Open Access
ARTICLE
The Ability of Some Aquatic and Terrestrial Plants to Purify Domestic Wastewater
1 Department of Botany, Government College Women University, Faisalabad, 38000, Pakistan
2 Department of Botany, University of Agriculture, Faisalabad, 38040, Pakistan
3 Department of Chemistry, Government College Women University, Faisalabad, 38000, Pakistan
4 Department of Agronomy, Muhammad Nawaz Shareef University of Agriculture, Multan, 66000, Pakistan
5 College of Agriculture, Fujian Agriculture and Forestry University, Fuzhou, 350002, China
* Corresponding Authors: Abida Kausar. Email: ; Ali Raza. Email:
(This article belongs to the Special Issue: Physiological and Molecular Interventions in Improving Abiotic Stress Tolerance in Plants)
Phyton-International Journal of Experimental Botany 2023, 92(8), 2245-2260. https://doi.org/10.32604/phyton.2023.028264
Received 07 December 2022; Accepted 07 April 2023; Issue published 25 June 2023
Abstract
The study aimed to evaluate the ability of some terrestrial and aquatic plants for wastewater purification. Aquatic plants can remove pollutants from wastewater by consuming and accumulating various contaminants in different parts of plants. Different aquatic and terrestrial plants (Rosa sinensis, Typha latifolia, Ocimm bacilicum, Azolla pinnata, and Salvinia molesta) which have the ability to decrease water pollution were utilized in this study. The capability of five different species of plants was investigated by measuring chemical oxygen demand (COD), biological oxygen demand (BOD), electrical conductivity (EC), total dissolved solids (TDS), and pH of the medium. In this research, some aquatic and terrestrial plants were transplanted in wastewater plastic pots containing domestic wastewater with different ratios of 50% and 100%. Then, after 30 days, the physiological and biochemical parameters of plants were calculated to observe the effect of wastewater on plants. Results revealed higher chlorophyll and carotenoids in typha plants treated with 100% wastewater. The highest percentage of elimination in BOD (65%), COD (27%), TDS (72%), EC (83%), and pH (6.8%) was noted with the use of typha and azolla. Intriguingly, total soluble sugars, total free amino acids, and total proteins were found maximum in the hibiscus plant as compared to the other plants under 100% and 50% domestic wastewater treatment, while typha and ocimum showed lower values of these parameters irrespective of wastewater treatments. Moreover, the COD, BOD, TDS, EC, and pH trend was higher in 100% wastewater as compared to 50% wastewater. Taking into account the accumulation capacity of the tested plants especially typha can be efficiently used for the treatment of domestic wastewater.Keywords
The challenges of abiotic stress on plant growth and development are evident among the emerging ecological impacts of climate change [1–8]. The constraints to agriculture production are also exacerbated by the increasing human population that compete for environmental resources. Underground and surface water are the primary sources of fresh water; however, the rapid development of industries and an overwhelming population are continuously polluting the water all over the world and causing risk of freshwater sacristy for agricultural production, especially in developing countries including Pakistan. Farmers are using sewage and industrial water to meet water requirements [9–11], these water sources are comprised of different types of pollutants that cause harmful effects on the natural ecosystem due to the presence of inorganic, organic toxins, including pathogens, nitrates, heavy metals and salts [12,13] that causing consequent risk for human health as well as for the aquatic ecosystem. Heavy metals and nutrients are beneficial at a lower dose, but cause a critical effect on the plant life cycle at higher concentrations [14–21]. Of all the wastewater resources, domestic wastewater makes up the largest portion generated by human activities, and up to 90% of the clean water consumption is discharged as wastewater [22]. The basic access of water consumption for minimum hygiene and hydration is 20 I/c/d, while the optimum access, including bathing, laundry, food preparation, and personal hygiene requires at least 100 I/c/d [23]. The aquatic database recorded that municipal water withdrawal from 180 countries in 2017 ranged from 1 to 359 m3/c/year (3 to 978 l/c/d) with a median of 54.3 m3/c/year (149 l/c/d), which is mainly comprised of domestic consumption but may include water for urban agriculture and industrial uses [24]. Approximately 70% of domestic wastewater is generated from households or residential buildings, and the rest is generated from public facilities, commercial areas, and office buildings [25], however, the both sources share similar constituents but differ in quantity and composition [26]. Currently, conventional wastewater treatment methods are not effective for the complete removal of water contaminants [27]. A small quantity of the contaminants can cause habitat degradation due to the toxic nature of these contaminants that can interfere with many ongoing cellular processes in plant tissues [28]. However, excess sludge discharge, carbon emission, high energy equipment’s and high maintenance costs pose serious challenges to the methods of wastewater removal. However, the critical analysis and wastewater treatment are still limited [29].
