Open Access
ARTICLE
Exploration of Distinct Physiological and Biochemical Alterations in Chickpea (Cicer arietinum L.) under Varying Organic Materials, Drought and Proline Application
1 Department of Agronomy, Bangladesh Agricultural University, Mymensingh, 2202, Bangladesh
2 Department of Crop Botany, Bangladesh Agricultural University, Mymensingh, 2202, Bangladesh
3 Department of Biology, College of Science, Taif University, Taif, 21944, Saudi Arabia
4 Nature Conservation Sector, Egyptian Environmental Affairs Agency (EEAA), Cairo, 11435, Egypt
* Corresponding Author: Md. Romij Uddin. Email:
Phyton-International Journal of Experimental Botany 2022, 91(12), 2775-2789. https://doi.org/10.32604/phyton.2022.023112
Received 10 April 2022; Accepted 02 June 2022; Issue published 29 August 2022
Abstract
Chickpea yield is decreasing day by day due to drought stress, which could be an immense risk for future food security in developing countries. Management practices could be the most excellent approach to diminish loss due to this abiotic factor. The current research work was designed to explore the tolerance reaction of chickpea genotypes against management practices, through morphological and biochemical parameters and evaluate yield performance across drought prone location of Bangladesh. Four genotypes BD-6048, BD-6045, BD-6090, BD-6092 and eight management practices, e.g., severe water stress (SWS), i.e., without irrigation, 10 cm thick mulching with rice straw (MRS), 10 cm thick mulching with water hyacinth (MWH), organic amendment through compost (OAC) @ 3 t ha−1, organic amendment through cow dung @ 5 t ha−1 (OACD), organic amendment through poultry manure @ 2 t ha−1 (OAPM), inorganic amendment through proline application (IAPA) as foliar spray and 16 h hydro-priming (HP). The study revealed that the genotypes BD-6048 showed excellent performance because of the highest chlorophyll, carotenoids, phosphorus, potassium, proline and protein content. The highest pod number plant−1 also increased seed yield in BD-6048. Considering management practices, IAPA increased relative water content, carotenoids, leaf phosphorus and potassium compared to other management practices and severe water stress. Finally, BD-6084 was selected as best genotype because of a significant increase in chlorophyll a and b, carotenoids, and relative water content with IAPA. Identified top performing genotypes can be used for releasing variety and cultivated for sustainable production in drought prone area of Bangladesh.Keywords
Nomenclature
BARI | Bangladesh Agricultural Research Institute |
Chl a | Chlorophyll a |
Chl b | Chlorophyll b |
DAS | Days after sowing |
G | Genotypes |
K | Potassium |
HP | Hydro-priming |
IAPA | Inorganic amendments through proline application |
M | Management |
MoP | Muriate of potash |
MRS | Mulching with rice straw |
MWH | Mulching with water hyacinth |
OAC | Organic amendments through compost |
OACD | Organic amendments through cow dung |
OAPM | Organic amendments through poultry manure |
P | Phosphorus |
ROS | Relative oxygen species |
RWC | Relative water content |
SWS | Severe water stress |
TSP | Triple super phosphate |
Chickpea is a popular legume crop that provides millions of people throughout the world with a good amount of protein, fiber, and minerals [1]. Due to symbiotic association with rhizobacteria upon nodulation it also enriches the soil through nitrogen fixation. It is cultivated chiefly in marginal land and rainfed areas [2,3]. Moreover, chickpea is a winter crop and experiences terminal drought during maturity and seed filling stage as a result of reduced or no rainfall which results severe yield reductions. Chickpea plants also face drought stress when planted in spring instead of winter [4].
Significant climate phenomena and weather extremes have recently been reported in several places of the world. Heat waves have become more common in several countries of Asia, Europe, and Australia [5]. Furthermore, climate change is putting a lot of strain on the hydrological cycle [6]. Unprecedented climatic changes, leading in high world temperatures (heat stress) and unpredictable rainfall patterns (floods and drought) have become important difficulties for chickpea production in recent years [7]. Such climatic extremes have a negative impact on plant growth and, as a result, crop output, particularly in chickpea grain yield, where losses of up to 19 percent have been reported as a result of these conditions [8].
Drought is the most significant abiotic stress, producing an obstacle in several crops’ growth and production though out the world [9–13]. Its severity is forecasted to enhance in the coming days under changing climate [14,15]. Dry spell contains an impressive influence on seed germination, branching, growing, seed abdicate, and seed weight in the midst of the vegetative and regenerative stages of improvement [16]. During the vegetative stage, it negatively influences cell division and leaf expansion [16]. Several studies have exposed that drought stress reduces the absorption of nutrients content during the vegetative stage [17–19].
Despite the fact that chickpea is more resistant to water stress than other legumes, it is the most important factor in sinking the physiological and morphological properties [20]. The strength and time of stress determines plants’ responses to water stress. These responses are categorized in two types: firstly, water deficiency in low intensity, which in low intensity, which is known as moderate stress and directs to transpiration decline, interruption of water conduction from roots to shoots, and decreased photosynthetic pigments and products, which eventually lowers crop yields productivity and morphological characters [21]. Water stress has been shown to affect CO2 absorption rate and photochemical efficiency in a variety of plants, including cotton [22], tomato [23] and lentil [24]. Secondly, severe water scarcity is treated as extreme stress that produces reactive oxygen species (ROS) in plants, causing damage to the D1 protein in PSII, chlorophyll content, and electron transport [25]. Severe water stress causes significant changes in morphological characteristics, nutritional uptake, and antioxidant enzyme action as a result of reduced soil water absorption [26]. Chickpea plantings suffer severe production losses due to terminal drought stress in these conditions [27].
