Open Access
ARTICLE
Circulating tumor cells: Biological features and survival mechanisms
1 Department of Oncology, Affiliated Quanzhou First Hospital of Fujian Medical University, Quanzhou, China
2 Department of Pathology, Affiliated Quanzhou First Hospital of Fujian Medical University, Quanzhou, China
3 Department of Medical Oncology, Affiliated Quanzhou First Hospital of Fujian Medical University, Quanzhou, China
4 Department of Dermatology, School of Medicine, Tongji Hospital, Tongji University, Shanghai, China
5 Department of Central Laboratory, Shanghai Tenth People’s Hospital, Shanghai, China
6 Shanghai East Hospital, The Institute for Biomedical Engineering & Nano Science, Tongji University School of Medicine, Shanghai, China
* Corresponding Author: Bingdi Chen,
(This article belongs to the Special Issue: Expert Opinions and Future Trends on Stem Cells, Biomaterials and Growth Factors)
BIOCELL 2023, 47(8), 1771-1781. https://doi.org/10.32604/biocell.2023.028343
Received 13 December 2022; Accepted 06 February 2023; Issue published 28 August 2023
Abstract
Circulating tumor cells (CTCs) are neoplastic cells that are detached from primary tumors and enter circulation. Enumeration and characterization of CTCs are of significance in cancer diagnosis, prognosis, and treatment monitoring. CTC survival in the bloodstream is a limiting step for the development of metastases in distant organs. Recent technological advances, especially in single-cell molecular analyses have uncovered heterogeneous CTC survival mechanisms. Undergoing epithelial-to-mesenchymal transition (EMT), increasing stem cell-like properties, and forming cell clusters enable CTCs to adapt to the harsh microenvironment of the circulation. Expressing and releasing several immunosuppressive molecules help CTCs escape from anti-cancer immune mechanisms. This review article summarizes the biological characteristics of CTCs and focuses on the recent understanding of the mechanisms by which CTCs survive in circulation. Additionally, the clinical and therapeutic implications of CTCs are discussed.Keywords
Abbreviations
CAF | Cancer-associated fibroblast |
CDX | CTC-derived xenograft |
CTC | Circulating tumor cells |
EMT | Epithelial-to-mesenchymal transition |
EpCAM | Epithelial cellular adhesion molecule |
MDSC | Myeloid derived suppressor cell |
Circulating tumor cells (CTCs) are a group of extraordinarily rare cancer cells that shed from solid tumors and enter the bloodstream. It is estimated that cancer patients have only 1 CTC per 1–10 million white blood cells (WBCs) (Dive and Brady, 2017). Compared to WBCs, CTCs possess unique biophysical and immunophenotypic characteristics. CTCs tend to be larger and express epithelial cell markers such as the epithelial cellular adhesion molecule (EpCAM) and cytokeratins (Micalizzi et al., 2017). These features enable the enrichment of CTCs from a vast number of blood cells. Recently, a variety of enrichment approaches, although technically challenging, have been developed to detect CTCs (Deng et al., 2022). Enumeration and phenotyping of CTCs are valuable for cancer diagnosis and prognosis. For example, a large, multicenter, prospective trial demonstrated that the presence of ≥3 CTCs at baseline predicted shorter survival in patients with metastatic renal cell carcinoma (Basso et al., 2021). CTC enumeration could be used to distinguish patients with colorectal cancer from non-cancerous populations, with a sensitivity and specificity of 85.3% and 78.5%, respectively (Tsai et al., 2021). Hence, CTC detection and characterization provide important biomarkers for screening and monitoring malignant diseases.
CTCs are considered to be an intermediate stage of metastasis, which involves a complex multistep process including cell detachment from the primary tumor, entry into vessels, extravasation, and colonization to distant tissues (Jerabkova-Roda et al., 2022; Stock, 2022). Once shed into the circulation, CTCs face a harsh niche that is completely different from the tumor microenvironment established at the primary site. Most cancer cells die in the vascular system where there are significant shear forces, robust innate immunity, and oxidative stress (Massagué and Obenauf, 2016). Only a few CTCs can adapt to the hostile conditions in the circulation. Several such mechanisms of CTC adaptation have been identified (Schuster et al., 2021; Pereira-Veiga et al., 2022). CTC aggregates have been frequently detected in the blood of patients with epithelial tumors (Cho et al., 2012). The formation of such CTC aggregates offered a survival advantage and increased cancer stemness, leading to a 20- to 100-fold greater metastatic potential than single CTCs (Schuster et al., 2021). CTCs from patients with melanoma exhibited the activation of lipogenic and iron homeostatic pathways, which conferred resistance to oxidative stress (Hong et al., 2021). In another finding, the supplementation of N-acetyl-L-cysteine (NAC) and other antioxidants could increase the ex vivo survival and expansion of CTCs (Teng et al., 2021). These studies suggest that the antioxidant defense system is important for the maintenance of CTC viability in blood circulation. CTCs typically undergo epithelial-to-mesenchymal transition (EMT), a reversible cellular process involving loss of cell-cell adhesion, epithelial polarization, and acquisition of mesenchymal cell features (Sun et al., 2018; Xin et al., 2020). The phenotypic plasticity allows CTCs to withstand the mechanical stress of the bloodstream (Xin et al., 2020).