Phytoremediation technology is environment friendly and used for the uptake of pollutants from soil and water resources [30]. The phytoremediation technology is easy to maintain and implement and does not require highly specialized personnel and expensive equipment’s [31], so the construction of wetlands as green technology can be used to reclaim various water sources. In this study, the phytoremediation potential of different aquatic and terrestrial plants such as T. latifolia (typha), R. sinensis (hibiscus), O. bacilicum (ocimum), A. pinnata (azolla) and S. molesta (salvinia) species were evaluated for domestic wastewater purification. Moreover, the effect of domestic wastewater was also assessed in the physiological and biochemical analysis of above-mentioned plants. A mechanistic diagram is depicted in Fig. 1.
Figure 1: A graphic illustration of the effect of domestic wastewater treatment on aquatic and terrestrial plants
2.1 Plant and Wastewater Collection for Experimental Setup
The experiment was designed to study the role of some aquatic and terrestrial plants in wastewater treatment. The experiment was conducted in the Research area of Government College Women University of Faisalabad (GCWUF). The five different plants such as typha, hibiscus, ocimum, azolla and salvinia were used in the experiment. Wastewater was collected from GCWUF. Then water was transferred to a hydroponic medium with three replicates containing 100% and 50% wastewater and control. The plants were completely washed under tap water and then transferred into hydroponic treatments. Half strength hogland solution was given during the experiment. The experiment lasted for 30 days and was designed in a completely randomized design (CRD) arranged under three factor factorial arrangement (time, plant species and wastewater treatment) for wastewater analysis, while two factor factorial (plant species and wastewater treatment) was used for plant analysis. The composition of wastewater is given in Table 1.
2.2 Wastewater Analysis (TDS, pH, EC, COD, and BOD)
TDS was measured in domestic wastewater with a TDS meter. The sample was collected in plastic bottles and transferred in a beaker and the TDS meter was dipped into a beaker until a stable value of TDS was observed. PH of domestic wastewater was measured with pH meter. The sample was collected in beakers and stirrer, pH meter until stable reading was measured. EC of domestic wastewater was measured with EC meter. The sample was collected in plastic bottles and transferred into a beaker for EC detection. COD of domestic wastewater samples was measured with COD meter (Tinto meter). In addition, BOD of domestic wastewater samples was measured with a VELP BOD meter.
2.3 Physio-Biochemical Attributes
2.3.1 Chlorophyll a, b, Total Chlorophyll Contents and Carotenoids
Watada et al. [32] method was used to determine the chlorophyll contents (a, b) and total chlorophyll contents, while, Arnon [33] method was used to measure carotenoid content. Fresh leaf material (0.1 g) of the plant was chopped and dipped in 1:1 solution of acetone (80%) and ethanol for 24 h, absorbance was measured by spectrophotometer (UV/Vis) at three different wavelengths (645, 663 and 480 nm).
Total soluble sugars were determined by the method of Yemm et al. [34]. A 0.1 g plant leaf sample was ground in 80% ethanol solution. Extract was incubated at 60°C for 6 h. The extract was added in 6 mL of freshly prepared anthrone reagent and heated the test tubes for 10 min in a boiling water bath. Then test tubes were ice cooled for 10 min, and again incubated at room temperature (25°C) for 20 min. Optical density was observed at 625 nm.
Total soluble protein was estimated by the method of Bonjoch et al. [35]. According to this method, 0.25 g of fresh leaves were ground with 5 mL of phosphate buffer having 7.0. Then, sample was centrifuged for 20 min at 8000 rpm. After extraction, 1 mL of supernatant was added in each test tube and then add 5 mL of Bradford dye, and mixed completely. Sample was filtered 2–3 times. The test tubes were incubated at room temperature for 5 min and then absorbance was observed at 595 nm by using a spectrophotometer.
Hamilton et al. [36] method was used to determine total free amino acids. Extraction of fresh plant material was done in 0.02 M phosphate buffer (pH 7). For, 1 mL extract, 1 mL 10% pyridine and 1 mL 2% Ninhydrine solution were added to each test tube and then covered the test tube with aluminum foil. Then, heat the test tubes in a boiling water bath for 30 min. Each test tube was filled with boiling water up to the capacity of 50 mL. The absorbance was measured at 570 nm.
The phytoremediation potential of five plant species on wastewater treatment was assessed under 100%, 50% and control conditions on physio-chemical attributes of plants and wastewater by using analysis of variance (ANOVA at 5% probability level with the help of statistical software “Statistix” (ver. 8.1, Tallahassee, FL, USA)). Two-way ANOVA (wastewater x plant species) was assessed for plant analysis, while three-way ANOVA was used for water analysis for time base studies. LSD test was performed to compare the relative means. PCA and heat map was constructed by using R. Different letters show significant differences (p < 0.05), while similar letters did not show significant differences.
