals in soils of different crops/%
Table 5 showed the mean values of the four heavy metals’ ecological risks in the soil of various crops. The ECd of leaf vegetables (254.14), root vegetables (214.27), solanaceous vegetables (298.23), and fruits (188.25) have high potential ecological risks. In the contrast, the ECu, EPb ECr of crops were less than 30, belonging to slight ecological risks.
The carcinogenic risk of several heavy metals in various crops was much higher in youngsters than in adults (Table 6). This finding might be explained by children’s unique behaviors, such as frequent pica, finger or hand sucking, and rapid breathing [50]. The carcinogenic risk ranks of the different crops were as follows: root vegetables > leaf vegetables > solanaceous vegetables> fruits. According to Kan et al. [51], CR below 10-6 indicates that there are no health risks; CR ranging between 10-6 and 10-4 is interpreted as acceptable, and CR greater than 10-4 is considered unacceptable. The CRs of Cd and Cr for adults and children through accidental ingestion range from 10-6 to 10-4, implying the carcinogenic risks from soil Cd and Cr in the study area were acceptable. The carcinogenic health risks produced by the various exposure routes for children and adults were ranked in order: food intake > accidental ingestion > dermal contact > inhalation, which was consistent with Wei’s results [52]. The results indicated that children suffer a greater risk of cancer than adults. The main reason for this phenomenon is that children are most likely to ingest metals through their hands and mouths [53]. The combined carcinogenic risk of different types of crops and their soils is from leaf vegetables > rootstocks > solanaceous vegetables > fruits and the combined carcinogenic risk of different heavy metals is ranked as Cd > Cr (Fig. 5). Comparable studies have been carried out in regions where e-waste was being dismantled, and similar tendencies for carcinogenic risk have been discovered [41,54,55].
Figure 5: Comprehensive carcinogenic risk of heavy metals in soil-crops
3.6 Non-Carcinogenic Health Risk
For children, their non-carcinogenic risk assessment of heavy metals was both significantly greater than those for adults, implying that the same contaminants were more likely to cause non-carcinogenic hazards for children under the same exposure pathway (Table 7). In addition, the non-carcinogenic risk is related to the exposure pathway, which showed food intake > accidental ingestion > dermal contact > inhalation. Zhang et al. [52] found that in terms of heavy metals exposure to street dusts, the non-carcinogenic health risk for adults was lower than for children. The hazard quotient (HQ) of Cd, Cr in the leaf vegetables and root vegetables for children was higher than 1, found that Cd and Cr were the major elements that posed a health risk to local residents, and they were the key elements that posed a health risk to local residents. Similar results indicated that the HQ total even ranked in the order of Cu > Cd ≈ Pb > Zn [17]. Additionally, the combined non-carcinogenic risk of leaf vegetables and root vegetables was higher than those of solanaceous vegetables (Fig. 6). Also, previous studies had shown that the risks caused by intake of leaf vegetables were much higher than those fruit vegetable intake [53].
Figure 6: Comprehensive non-carcinogenic risk of heavy metals in soil-crops
This research revealed the contamination status of Cd, Pb, Cu, and Cr in the soil-crop system. The study area has been polluted by Cd, Pb, Cu, and Cr as a result of irrational e-waste dismantling activities, especially for Cd. Leaf vegetables suffer more serious heavy metal pollution in the area, which may be due to their high transfer capabilities. The results of the health risk assessment showed that residents in the area faced potential health hazards. Therefore, it is suggested that decrease the intake of these toxic elements and decrease planting high accumulators like leaf vegetables in heavy metal polluted farmland to reduce heavy metal accumulation in crops. Local governments must closely monitor the situation and take steps to address e-waste-related pollution, then ensure that local residents can safely consume the agricultural product.
Acknowledgement: We thank Luyao Zhang for her help in the laboratory analysis and the figure production. Particularly thanks to Prof. Fu for his supervision to our study.
Authorship: The authors confirm contribution to the paper as follows: Rao S.T. contributed to the experiments, data analysis and manuscript writing. Fang J. was responsible for the data analysis and manuscript writing. Zhao K.L. supervised this work and edited this manuscript. All authors read and approved the final manuscript.