Based on plants-soil viewpoint, application of fertilizers can moderate the properties of drought on soil moisture and plant productivity [28,29]. The utilization of organic fertilizers supplies important nutrients and progress some soil properties like water and nutrient holding capacity and drought tolerance [30,31]; however, diverse categories of fertilizer donate differently. For example, vermicompost can alleviate organic waste. In that case, earthworms decompose organic material and are competent to renovate this material to wealthy nutrient composites that shape an environment in the soil and enhance plant growth [32]. Several studies revealed that soil amendment with organic material increases agricultural production by absorbing more nutrients, enhancing enzymatic activity, water holding and cation exchange capacity [32,33].
Generally, the literature only illustrated the individual result of drought and vermicompost but not the details on physiological and biochemical changes with different organic fertilizers and spray of proline and hydro priming. It was assumed that the physiological and biochemical characteristics would vary in genotypes with and without application of organic fertilizers. The study was aimed to validate the interactive effect of organic fertilizers and genotypes on physiological and biochemical traits along with yield of chickpea.
Vigorous and disease-free seeds of four chickpea genotypes were collected from Bangladesh Agricultural Research Institute (BARI), Gazipur, Bangladesh to perform the present study (Table 1).
We conducted the experiments at the research field of Rajshahi (drought prone area), Bangladesh during the period from November to June of two consecutive years 2018–2019 and 2019–2020. The treatment prepared with eight management practices as follows: severe water stress (SWS), i.e., without irrigation, 10 cm thick mulching with rice straw (MRS), 10 cm thick mulching with water hyacinth (MWH), organic amendment through compost (OAC) @ 3 t ha−1, organic amendment through cow dung @ 5 t ha−1 (OACD), organic amendment through poultry manure @ 2 t ha−1 (OAPM), inorganic amendment through proline application (IAPA) as foliar spray and 16 h hydro-priming (HP). The experiment was arranged in split plot design by assigning management practices in the main plot and genotype in the subplot with three replications. The individual plot size was 5 m × 4 m.
Urea, triple super phosphate (TSP), muriate of potash (MoP), gypsum and boron were used to fertilize the soil at the rate of 30, 80, 35, 50 and 7 kg ha−1, respectively [34]. The whole amounts of TSP, MoP, gypsum and boron were applied during final land preparation. Straight furrows of three to four cm deep were constructed with an iron rod popularly known as “tine”. Two seeds were sown per hill in the furrows at a seed rate of 40 kg ha−1 [34]. The distance between lines was 40 cm. The seeds were covered with loose soil after they were sown. Just after germination, MRS and MWH were applied. Fifteen days before sowing, OAC, OACD and OAPM were done. After seeding, proline was sprayed @ 250 ppm at 50 days after sowing (DAS). Before sowing, the seed was hydro-primed by soaking it in pure water and re-drying it to its original moisture content. Weeding was done by hand at 15 and 30 DAS. There was no noticeable infestation of insects, pests, or diseases in the experimental plots, no pest control measures were done for the crop.
2.4 Physiological Parameter Measurements
For adequate extraction of chlorophyll and carotenoids, fresh leaf samples of 50 mg were immersed in 10 mL of 80 percent acetone and stored in the dark for seven days. A UV–vis spectrophotometer was used to determine chlorophyll a (Chl a), chlorophyll b (Chl b), total chlorophyll (Chl a + Chl b), and total carotenoids content from the absorbance at 470, 648.6, and 664.2 nm using the Lichtenthaler technique [35].
For measuring relative water content (RWC), 100 mg fresh leaves were preserved in distilled water for 4 h. After blotting the excess water from the surface of the sample, the turgid weight was recorded. Dry weight was acquired by drying the samples in an oven at 70°C until they reached a consistent weight. The following formula was used to determine RWC:
2.5 Phosphorous and Potassium Determination
Jackson’s procedure [36] was utilized to assess chickpea leaf’s phosphorus (P) substance. In a 50 mL volumetric jar, 1 milliliter of liquor of plant diacid extricate was blended with 10 mL of vanadate molybdate reagent and after that weakened to 50 mL with refined water. After 30 min, the color was examined at 470 nm. The standard bend was utilized to compute the phosphorus concentrations in percent. The potassium (K) content of chickpea leaves was determined using the technique adopted by Chapman et al. [37]. The final concentration of the plant extract (diacid digested) was measured immediately on a flame photometer or after adequate dilution in a range of 0 to 50 mg potassium per litre. Simultaneously, a blank sans sample was run. The result was computed using a potassium solution standard perusing and communicated as percent potassium.
2.6 Biochemical Parameters Measurements
The substance of the proline was decided utilizing Bate’s strategy [38]. An aliquot of new green chickpea leaf was homogenized in 10 mL of 3 percent sulphosalicylic acid, at that point centrifuged for 15 min at 5000 rpm. 2 milliliters of the supernatant were combined with 2 milliliters of acid ninhydrin (1.25 g ninhydrin dissolved in 30 milliliters of glacial acetic acid and 20 milliliters of 6 M phosphoric acid) and 2 milliliters of glacial acetic acid for 1 h at 100 degrees Celsius, taken after by an ice shower. 4 mL toluene was utilized to extricate the colored response blend. The absorbance was measured at 520 nm. A standard bend was utilized to calculate proline substance.
The protein content of seeds was calculated using Microkjeldhal’s method [39]. In a digestion flask, one gram of seed was stored with a little amount of catalyst mixture (K2SO4 + CuSO4), 10 mL of 96 percent concentrated sulphuric acid and kept for complete digestion. A distilled sample of the digested sample was obtained. The amount of ammonia in the distilled solution was titrated with 0.1 N H2SO4.