Statically results showed significant (p < 0.000) differences among aquatic plants, domestic wastewater treatments and weeks, and the interaction among these factors was also significant (p < 0.000) for pH of wastewater. The pH value decreased after week by week. Results showed that the pH value of 100% domestic water exposed plants decreased for typha (6.8%), hibiscus (0.2%), ocimum (6.7%), azolla (0.2), and salvinia (6.7%) after 4 weeks. The pH value of 50% wastewater decreased by using typha (2.3%), hibiscus (6.4%), ocimum (4.5%), azolla (2.5%), and salvinia (6.1%) after four weeks. the maximum decrease was observed in typha plant subjected to 100% wastewater. The maximum decrease was observed in hibiscus plant subjected to 50% wastewater and control water (Fig. 2A, Table 2).
Figure 2: Effect of aquatic and terrestrial plants on the pH, EC and TDS of domestic waste water. (A) pH, (B) EC, (C) TDS
3.1.2 Electrical Conductivity (EC)
Statically results showed significant (p < 0.000) differences among aquatic plants, domestic wastewater treatments and weeks, and the interaction among these factors was also significant (p < 0.000) for EC of wastewater. The EC value decreased after week by week. Results showed that the EC value decreased under 100% domestic water by growing typha (83%), hibiscus (87%), ocimum (80%), azolla (35%), and salvinia (57%) after 4 weeks. The reduction in EC value of 50% wastewater was observed for typha (16%), hibiscus (19%), ocimum (30%), azolla (21%) and salvinia (11%) after four weeks. The maximum decrease was observed in hibiscus plant subjected to 100% wastewater, while the maximum decrease was observed in ocimum plant water subjected to 50% wastewater and control water (Fig. 2B, Table 2).
3.1.3 Total Dissolved Solids (TDS)
Statically results showed significant (p < 0.000) differences among aquatic plants, domestic wastewater treatments and weeks, and the interaction among these factors was also significant (p < 0.000) for TDS of wastewater. These values decreased week after week. Results showed that the TDS value of domestic water (100%) decreased by growing typha (72%), hibiscus (67%), ocimum (24%), azolla (22%), and salvinia (23%) after 4 weeks. The TDS value of 50% wastewater decreased were also decreased by harvesting typha (31%), hibiscus (35%), ocimum (49%), azolla (9%) and salvinia (26%) after four weeks. The maximum decrease was observed in typha plant subjected to 100% wastewater, while under 50% wastewater the maximum decrease was observed in ocimum (Fig. 2C, Table 2).
3.1.4 Biological Oxygen Demand (BOD)
Statically results showed significant (p < 0.000) differences among aquatic plants, domestic wastewater treatments and weeks, and the interaction among these factors was also significant (p < 0.000) for BOD of wastewater. Results showed that the BOD value of 100% domestic water decreased for typha (23%), hibiscus (22%), ocimum (25%), azolla (19%) and salvinia (24%) after 4 weeks. The BOD value of 50% wastewater of typha decreased (65%), hibiscus (38%), ocimum (43%), azolla (37%) and salvinia (50%) after four weeks. The maximum decrease was observed in salvinia plant subjected to 50% wastewater. The maximum decrease was observed in ocimum plant subjected to 100% wastewater and control water (Fig. 3A, Tables 2 and 3).
Figure 3: Effect of aquatic and tersstial plants on the BOD and COD of domestic waste water. (A) BOD, (B) COD
3.1.5 Chemical Oxygen Demand (COD)
Statically results showed significant (p < 0.000) differences among aquatic plants, domestic wastewater treatments and weeks, and the interaction among these factors was also significant (P < 0.000) for COD of wastewater. The COD value decreased after week by week. Results showed that the COD value of 100% domestic water decreased for typha (20%), hibiscus (16%), ocimum (14%), azolla (13%), and salvinia (16%) after 4 weeks. The COD value decreased at 50% wastewater for typha decreased (27%), hibiscus (18%), ocimum (22%), azolla (39%) and salvinia (19%) after four weeks. The maximum decrease was observed in typha plant subjected 100% wastewater. The maximum decrease was observed in azolla plant subjected to 50% wastewater and control water (Fig. 3B, Tables 2 and 3).
3.2 Physio-Biochemical Parameters
The chlorophyll a content varied significantly (p > 0.000) in all plant species plants under all wastewater treatments. Results showed that chl a content increased up to 37%, 34%, 32%, 69% and 9% in hibiscus, typha, ocimum, azolla, and salvinia, respectively at 100% wastewater. Chl a content in plants subjected to 50% wastewater showed 60%, 31%, 22%, 6% and 11% reductions in hibiscus, typha, ocimum, azolla, and salvinia, respectively (Fig. 4A).
Figure 4: Effect of waste water on the physiochemical attributes of different aquatic and terrestrial plant species (A) Chl a, (B) Chl b, (C) carotenoids, (D) total soluble sugars, (E) total soluble proteins and (F) total amino acids. The alphabets indicate the significant (p < 0.05) difference among the treatments
Maximum increase in chl b contents were observed in hibiscus plant subjected to 50% wastewater. Typha, azolla, ocimum and salvinia showed maximum increase at 100% wastewater as compared to normal water (Fig. 4B).