Funding Statement: This work was financially supported by the National College Students’ Innovation and Entrepreneurship Training Program (202110341014).
Conflicts of Interest: The authors declare that they have no conflicts of interest to report regarding the present study.
References
1. Baltas, H., Sirin, M., Gökbayrak, E., Ozcelik, A. E. (2020). A case study on pollution and a human health risk assessment of heavy metals in agricultural soils around Sinop Province, Turkey. Chemosphere, 241(11), 125015. DOI 10.1016/j.chemosphere.2019.125015. [Google Scholar] [CrossRef]
2. Hussain, N., Shafiq Ahmed, K., Asmatullah, Ahmed, M. S., Hussain, S. M. et al. (2021). Potential health risks assessment cognate with selected heavy metals contents in some vegetables grown with four different irrigation sources near Lahore, Pakistan. Saudi Journal of Biological Sciences, 29, 181–1824. DOI 10.1016/j.sjbs.2021.10.043. [Google Scholar] [CrossRef]
3. Cui, Y. B., Li, B., Li, C. H., He, Z. J., Liu, X. R. (2022). Assessment of heavy metal contamination levels and health risks in environmental media in the northeast region. Sustainable Cities and Society, 80(1), 103796. DOI 10.1016/j.scs.2022.103796. [Google Scholar] [CrossRef]
4. Fu, W. J., Dong, J. Q., Ding, L. Z., Yang, H. S., Ye, Z. Q. et al. (2021). Spatial correlation of nutrients in a typical soil-hickory system of Southeastern China and its implication for site-specific fertilizer application. Soil and Tillage Research, 217(2), 105265. DOI 10.1016/j.still.2021.105265. [Google Scholar] [CrossRef]
5. Luo, C. L., Liu, C. P., Wang, Y., Liu, X., Li, F. B. et al. (2011). Heavy metal contamination in soils and vegetables near an e-waste processing site, South China. Journal of Hazardous Materials, 186(1), 481–490. DOI 10.1016/j.jhazmat.2010.11.024. [Google Scholar] [CrossRef]
6. Zerizghi, T., Guo, Q. J., Tian, L. Y., Wei, R. F., Zhao, C. Q. (2022). An integrated approach to quantify ecological and human health risks of soil heavy metal contamination around coal mining area. Science of the Total Environment, 814(27), 152653. DOI 10.1016/j.scitotenv.2021.152653. [Google Scholar] [CrossRef]
7. Yang, S. Y., Gu, S. B., He, M. J., Tang, X. J., Ma, L. Q. et al. (2020). Policy adjustment impacts Cd, Cu, Ni, Pb and Zn contamination in soils around E-waste area: Concentrations, sources and health risks. Science of the Total Environment, 741(2), 140442. DOI 10.1016/j.scitotenv.2020.140442. [Google Scholar] [CrossRef]
8. Kaufmann, R. B., Staes, C. J., Matte, T. D. (2003). Deaths related to lead poisoning in the United States, 1979–1998. Environmental Research, 91(2), 78–84. DOI 10.1016/S0013-9351(02)00017-8. [Google Scholar] [CrossRef]
9. Dutta, D., Goel, S., Kumar, S. (2022). Health risk assessment for exposure to heavy metals in soils in and around E-waste dumping site. Journal of Environmental Chemical Engineering, 10(2), 107269. DOI 10.1016/j.jece.2022.107269. [Google Scholar] [CrossRef]
10. Zhao, K. L., Zhang, L. Y., Dong, J. Q., Wu, J. S., Ye, Z. Q. et al. (2020). Risk assessment, spatial patterns and source apportionment of soil heavy metals in a typical Chinese hickory plantation region of Southeastern China. Geoderma, 360(1–4), 114011. DOI 10.1016/j.geoderma.2019.114011. [Google Scholar] [CrossRef]
11. Joo, J., Kwon, E. E., Lee, J. (2021). Achievements in pyrolysis process in E-waste management sector. Environmental Pollution, 287, 117621. DOI 10.1016/j.envpol.2021.117621. [Google Scholar] [CrossRef]
