Open Access
ARTICLE
Profiles of the Headspace Volatile Organic and Essential Oil Compounds from the Tunisian Cardaria draba (L.) Desv. and Its Leaf and Stem Epidermal Micromorphology
1 Laboratory of Biodiversity, Biotechnology and Climate Change (LR11-ES09), Faculty of Sciences of Tunis, University of Tunis El Manar, Tunis, 1060, Tunisia
2 Laboratory RME-Resources, Materials and Ecosystems, Faculty of Sciences of Bizerte, University of Carthage, Jarzouna, Bizerte, 7021, Tunisia
3 Dipartimento di Farmacia, Università di Pisa, Via Bonanno 33, Pisa, 56126, Italy
4 Laboratory of Bioresources: Integrative Biology and Valorization (LR14-ES06), High Institute of Biotechnology of Monastir, University of Monastir, Monastir, 5000, Tunisia
* Corresponding Author: Noomene Sleimi. Email:
Phyton-International Journal of Experimental Botany 2024, 93(4), 725-744. https://doi.org/10.32604/phyton.2024.048110
Received 28 November 2023; Accepted 19 March 2024; Issue published 29 April 2024
Abstract
In this work, we investigated aroma volatiles emanated by dry roots, stems, leaves, flowers, and fruits of Cardaria draba (L.) Desv. growing wild in Tunisia and its aerial part essential oils (EOs) composition. A total of 37 volatile organic compounds (96.7%–98.9%) were identified; 4 esters, 4 alcohols, 7 hydrocarbons, 12 aldehydes, 5 ketones, 1 lactone, 1 organosulfur compound, 2 organonitrogen compounds, and 1 acid. The hydrocarbons form the main group, representing 49.5%–84.6% of the total detected volatiles. The main constituent was 2,2,4,6,6-pentamethylheptane (44.5%–76.2%) reaching the highest relative percentages. Forty-two compounds were determined in the two fractions of EOs, representing 98.8% and 97.2% of the total oil composition, respectively. The principal components were hexadecanoic acid (34.6%), 6-methyl-5-hepten-2-one (18.3%), decanal (15.0%), 6,10,14-trimethyl-2-pentadecanone (13.2%), and n-pentacosane (13%). Micromorphological details of the leaf and stem epidermis using light microscopy revealed polygonal cells with sinuous walls in the adaxial and abaxial leaf surfaces and nearly rectangular and long ones with linear and thick walls for the stem epidermis. The stomata complexes were anisocytic in the leaf epidermis and mainly anisocytic and rarely paracytic in the stem epidermis. Non-glandular trichomes were unbranched and long with an acute apex or short with a convex apex. The glandular ones were identified for the first time in this species. They were short-stalked with a large secretory head. The highest stomatal index (17.02%) was recorded in the abaxial leaf surface. The identification of headspace volatiles and essential oil compounds can be used to characterize this species, and the various epidermis micromorphological features are very useful for biosystematics taxonomic studies within Brassicaceae.Keywords
Plants can produce and release natural fragrant aroma molecules widely used in food, pharmaceutical, agricultural, chemical, and cosmetic perfumery industries [1]. Currently, numerous wild species belonging to various families are used due to their richness in natural volatile compounds. Among them, the Brassicaceae Burnett is one of the prominent plant families of vegetal crops and medicinal plants having economic, agricultural, and scientific importance [2]. It comprises about 321 genera and more than 3660 species [3]. Cardaria Desv. is the largest genus including at least 250 species spread over the Americas, Africa, Asia, Europe, and Australia [2]. Cardaria draba (L.) Desv. (common name White top) [4,5] is indigenous to Eurasia and the Mediterranean area [6]. It is an herbaceous and spontaneous plant growing along roadsides and in sub-irrigated pastures [7]. Stems and leaves are covered by soft white trichomes [8]. Numerous fragrant white flowers are disposed of in a corymb inflorescence. Long fruiting pedicels carry indehiscent heart-shaped silicles [4].
White top is considered as an edible species of high nutritional value [9]. The plant decoction exhibits diuretic properties and previously, the fruits were employed as a condiment [10]. Young leaves have also forage value, given their higher content of protein [11]. Moreover, it is an allelopathic plant and is considered as a hyperaccumulator of heavy metals with a great phytoremediation potential [12–14]. Furthermore, C. draba is a high-value wild medicinal plant widely used by drug manufacturing given the richness of its extracts in alkaloid, phenol, flavonoid, terpenoid, and glucosinolate compounds with strong antioxidant, antibacterial, antimicrobial, antifungal, analgesic and antidiabetic activities [15–21].
Despite the numerous health benefits of essential oils (EOs) and volatile organic compounds (VOCs) in aroma therapy, medicinal uses, and food preservation due to their great biological potential, there is a lack of information concerning the qualitative and quantitative compositions of C. draba volatiles. Previous studies have been limited to C. draba particularly growing wild in Croatia, Iran, Turkey, and Algeria. The chemical composition of the volatiles was characterized by the predominance of 3-butenyl isothiocyanate, 4-methyl sulfinylbutyl isothiocyanate, 5-methylthio-pentanenitrile, bis (2-ethylhexyl) phthalate, 6,10,14-trimethyl-2-pentadecanone, and (E)-phytol [10,22–25].
To the best of our knowledge, no work has previously been done on Tunisian C. draba, particularly on its volatile profiles. Previous works focused on the fungal pathogens affecting this Tunisian species [26]. These volatile compounds are produced by specific secretory tissues known as glandular trichomes important for phylogenetic and biosystematic studies of many plants. Epiderm micromorphology studies of C. draba were focused only on Iraqi and Iranian species [27–29].
In this sense, this study aimed firstly to characterize the chemical compositions of the VOCs of roots, stems, leaves, flowers, and fruits, and of the EOs extracted from the aerial parts (leaves, stems, and flowers) of C. draba. They are important to precise the specificity of the Tunisian species in terms of aroma compounds commonly used in fragrance, cosmetic, pharmaceutical, and agri-food industries, as nutritional supplements. Secondly, we focused on the investigation of the micromorphological leaf and stem epidermal properties, to identify and describe the responsible secretory structures of these volatile compounds which are unidentified in this species and even in other species of the Brassicaceae family.
Plant samples of Cardaria draba (fresh leaves, stems, flowers, and roots) were collected in spring (March 2023) during the full flowering stage from populations growing wild in the Ksour-Essaf site (Latitude: 35°25′.02 Nord, Longitude: 10°59′.694 East, elevation: 15 m), located at 17 km from Mahdia; Center-East of Tunisia. Two months later (May 2023) fresh fruits were collected from the same population. Voucher specimens (N°124Cd1-5) authenticated by the botanist Pr. Fethia Harzallah-Skhiri was maintained in the herbarium of the Laboratory of Bioresources: Integrative Biology and Valorization (LR14-ES06), High Institute of Biotechnology of Monastir, Tunisia. In the laboratory, the collected material was dried at room temperature in the dark after separating leaves, flowers, fruits, and roots from the stem, then, ground into a homogenous fine-grade powder using an electric grinder (Duronic CG 250 Premium 250 W) and weighed. The obtained powders were placed in glass jars until use. In addition, a part of the fresh organs (leaves and stems) was kept aside for micromorphological investigations of foliar and stem epidermis.