The carotenoid content varied significantly (p > 0.000) in all plant species plants under all wastewater treatments. Results showed that carotenoid contents in leaves increased up to 49%, 85%, 72%, 69% and 9% in hibiscus, typha, ocimum, azolla, and salvinia, respectively at 100% wastewater. Carotenoid contents in plants subjected to 50% wastewater observed were increased up to 54%, 82%, 39% 6% and 11% in hibiscus, typha, ocimum, azolla, and salvinia, respectively (Fig. 4C).
Results showed that the amount of total amino acids increased up to 52%, 44%, 70%, 38% and 61% in hibiscus, typha, ocimum, azolla, and salvinia, respectively at 100% wastewater. Amino acid content acids in plants subjected to 50% wastewater were increased up to 35%, 55%, 97%, 9% and 37% in hibiscus, typha, ocimum, azolla, and salvinia, respectively (Fig. 4D).
The soluble protein content varied significantly (p > 0.000) in all plant species plants under all wastewater treatments. Results showed that the amount of total soluble proteins content decrease up to 10%, 29%, 73%, 10% and 34% in hibiscus, typha, ocimum, azolla, and salvinia, respectively at 100% wastewater. Total soluble sugar proteins in plants subjected to 50% wastewater (3.8%) decreased up to 3.8%, 12%, 39%, 14 and 21% in hibiscus, typha, ocimum, azolla, and salvinia, respectively (Fig. 5E).
Figure 5: Pearson correlation of domestic waste parameters with plant physiochemical properties. The red color depicts negative correlation, while blue for positive correlation. TSP (total soluble proteins), Carot (Carotenoids), TSS (total soluble sugars), TFAA (total free amino acids)
The soluble sugar content varied significantly (p > 0.000) in all plant species plants under all wastewater treatments. Results showed that the amount of total soluble sugars was increased up to 52%, 12%, 70%, 38% and 61% in hibiscus, typha, ocimum, azolla, and salvinia, respectively at 100% wastewater. Amount of total soluble sugars in plants subjected to 50% wastewater was decreased up to 35%, 31%, 97%, 9%, and 37% in hibiscus, typha, ocimum, azolla, and salvinia, respectively. As compared to normal water (Fig. 5F).
Pearson correlation showed a positive correlation of BOD and COD with chlorophyll a, while a negative linear correlation with total soluble sugars and total soluble proteins. Total soluble proteins and total soluble sugars showed a strong positive relationship with chlorophyll b. Moreover, total free amino acids showed a negative correlation with chlorophyll a, BOD and COD, and a positive correlation with total soluble sugars and total soluble proteins. Intriguingly, carotenoids, EC and TDS showed a positive linear relationship with all prescribed parameters, except for chlorophyll b (Fig. 5). Heat map showed that COD, BOD showed a positive relation with increasing domestic wastewater and showed maximum value for 100% domestic wastewater, while lower for control and medium for 50% domestic water irrespective for plant species and weeks variations. While pH, EC and TDS showed a medium response in almost all the treatments (Fig. 6). Heat map for plant analysis reveals that azolla and ocimum had lower chlorophyll a and chlorophyll b content, respectively irrespective of all the treatments. Similarly, lower carotenoid content was shown in azolla specie as compared to other plants. While typha, hibiscus and typha showed varying responses for photosynthetic pigments. Intriguingly, total soluble sugars, total free amino acids and total proteins were found maximum in hibiscus plant as compared to others under 100% and 50% domestic wastewater treatment, while typha and ocimum showed lower values of above-mentioned attributes under wastewater treatment. Moreover, silvinia and Azolla showed a medium response against stress (Fig. 7).