The equation stated below was used to calculate the nitrogen and protein content:
2.7 Measurement of Yield and Yield Components
The entire plant was cut at ground level with a sickle as it reached maturity. Each plot’s harvested produce was packed separately and labeled correctly. At long last, information on yield-related characteristics such as number of pods per plant−1 and seed yield were independently recorded.
2.8 Statistical Analysis of Recorded Data
MSTAT-C version 4 [40] was utilized to assess all of the information measurably. Duncan’s multiple range tests were used to demonstrate the variability of the results and determine whether there was a significant (P ≤ 0.05) difference between treatment groups using MSTAT-C software.
The contrast of means showed that BD-6048 exhibited a substantial increase in Chl a and Chl b compared with other genotypes (Table 2). Chl a and Chl b content were diminished under the severe stress compared to other non-stress conditions. Results for the interaction of genotype and management practices on the Chl a and Chl b showed that BD-6048 with proline application had the highest Chl a and Chl b content contrasted to MRS, OAC, OACD, OAPM and HP application. Carotenoids content was significantly influenced by chickpea genotypes (Table 3). Means comparison on carotenoids content demonstrated that the highest carotenoids content was recorded from the genotype BD-6048 followed by genotype BD-6045. The application of proline as foliar spray caused a substantial rise in carotenoids’ content by 20.55% compared to severe water stress for BD-6048. Based on interactive effect, the maximum carotenoids content was detected from BD-6048 with proline application. Under rigorous water stress, lowest concentrations of carotenoids were detected for the genotype BD-6092 (Table 3). Fig. 1 exposed significant relationship (R2 = 0.44, P < 0.05) between carotenoids content and seed yield. The assessment of the data linked with the RWC illustrated that RWC had a significant increase by using genotype BD-6048. Under SWS, there was lowest RWC in comparison with MRS, OAC, OACD, OAPM and HP application (Table 3).
Figure 1: Functional relationships between carotenoids content and seed yield of chickpea
3.2 Concentration of P and K in Leaf
Table 4 showed that genotypes had significant difference in leaf K concentration and highest K concentration was observed in BD-6048. Evaluation of results on K concentration demonstrated that K concentration increased at peak due to application of proline and it was increased by 48.81% compared to SWS for BD-6048. Comparison of means for the interaction of genotypes and management practices on the K content showed that under proline application the genotype BD-6048 showed significantly highest K concentration over severe water stress with BD-6092. Results on leaf P concentration explained that P concentration was significantly affected by genotype (Table 4). The highest P concentration was obtained from genotype BD-6048. For leaf P concentration, significant difference was observed among management treatments. SWS decreased P concentration compared to MRS, OAC, OACD, OAPM and HP. Assessment of means of the interactions of genotypes and management practices are shown in Table 4. P concentration varied significantly due variation of available water in different management practices and this was identified to be variable according to genotype also.
3.3 Protein and Proline Content
Comparing the mean protein content data in chickpea seed demonstrated that the genotype BD-6048 produced the highest protein content related to other genotypes. Under different management conditions, the proline application treatments had significantly higher protein content but under SWS, it showed lower value (Table 5). The interaction effects of genotypes and management practices showed that SWS led to a significant decrease in protein content compared to MRS, OAC, OACD, OAPM and HP application with BD-6092. Results illustrated that exogenous proline application treatments helps in remarkable rise (19.93%) in the leaf proline content compared with the SWS and other management treatments for BD-6048 (Table 5). In respect of interactive effect, BD-6048 produced highest proline content in the leaf and lowest proline was observed in BD-6092 under SWS condition. Proline was accountable for seed yielding genotypes (R2 = 0.36, P < 0.05) (Fig. 2).
Figure 2: Functional relationships between proline content and seed yield of chickpea
3.4 Yield and Yield Attributes
The genotype, BD-6048 produced the highest number of pods irrespective of treatments (Table 6). Pod number was affected by management practices. The SWS reduced pod numbers plant−1. Interactions between genotypes and management treatments were significant for pod number. The seed yields in different chickpea genotypes are presented in Table 6. The seed yield of all chickpea genotypes was influenced by management practices. Interactions between genotype and management treatments were significant. The highest seed yield was gained from the genotype, BD-6048 with foliar application of proline. There was a positive relationship (R2 = 0.79, P < 0.01) between pod number plant−1 and seed yield (Fig. 3).
Figure 3: Functional relationships between pods number and seed yield of chickpea
Around 90% of chickpea is cultivated rainfed and depends on left-over moisture from rainfall in the soil so drought tolerance has been one of the most studied features in chickpea [41]. Drought-induced yield reduction as a drought-tolerance measure was used in over a hundred research, with considerable differences in the results [42]. As a result, when choosing genotypes to be produced as cultivars, consider not only their yield in drought-stressed and non-stressed environments, but also their adaptability to their growth environments and management.
Increased production of chickpeas can help with global food supply and farming systems. Chickpea output can be increased to a sustainable level by using prospective/potential genotypes and cultural activities. These management approaches must be acceptable in both plant features and a cost-effective production scale to increase yield. Dry spell push is one of the essential limits to agrarian yield around the world [43], and it has a significant impact on the chickpea crop at all phases of development [44]. Adopting improved genotypes with proper cultural activities at the farmers’ level may reduce the environmental stresses on chickpeas to produce a higher yield. Our study hypothesizes a positive impact of the interaction of organic amendment and genotypes on physiological and biochemical properties and chickpea yield. The management practices used in this investigation showed a remarkable result on the physiological, biochemical, and yield attributes of chickpea genotypes.