Given that hydrodistillation of separate C. draba aerial parts did not yield essential oil, we mixed the dried powders of stems, leaves, and flowers. For each organ, we used 50 g. Hydrodistillation of 4 × 150 g from this mixture was conducted during 4 h with a Clevenger-type apparatus. The hydrodistillation afforded two separate and successive EO fractions. Fraction 1 (EOFr1) was obtained after 3 h of extraction, followed by the second one (Fraction 2; EOFr2), after 4 h. The two fractions were dried using anhydrous sodium sulfate (anh. Na2SO4) and subsequently weighed. The calculated essential oil fraction yield was expressed in % (v/w) concerning the dried weight. The obtained essential oil fractions were stored at 4°C in glass vials until analysis.
2.3 Headspace Solid-Phase Micro-Extraction (HS-SPME)
The Headspace analysis was used for screening of volatile organic compound (VOCs) profiles of 0.2 g of each root, stem, leaf, flower, and fruit dry powders with Solid Phase Micro-extraction (SPME) devices as described by Arbia et al. [30]. Briefly, after an equilibration period, the fiber was introduced to the headspace for 10 s then withdrawn into the needle, and then transferred to the GC/MS injector. Quantitative comparisons of relative peak areas were performed between the same chemicals in the different samples. Identification of the constituents was based on a comparison of the retention times with those of authentic samples, comparing their linear retention indices relative to the series of n-hydrocarbons, and on computer matching against commercial and home-made library mass spectra built up from pure substances and components. GC/EI-MS analyses were performed with a Varian CP-3800 gas chromatograph using a DB-5 capillary column (30 m × 0.25 mm; coating thickness 0.25 um) and a Varian Saturn 2000 mass detector. Analytical conditions: injector and transfer line temperatures 220°C and 240°C, respectively; oven temperature programmed from 60°C to 240°C at 3°C/min; carrier gas helium at 1 ml/min; for essential oils: injection of 0.2 μl (10% hexane solution); split ratio 1:30; for SPME: injector temperature 250°C, splitless injection.
2.4 Preparing Epidermis Samples
To procure epidermal cells, a small incision of fresh stems and leaves where made with a scalpel, perpendicularly to the axis of the leaf and the stem. Thin and transparent pellicles of the epidermis layer from the stem and from both the abaxial and adaxial surfaces of the leaf were removed using fine forceps and then placed on a drop of water on a microscope glass slide. The obtained slides with the epidermis samples were observed under a light microscope from Leica Microsystems. The number, density, and distribution of stomata, epidermis cells, and trichomes are recorded in fifteen-unit areas, of 1 mm2 of surface, randomly selected on the stem and on both leaf surfaces. Micrographs were taken from different regions of the sections using ×10, ×40, and ×100 magnifications.
To calculate the Stomatal index, the formula mentioned in Ditcher [31] was used. See Eq. (1).
S: stomata number per unit area (1 mm2); E: epidermal cells number in the same unit area.
The epidermal cell number, stomata, and trichomes of the stem and both adaxial and abaxial leaf surfaces were investigated using analysis of variance (ANOVA) with SPSS 13.0 for Windows (SPSS Inc. Chicago, II, USA). The results are expressed as the mean ± standard deviation (SD) in the lower and upper limits, followed by a mean value. The p < 0.05 was considered to indicate a significant statistical difference using Duncan’s multiple range test.
3.1 Headspace Volatile Organic Compounds from Five Cardaria draba Organs
A total of 37 VOCs emanating from C. draba organs, representing 96.7%–98.9% of the total composition, were recorded (Table 1). Extracted volatile compounds belonged to several chemical groups that included 4 esters, 4 alcohols, 7 hydrocarbons, 12 aldehydes, 5 ketones, 1 lactone, 1 organosulfur compound, 2 organonitrogen compound, and 1 acid. The hydrocarbons form the main group, representing 49.5%–84.6% of total detected volatiles.
In the headspace of the root powder, 16 VOCs were isolated corresponding to 97.6% of the total volatiles. These compounds were detected in low to high proportions (0.3%–76.2%). They have been classified as 4 hydrocarbons (84.6%), 7 aldehydes (11.1%), 3 ketones (1.1%), 1 organonitrogen compound (0.4%), and 1 alcohol (0.4%). The major compound was identified as a hydrocarbon; 2,2,4,6,6-pentamethylheptane (No 10%, 76.2%), followed by limonene (11%, 7.5%) and (E, E)-2,4-decadienal (26%, 3.4%). Fifteen VOCs were common with the stem, such as 1-hexanol (5), n-dodecane (14), and benzyl nitrile (36), and nine compounds were in common with the stem, leaf, flower, and fruit powder, no specific root VOCs were identified. For the stem headspace powder, 26 constituents were detected (96.7%) identified as 7 hydrocarbons (74.5%), 9 aldehydes (14.3%), 5 ketones (4.7%), 1 organosulfur (1.4%), 2 alcohols (0.9%), 1 lactone (0.6%), and 1 organonitrogen (0.3%). As in root, 2,2,4,6,6-pentamethylheptane (10%, 63.5%), limonene (11%, 7.4%), nonanal (20%, 3.9%) and (E, E)-2,4-decadienal (26%, 3.4%) were the main component. Moreover, 2 trace volatile constituents were specific to the stem; β-pinene (9%, 0.3%) and heptanal (17%, 0.2%). On the other hand, 23 compounds (98.4%) were extracted in the leaf powder: 2 hydrocarbons (50.8%), 9 aldehydes (16.8%), 5 ketones (17.5%), 2 alcohols (5.3%), 1 lactone (4.4%), 2 esters (2.0%), 1 organosulfur compound (1.2%), and 1 organonitrogen compound (0.4%). Dihydrolinalyl acetate (ester, 4%, 0.6%) and 2-ethylpyridine (35%, 0.4%) were specific to leaf powder. The major components were 2,2,4,6,6-pentamethylheptane (10%, 46.2%), (E)-β-ionone (32%, 7.8%), limonene (11%, 4.6%), dihydroactinidiolide (lactone, 33%, 4.4%), and (E, E)-2,4-decadienal (26%, 4.0%). The 2,2,4,6,6-pentamethylheptane percentages (46.2%) were lower than those recorded in the root and stem powders. Methyl valerate (1%, 1.4%), safranal (21%,1.4%), and β-cyclocitral (23%, 1.9%) were only common with flower and fruit samples. However, benzyl alcohol (6%, 2.0%) was only common with fruit powder. Twenty-four compounds (97.5%) were identified in the dried flowers. Three were specific to this organ, particularly hexanoic acid (37) reaching 4.2%. The main constituent was 2,2,4,6,6-pentamethylheptane (10%; 44.5%) followed by dimethyl sulfoxide (34%; 8%). Among the 25 constituents in the fruit powder (98.9%), the main one was 2,2,4,6,6-pentamethylheptane (10%, 58%) as is the case for all the other samples. Limonene (11), nonanal (20), and (E, E)-2,4-decadienal (26) were detected at 5.4%, 3.8%, and 3.6%, respectively. None of the VOCs was specific to fruits.