Figure 6: Heat map of timely changes in domestic wastewater in relation to pH, EC, TDS, BOD and COD. The red color depicts lower values while, yellow and blue for medium and high values, respectively
Figure 7: Heat map of timely changes in domestic wastewater in relation to chlorophyll a, chlorophyll b, carotenoids, total soluble sugars, total soluble proteins and total free amino acids. The red color depicts lower values while, yellow and blue for medium and high values, respectively
Abiotic stresses, such as high and low temperatures, ultraviolet radiations, heavy metals, salinity and excessive and deficient water, are hostile to plant growth and production, leading to higher yield reduction across the world [7,37–44], however, these consequences were exacerbated with overwhelming population and current climate changes [43]. Anthropogenic activities increase the risk of environmental pollutants, and the major risk is freshwater resources. The use of plants to recover water and soil from contaminants has been proven to be an effective approach and widely accepted as a cost effective and environmentally friendly technique in the last two decades for wastewater purification soil remediations [45,46]. Aquatic plants that float on the surface of water floating (azolla, and salvinia) and submerged macrophytes typha, hibiscus, and ocimum were found to be used for wastewater purification because these plants are very efficient for the elimination of heavy metals from sewage water. Aquatic plants are beneficial for improving water quality due to their higher efficiency in reducing COD, BOD, pH, TDS and EC (Sivakumar et al. [47], Nikam et al. [48], Pratik et al. [49]). In the present research, EC, TDS, BOD, COD and pH were observed with quite the same decreasing pattern in both 100% and 50% wastewater as compared to control. From, five different aquatic plants (typha, hibiscus, azolla, ocimum, and salvinia), typha showed a high reduction in BOD, COD, EC, TDS and pH (Figs. 2A–2C and 3A and 3B) (Table 2). The reason for the reduction of above-mentioned parameters in typha is that it founds near water and is a wetland specie and can grow in flooded water with water depth almost 2.6 feet. Typha has an extensive root system that acts as a source of accumulation of heavy metals and helps in wastewater purification. Our results corroborate with the findings of Ezeogu et al. [31] by using Eichinochloa pyramidali as the experimental plant. They found BOD and COD removal rate up to 87.68% and 95.1%, respectively. In another study, Sivakumar et al. [47] found maximum removal percentage of COD, BOD and TDS in a paper mill effluent and percent reduction was 79.1%, 88.6%, and 82.3%, respectively with the use of Azolla caroliniana. Moreover, according to Nikam et al. [48] Typha lotifolia removes 85%, 70.5% and 83.3% TDS, BOD and COD. In addition, they found pH reduction from 7.6 to 6.2 and 6.1 by using typha and Phragmites australis as model plants. Additionally, Pratik et al. [49] noted % reduction in NH3-N, BOD, COD and the percent reduction was 89.42%, 89.21%, 76.90%, respectively. The reason for SOD, BOD and TDS reduction is the phytoextraction ability of these plants especially typha.
The biochemical attributes such as, amino acids, total soluble sugars, and total soluble proteins of all plants were also observed after 30 days of phytoremediation process in plants subjected to 100%, 50% wastewater and control as shown in Figs. 4A–4F. The increase in these contents was observed in 100%> 50%> wastewater, the reason is the presence of various nutrients essential for the synthesis of these biochemical attributes. It was also observed that total soluble sugars were higher in plants subjected to wastewater than control. These results are corroborated by the findings of Fan et al. [50], who noted higher photosynthetic efficiency under domestic wastewater that might be due to the higher accumulation of nutrients that assimilates into chlorophyll (as nitrogen and magnesium are the part of chlorophyll). However, Gupta et al. [51] found that total chlorophyll and total free amino acids decreased, while phenols, ascorbic acid, total protein, and soluble sugars increased in Raphanus sativus and Colocasia esculentum expect for Brassica nigra L. for the protein under wastewater. The higher sugar concentration in this study elaborates the defense responses under wastewater treatment [52]. Plant adjusts energy metabolism to enhance acclimation demands by activating photosynthetic machinery. Moreover, tolerant plants constitutively enhanced detoxification and several stress related proteins against stress [53,54]. So, it evaluated due to stress conditions increased plant sugar and protein content. Similarly, correlation data also showed that total soluble sugars, total free amino acids and total proteins were found maximum in hibiscus plant as compared to others under 100% and 50% domestic wastewater treatment, while typha and ocimum showed lower values (Figs. 5–7). It is recommended that more concentration of soluble sugars and protein contents could provide an adaptive mechanism to maintain favorable osmotic potential under metal toxicity [55]. Moreover, more aquatic plants can be tested for their phytoremediation potential to remediate wastewater resources.
In this study, typha showed better performance concerning pollutant uptake from domestic effluents than hibiscus, azolla, ocimum, and salvinia. Interestingly, total free amino acids, total soluble sugars, and total proteins were found higher in hibiscus plant as compared to others under 100% and 50% domestic wastewater treatment, while typha and ocimum showed lower values. A penalty scheme or incentive is required to build up to ensure the quality of domestic water treatment and to phytoremidate the wastewater resources at the community or central level. Further research on domestic wastewater treatment and long term uses for full-scale operation is urgently needed to avoid freshwater scarcity.
Funding Statement: The authors received no specific funding for this study.
Author Contributions: AK conceived the idea. HK and NZ performed the research, and analyze the dataset and prepared the first draft. AR, AR, SA, and AK helped in revising and improving the first draft. All authors reviewed and edited the final draft. All authors have read and approved the final version of the manuscript.
Availability of Data and Materials: The datasets used and/or analyzed during the current study are available from the corresponding author on reasonable request.
Conflicts of Interest: The authors declare that they have no conflicts of interest to report regarding the present study.