In the past two decades, single-cell profiling approaches have gained increasing attention in cancer studies (Ho et al., 2019; Wu et al., 2021). These techniques enable the investigation of intra-tumoral heterogeneity at single-cell resolution. A variety of single-cell multi-omics studies have indicated that CTCs are highly heterogeneous (Sun et al., 2021b; Barnett et al., 2022; Khan et al., 2022). For example, Suvilesh et al. (2022) established liquid biopsy CTC-derived xenograft (CDX) tumor models in immunodeficient mice and found different drug responses and cell heterogeneity in CDX tumors. Using a single-cell immunoblotting chip technology, Abdulla et al. (2022) identified a novel CTC subpopulation that lacks the expression of Bax, a proapoptotic member of the Bcl-2 protein family that also displays chemoresistance. In another study, Chen et al. (2022) reported that the expression levels of epithelial and stem cell-like markers vary in two distinct groups of CTCs.
In this review article, we summarize recent advances in understanding the biological characteristics of CTCs. Given the importance of CTC survival in cancer dissemination, we focus on the mechanisms by which CTCs survive in blood circulation. Additionally, we discuss the clinical and therapeutic implications of CTCs.
In the ongoing single-cell analysis era, the vast heterogeneity of CTCs is being uncovered. CTCs are known to comprise a heterogeneous population of malignant cells. Cho et al. (2021) reported that CTCs from the same patient with metastatic gastric cancer are heterogeneous in terms of morphology and marker expression, indicating an intrapatient heterogeneity. Chen et al. (2021b) noted that CTCs isolated from gastric cancer patients vary greatly in size. Single cell-based DNA sequencing analysis revealed that small and large CTCs have distinct genomic mutations. While the former have an enrichment in the Kirsten rat sarcoma viral oncogene homolog (KRAS) and Rap1 pathways, the latter is enriched in the phosphoinositide-3-kinase–protein kinase B/Akt or MET/PI3K/AKT pathway. Tumor heterogeneity has been partly ascribed to genetic instability and somatic mutations (Knoche et al., 2021). Genomic profiling analysis has shown that CTCs share similar mutational signatures with the matched primary tumor samples (Alves et al., 2022). Su et al. (2019) performed genomic analyses of tumor and CTC samples obtained from small-cell lung cancer patients and found that the majority of mutations in primary tumors are retained in CTCs. Kondo et al. (2017) analyzed the KRAS genotype in CTCs from 11 patients with metastatic colorectal cancer and found that 10 of 11 patients had the same KRAS status between the primary tumors and CTCs. Paoletti et al. (2018) performed next-generation sequencing (NGS) of CTCs and paired tissue metastases from patients with breast cancer and observed 85% concordance in somatic mutation and copy-number profiles. They also found a small set of CTC subclones with unique somatic mutations, confirming the heterogeneity of CTCs. However, Kim et al. (2020) reported conflicting findings on bladder cancer samples. They performed whole exome sequencing of 20 matched pairs of primary bladder cancers and CTCs and demonstrated that their mutation concordance is only 3%–24%. Despite such a report, somatic mutation represents an important cause of CTC heterogeneity.
The heterogeneity of CTCs can be coordinated by epigenetic and microenvironmental factors. Genome-wide analyses of DNA methylation in CTCs from breast cancer patients indicate the heterogeneity of CTCs at the epigenetic level (Cho et al., 2012; Pixberg et al., 2017). Epigenetic modulation of genes involved in proliferation, stemness, and EMT likely contributes to the survival of CTCs in circulation. In addition to genetic and epigenetic heterogeneity, CTCs show spatial heterogeneity. Sun et al. (2021a) observed that CTCs from different vascular sites of hepatocellular carcinoma patients displayed distinct transcriptional profiles, which was revealed by single-cell RNA sequencing. The spatial heterogeneity of CTCs has also been detected in lung cancer patients with brain metastasis (Ruan et al., 2020). As CTCs are usually arrested in capillary beds (Perea Paizal et al., 2021), blood flow generates different levels of mechanical forces at these CTC-arrested sites, thereby contributing to spatial heterogeneity (Follain et al., 2018). Additionally, CTC diversity is increased upon anti-cancer treatments. Stewart et al. (2020) reported that there is a significant increase in the intratumoral heterogeneity score of CTCs isolated from relapsed small-cell lung cancer patients after chemotherapy. The complex heterogeneity of CTCs makes it necessary to dissect the characteristics of CTC subpopulations at the single-cell level.