In the headspace analyses for all the plant parts, esters were absent in root and stem VOCs, acids were present only in VOCs flowers, organosulfur compound, and lactone were absent in VOCs roots, and organonitrogen compounds were absent in leaves VOCs. The aliphatic hydrocarbon 2,2,4,6,6-pentamethylheptane (10), known as isododecane, largely contributed to the smell of C. draba organs and was the most plentiful constituent of VOCs extracted from roots (76.2%), stems (63.5%), and fruits (58.0%). According to Bell et al. [32], non-glucosinolate-derived compounds can contribute significantly to the Brassicaceae species aromas. The 2,2,4,6,6-pentamethylheptane component is known for its low odor and toxicity and it is a key ingredient in the cosmetics market as a fragrance agent [33]. Furthermore, this compound (10) has been reported previously belonging to the cattle and lamb meats’ volatile profile having a characteristic odor [34]. In previous studies, this component has never been detected in the volatile emission profiles of C. draba. However, Bell et al. [32] determined volatile and odorant compounds in the headspace of four Brassicaceae species; Eruca sativa Mill., Armoracia rusticana G. Gaertn., Eutrema japonicum (Sieb.), and Nasturtium officinale L. Among the identified compounds, 2,2,4,6,6-pentamethylheptane was only detected in N. officinale but in very low content (0.69%). Besides, it was also detected among the highest contributing components and the source of fruity aromas of 9 types of Fenghuang Dancong tea, reaching 20.32% [35].
The second most important hydrocarbon in our samples is limonene (11), classified as a cyclic monoterpene. It is detected in all organ powders but mainly in roots and stems. According to Erasto et al. [36], it is among the frequently encountered volatile components of Citrus. Its occurrence could be attributed to its defensive role against herbivores. It possesses a pleasant lemon-like odor, which makes it extensively employed as a flavor additive in common food and cosmetics formulations. Its isomer D-Limonene occurs more commonly in 12 Brassicaceae vegetables such as Brussels sprouts, broccoli, radish, and cherry [37] and in B. rapa L. [38]. Limonene reached 21.1% of the VOCs identified in the floral scent of B. oleracea [39].
In the headspace of the different analyzed powders, except this from roots, a ketone (E)-β-ionone (32) was detected in moderate abundance (7.8%–1.0%). The name ionone is derived from the Greek term iona meaning violet odor. It is a natural plant component derived from the degradation of carotenoids [40]. In recent years, according to Paparella et al. [41], β-ionone has antibacterial and fungicidal properties and an important potential as an anticancer treatment. It is also considered as an attractant or repellent of insects and is released following wounding by herbivores.
Dimethyl sulfoxide (34) was the only volatile sulfur compound detected in stems, leaves flowers, and fruit volatiles. It seems to have a moderate contribution, particularly, to the smell of C. draba flowers (8%). According to Zhou et al. [42], it could have originated from isothiocyanate responsible for its onion, cabbage, garlic, and sulfurous-pungent-like odors. This flavoring agent has different pharmaceutical applications and is an important contributor to the flavors in food industries [43]. Previously, it was detected by Nadaf et al. [24] in the volatiles of the Iranian C. draba aerial parts, but with a lower percentage (3.6%) than that recorded in our study.
According to Smart et al. [44], phenyl ethyl alcohol (8), and benzyl alcohol (6) which have been detected in flowers or fruit have a role of pollinator attraction in Brassicaceae species and characterize the flower fragrance of several other species. They are among the aroma compounds of three B. juncea L. accessions leaves [45]. Moreover, benzyl alcohol and phenyl ethyl alcohol are some of the volatiles identified in the fragrance of B. nigra (L.) Koch flowers (0.5%; 2.6%, respectively), B. oleracea L. (2.2%; 19.5%, respectively), B. juncea (0.3% 2.4%, respectively), B. napus (0.8%; 22.3%, respectively), and B. carinata A. Braun (1.3%; 11.6%, respectively) [39]. Nonanal (20) contributed also to the aroma of C. draba organs (2.3%–3.9%). It is known for its contribution to the nectar scents of certain flowers such as those of Orchids attracting mosquitoes [46].
3.2 Chemical Composition of Cardaria draba Aerial Parts Essential Oil
EOFr1 was colorless and had an intense and pungent aroma. Whilst, the EOFr2 showed a pale-yellow color with low odor strength and solidified when in contact with air. They appeared to contain both fragrant molecules and cuticular waxes. The yields of EOFr1 and EOFr2 were 0.031% and 0.010% (v/w), respectively.
3.2.1 Chemical Composition of the EO Fractions
A total of 42 chemical compounds (22 detected in the EOFr1 and 20 in the EOFr2) were identified. They account for 98.8% and 97.2% of the total EO composition, respectively. The identified EO compounds and their relative contents are presented in Table 2.
3.2.2 Chemical Composition of the EOFr1
Among the 22 compounds detected in the EOFr1 (Table 2), 6-methyl-5-hepten-2-one (2) followed by decanal (11) showed the highest percentages (18.3% and 15%, respectively). Three additional compounds were identified at moderate levels; nonanal (8), α-bisabolol oxide A (22), and (E)-geranyl acetone (14) (9.8%, 9.2%, and 7.7%, respectively). α-bisabolone oxide A (19), butyrolactone (1), and α-bisabolol oxide B (18) were represented in low relative contents in the EOFr1 (5.2%, 4.6%, and 4.1%, respectively). All the 14 other compounds were detected in very low relative abundances not exceeding 2.8%.
The main compound of the EOFr1; 6-methyl-5-hepten-2-one (syn. sulcatone) (2), is an unsaturated methylated ketone. It is a floral compound produced by over 400 plant species and characterized by its Citrus odor representing the principal component of Citronella, Lemon-grass, and Palma rosa EOs [47]. It acts as an anti-leukemic and induces apoptosis [48]. Decanal is a saturated fatty aldehyde with a pleasant sweet, waxy, floral, citrus, and pronounced odor [49]. It has a powerful role as an antifungal agent [50]. Nonanal is a saturated fatty aldehyde characterized by an orange-rose odor. It is a prevalent volatile compound found in at least 20 EOs, including Rose, Citrus, and Pine ones [51]. It is used in the perfume industry [52]. α-Bisabolol oxide A is a significant sesquiterpene alcohol. It is derived naturally from EOs of various ornamental and edible species [53]. It has a fruity nutty aroma close to coconut [54]. Its most important biological activities are its anti-inflammatory, anti-irritant, anti-bacterial, anti-cancer, and non-allergenic properties. It is also known for its neuro-, cardio-and nephro-protective and analgesic effects [54]. (E)-Geranyl acetone plays various roles in insect and plant interactions [55]. It is a flavoring agent having an apple-like fruity aroma [56]. Limonene (4) was detected in a low percentage (2.8%) confirming the results found by Bayan [22] where this component is present in the EO of C. draba aerial parts with percentages not exceeding 3.23%. However, the EO seed of Brassica napus L. was predominantly made up of limonene (90%) [57]. Another component, β-ionone (16), generally synthesized at low concentrations in plants, is present at a low percentage (1.4%). It was previously found in the EOs of the Algerian C. draba (2.01% and 2.57% in the aerial parts and leaves, respectively) [25] and of the Croatian species (1.5% in a mixture of leaves and flowering heads) [23].