12. Mowla, M., Rahman, E., Islam, N., Aich, N. (2021). Assessment of heavy metal contamination and health risk from indoor dust and air of informal E-waste recycling shops in Dhaka. Bangladesh Journal of Hazardous Materials Advances, 4(12), 100025. DOI 10.1016/j.hazadv.2021.100025. [Google Scholar] [CrossRef]
13. Shi, A., Shao, Y. F., Zhao, K. L., Fu, W. J. (2020). Long-term effect of E-waste dismantling activities on the heavy metals pollution in paddy soils of Southeastern China. Science of the Total Environment, 705, 135971. DOI 10.1016/j.scitotenv.2019.135971. [Google Scholar] [CrossRef]
14. Zhao, K. L., Fu, W. J., Qiu, Q. Z., Ye, Z. Q., Li, Y. F. et al. (2019). Spatial patterns of potentially hazardous metals in paddy soils in a typical electrical waste dismantling area and their pollution characteristics. Geoderma, 337(2–3), 453–462. DOI 10.1016/j.geoderma.2018.10.004. [Google Scholar] [CrossRef]
15. Joo, J., Kwon, E. E., Lee, J. (2021). Achievements in pyrolysis process in e-waste management sector. Environmental Pollution, 287, 17621. DOI 10.1016/j.envpol.2021.117621. [Google Scholar] [CrossRef]
16. Meng, M., Yang, L. S., Wei, B. G., Cao, Z. Q., Yu, J. P. et al. (2021). Plastic shed production system: The migration of heavy metals from soil to vegetables and human health risk assessment. Ecotoxicology and Environmental Safety, 215(10), 112106. DOI 10.1016/j.ecoenv.2021.112106. [Google Scholar] [CrossRef]
17. Chan, J. K. Y., Man, Y. B., Wu, S. C., Wong, M. H. (2013). Dietary intake of PBDEs of residents at two major electronic waste recycling sites in China. Science of the Total Environment, 463–464, 1138–1146. DOI 10.1016/j.scitotenv.2012.06.093. [Google Scholar] [CrossRef]
18. Zhang, T., Ruan, J. J., Zhang, B., Lu, S. Y., Gao, C. Z. et al. (2018). Heavy metals in human urine, foods and drinking water from an e-waste dismantling area: Identification of exposure sources and metal-induced health risk. Ecotoxicology and Environmental Safety, 169, 707–713. DOI 10.1016/j.ecoenv.2018.10.039. [Google Scholar] [CrossRef]
19. Tostar, S., Stenvall, E., Boldizar, A., Foreman, M. R. S. J. (2013). Antimony leaching in plastics from waste electrical and electronic equipment (WEEE) with various acids and gamma irradiation. Waste Management, 33(6), 1478–1482. DOI 10.1016/j.wasman.2013.03.002. [Google Scholar] [CrossRef]
20. Li, W. L., Achal, V. (2020). Environmental and health impacts due to e-waste disposal in China–A review. Science of the Total Environment, 737(2), 139745. DOI 10.1016/j.scitotenv.2020.139745. [Google Scholar] [CrossRef]
21. Hossain, M. M., Chowdhury, M. A., Hossain, M. J., Rashid, H. A., Uddin, N. et al. (2021). Heavy metal pollution in the soil-vegetable system of Tannery Estate. Environmental Nanotechnology, Monitoring & Managemen, 16, 100557. DOI 10.1016/j.enmm.2021.100557. [Google Scholar] [CrossRef]
22. Fan, Y., Li, H., Xue, Z. J., Zhang, Q., Cheng, F. Q. (2017). Accumulation characteristics and potential risk of heavy metals in soil-vegetable system under greenhouse cultivation condition in Northern China. Ecological Engineering, 102(18), 367–373. DOI 10.1016/j.ecoleng.2017.02.032. [Google Scholar] [CrossRef]
23. Baruah, S. G., Ahmed, I., Dasa, B., Ingtipi, B., Boruah, H. et al. (2021). Heavy metal (loid)s contamination and health risk assessment of soil-rice system in rural and peri-urban areas of lower brahmaputra valley, Northeast India. Chemosphere, 266(1), 129150. DOI 10.1016/j.chemosphere.2020.129150. [Google Scholar] [CrossRef]