Chickpea production varies due to varietal differences in yield potentiality, which is susceptible to water and fertilizer application, and climate conditions such as excess soil moisture/humidity and rainfall [45]. Severe water stress affects the physiological and biochemical traits and yield and yield parameters [46–48]. Drought stress has a substantial impact on a decrease in Chl a and Chl b throughout vegetative growth stages due to an increase in leaf temperature following stomatal closure [49]. Proline content is a significant criterion for stress tolerance in chickpea genotypes [50], with proline levels increasing in leaves beneath drought stress [51].
In our study, we found the highest Chl a (12.99 mg g−1) and Chl b (20.11 mg g−1), carotenoids content (2.92 mg g−1) and RWC (80.03%) in chickpea genotypes BD-6048 with proline application contrasted to MRS, OAC, OACD, OAPM and HP application. Water stress significantly reduced chlorophyll content in chickpea genotypes [52,53] as did rainfed conditions compared to irrigation-supplied situations [54]. The application of cowdung, compost, and poultry manure to the field would supply nitrogen to soil, enhancing chlorophyll content in chickpea plants. Increasing chlorophyll content through chlorophyll production is a benefit of adding nitrogen to the soil [55]. This may lead higher chlorophyll concentration in chickpea genotypes under OAC, OACD, OAPM managements, but the proportion of nitrogen is not so high. As a result, the magnitude of chlorophyll content is less than proline application. To boost growth in chickpea plants, proline was added to the photosynthetic pigments that maintain chlorophyll concentration. Proline interacts with enzymes to protect photosynthetic activity and preserve protein structures and functions [56], regulate chlorophyll biosynthesis and inhibit chlorophyll breakdown under oxidative stress, resulting in increased chlorophyll content [57]. In some circumstances, carotenoids biosynthesis is influenced by external conditions, though it is genetic in plants and plays an important part in photosynthesis [58]. The plant capacity is enhanced by increased chlorophyll concentration with proline, leading to increased carotenoids concentration.
RWC varies among the studied chickpea genotypes due to different management practices. Severe water stress reduces the RWC in chickpea plants. Due to drought stress, the RWC was reduced by 68% in chickpea plants [43]. Mulches conserve the soil moisture around the plants by reducing evaporation. Plants utilized more water from underneath soil of mulch to increase production. Our study found the highest RWC in proline applied plants. Proline promotes plant-water relations by sustaining cell turgidity and stomatal function in stressful situations and increasing water intake and photosynthetic rate [59]. This may cause increased RWC in chickpea plants grown with proline application than other adopted management practices.
Proline application has altogether expanded protein content in chickpea plants. Similar findings also found that the exogenous proline application increased the protein and carbohydrate content in quinoa [60] and faba bean [61]. The increased protein accumulation was linked to a significant increase in chlorophyll content due to proline application. Again, proline itself in an amino acid constituted of protein and shows a significant role in plant growth and development [62]. Thus, protein content increase in plants grown with proline application. Exogenous proline application can effectively regulate endogenous proline metabolism via differential expression of proline-related genes [63]. Exogenous proline application significantly increased the proline substance of rice [62], maize [64] and chickpea [65]. Exogenous proline application combined with endogenous proline biosynthesis may cause increased proline content in chickpea plants.
Proline applied chickpea genotypes produced a higher number of pods plant−1 and seed yield in comparison to SWS, MRS, MWH, OAC, OACD, OAPM and HP. Proline application increased the seed yield in fennel [66], pod and seed yield in chickpea [67], sugar and yield in sugar beet [68], pod and seed yield in Cowpea [69]. Exogenous proline application also alleviates the water stress through stimulated growth, accomplished by induced antioxidating mechanism, eased oxidative damage, improved compatible solute synthesis, and accelerated proline accumulation, resulting in improved photosynthesis yield attributes [70]. In severe water stress we found most of the morphological and biochemical and yield attributes in the lower rate. Though the mulching, organic amendment and hydro priming have played positive role in the studied parameters, proline performed the highest among the adopted management practices in chickpea genotypes. Proline accumulation in plants is a genetic trait in chickpea but the variation is also evident in accumulation in different genotypes due to different management practices and environmental situations. Exogenous proline can promote several metabolic processes in plants, leading to increased chlorophyll, carotenoids, P, K, protein, and endogenous proline content. These increasing pigment and solute enhance the photosynthesis process to produce more food to develop healthy plants resulting in higher pods and seed yield in chickpea.
Regarding present knowledge, inadequate research is accessible in chickpea to recognize the effects of management practices on physiological, biochemical and yield parameters. Therefore, it is a novel report in which different management practices, including proline application, were planned to screen potential genotype. Stress tolerant genotypes were recognized by investigating physiological and biochemical attributes. Among all the studied traits, carotenoids, Chl a, Chl b, proline and protein content explicated more data variability. BD-6048 was the most excellent genotype in proline application under combined treatment. The discovery of the top-performing genotypes can be promoted for large scale cultivation to gain sustainable production under changing climate conditions.
Acknowledgement: The authors would like to thank Department of Agronomy, Bangladesh Agricultural University, Bangladesh. The authors extend their appreciation to Taif University for funding current work by Taif University Researchers Supporting Project No. (TURSP-2020/59), Taif University, Taif, Saudi Arabia.
Authorship: The authors confirm contribution to the paper as follows: study conception and design: U. K. S, A. N. M. A. K, N. I, M. R. U, A. K. H; data collection: A. N. M. A. K, U. K. S; analysis and interpretation of results: U. K. S, A. N. M. A. K; draft manuscript preparation: U. K. S, F. M. J. U, A. K. H, M. R. U, N. I, M. A. H, M. M. H, S. H, M. F. K. S. All authors reviewed the results and approved the final version of the manuscript.