References
1. Al-Zahrani, H. S., Alharby, H. F., Fahad, S. (2022). Antioxidative defense system, hormones, and metabolite accumulation in different plant parts of two contrasting rice cultivars as influenced by plant growth regulators under heat stress. Frontiers in Plant Science, 13, 911846. [Google Scholar]
2. Rahi, A. A., Younis, U., Ahmed, N., Ali, M. A., Fahad, S. et al. (2021). Toxicity of Cadmium and nickel in the context of applied activated carbon biochar for improvement in soil fertility. Saudi Journal of Biological Sciences, 29(2), 743–750. [Google Scholar] [PubMed]
3. Bamagoos, A., Alharby, H., Fahad, S. (2021). Biochar coupling with phosphorus fertilization modifies antioxidant activity, osmolyte accumulation and reactive oxygen species synthesis in the leaves and xylem sap of rice cultivars under high-temperature stress. Physiology and Molecular Biology of Plants, 27(9), 2083–2100. [Google Scholar] [PubMed]
4. Mahmood, A., Awan, M. I., Sadaf, S., Mukhtar, A., Wang, X. et al. (2022). Bio-diesel production of sunflower through sulphur management in a semi-arid subtropical environment. Environmental Science and Pollution Research, 29(9), 13268–13278. [Google Scholar] [PubMed]
5. Shahzad, A., Gul, H., Ahsan, M., Wang, D., Fahad, S. (2022). Comparative genetic evaluation of maize inbred lines at seedling and maturity stages under drought stress. Journal of Plant Growth Regulation, 42(2), 989–1005. [Google Scholar]
6. Raza, A., Salehi, H., Rahman, M. A., Zahid, Z., Madadkar Haghjou, M. et al. (2022). Plant hormones and neurotransmitter interactions mediate antioxidant defenses under induced oxidative stress in plants. Frontiers in Plant Science, 13, 961872. [Google Scholar] [PubMed]
7. Raza, A., Mubarik, M. S., Sharif, R., Habib, M., Jabeen, W. et al. (2023). Developing drought-smart, ready-to-grow future crops. The Plant Genome, 16(1), e20279. [Google Scholar] [PubMed]
8. Raza, A., Tabassum, J., Fakhar, A. Z., Sharif, R., Chen, H. et al. (2022). Smart reprograming of plants against salinity stress using modern biotechnological tools. Critical Reviews in Biotechnology, 12, 1–28. [Google Scholar]
9. Nizar, M., Shaukat, K., Zahra, N., Hafeez, M. B., Raza, A. et al. (2022). Exogenous application of salicylic acid and hydrogen peroxide ameliorate cadmium stress in milk thistle by enhancing morpho-physiological attributes grown at two different altitudes. Frontiers in Plant Science, 12, 3157. [Google Scholar]
10. Rezapour, S., Atashpaz, B., Moghaddam, S. S., Damalas, C. A. (2019). Heavy metal bioavailability and accumulation in winter wheat (Triticum aestivum L.) irrigated with treated wastewater in calcareous soils. Science of the Total Environment, 656, 261–269. [Google Scholar] [PubMed]
11. Zahra, N., Hafeez, M. B., Shaukat, K., Wahid, A., Hasanuzzaman, M. (2021a). Fe toxicity in plants: Impacts and remediation. Physiologia Plantarum, 173(1), 201–222. [Google Scholar] [PubMed]
12. Jaramillo, M. F., Restrepo, I. (2017). Wastewater reuse in agriculture: A review about its limitations and benefits. Sustainability, 9(10), 1734. [Google Scholar]
13. Raza, A., Habib, M., Kakavand, S. N., Zahid, Z., Zahra, N. et al. (2020). Phytoremediation of cadmium: Physiological, biochemical, and molecular mechanisms. Biology, 9(7), 177. [Google Scholar] [PubMed]
14. Saud, S., Chen, Y., Long, B., Fahad, S., Saddiq, A. (2013). The different impact on the growth of cool season turf grass under the various conditions on salinity and draught stress. International Journal of Agricultural Science, 3, 77–84. [Google Scholar]
15. Saud, S., Fahad, S., Yajun, C., Ihsan, M. Z., Hammad, H. M. et al. (2017). Effects of nitrogen supply on water stress and recovery mechanisms in Kentucky bluegrass plants. Frontiers in Plant Science, 8, 983. [Google Scholar] [PubMed]
16. Saud, S., Li, X., Chen, Y., Zhang, L., Fahad, S. et al. (2014). Silicon application increases drought tolerance of Kentucky bluegrass by improving plant water relations and morphophysiological functions. The Scientific World Journal, 2014, 1–10. [Google Scholar]
17. Saud, S., Yajun, C., Fahad, S., Hussain, S., Na, L. et al. (2016). Silicate application increases the photosynthesis and its associated metabolic activities in Kentucky bluegrass under drought stress and post-drought recovery. Environmental Science and Pollution Research, 23(17), 17647–17655. [Google Scholar] [PubMed]