24. Wang, J., Su, J. W., Li, Z. G., Liu, B. X., Cheng, G. H. et al. (2019). Source apportionment of heavy metal and their health risks in soil-dustfall-plant system nearby a typical non-ferrous metal mining area of Tongling, Eastern China. Environmental Pollution, 254, 113089. DOI 10.1016/j.envpol.2019.113089. [Google Scholar] [CrossRef]
25. Dong, J., Zhou, K., Jiang, P., Wu, J., Fu, W. (2021). Revealing horizontal and vertical variation of soil organic carbon, soil total nitrogen and C:N ratio in subtropical forests of Southeastern China. Journal of Environmental Management, 289(8), 112483. DOI 10.1016/j.jenvman.2021.112483. [Google Scholar] [CrossRef]
26. Zhao, K. L., Fu, W. J., Liu, X. M., Huang, D. L., Zhang, C. S. et al. (2014). Spatial variations of concentrations of copper and its speciation in the soil-rice system in Wenling of Southeastern China. Environmental Science and Pollution Research, 21(11), 7165–7176. DOI 10.1007/s11356-014-2638-9. [Google Scholar] [CrossRef]
27. Chi, X. W., Wang, M. Y. L., Reuter, M. A. (2014). E-waste collection channels and household recycling behaviors in Taizhou of China. Journal of Cleaner Production, 80(1), 87–95. DOI 10.1016/j.jclepro.2014.05.056. [Google Scholar] [CrossRef]
28. Affum, A. O., Osae, S. D., Kwaansa-Ansah, E. E., Miyittah, M. K. (2020). Quality assessment and potential health risk of heavy metals in leafy and non-leafy vegetables irrigated with groundwater and municipal-waste-dominated stream in the Western Region, Ghana. Heliyon, 6(12), e05829. DOI 10.1016/j.heliyon.2020.e05829. [Google Scholar] [CrossRef]
29. Tomno, R. M., Nzeve, J. K., Mailu, S. N., Shitanda, D., Waswa, F. (2020). Heavy metal contamination of water, soil and vegetables in urban streams in Machakos municipality, Kenya. Scientific African, 9(1), e00539. DOI 10.1016/j.sciaf.2020.e00539. [Google Scholar] [CrossRef]
30. Guadie, A., Yesigat, A., Gatew, S., Workuc, A., Liu, W. Z. et al. (2021). Evaluating the health risks of heavy metals from vegetables grown on soil irrigated with untreated and treated wastewater in Arba Minch, Ethiopia. Science of the Total Environment, 761, 143302. DOI 10.1016/j.scitotenv.2020.143302. [Google Scholar] [CrossRef]
31. Xu, C. B., Qi, J., Yang, W. J., Chen, Y., Yang, C. et al. (2019). Immobilization of heavy metals in vegetable-growing soils using nano zero-valent iron modified attapulgite clay. Science of the Total Environment, 686(51), 476–483. DOI 10.1016/j.scitotenv.2019.05.330. [Google Scholar] [CrossRef]
32. Liu, X. M., Gu, S. B., Yang, S. Y., Deng, J. S., Xu, J. M. (2021). Heavy metals in soil-vegetable system around E-waste site and the health risk assessment. Science of the Total Environment, 779(25), 146438. DOI 10.1016/j.scitotenv.2021.146438. [Google Scholar] [CrossRef]
33. Sawut, R., Kasim, N., Maihemuti, B., Hu, L., Abliz, A. et al. (2019). Pollution characteristics and health risk assessment of heavy metals in the vegetable bases of Northwest China. Science of the Total Environment, 642(1), 864–878. DOI 10.1016/j.scitotenv.2018.06.034. [Google Scholar] [CrossRef]
34. Hakanson, L. (1980). An ecological risk index for aquatic pollution control. A sedimentological approach. Water Research, 14(8), 975–1001. DOI 10.1016/0043-1354(80)90143-8. [Google Scholar] [CrossRef]
35. Adedeji, O. H., Olayinka, O. O., Tope-Ajayi, O. O., Adekoya, A. S. (2020). Assessing spatial distribution, potential ecological and human health risks of soil heavy metals contamination around a Trailer Park in Nigeria. Scientific African, 10(22), e00650. DOI 10.1016/j.sciaf.2020.e00650. [Google Scholar] [CrossRef]