Funding Statement: This research was funded by Bangladesh Agricultural Research Council, Bangladesh. The current work was also funded by Taif University Researchers Supporting Project No. (TURSP-2020/59), Taif University, Taif, Saudi Arabia.
Conflicts of Interest: The authors declare no conflict of interest to report regarding the present study.
References
1. Dadon Bar-El, S., Abbo, S., Reifen, R. (2017). Leveraging traditional crops for better nutrition and health-the case of chickpea. Trends in Food Science and Technology, 64, 39–47. DOI 10.1016/j.tifs.2017.04.002. [Google Scholar] [CrossRef]
2. Rafiq, C. M., Mahmood, M. T., Ahmed, M., Ali, I., Shafiq, M. et al. (2018). Exploration of genetic divergence and performance of morpho-agronomic traits of chickpea. Science and Technology Development, 37(4), 202–208. DOI 10.3923/std.2018.202-208. [Google Scholar] [CrossRef]
3. Nisa, Z. U., Arif, A., Waheed, M. Q., Shah, T. M., Iqbal, A. et al. (2020). A comparative metabolomic study on desi and kabuli chickpea (Cicer arietinum L.) genotypes under rainfed and irrigated field conditions. Scientific Reports, 10(1), 13919. DOI 10.1038/s41598-020-70963-6. [Google Scholar] [CrossRef]
4. Richards, M. F., Preston, A. L., Napier, T., Jenkins, L., Maphosa, L. (2020). Sowing date affects the timing and duration of key chickpea (Cicer arietinum L.) growth phases. Plants, 9(10), 1257. DOI 10.3390/plants9101257. [Google Scholar] [CrossRef]
5. Venkatramanan, V., Shah, S. (2019). Climate smart agriculture technologies for environmental management: The intersection of sustainability, resilience, wellbeing and development. In: Shah, S., Venkatramanan, V., Prasad, R. (Eds.Sustainable green technologies for environmental management, pp. 29–51. Singapore: Springer. DOI 10.1007/978-981-13-2772-8_2. [Google Scholar] [CrossRef]
6. Shafiq, M., Arif, M., Akhtar, N., Yousaf, M., Ghaffar, A. (2021). Exogenous application of growth promoters can improve the chickpea productivity under terminal heat stress conditions by modulating the antioxidant enzyme system. Pakistan Journal of Agricultural Sciences, 58(1), 35–42. DOI 10.21162/PAKJAS/21.624. [Google Scholar] [CrossRef]
7. Kang, Y., Khan, S., Ma, X. (2009). Climate change impacts on crop yield, crop water productivity and food security–A review. Progress in Natural Science, 19(12), 1665–1674. DOI 10.1016/j.pnsc.2009.08.001. [Google Scholar] [CrossRef]
8. Kadiyala, M., Kumara Charyulu, D., Nedumaran, S., Shyam, M., Gumma, M. (2016). Agronomic management options for sustaining chickpea yield under climate change scenario. Journal of Agrometeorology, 18(1), 41–47. DOI 10.54386/jam.v18i1.897. [Google Scholar] [CrossRef]
9. Matiu, M., Ankerst, D. P., Menzel, A. (2017). Interactions between temperature and drought in global and regional crop yield variability during 1961–2014. PLoS One, 12(5), e0178339. DOI 10.1371/journal.pone.0178339. [Google Scholar] [CrossRef]
10. Bistgani, Z. E., Siadat, S. A., Bakhshandeh, A., Pirbalouti, A. G., Hashemi, M. (2017). Interactive effects of drought stress and chitosanapplication on physiological characteristics and essential oil yield of Thymus daenensis Celak. The Crop Journal, 5(5), 407–415. DOI 10.1016/j.cj.2017.04.003. [Google Scholar] [CrossRef]
11. Pandey, P., Irulappan, V., Bagavathiannan, M. V., Senthil-Kumar, M. (2017). Impact of combined abiotic and biotic stresses on plantgrowth and avenues for crop improvement by exploiting physio-morphological traits. Frontiers in Plant Science, 8, 537. DOI 10.3389/fpls.2017.00537. [Google Scholar] [CrossRef]
12. Fahad, S., Bajwa, A. A., Nazir, U., Anjum, S. A., Farooq, A. et al. (2017). Cropproduction under drought and heat stress: Plant responses and management options. Frontiers in Plant Science, 8, 1147. DOI 10.3389/fpls.2017.01147. [Google Scholar] [CrossRef]
13. Wang, P., Su, L., Gao, H., Jiang, X., Wu, X. et al. (2018). Genome-wide characterization of bHLH genes in grape and analysis of their potential relevance to abiotic stress tolerance and secondary metabolite biosynthesis. Frontiers in Plant Science, 9, 64. DOI 10.3389/fpls.2018.00064. [Google Scholar] [CrossRef]
14. Hussain, F., Hussain, I., Khan, A. H. A., Muhammad, Y. S., Iqbal, M. et al. (2018). Combined application of biochar, compost, and bacterial consortia with Italian ryegrass enhanced phyto remediation of petroleum hydrocarbon contaminated soil. Environmental and Experimental Botany, 153, 80–88. DOI 10.1016/j.envexpbot.2018.05.012. [Google Scholar] [CrossRef]
15. Tadayyon, A., Nikneshan, P., Pessarakli, M. (2018). Effects of drought stress on concentration of macro and micronutrients in castor (Ricinus communis L.) plant. Journal of Plant Nutrition, 41(1), 304–310. DOI 10.1080/01904167.2017.1381126. [Google Scholar] [CrossRef]