3.2.3 Chemical Composition of the EOFr2
In EOFr2, 20 specific compounds were found. The 6,10,14-trimethyl-2-pentadecanone (25) (Table 2) common to the two fractions, was detected in high relative content (13.2% against 1.5% in EOFr1). The main constituent of the EOFr2 was hexadecanoic acid (31%; 34.6%), also called palmitic acid; the most common saturated long-chain fatty acid found in plants, animals, and microorganisms [58]. It is considered an important cuticular wax compound in plants [59]. It is used as an insecticide and an acaricide, and it has several benefits on skin health due to its anti-inflammatory properties [60]. It is followed by the 6,10,14-trimethyl pentadecan-2-one compound (25%; 13.2%) characterized by an odor and a tasting of Jasmin and celery woody [61]. It is the major constituent of numerous aromatic and medicinal plants EOs. It has antibacterial, anti-nociceptive, and anti-inflammation activities [62]. This latter compound is followed by n-pentacosane (42%; 13%) known for its anticancer, antimicrobial, antifungal, anti-inflammatory, antioxidant, and antiviral activities [63]. Moreover, eicosanoic acid (syn. arachidic acid) (40) was detected in moderate percentage (9.6%). It is a saturated long-chain fatty acid essential to brain development, repair, and maintenance, and neuron protection [37]. n-Tricosane was also detected in moderate percentage (40) (6.6%). The other components were present in low to very low relative abundances (0.2%–4.9%).
3.2.4 Chemical Classes Variation in the Two Cardaria draba Aerial Parts EO Fractions
The types and contents of the different chemical classes of the aerial parts EO components are reported in Table 2. Six classes were detected for the EOFr1 constituents, against 3 ones for those for EOFr2. The two fractions are rich in non-terpene derivative compounds, 83.3% for EOFr2, and 58.3% for EOFr1. Sesquiterpene hydrocarbons, monoterpene, and oxygenated monoterpenes and sesquiterpenes were specific to the EOFr1. Among the main oxygenated sesquiterpenes, compounds 18, 19, and 22 are particularly significant. However, the diterpenes chemical class was specific to the EOFr2 and represented by the isophytol (30). Percentages in apo carotenes constituents were almost identical in the two fractions represented mainly by compound 25 (13.2%) for EOFr2 and by particularly compound 14 for EOFr1. There was notable variation within the EO fraction. During the first hours of extraction, more volatile smaller compounds such as monoterpenes and sesquiterpenes are extracted, and in later periods larger fewer volatile compounds are extracted, such as alkanes.
Comparing our results with those of the published studies, qualitative and quantitative differences emerge. The EO composition exhibits significant variation depending on the geographical location and climate conditions [64]. The composition of the EOs of C. draba collected in Iran was different. Afsharypuor et al. [10] described a composition mainly dominated by 3-butenyl isothiocyanate (80.5%) for the EO derived from flowering aerial parts, the 4-methyl sulfinylbutyl isothiocyanate for the fruit and root EOs (72.1% and 30%, respectively), and hexadecanoic acid for the root EO (24.1%). All these compounds are not detected in Tunisian C. draba, except hexadecanoic acid (31) which has been detected at a slightly higher percentage (34.6%). The main volatile compounds isolated from the aerial parts of C. draba from Croatia, were 4-methylsulfanylbutyl isothiocyanate (28%), pentanenitrile (19.2%), hexadecanoic acid (10.8%), phytol (10.2%), and dibutyl phthalate (4.5%) [65]. Moreover, as specified by Kazemi et al. [66], the main components of the aerial and flowering parts EO of C. draba from Iran were Z-phytol (12.9%) and β-ionone (4.5%). In our study, we have also found these two compounds but in lower amounts (2.4% and 1.4%, respectively). For Nadaf et al. [24], the EO aerial parts collected at the flowering stage contain mainly bis2-ethylhexylphthalate (12.5%), dicyclohexyl-propanedinitrile (7.4%), dodecane (6.3%), 9,12,15-octadecatriene-1-ol (5.2%), tridecane (4.8%), and decahydro-1,6-dimethyl-naphthalene (4.6%). All these compounds are not identified in our study. The EOs of the air-dried leaves and the flowering parts of the Algerian Wite top exhibited the existence of two main constituents; 6,10,14-trimethyl-2-pentadecanone (11.08% and 20.61%, respectively) and (E)-phytol (39.67% and 11.38%, respectively) contributing to the high antioxidant capacity of those EOs [25]. In our work, 6,10,14-trimethyl-2-pentadecanone was also predominant in the EOFr2 (25; 13.2%). Phytol component (35) was identified in a lower percentage (2.4%). For the five compounds, 6-methyl-5-hepten-2-one (2), decanal (11), nonanal (8), (E)-gerany lacetone (14) and (E)-β-ionone (16) higher quantities were found in Algerian species [25]. The major constituents of the C. draba EO growing in Turkey were 5-methylthio-pentanenitrile (41.13%), decane (11.40%), and nonane (10.93%) [22]. These components were not detected in the specific EO profiles of the Tunisian species.
3.3 Micromorphological Features of Epidermal Cells, Stomatal Complexes, and Trichomes of C. draba Leaf and Stem
3.3.1 Leaf and Stem Epidermal Cells
Micromorphological features of epidermal cells in adaxial and abaxial leaf and stem surfaces are presented in Fig. 1. In the leaf, the epidermal cells have an irregular shape. On both surfaces, they were polygonal with sinuous walls. Walls of epidermal cells, on the leaf adaxial surface, seem to be slightly more undulating than those of the abaxial surface (Figs. 1a–1e). For Doaigey et al. [67], leaf epidermal cells of C. draba of Saudia Arabia are sinuous with thick anticlinal cell walls. For Iraqi C. draba leaf epidermal cells, Esmaeel et al. [27], described them as polygonal with feebly sinuous walls. Al-Saadi et al. [29] showed that the anatomical features of the leaf epidermal cells varied widely in 13 different Brassicaceae species. The cell walls can be thicker or thin, strongly undulate anticlinal or sinuous anticlinal according to the species. For C. draba, they are straight-sinuate anticlinal. Bibi et al. [68] studied the evolutionary and taxonomic potential of microscopic features in the leaf epidermis of 27 Pakistan Brassicaceae species. They noted the presence, often, of epidermal cells varied in form, encompassing polygonal, pentagonal, hexagonal, and tubular shapes. Leaf epidermal features have potential taxonomic significance. They help delimit the related taxa in Brassicaceae [69], particularly the sinuous character of epidermal cells [70].
Figure 1: Epidermal characters in C. draba leaf and stem surfaces (Scale 50 µm). Epidermal cells and stomatal complexes with anisocytic stomatas on adaxial (a–c) and on abaxial (d, e) leaf surfaces; epidermal cells and stomatal complexes on stem surface (f–i); anisocytic stomatas (g) and paracytic stomatas (h, i) on stem epidermal surface. (a, d) ×40; (b, e, f, g) ×100; (c, h, i) ×400
On the contrary, the form of stem epidermal cells was nearly rectangular, long, and tapered with linear and thick walls. They were much longer than wide (Figs. 1f–1i). Mousavi et al. [28] previously described in cross-section of C. draba stem, rectangular epidermal cells. For Esmaeel et al. [27], the shape of the epidermal cells of the stem was oblong to longley with straight walls.