36. Jin, J., Wang, L., Muller, K., Wu, J., Wang, H. et al. (2021). A 10-year monitoring of soil properties dynamics and soil fertility evaluation in Chinese hickory plantation regions of Southeastern China. Scientific Reports, 11(1), 23531. DOI 10.1038/s41598-021-02947-z. [Google Scholar] [CrossRef]
37. Zheng, S. N., Wang, Q., Yuan, Y. Z., Sun, W. M. (2020). Human health risk assessment of heavy metals in soil and food crops in the Pearl River Delta urban agglomeration of China. Food Chemistry, 316(1–3), 126213. DOI 10.1016/j.foodchem.2020.126213. [Google Scholar] [CrossRef]
38. Li, N., Kang, Y., Pan, W. J., Zeng, L. X., Zhang, Q. Y. et al. (2015). Concentration and transportation of heavy metals in vegetables and risk assessment of human exposure to bioaccessible heavy metals in soil near a waste-incinerator site, South China. Science of the Total Environment, 521–522, 144–151. DOI 10.1016/j.scitotenv.2015.03.081. [Google Scholar] [CrossRef]
39. Yang, L., Ren, Q., Zheng, K. X., Jiao, Z. Q., Ruan, X. L. et al. (2021). Migration of heavy metals in the soil-grape system and potential health risk assessment. Science of the Total Environment, 806(6), 150646. DOI 10.1016/j.scitotenv.2021.150646. [Google Scholar] [CrossRef]
40. Qing, X., Zong, Y. T., Lu, S. G. (2015). Assessment of heavy metal pollution and human health risk in urban soils of steel industrial city (AnshanLiaoning, Northeast China. Ecotoxicology and Environmental Safety, 120, 377–385. DOI 10.1016/j.ecoenv.2015.06.019. [Google Scholar] [CrossRef]
41. Chen, H. R., Wang, L., Hu, B. F., Xu, J. M., Liu, X. M. (2022). Potential driving forces and probabilistic health risks of heavy metal accumulation in the soils from an e-waste area, southeast China. Chemosphere, 289(8), 133182. DOI 10.1016/j.chemosphere.2021.133182. [Google Scholar] [CrossRef]
42. Yekeen, T. A., Xu, X. J., Zhang, Y. L., Wu, Y. S., Kim, S. (2016). Assessment of health risk of trace metal pollution in surface soil and road dust from e-waste recycling area in China. Environmental Science Pollution Research International, 23(17), 17511–17524. DOI 10.1007/s11356-016-6896-6. [Google Scholar] [CrossRef]
43. Fu, W. J., Jiang, P. K., Zhou, G. M., Zhao, K. L. (2014). Using Moran’s I and GIS to study the spatial pattern of forest litter carbon density in a subtropical region of Southeastern China. Biogeosciences, 11(8), 2401–2409. DOI 10.5194/bg-11-2401-2014. [Google Scholar] [CrossRef]
44. Manta, D. S., Angelone, M., Bellanca, A., Neri, R., Sprovieri, M. (2002). Heavy metals in urban soils: A case study from the city of Palermo (SicilyItaly. Science of the Total Environment, 300(1–3), 229–243. DOI 10.1016/S0048-9697(02)00273-5. [Google Scholar] [CrossRef]
45. Adamo, P., Iavazzo, P., Albanese, S., Agrelli, D., Vivo, B. D. et al. (2014). Bioavailability and soil-to-plant transfer factors as indicators of potentially toxic element contamination in agricultural soils. Science of the Total Environment, 500–501(3), 11–22. DOI 10.1016/j.scitotenv.2014.08.085. [Google Scholar] [CrossRef]
46. Zhang, J. R., Li, H. Z., Zhou, Y. Z., Dou, L., Cai, L. M. et al. (2018). Bioavailability and soil-to-crop transfer of heavy metals in farmland soils: A case study in the Pearl River Delta, South China. Environmental Pollution, 235(4), 710–719. DOI 10.1016/j.envpol.2017.12.106. [Google Scholar] [CrossRef]
47. Wan, M. X., Hu, W. Y., Wang, H. F., Tian, K., Huang, B. et al. (2021). Comprehensive assessment of heavy metal risk in soil-crop systems along the Yangtze River in Nanjing, Southeast China. Science of the Total Environment, 780, 146567. DOI 10.1016/j.scitotenv.2021.146567. [Google Scholar] [CrossRef]