16. Mirzaei, A., Naseri, R., Moghadam, A., Esmailpour-Jahromi, M. (2013). The effects of drought stress on seed yield and some agronomic traits of canola cultivars at different growth stages. Bulletin of Environment, Pharmacology and Life Sciences, 2(11), 115–121. [Google Scholar]
17. Ghandilyan, A., Barboza, L., Tisné, S., Granier, C., Reymond, M. et al. (2009). Genetic analysis identifies quantitative trait loci controlling rosette mineral concentrations in Arabidopsis thaliana under drought. New Phytologist, 184(1), 180–192. DOI 10.1111/j.1469-8137.2009.02953.x. [Google Scholar] [CrossRef]
18. Masclaux-Daubresse, C., Daniel-Vedele, F., Dechorgnat, J., Chardon, F., Gaufichon, L. et al. (2010). Nitrogen uptake, assimilation and remobilization in plants: Challenges for sustainable and productive agriculture. Annals of Botany, 105(7), 1141–1157. DOI 10.1093/aob/mcq028. [Google Scholar] [CrossRef]
19. Etienne, P., Diquelou, S., Prudent, M., Salon, C., Maillard, A. et al. (2018). Macro and micronutrient storage in plants and their remobilization when facing scarcity: The case of drought. Agriculture, 8(1), 14. DOI 10.3390/agriculture8010014. [Google Scholar] [CrossRef]
20. Ganjeali, A., Porsa, H., Bagheri, A. (2011). Assessment of Iranian chickpea (Cicer arietinum L.) germplasms for drought tolerance. Agricultural Water Management, 98(9), 1477–1484. DOI 10.1016/j.agwat.2011.04.017. [Google Scholar] [CrossRef]
21. Hosseinzadeh, S. R., Amiri, H., Ismaili, A. (2016). Effect of vermicompost fertilizer on photosynthetic characteristics of chickpea (Cicer arietinum L.) under drought stress. Photosynthetica, 54(1), 87–92. DOI 10.1007/s11099-015-0162-x. [Google Scholar] [CrossRef]
22. Mssacci, A., Nabiev, S. M., Pietrosanti, L., Nematov, S. K., Chernikova, T. N. et al. (2008). Response of photosynthetic apparatus of cotton (Gossypium hirsutum) to the onest of water stress under field conditions studied by gas-exchange analysis and chlorophyll fluorescence imaging. Plant Physiology and Biochemistry, 46(2), 189–195. DOI 10.1016/j.plaphy.2007.10.006. [Google Scholar] [CrossRef]
23. Bender Özenç, D. (2008). Growth and transpiration of tomato seedlings grown in hazelnut husk compost under water deficit stress. Compost Science and Utilization, 16(2), 125–138. DOI 10.1080/1065657X.2008.10702367. [Google Scholar] [CrossRef]
24. Ahmadpour, R., Hosseinzadeh, S. R., Armand, N. (2016). Evaluation of methanol role in reducing the negative effects of water deficit stress in lentil (Lens culinaris Medik). Journal of Plant Process and Function, 5, 1–13 (in Persian). [Google Scholar]
25. Pagter, M., Bragato, C., Brix, H. (2005). Tolerance and physiological responses of Phragmites australis to water deficit. Aquatic Botany, 81(4), 285–299. DOI 10.1016/j.aquabot.2005.01.002. [Google Scholar] [CrossRef]
26. Armand, N. H., Amiri, H., Ismaili, A. (2016). Interaction of methanol spray and water-deficit stress on photosynthesis and biochemical characteristics of Phaseolus vulgaris L. cv. Sadry. Photochemistay and Photobiology, 92(1), 102–110. DOI 10.1111/php.12548. [Google Scholar] [CrossRef]
27. Shah, T. M., Imran, M., Atta, B. M., Ashraf, M. Y., Hameed, A. et al. (2020). Selection and screening of drought tolerant high yielding chickpea genotypes based on physio-bio chemical indices and multi-environmental yield trials. BMC Plant Biology, 20, 1–16. DOI 10.1186/s12870-020-02381-9. [Google Scholar] [CrossRef]
28. Dong, W., Qin, J., Li, J., Zhao, Y., Nie, L. et al. (2011). Interactions between soil water content and fertilizer on growth characteristics and biomass yield of Chinese white poplar (Populusto mentosa Carr.) seedlings. Journal of Soil Science and Plant Nutrition, 57(2), 303–312. DOI 10.1080/00380768.2010.549445. [Google Scholar] [CrossRef]
29. Bindraban, P. S., Dimkpa, C. O., Pandey, R. (2020). Exploring phosphorus fertilizers and fertilization strategies for improved human and environmental health. Biology and Fertility of Soils, 56(3), 299–317. DOI 10.1007/s00374-019-01430-2. [Google Scholar] [CrossRef]
30. Azab, E. (2016). Effect of water stress and biological fertilization on maize growth, chemical composition and productivity in calcareous. American Journal of Plant Physiology, 11(1–3), 1–11. DOI 10.3923/AJPP.2016.1.11. [Google Scholar] [CrossRef]
31. Ghosh, M., Devi, A. (2019). Assessment of crop growth, soil properties and crop yield in an upland acidic soil with inorganic fertilizer blended with organic amendments in summer rice cropping seasons. International Journal of Recycling of Organic Waste in Agriculture, 8, 1–9. DOI 10.1007/s40093-019-0252-z. [Google Scholar] [CrossRef]
32. Huerta, E., Vidal, O., Jarquin, A., Geissen, V., Gomez, R. (2010). Effect of vermicompost on the growth and production of amashito pepper, interactions with earthworms and rhizobacteria. Compost Science and Utilization, 18(4), 282–288. DOI 10.1080/1065657X.2010.10736967. [Google Scholar] [CrossRef]