3.3.2 Leaf and Stem Stomatal Complexes
In the examined C. draba leaf epidermis, the shape of the stomata was elliptic to elliptic-oblong. The stomatal complex, consisting of the stoma plus adjacent epidermal cells, was anisocytic on both adaxial and abaxial leaf surfaces of the Tunisian C. draba. It is characterized by three unequally sized subsidiary cells, easily distinct from other epidermal cells, accompanying the pair of guard cells. Among these three cells, one is comparatively smaller than the other two (Figs. 1c, 1e). Anisocytic stomata are created when the meristemoid divides two more times asymmetrically, forming surrounding subsidiary cells [71]. According to Al-Saadi et al. [29], the anisocytic type is dominant but other types can intermix in other Brassicaceae species. The stomatal complex variation type seems to be about the ecological environments [69]. Our result agreed with those of Esmaeel et al. [27] in the leaf epidermis of C. draba growing wild in Iraq, and in other Cardaria species [72]. For Bibi et al. [68], three various stomata types were identified in the leaf epidermis of Pakistan Brassicaceae species; anisocytic, anomocytic, and staurocytic. According to Doaigey et al. [67], the stomata are frequently anisocytic in the leaf epidermis of 34 Brassicaceae species but combined with anomocytic type in 21 species.
Microscopic investigation of the Tunisian C. draba stem epidermis revealed two different types of stomata complexes; mainly anisocytic (Fig. 1g) and rarely paracytic ones (Figs. 1h, 1i). As defined by van Cotthem [73], the paracytic type includes side subsidiary cells oriented parallel to the guard cells. The sole previous work carried out by Esmaeel et al. [27] on the stem epidermis of C. draba in Iraq confirmed the occurrence of both anisocytic and paracytic stomata complexes in the stem epidermis of this species.
In the present investigation non-glandular trichomes have been identified in each epidermis face of the leaf (Fig. 2) and that of the stem (Fig. 3). All trichomes are surrounded by thick walls. Two types of spaced unicellular and unbranched non-glandular trichomes were recorded. The first type was long with an acute apex (type 1) (Figs. 2a–2d, 2j–2land 3a–3f) and the second one was short with a convex apex (type 2) (Figs. 2e–2g, 2m, 2nand 3g–3j).
Figure 2: Leaf epidermal trichomes of C. draba (Scale 50 µm). Adaxial leaf surface non-glandular type 1 (a–d), non-glandular type 2 (e–g), and glandular (h, i) trichomes. Abaxial leaf surface non-glandular type 1 (j–l), non-glandular type 2 (m, n), and glandular (o, p) trichomes. (a) ×40; (b, e) ×100; (c, d, f, g, h, i, k, l, m, n, o, p) ×400
Figure 3: Stem epidermal trichomes of C. draba (Scale 50 µm). Non-glandular trichomes type 1 (a–f) and type 2 (g–j). Glandular trichome (k). (a, b) ×40; (c) ×100; (d–k) ×400
Previous works revealed that trichomes in the leaf and stem epidermis of C. draba are non-glandular, short and long single-celled, conical, warty, and very dispersed [27,28]. They are backed with our results.
Glandular trichomes are identified in the leaf and stem epidermis. They are capitate type, short-stalked with a large secretory head (Figs. 2h, 2i, 2o, 2pand Fig. 3k). They are the site of production of specialized metabolites largely used as pesticides, fragrances, and pharmaceutical ingredients [74]. It is important to note that in the previous studies carried out on C. draba, glandular trichomes have never been reported contrary to our work. Ilyinska [75] recorded the occurrence of glandular structures in 14 species of Brassicaceae other than C. draba. The presence or absence of trichomes, the size, and the shape change depending on geographic location and climatic conditions have been considered important for the identification of Brassica species [76].
3.3.4 Density of Epidermal Cells, Stomata, and Trichomes in Leaf and Stem
The average numbers of the leaf epidermal cells are more important (996.0 cells/mm2 against 967.4 cells/mm2 in the adaxial and abaxial leaf surfaces, respectively) than that of the stem surface (755.2 cells/mm2) (Table 3). These numbers are slightly elevated compared to those recorded by Al-Saadi et al. [29] on the leaf epidermis of C. draba (924.0 cells/mm2 and 900.0 cells/mm2 on the adaxial and the abaxial one, respectively). These authors reported that the epidermal cells number varies in 12 other Brassicaceae species. On the adaxial leaf epidermis, it ranged between 420.0 cells/mm2 in Lobularia maritima (L.) Desv. and 1920.8 cells/mm2 in Nasturtium officinale W.T.Aiton. On the abaxial epidermis, it varied from 361.0 cells/mm2 in L. maritima to 1665.0 cells/mm2 in Sinapis alba L.
Concerning the counted number of stomata, it was higher in the abaxial surface of the leaf epidermis (198.4/mm2), followed by the adaxial one (182.2/mm2) and then in the stem (42.6/mm2) (Table 3). Al-Saadi et al. [29] also found that the stomata number of the abaxial leaf epidermis (186.23/mm2) of C. draba was slightly higher than that of the adaxial one (184.52/mm2).
In our study, the highest stomatal index percent (density of stomata relative to the epidermal cell count), was 17.02% recorded on the abaxial surface of the leaf, followed by 15.46% on the adaxial surface of the leaf and the lowest index (5.34%) was found in stem surface epidermis (Table 3). Comparable findings were reported by Al-Saadi et al. [29] for C. draba. The stomatal indexes of the abaxial and adaxial leaf faces were 17.14% and 16.64%, respectively. However, in previous work on C. draba epidermis, Esmaeel et al. [27] reported stomatal indexes higher than those found in our study. They reached 26.66%, 44.44%, and 16.66% in abaxial, adaxial leaf, and stem surfaces, respectively. These differences can be explained by the species-specific adaptation influenced by both genetic and environmental factors [77]. In addition, Segev et al. [78] found a correlation between photosynthesis and the stomatal index. An important value of the stomatal index correlates to a higher photosynthesis rate. According to Huma et al. [79], the stomata numbers, types, distribution, and frequencies show environmental conditions.
The trichome counting in both leaf surfaces, and stem epidermis, revealed that the average numbers of non-glandular trichomes are much higher than the glandular ones (113.2, 87.2, and 96.6/mm2 against 6.4, 3.4, and 2.2/mm2, respectively). The adaxial leaf surface epidermis was the richest in non-glandular and glandular trichomes (113.2 and 6.4, respectively). The non-glandular trichome results are in concordance with those recorded by Al-Saadi et al. [29] and Ilyinska [75] except for the glandular ones which have not been identified.