18. Shah, S., Li, X., Jiang, Z., Fahad, S., Hassan, S. (2022). Exploration of the phytohormone regulation of energy storage compound accumulation in microalgae. Food and Energy Security, 11(4), e418. [Google Scholar]
19. Wang, D., Fahad, S., Saud, S., Kamran, M., Khan, A. et al. (2019). Morphological acclimation to agronomic manipulation in leaf dispersion and orientation to promote Ideotype breeding: Evidence from 3D visual modeling of super rice (Oryza sativa L.). Plant Physiology and Biochemistry, 135, 499–510. [Google Scholar] [PubMed]
20. Wei, C., Jiao, Q., Agathokleous, E., Liu, H., Li, G. et al. (2022). Hormetic effects of zinc on growth and antioxidant defense system of wheat plants. Science of the Total Environment, 807, 150992. [Google Scholar] [PubMed]
21. Raza, A., Tabassum, J., Zahid, Z., Charagh, S., Bashir, S. et al. (2022). Advances in “Omics” approaches for improving toxic metals/metalloids tolerance in plants. Frontiers in Plant Science, 12, 794373. [Google Scholar] [PubMed]
22. Ghaitidak, D. M., Yadav, K. D. (2013). Characteristics and treatment of greywater—A review. Environmental Science and Pollution Research, 20(5), 2795–2809. [Google Scholar] [PubMed]
23. Howard, G., Bartram, J., Water, S., Organization, W. H. (2003). Domestic water quantity, service level and health. World Health Organization, Geneva. [Google Scholar]
24. FAO (2021). Food and agriculture oganization (AQUASTAT database). In: AQUASTAT Website. http://www.fao.org/aquastat/statistics/query/ [Google Scholar]
25. Wirawan, S. M. S. (2020). Community preparation for domestic wastewater management development in Jakarta. International Journal of Innovative Science and Research Technology, 5, 133–143. [Google Scholar]
26. Va, V., Setiyawan, A. S., Soewondo, P., Putri, D. W. (2018). The characteristics of domestic wastewater from office buildings in Bandung, West Java, Indonesia. Indonesian Journal of Urban and Environmental Technology, 1(2), 199–214. [Google Scholar]
27. Mohiyaden, H. A., Sidek, L. M., Salih, G. H. A., Birima, A. H., Basri, H. et al. (2016). Conventional methods and emerging technologies for urban river water purification plant: A short review. Journal of Engineering and Applied Sciences, 11(4), 2547–2556. [Google Scholar]
28. Forni, C. (2014). Responses of floating macrophytes to different environmental stressors. In: Capello, R. (Ed.Macrophytes: Biodiversity, role in aquatic ecosystems and management strategies, pp. 27–43. New York: Novinka. [Google Scholar]
29. Wulan, D. R., Hamidah, U., Komarulzaman, A., Rosmalina, R. T., Sintawardani, N. (2022). Domestic wastewater in Indonesia: Generation, characteristics and treatment. Environmental Science and Pollution Research, 29(22), 32397–32414. [Google Scholar] [PubMed]
30. Moogouei, R. (2018). Use of terrestrial plants for phytoremediation of pollutants from solutions. Iranian Journal of Science and Technology, Transactions A: Science, 42(4), 1753–1759. [Google Scholar]
31. Ezeogu, N. G., Aisien, F. A. (2021). Effects of phytoremediation treatment method on degradation of bod, COD and TPH in petroleum refinery wastewater. World Journal of Engineering Research and Technology, 7(1), 120–130. [Google Scholar]
32. Watada, A. E., Norris, K. H., Worthington, J. T., Massie, D. R. (1976). Estimation of chlorophyll and carotenoid contents of whole tomato by light absorbance technique. Journal of Food Science, 41(2), 329–332. [Google Scholar]
33. Arnon, D. I. (1949). Copper enzymes in isolated chloroplasts. Polyphenoloxidase in Beta vulgaris. Plant Physiology, 24(1), 1. [Google Scholar] [PubMed]
34. Yemm, E., Willis, A. (1954). The estimation of carbohydrates in plant extracts by anthrone. Biochemical Journal, 57(3), 508–514. [Google Scholar] [PubMed]
35. Bonjoch, N. P., Tamayo, P. R. (2001). Protein content quantification by Bradford method. In: Reigosa Roger, M. (Ed.Handbook of plant ecophysiology techniques, pp. 283–295. Dordrecht, The Netherlands: Springer. [Google Scholar]
36. Hamilton, P. B., van Slyke, D. D., Lemish, S. (1943). The gasometric determination of free amino acids in blood filtrates by the ninhydrin-carbon dioxide method. Journal of Biological Chemistry, 150, 231–250. [Google Scholar]
37. Fahad, S., Bajwa, A. A., Nazir, U., Anjum, S. A., Farooq, A. et al. (2017). Crop production under drought and heat stress: Plant responses and management options. Frontiers in Plant Science, 8, 1147. [Google Scholar] [PubMed]