33. Lakhdar, A., Rabhi, M., Ghnaya, T., Montemurro, F., Jedidi, N. et al. (2009). Effectiveness of compost use in salt-affected soil. Journal of Hazardous Materials, 171(1–3), 29–37. DOI 10.1016/j.jhazmat.2009.05.132. [Google Scholar] [CrossRef]
34. BARC (2018). Fertilizer recommendation guide, Farmgate, Dhaka, Bangladesh: Bangladesh Agricultural Research Council. [Google Scholar]
35. Lichtenthaler, A., Wellburn, R. (1983). Determinations of total carotenoids and chlorophylls a and b of leaf extracts in different solvents. Biochemical Society Transactions, 11(5), 591–592. DOI 10.1042/BST0110591. [Google Scholar] [CrossRef]
36. Jackson, M. L. (1975). Soil chemical analysis. New Delhi: Prentice Hall of India Pvt. Ltd. [Google Scholar]
37. Chapman, H. D., Pratt, P. R. (1961). Methods of analysis for soils, plants and water. Barley, pp. 169–170. USA: University of California. [Google Scholar]
38. Bates, L. S., Waldren, R. P., Teare, L. D. (1973). Rapid determination of free proline for water stress studies. Plant and Soil, 39(1), 205–207. DOI 10.1007/BF00018060. [Google Scholar] [CrossRef]
39. AOAC (1965). Official methods of analysis of association of official analytical chemists, 15th ed. Washington DC, USA: Association of Official Analytical Chemists. [Google Scholar]
40. MSTAT-C (1987). Software program for the design and analysis of agronomic research experiments (Version 4). East Lansing, Michigan, USA: Michigan University. [Google Scholar]
41. Daryanto, S., Wang, L., Jacinthe, P. A. (2015). Global synthesis of drought effects on food legume production. PLoS One, 10(6), e0127401. DOI 10.1371/journal.pone.0127401. [Google Scholar] [CrossRef]
42. Kumar, J., Abbo, S. (2001). Genetics of flowering time in chickpea and its bearing on productivity in semiarid environments. Advances in Agronomy, 72, 107–138. DOI 10.1016/s0065-2113(01)72012-3. [Google Scholar] [CrossRef]
43. Khan, N., Bano, A., Babar, M. A. (2019). Metabolic and physiological changes induced by plant growth regulators and plant growth promoting rhizobacteria and their impact on drought tolerance in Cicer arietinum L. PLoS One, 14(3), e0213040. DOI 10.1371/journal.pone.0213040. [Google Scholar] [CrossRef]
44. Maqbool, M. A., Aslam, M., Ali, H. (2017). Breeding for improved drought tolerance in chickpea (Cicer arietinum L.). Plant Breeding, 136(3), 300–318. DOI 10.1111/pbr.12477. [Google Scholar] [CrossRef]
45. Islam, M. R. M. A. M., Islam, M. H. R., Choudhury, M. A. R. (2016). Evaluation of some advance chickpea genotypes in different region of Bangladesh. Eco-Friendly Agriculture Journal, 9(1), 1–5. [Google Scholar]
46. Talebi, R., Ensafi, M. H., Baghebani, N., Karami, E., Mohammadi, K. (2013). Physiological responses of chickpea (Cicer arietinum) genotypes to drought stress. Environmental and Experimental Biology, 11, 9–15. [Google Scholar]
47. Shah, T. M., Imran, M., Atta, B. M. (2020). Selection and screening of drought tolerant high yielding chickpea genotypes based on physio-biochemical indices and multi-environmental yield trials. BMC Plant Biology, 20(1), 171. DOI 10.1186/s12870-020-02381-9. [Google Scholar] [CrossRef]
48. Hussain, T., Akram, Z., Shabbir, G., Manaf, A., Ahmed, M. (2021). Identification of drought tolerant chickpea genotypes through multi trait stability index. Saudi Journal of Biological Sciences, 28(12), 6818–6828. DOI 10.1016/j.sjbs.2021.07.056. [Google Scholar] [CrossRef]
49. Pouresmael, M., Khavari-Nejad, R. A., Mozafari, J., Najafi, F., Moradi, F. (2012). Wild cicer species response to drought stress through different mechanisms. Advances in Environmental Biology, 6(11), 2966–2975. [Google Scholar]
50. Jaleel, C. A., Gopi, R., Sankar, B., Manivannan, P., Kishorekumar, A. et al. (2007). Studies on germination, seedling vigour, lipid peroxidation and proline metabolism in Catharanthus roseus seedlings under salt stress. South African Journal of Botany, 73(2), 190–195. DOI 10.1016/j.sajb.2006.11.001. [Google Scholar] [CrossRef]
51. Mafakheri, A., Siosemardeh, A., Bahramnejad, B., Struik, P. C., Sohrabi, Y. (2010). Effect of drought stress on yield, proline and chloro phyll contents in three chickpea cultivars. Australian Journal of Crop Science, 4(8), 580–585. [Google Scholar]
52. Kataria, N., Singh, N. (2013). Amelioration of water stress by potassium fertilizer in chickpea (Cicer arietinum L.). Journal of Tropical Plant Physiology, 5, 22–32. [Google Scholar]
53. Dalvi, U. S., Naik, R. M., Awari, V. R. (2016). Morpho-physiological responses of chickpea (Cicer arietinum L.) genotypes under drought stress. International Journal of Science, Environment and Technology, 5(3), 997–1007. [Google Scholar]