The present research work is the first report on the chemical composition of the headspace aroma and essential oils from several organs of the wild Tunisian Cardaria draba. It provides additional information about the selection of raw materials and odor markers in C. draba fragrant volatile compounds and essential oils, particularly root and stem VOCs. Several compounds in high quantities in essential oils were recorded. They have medicinal potential and could be introduced for applications in agri-food, cosmetic, and flavor factories. However, to fully use this species as a new source, large quantities of plant material are needed because of its low yield. Additionally, the study improves knowledge of C. draba leaf and stem epidermal characteristics which are useful anatomical tools and criteria to support taxonomic studies. Glandular trichomes are identified as secretory structures of volatiles for the first time in this Brassicaceae species.
Acknowledgement: Not applicable.
Funding Statement: The authors received no specific funding for this study.
Author Contributions: Study conception and design: S. Stambouli-Essassi, F. Harzallah-Skhiri; data collection: G. Flamini, R. Ascrizzi, S. Kahlaoui, K. Hcini, A. Haddada; N. Sleimi; analysis and interpretation of results: W. Saadellaoui, R. Ascrizzi, S. Stambouli-Essassi, draft manuscript preparation: W. Saadellaoui, G. Flamini, S. Stambouli-Essassi, F. Harzallah-Skhiri. All authors reviewed the results and approved the final version of the manuscript.
Availability of Data and Materials: Not applicable.
Ethics Approval: Not applicable.
Conflicts of Interest: The authors declare that they have no conflicts of interest to report regarding the present study.
References
1. Danel A, Puła J. Plants as a treasury of fragrant substances for food industry and perfumery. Annales Universitatis Paedagogicae Cracoviensis Studia Naturae. 2019;4(1):149–60. [Google Scholar]
2. Jabeen N. Agricultural, economic and societal importance of Brassicaceae plants. In: Mirza H, editor. The plant family Brassicaceae. Singapore: Springer; 2020. pp. 45–128. [Google Scholar]
3. Al-Shehbaz IA. A generic and tribal synopsis of the Brassicaceae (Cruciferae). Taxon. 2012;61(5):931–54. [Google Scholar]
4. Pottier-Alapetite G. Flore de la Tunisie (Tome 1Angiospermes-Dicotylédones Apétales-Dialypétales. Tunis: Ministère de l’Enseignement Supérieur et de la Recherche Scientifique et le Ministère de l’Agriculture; 1979. [Google Scholar]
5. Le Floc’h E, Boulos L, Véla E. Flore de Tunisie, Catalogue Synonymique Commenté. Tunis : Banque Nationale de Gènes; 2010. [Google Scholar]
6. Mummenhoff K, Brüggemann H, Bowman JL. Chloroplast DNA phylogeny and biogeography of Lepidium (Brassicaceae). Am J Bot. 2001;88:2051–63. [Google Scholar] [PubMed]
7. Jacobs J. Ecology and Management of Hoary Cress (Cardaria draba (L.) Desv.). Bozeman: United States Department of Agriculture; 2007. [Google Scholar]
8. Mangold J. Biology, Ecology and Management of Whitetop (Lepidium spp.). USA: Montana State University; 2018. [Google Scholar]
9. Mahomoodally MF, Zengin G, Aumeeruddy MZ, Sezgin M, Aktumsek A. Phytochemical profile and antioxidant properties of two Brassicaceae species: Cardaria draba subsp. draba and Descurainia sophia. Biocatal Agric Biotechnol. 2018;16:453–8. [Google Scholar]
10. Afsharypuor S, Jamali M. Volatile constituents of the flowering aerial parts, fruits and roots of Cardaria draba L. J Essent Oil Res. 2006;18(6):674–5. [Google Scholar]
11. Sasoli MA, Abro R, Marghazani IB, Mughal GA, Reki MY, Khaskheli AK, et al. Identification and nutrients composition of different rangeland species (grasses, herbs, shrubs, and trees) grazed by small and large ruminants in Balochistan: kharan region. Pure Appl Biol. 2022;11(3):823–34. [Google Scholar]
12. Hosseini NS, Sobhanardakani S, Cheraghi M, Lorestani B, Merrikhpour H. Expansive herbaceous species as bio-tools for elements detectionin the vicinity of major roads of Hamedan, Iran. Int J Environ Sci Technol. 2022;19:1611–24. [Google Scholar]
13. Sobhan-Ardakani S, Hosseini NS. Evaluating the ability of Cardaria draba L. Desv and Achillea wilhelmsii C. Koch as biomonitors and bioremediators of potentially toxic elements in the roadside environment. J Nat Environ. 2023;13:21–38. [Google Scholar]
14. Kaya Y, Aksakal O, Sunar S, Erturk FA, Bozari S, Agar G, et al. Phytotoxical effect of Lepidium draba L. extracts on the germination and growth of monocot (Zea mays L.) and dicot (Amaranthus retroflexus L.) seeds. Toxicol Ind Health. 2015;31(3):247–54. [Google Scholar] [PubMed]
15. Ali Q, Khalil R, Nadeem M, Azhar MM, Hafeez MM, Malik A. Antibacterial, antioxidant activities and association among plant growth related traits of Lepidium draba. Biol Clin Sci Res J. 2020;1:e11. [Google Scholar]
16. Roshanak S, Alizadeh-Behbahani B, Shahidi F, Tabatabaei-Yazdi F, Vasiee AR, Hashemi A. Evaluation of antioxidant potential and antimicrobial activity of Mocheh (Lepidium draba) extract in vitro. Iranian Food Sci Technol. 2021;17(3):17–24. [Google Scholar]
17. Necip A, Durgun M. Antioxidant properties, total phenolic content and LC-MS/MS analysis of Mentha pulegium, Lepidium draba and Centaurea solstitialis. J Inst Sci Technol. 2022;12(4):2375–85. [Google Scholar]
18. Eruygur N, Ayaz F, Bagci Y, Guler E, Cagil EM. Phenolic composition, in-vitro antioxidant and enzyme inhibition activities of Cardaria draba different parts. Eur J Sci Technol. 2022;35:424–31. [Google Scholar]
19. Feng J, Gao H, Yang L, Xie Y, El-Kenawy AE, El-Kott AF. Renoprotective and hepatoprotective activity of Lepidium draba L. extracts on oxymetholone-induced oxidative stress in rat. J Food Biochem. 2022;46(9):e14250. [Google Scholar] [PubMed]
20. Shehab NW, Qahtan Mostafa M, Saeed AY, Jamal L, Ibrahim BA. The effect of aqueous extract and tannins from the Lepidium draba plant on the bacteria contaminating restaurants in the city of Sharqat. In: Proceedings of the First Samarra International Conference for Pure and Applied Sciences. 2022; Sammara, Iraq. p. 2394. [Google Scholar]