38. Shahid, S., Kausar, A., Zahra, N., Hafeez, M. B., Raza, A. et al. (2022). Methionine-induced regulation of secondary metabolites and antioxidants in maize (Zea mays L.) subjected to salinity stress. Gesunde Pflanzen, 29, 1–13. [Google Scholar]
39. Wu, C., Song, Y., Qi, B., Fahad, S. (2022). Effects of asymmetric heat on grain quality during the panicle initiation stage in contrasting rice genotypes. Journal of Plant Growth Regulation, 42, 630–636. [Google Scholar]
40. Zahra, N., Al Hinai, M. S., Hafeez, M. B., Rehman, A., Wahid, A. et al. (2022). Regulation of photosynthesis under salt stress and associated tolerance mechanisms. Plant Physiology and Biochemistry, 178, 55–69. [Google Scholar] [PubMed]
41. Zahra, N., Hafeez, M. B., Shaukat, K., Wahid, A., Hussain, S. et al. (2021). Hypoxia and anoxia stress: Plant responses and tolerance mechanisms. Journal of Agronomy and Crop Science, 207(2), 249–284. [Google Scholar]
42. Raza, A., Charagh, S., García-Caparrós, P., Rahman, M. A., Ogwugwa, V. H. et al. (2022). Melatonin-mediated temperature stress tolerance in plants. GM Crops & Food, 13(1), 196–217. [Google Scholar]
43. Farooq, M. S., Uzaiir, M., Raza, A., Habib, M., Xu, Y. et al. (2022). Uncovering the research gaps to alleviate the negative impacts of climate change on food security: A review. Frontiers in Plant Science, 13, 927535. [Google Scholar] [PubMed]
44. Bhardwaj, S., Verma, T., Raza, A., Kapoor, D. (2023). Silicon and nitric oxide-mediated regulation of growth attributes, metabolites and antioxidant defense system of radish (Raphanus sativus L.) under arsenic stress. Phyton-International Journal of Experimental Botany, 92(3), 763–782. https://doi.org/10.32604/phyton.2023.025672 [Google Scholar] [CrossRef]
45. Abdul Aziz, N. I. H., Mohd Hanafiah, M., Halim, N. H., Fidri, P. A. S. (2020). Phytoremediation of TSS, NH3-N and COD from sewage wastewater by Lemna minor L., Salvinia minima, Ipomea aquatica and Centella asiatica. Applied Sciences, 10(16), 5397. [Google Scholar]
46. Amin, M. (2012). Phytoremediation of heavy metals from municipal wastewater by Typhadomingensis. African Journal of Microbiology Research, 6(3), 643–647. [Google Scholar]
47. Sivakumar, D., Nouri, J. (2015). Removal of contaminants in a paper mill effluent by Azolla caroliniana. Scientific Information Database. https://www.sid.ir/paper/343385/en [Google Scholar]
48. Nikam, N., Gaikwad, R. (2021). Phytoremediation of synthetic textile industry effluent using phragmites australis and typha latifolia in constructed wetland. Journal of Indian Water Works Association, 2, 150–152. [Google Scholar]
49. Pratik, P., Nishith, D. (2013). Phytoremediation of sugar industry effluent using typha angustata and phragmites australis through constructed wetland. Journal of Chemical, Biological and Physical Sciences, 4(1), 846–851. [Google Scholar]
50. Fan, H., Wang, K., Wang, C., Yu, F., He, X. et al. (2020). A comparative study on growth characters and nutrients removal from wastewater by two microalgae under optimized light regimes. Environmental Technology & Innovation, 19(8), 100849. [Google Scholar]
51. Gupta, S., Satpati, S., Nayek, S., Garai, D. (2010). Effect of wastewater irrigation on vegetables in relation to bioaccumulation of heavy metals and biochemical changes. Environmental Monitoring and Assessment, 165(1), 169–177. [Google Scholar] [PubMed]
52. Jeandet, P., Formela-Luboińska, M., Labudda, M., Morkunas, I. (2022). The role of sugars in plant responses to stress and their regulatory function during development. International Journal of Molecular Sciences, 23(9), 5161. [Google Scholar] [PubMed]
53. Kosová, K., Vítámvás, P., Urban, M. O., Prášil, I. T., Renaut, J. (2018). Plant abiotic stress proteomics: The major factors determining alterations in cellular proteome. Frontiers in Plant Science, 9, 122. [Google Scholar]
54. Raza, A., Hussain, S., Javed, R., Hafeez, M. B., Hasanuzzaman, M. (2021). Antioxidant defense systems and remediation of metal toxicity in plants. In: Approaches to the remediation of inorganic pollutants, pp. 91–124. Singapore: Springer. [Google Scholar]
55. Verma, S., Dubey, R. (2001). Effect of cadmium on soluble sugars and enzymes of their metabolism in rice. Biologia Plantarum, 44(1), 117–123. [Google Scholar]
Cite This Article
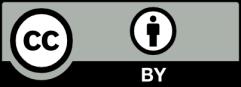