54. Ghiabi, S., Sharafi, S., Talebi, R. (2013). Morpho-physiological and biochemical alternation responses in different chickpea (Cicer arietinum L.) genotypes under two constructing water regimes. International Journal of Biological Sciences, 3, 57–65. DOI 10.12692/ijb/3.8.57-65. [Google Scholar] [CrossRef]
55. Cecchin, I., Terezinha, F. F. (2004). Effect of nitrogen supply on growth and photosynthesis of sunflower plants grown in the greenhouse. Plant Science, 166(5), 1379–1385. DOI 10.1016/J.PLANTSCI.2004.01.020. [Google Scholar] [CrossRef]
56. Hamilton, E. W., Heckathorn, S. A. (2001). Mitochondrial adaptations to NaCl. Complex I is protected by anti-oxidants and small heat shock proteins, whereas complex II is protected by proline and betaine. Plant Physiology, 126(3), 1266–1274. DOI 10.1104/126.3.1266. [Google Scholar] [CrossRef]
57. Kattab, H. (2007). Role of glutathione and polyadenylic acid on the oxidative defense systems of two different cultivars of canola seedlings grown under saline condition. Australian Journal of Basic and Applied Sciences, 1(3), 323–332. [Google Scholar]
58. Yadav, A., Garg, V. K. (2015). Influence of vermi-fortification on chickpea (Cicer arietinum L.) growth and photosynthetic pigments. International Journal of Recycling of Organic Waste in Agriculture, 4, 299–305. DOI 10.1007/s40093-015-0109-z. [Google Scholar] [CrossRef]
59. Shamsul, H., Qaiser, H., Alyemeni, M. N., Wani, A. S., Pichtel, J. et al. (2012). Role of proline under changing environments: A review. Plant Signaling and Behavior, 7(11), 1456–1466. DOI 10.4161/psb.21949. [Google Scholar] [CrossRef]
60. Abdallah, M. M. S., El-Bassiouny, H. M. S. (2016). Impact of exogenous proline or tyrosine on growth, some biochemical aspects and yield components of quinoa plant grown in sandy soil. International Journal of Pharmacy and Technology, 9(7), 12–23. [Google Scholar]
61. Taie, H. A. A., Abdelhamid, M. T., Dawood, M. G., Nassar, R. M. A. (2013). Pre-sowing seed treatment with proline improves some physiological, biochemical and anatomical attributes of faba bean plants under sea water stress. Journal of Applied Sciences Research, 9(4), 2853–2867. [Google Scholar]
62. Suekawa, M., Fujikawa, Y., Esaka, M. (2019). Exogenous proline has favorable effects on growth and browning suppression in rice but not in tobacco. Plant Physiology and Biochemistry, 142, 1–7. DOI 10.1016/j.plaphy.2019.06.032. [Google Scholar] [CrossRef]
63. El Moukhtari, A., Cabassa-Hourton, C., Farissi, M., Savouré, A. (2020). How does proline treatment promote salt stress tolerance during crop plant development? Frontiers in Plant Science, 11, 1127. DOI 10.3389/fpls.2020.01127. [Google Scholar] [CrossRef]
64. Alam, R., Das, D., Islam, M., Murata, Y., Hoque, M. (2016). Exogenous proline enhances nutrient uptake and confers tolerance to salt stress in maize (Zea mays L.). Progressive Agriculture, 27(4), 409–417. DOI 10.3329/pa.v27i4.32120. [Google Scholar] [CrossRef]
65. El-Beltagi, H. S., Mohamed, H. I., Sofy, M. R. (2020). Role of ascorbic acid, glutathione and proline applied as singly or in sequence combination in improving chickpea plant through physiological change and antioxidant defense under different levels of irrigation intervals. Molecules, 25(7), 1702. DOI 10.3390/molecules25071702. [Google Scholar] [CrossRef]
66. Zali, A. G., Ehsanzadeh, P. (2018). Exogenous proline improves osmoregulation, physiological functions, essential oil, and seed yield of fennel. Industrial Crops and Products, 111, 133–140. DOI 10.1016/j.indcrop.2017.10.020. [Google Scholar] [CrossRef]
67. Kaur, G., Kumar, S., Thakur, P., Malik, J. A., Bhandhari, K. et al. (2011). Involvement of proline in response of chickpea (Cicer arietinum L.) to chilling stress at reproductive stage. Scientia Horticulturae, 128(3), 174–181. DOI 10.1016/j.scienta.2011.01.037. [Google Scholar] [CrossRef]
68. Ghaffari, H., Tadayon, M. R., Bahador, M., Razmjoo, J. (2021). Investigation of the proline role in controlling traits related to sugar and root yield of sugar beet under water deficit conditions. Agricultural Water Management, 243, 106448. DOI 10.1016/j.agwat.2020.106448. [Google Scholar] [CrossRef]
69. Merwad, A. R. M., Desoky, E. S. M., Rady, M. M. (2018). Response of water deficit-stressed Vigna unguiculata performances to silicon, proline or methionine foliar application. Scientia Horticulturae, 228, 132–144. DOI 10.1016/j.scienta.2017.10.008. [Google Scholar] [CrossRef]
70. Anjum, S. A., Xie, X. Y., Wang, L. C., Saleem, M. F., Man, C. et al. (2011). Morphological, physiological and biochemical responses of plants to drought stress. African Journal of Agricultural Research, 6(9), 2026–2032. DOI 10.5897/AJAR10.027. [Google Scholar] [CrossRef]
Cite This Article
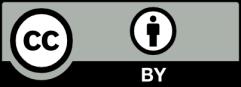