21. Fathi H, Ebrahimzadeh MA, Ahanjan M, Shokri F, Jafari H, Eslami S, et al. Antioxidant capacity, chromatographic analysis of phenolic compounds and anti-microbial effects of Cardaria draba growing in Iran. Int J Pharm Sci Res. 2023;14(1):373–80. [Google Scholar]
22. Bayan Y. Chemical composition and antifungal activity of the plant extracts of the Turkey Cardaria draba (L.) Desv. Egypt J Biol Pest Control. 2016;26(3):597–81. [Google Scholar]
23. Radonić A, Blažević I, Mastelić J, Zekić M, Skočibušić M, Moravić A. Phytochemical analysis and antimicrobial activity of Cardaria draba (L.) Desv. volatiles. Chem Biodivers. 2011;8(6):1170–81. [Google Scholar] [PubMed]
24. Nadaf M, Halimi M, Nasrabadi M. GC-MS analysis of n-hexane extract from aerial parts of Cardaria draba and phytochemistry studies. In: Proceedings of the Second National Conference on applied Research of Chemistry, Biology and Geology. 2015; Tehran, Iran. p. 1–6. [Google Scholar]
25. Younes K, Merghache S, Djabou N, Selles C, Muselli A, Tabti B, et al. Chemical composition and free radical scavenging activity of essential oils and extracts of algerian Cardaria draba (L.) Desv. J Essent Oil-Bear Plants. 2015;18(6):1448–58. [Google Scholar]
26. Souissi T, Berner DK, Dubin HJ. First report of a leaf spot caused by Cercospora bizzozeriana on Lepidium draba subsp. draba in Tunisia. Plant Dis. 2005;89(2):206. [Google Scholar] [PubMed]
27. Esmaeel ZA, Al-Musawi AH. Comparative anatomical features of Cardaria draba (L.) Desv. and Sisymbrium irio L., Brassicaceae. J Educ Sci Stud. 2014;3:8–29. [Google Scholar]
28. Mousavi SM, Sharifi-Rad J. Anatomical, palynological and micromorphological study of seed, trichome and stomata of Cardaria draba L. Desv (Brassicaceae) in Sistan, Iran. Int J Biosci. 2014;5(11):63–9. [Google Scholar]
29. Al-Saadi SAAM, Qader KO, Fetah HU. Anatomical characters used to delimit species of some genera of Brassicaceae in Iraq. J Zankoy Sulaimani. 2019;21(1):37–46. [Google Scholar]
30. Arbia F, Ayari-Gribaa O, Souilem F, Chiboub W, Zardi-Bergaoui A, Ben Jannet H, et al. Profiles of the essential oils and headspace analysis of volatiles from Mandragora autumnalis growing wild in Tunisia. Chem Biodivers. 2019;16:e1900345. [Google Scholar] [PubMed]
31. Ditcher DL. Approaches to the identification of angiosperm leaf remains. Bot Rev. 1974;4(1):1–157. [Google Scholar]
32. Bell L, Kitsopanou E, Oloyede OO, Lignou S. Important odorants of four Brassicaceae species, and discrepancies between glucosinolate profiles and observed hydrolysis products. Foods. 2021;10(1055):1–28. [Google Scholar]
33. Bioenergies G. Release of the first batch of cosmetic-grade renewable isododecane. https://bioenergyinternational.com/global-bioenergies-releases-first-commercial-batch-of-cosmetic-grade-renewable-isododecane/. [Accessed 2021]. [Google Scholar]
34. Insausti K, Murillo-Arbizu MT, Urrutia O, Mendizabal JA, Beriain MJ, Colle MJ, et al. Volatile compounds, odour and flavour attributes of lamb meat from the Navarra Breed as affected by ageing. Foods. 2021;10(493):1–14. [Google Scholar]
35. Li Z, Wang J. Identification and similarity analysis of aroma substances in main types of Fenghuang Dancong tea. PLoS One. 2020;15(12):e0244224. [Google Scholar] [PubMed]
36. Erasto P, Viljoen AM. Limonene—A review: biosynthetic, ecological and pharmacological relevance. Nat Prod Commun. 2008;3(7):1193–201. [Google Scholar]
37. Liu Y, Zhang H, Umashankar S, Liang X, Lee HW, Swarup S, et al. Characterization of plant volatiles reveals distinct metabolic profiles and pathways among 12 Brassicaceae vegetables. Metabolites. 2018;8(4):94. [Google Scholar] [PubMed]
38. Kumar J, Paul B, Nebapure SM, Singh S. Comparative GC-MS analysis of two Brassica rapa L. varieties for identification of volatile compounds. Chem Sci Rev Lett. 2017;6(22):884–9. [Google Scholar]
39. Kobayashi K, Arai M, Tanaka A, Matsuyama S, Honda H, Ohsawa R. Variation in floral scent compounds recognized by honeybees in Brassicaceae crop species. Breed Sci. 2012;62:293–302. [Google Scholar] [PubMed]
40. Baldermann S, Yamamoto M, Yang Z, Kawahashi T, Kuwano K, Watanabe N. C13-apocarotenoids: more than flavor compounds?. In: Winterhalter P, et al., editors. Carotenoid cleavage products. Washington DC: ACS Publications; 2013. p. 73–80. [Google Scholar]
41. Paparella A, Shaltiel-Harpaza L, Ibdah M. β-Ionone: its occurrence and biological function and metabolic engineering. Plants. 2021;10(4):754. [Google Scholar] [PubMed]
42. Zhou Q, Tang H, Jia X, Zheng C, Huang F, Zhang M. Distribution of glucosinolate and pungent odors in rapeseed oils from raw and microwaved seeds. Int J Food Prop. 2018;21(1):2296–308. [Google Scholar]
43. McGorrin RJ. The significance of volatile sulfur compounds in food flavors. An overview. In: Qian M, et al., editors. Volatile sulfur compounds in food. Washington: American Chemical Society; 2011. p. 1–31. [Google Scholar]
44. Smart LE, Blight MM. Field discrimination of oilseed rape, Brassica napus volatiles by cabbage seed weevil, Ceutorhynchu Sassimilis. J Chem Ecol. 1997;23:2555–67. [Google Scholar]
45. Oulad El Majdoub Y, Alibrando F, Cacciola F, Arena K, Pagnotta E, Matteo R, et al. Chemical characterization of three accessions of Brassica juncea L. extracts from different plant tissues. Mol. 2020;25(5421):1–25. [Google Scholar]
46. Lahondèrea C, Vinaugera C, Okuboa RP, Wolffa GH, Chan JK, Akbari OS, et al. The olfactory basis of orchid pollination by mosquitoes. PNAS. 2019;117(1):708–16. [Google Scholar]
47. El-Sayed AM. The pherobase: database of pheromones and semiochemicals. Available from: http://www.pherobase.com. [Accessed 2018]. [Google Scholar]
48. Wolken WA, Ten Have R, van der Werf MJ. Aminoacid-catalyzed conversion of citral: cis-trans isomerization and its conversion into 6-methyl-5-hepten-2-one and acetaldehyde. J Agr Food Chem. 2000;48(11):5401–5. [Google Scholar]
49. Burdock GA. Fenaroli’s Handbook of Flavor Ingredients. Boca Raton: CRC Press; 2010. [Google Scholar]
50. Tao N, Jia L, Zhou H. Anti-fungal activity of Citrus reticulata Blanco essential oil against Penicillium italicum and Penicillium digitatum. Food Chem. 2014;153:265–71. [Google Scholar] [PubMed]