48. Cao, C., Yang, Y., Kwan, M., Ma, Z., Karthikeyan, R. et al. (2022). Crop selection reduces potential heavy metal(loid)s health risk in wastewater contaminated agricultural soils. Science of the Total Environment, 819, 152502. DOI 10.1016/j.scitotenv.2021.152502. [Google Scholar] [CrossRef]
49. Yu, Z. Y., Dong, J. Q., Fu, W. J., Ye, Z. Q., Li, W. Y. et al. (2019). The transfer characteristics of potentially toxic trace elements in different soil-rice systems and their quantitative models in Southeastern China. International Journal of Environmental Research and Public Health, 16(14), 2503. DOI 10.3390/ijerph16142503. [Google Scholar] [CrossRef]
50. Wang, Y. C., Qiao, M., Liu, Y. X., Zhu, Y. G. (2012). Health risk assessment of heavy metals in soils and vegetables from wastewater irrigated area, Beijing-Tianjin city cluster, China. Journal of Environmental Science, 24(4), 690–698. DOI 10.1016/S1001-0742(11)60833-4. [Google Scholar] [CrossRef]
51. Kan, X. Q., Dong, Y. Q., Feng, L., Zhou, M., Hou, H. B. (2021). Contamination and health risk assessment of heavy metals in China’s lead-zinc mine tailings: A meta-analysis. Chemosphere, 267, 128909. DOI 10.1016/j.chemosphere.2020.128909. [Google Scholar] [CrossRef]
52. Zhang, W. H., Yan, Y., Yu, R. L., Hu, G. R. (2021). The sources-specific health risk assessment combined with APCS/MLR model for heavy metals in tea garden soils from South Fujian Province, China. Catena, 203, 105306. DOI 10.1016/j.catena.2021.105306. [Google Scholar] [CrossRef]
53. Wei, X., Gao, B., Wang, P., Zhou, H. D., Lu, J. (2015). Pollution characteristics and health risk assessment of heavy metals in street dusts from different functional areas in Beijing, China. Ecotoxicology and Environmental Safety, 112, 186–192. DOI 10.1016/j.ecoenv.2014.11.005. [Google Scholar] [CrossRef]
54. Kusin, F. M., Azani, N. N. M., Hasan, S. N. M. S., Sulong, N. A. (2018). Distribution of heavy metals and metalloid in surface sediments of heavily-mined area for bauxite ore in Pengerang, Malaysia and associated risk assessment. Catena, 165(3), 454–464. DOI 10.1016/j.catena.2018.02.029. [Google Scholar] [CrossRef]
55. Puangprasert, S., Prueksasit, T. (2019). Health risk assessment of airborne Cd, Cu, Ni and Pb for electronic waste dismantling workers in Buriram Province, Thailand. Journal of Environmental Management, 252, 109601. DOI 10.1016/j.jenvman.2019.109601. [Google Scholar] [CrossRef]
Appendix
Formula:
where Pi represents the single element pollution, Ci is the concentration of heavy metals in soil, Si is the standard concentration of heavy metals in soil.
where Ei is the risk index ( RI ) of metal; Ti is the toxic response factor of metal (TCd = 30, TCu = TPb = 5,TCr = 2); Ci is concentration of metal.
where ADD is the chronic daily intake of heavy metals; ADDing, ADDderm, ADDinh represent the average daily respective exposures of adults and children to heavy metal through the hand-to-mouth intake, dermal contact, and inhalation; C is the mean concentration of heavy metals in vegetables; IngR represents the daily intake of soil; InhR represents the daily intake of air; IR represents the daily intake of crops; EF is the exposure frequency; ED is exposure duration; AT represents the mean exposure time; BW is the mean body weight; RFD was the reference consumption of heavy metals.
where HI (Hazard Index) is the sum of HQ (hazard quotient) of each heavy metal and represents the total non-carcinogenic risk; CR (carcinogenic risk) is the total carcinogenic risk (USEPA, 2009, 2011).
Cite This Article
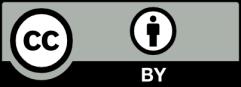