51. Saveer AM, Hatano E, Wada-Katsumata A, Meagher RL, Schal C. Nonanal, a new fall armyworm sex pheromone component, significantly increases the efficacy of pheromone lures. Pest Manage Sci. 2023;79:2831–9. [Google Scholar]
52. Fahlbusch KG, Franz-Josef H, Panten J, Pickenhagen W, Schatkowski D, Bauer K, et al. Flavors and fragrances in Ullmann’s encyclopedia of industrial chemistry. Wiley-VCH; 2003. p. 74–198. [Google Scholar]
53. Eddin LB, Jha NK, Goyal SN, Agrawal YO, Subramanya SB, Bastaki SMA, et al. Health benefits, pharmacological effects, molecular mechanisms, and therapeutic potential of α-Bisabolol. Nutr. 2022;14(7):1370. [Google Scholar]
54. Kamatou GPP, Viljoen AM. A review of the application and pharmacological properties of α-bisabolol and α-bisabolol-richoils. J Am Oil Chem’ Soc. 2010;87:1–7. [Google Scholar]
55. Wróblewska-Kurdyk A, Dancewicz K, Gliszczyńska A, Gabry B. Antifeedant potential of geranylacetone and nerylacetone and their epoxy-derivatives against myzuspersicae (Sulz.). Mol. 2022;27(24):8871. [Google Scholar]
56. Ye F, Qiao X, Gui A, Liu P, Wang S, Wang X, et al. Characterization of roasting time on sensory quality, color, taste, and nonvolatile compounds of yuan a yellow tea. Mol. 2022;27(13):4119. [Google Scholar]
57. Acton WJF, Jud W, Ghirardo A, Wohlfahrt G, Hewitt CN, Taylor JE, et al. The effect of ozone fumigation on the biogenic volatile organic compounds (BVOCs) emitted from Brassica napus above-and below-ground. PLos One. 2018;13(12):e0208825. [Google Scholar] [PubMed]
58. Stambouli-Essassi S, Mejri F, Dhoueibi M, Mrabet Y, Harzallah-Skhiri F, Hosni K. Anatomical features, fatty acid profile and tocopherol content of the Tunisian Cakile maritima subsp. maritima Scop. fruit. J Anim Plant Sci. 2020;43(1):7366–79. [Google Scholar]
59. Wu X, Chen Y, Shi X, Qi K, Cao P, Liu X, et al. Effects of palmiticacid (16:0hexacosanoicacid (26:0ethephon and methyljasmonate on the cuticular wax composition, structure and expression of key gene in the fruits of threepear cultivars. Funct Plant Biol. 2020;47(2):156–69. [Google Scholar] [PubMed]
60. Lewis KA, Tzilivakis J, Warner D, Green A. An international database for pesticide risk assessments and management. Human Ecol Risk Assess: An Int J. 2016;22(4):1050–64. [Google Scholar]
61. Watson SB, Jüttner F. Biological production of taste and odor compounds. In: Lin TF et al., editors. Taste and odour in source and drinking water: causes, controls, and consequences. London: International Water Association Publications; 2019. p. 63–112. [Google Scholar]
62. Avoseh ON, Mtunzi FM, Ogunwande IA, Ascrizzi R, Guido F. Albizia lebbeck and Albizia zygia volatile oils exhibit anti-nociceptive and anti-inflammatory properties in pain models. J Ethnopharmacol. 2021;25(268):113676. [Google Scholar]
63. Gad HA, Mukhammadiev EA, Zengen G, Musayeib NMA, Hussain H, Bin Ware I, et al. Chemometric analysis based on GC-MS chemical profiles of three Stachys species from Uzbekistan and their biological activity. Plants. 2022;11:1215. [Google Scholar] [PubMed]
64. Şanli A, Karadoğan T. Geographical impact on essential oil composition of endemic Kundmannia anatolica hub.mor. (Apiaceae). Afr J Tradit, Complement Altern Med. 2016;14(1):131–7. [Google Scholar] [PubMed]
65. Kostova P, Karcheva-Bahchevanska D. Chemical composition of essential oil obtained from a wild population of Bulgarian Cardaria draba (L.). Int J Dent Med Sci Res. 2023;5(4):26–31. [Google Scholar]
66. Kazemi M, Bigdeli-Azari Z, Dadkhah A, Teimuri F. Biological activities of Cardaria draba L. (desv.) essential oil. Proceedings of the Iran’s Fifth National Congress on Medicinal Plants. Kish Island, Iran; 2012; p. 77. [Google Scholar]
67. Doaigey A, El-Habashy I, Al-Watban A, Milagy AE, Al Sahli A, Siddiqui M, et al. Epidermal characteristics of 34 species of Brassicaceae growing in desert of Saudi Arabia. Wulfenia J. 2013;20(9):202–21. [Google Scholar]
68. Bibi S, Ullah Z, Ali A, Alam N, Khan RA, Ali N. Systematic significance of leaf epidermal features in Brassicaceae of Swat, Pakistan. Kashmir J Sci. 2022;1(1):31–42. [Google Scholar]
69. Ahmad K, Khan MA, Ahmad S, Nazir NA. Taxonomic diversity in epidermal cells of some sub-tropical plant species. Int J Agric Biol. 2010;12:115–8. [Google Scholar]
70. Culter D, Botha T, Stevenson D. Plant anatomy and applied approach. USA: Blackwell Publishing; 2007. [Google Scholar]
71. Pillitteri LJ, Dong J. Stomatal development in Arabidopsis. In: Arabidopsis book. 2013. p. 11. [Google Scholar]
72. Sun ZY, Li FZ. Studies on the leaf epidermal features of Lepidium (Brassicaceae) from China. J Chinese Med Mat. 2007;30(7):780–5. [Google Scholar]
73. van Cotthem WRF. A classification of stomatal types. Bot J Linn Soc. 1970;63(3):235–46. [Google Scholar]
74. Schuurink R, Tissier A. Glandular trichomes: micro-organs with model status. New Phytol. 2020;225:2251–66. [Google Scholar] [PubMed]
75. Ilyinska A. Structural diversity of trichomes and pubescence of Brassicaceae Burnett of the flora of Ukraine with an emphasis on ecology. Agrobiodivers Improv Nutr, Health Life Qual. 2021;5(1):133–59. [Google Scholar]
76. Akbar F, Begum KN. A comparative anatomical investigation of three taxa of Brassica from Bangladesh. Bangl J Plant Taxon. 2020;27(1):15–26. [Google Scholar]
77. Zhang L, Niu H, Wang S, Zhu X, Luo C, Li Y, et al. Gene or environment? Species-specific control of stomatal density and length. Ecol Evol. 2012;2(5):1065–70. [Google Scholar] [PubMed]
78. Segev R, Nannapaneni R, Sindurakar P, Kim H, Read H, Lijek S. The effect of the stomatal index on the net rate of photosynthesis in the leaves of Spinacia oleracea, Vinca minor, Rhododendron spp, Epipremnum aureum, and Hedera spp. J Emerg Investig. 2015;14:1–5. [Google Scholar]
79. Huma ZE, Hameed I, Sher U, Chen JM, Wang QF. Comparison of the leaf stomatal characteristics between Brassica campestris and B. napus (Brassicaceae: Brassica). Sch J Agric Sci. 2018;8(1):1–6. [Google Scholar]
Cite This Article
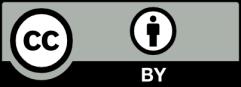