Epithelial-mesenchymal plasticity is an important feature of CTCs (Burr et al., 2020). During intravasation, circulation, and extravasation, CTCs typically undergo epithelial-to-mesenchymal or mesenchymal-to-epithelial transitions. These morphological changes involve the dysregulation of many EMT-related genes (Qi et al., 2018; Burr et al., 2020). Yu et al. (2013) reported that CTCs from breast cancer patients express transforming growth factor (TGF)-β pathway components and the Forkhead box C1 (FOXC1) transcription factor, which are key regulators of EMT. Similarly, EMT markers were also enriched in CTCs isolated from non-small cell lung cancer patients (Ntzifa et al., 2021). Transcriptomic analysis has demonstrated that surviving CTCs express both epithelial and mesenchymal markers, indicating a hybrid epithelial/mesenchymal state (Genna et al., 2020). Using single-cell sequencing technology, Sun et al. (2018) uncovered dynamic changes in CTCs isolated from hepatocellular carcinoma patients. The epithelial phenotype of CTCs switched to the mixed epithelial/mesenchymal phenotype along disease progression, which was causally linked to the activation of Smad2 and β-catenin-related signaling pathways (Sun et al., 2018). Blood flow forces have also been reported to promote EMT in CTCs via c-Jun N-terminal kinase (JNK) signaling (Xin et al., 2020).
In addition to morphological plasticity, CTCs exhibit metabolic plasticity (Chen et al., 2018a; Zafeiriadou et al., 2022). Compelling evidence indicates that metabolic reprogramming augments the adaptation of cancer cells to microenvironmental stress (Martínez-Reyes and Chandel, 2021; Tang et al., 2022). Increased expression of phosphoglycerate kinase (PGK1) and glucose-6-phosphate dehydrogenase (G6PD), the key regulators of glycolysis and the pentose phosphate pathway, respectively, suggests active glucose metabolism of tumor cells (Chen et al., 2020). CTCs from prostate cancer patients (Chen et al., 2018b) and breast cancer (Chen et al., 2020) patients also abundantly expressed PGK1 and G6PD. The CTCs with high expression of PGK1 and G6PD represent an aggressive subpopulation and serve as a potential marker for poor prognosis (Chen et al., 2018b; Chen et al., 2020). CTCs show increased expression of atonal bHLH transcription factor 8 (ATOH8) upon shear stress, which promotes hexokinase 2 (HK2)-mediated glycolysis and survival (Huang et al., 2020). These studies suggest that metabolic plasticity contributes to an aggressive phenotype of CTCs.
Stem-like cancer cell populations have been identified in all solid tumors including brain, liver, lung, breast, stomach, ovary, colon, thyroid, and prostate cancers (Du et al., 2022). They possess self-renewal and tumor-initiating capacities. A large number of studies have reported the link between stem cell signatures and the survival of cancer patients (Ponomarev et al., 2022), highlighting the involvement of cancer stem cells in tumor progression.
In recent years, the stem-like properties of CTCs have attracted increasing attention (Chen et al., 2021a; Lee et al., 2022). CTCs can express a variety of stem cell markers. Wang et al. (2022) reported that the stem cell marker CD44 was detected in CTCs isolated from gastric cancer patients. The presence of CD44-positive CTCs was associated with recurrent disease and poor survival of patients. CTCs from melanoma patients had a high aldehyde dehydrogenase 1 activity and the expression of multiple stemness transcription factors such as OCT3/4, NANOG, and SOX2 (Wang et al., 2022). When injected into immunodeficient mice, melanoma CTCs showed tumorigenic and metastatic potentials. CTCs from patients with glioblastoma showed a robust expression of stemness-associated genes including SOX2, OCT4, and NANOG (Felici et al., 2022). Cancer stemness is suggested to also drive therapeutic resistance (Najafi et al., 2019). Consistently, circulating glioma cells exhibited resistance to radiotherapy and chemotherapy (Liu et al., 2018). Zhang et al. (2020) demonstrated that CTCs from bladder cancer patients coexpressed both stem cell and EMT markers, suggesting the relationship between EMT and stemness. Leucine-rich G-protein-coupled receptor 5 (LGR5) is an important driver of cancer stemness (Zhang et al., 2017). A study showed that the high LGR5 expression in CTCs was associated with colorectal cancer metastasis (Wang et al., 2018). These observations show that stem-like CTCs may contribute to metastatic lesion formation.
Survival Mechanisms of CTCs in the Circulation
When cancer cells are shed from primary tumors enter the circulation, they have to overcome multiple challenges that impair cell survival (Fig. 1). Most CTCs lack the ability to survive in circulation, with an estimated half-life of 1–2.4 h (Meng et al., 2004). However, a small minority of CTCs can survive and then seed metastases. Scrutiny of their survival strategies is of significance in eliminating persistent CTCs in therapy.
Figure 1: The mechanisms linked to circulating tumor cell (CTC) survival. To survive in circulation, CTCs can form homoclusters or heteroclusters with neutrophils, macrophages, cancer-associated fibroblasts, and platelets. Undergoing metabolic reprogramming and epithelial-to-mesenchymal transition (EMT) and acquiring stem-like properties can all enhance CTC survival. In addition, CTCs can express multiple immune checkpoint molecules and release cytokines and chemokines to recruit regulatory T cells and myeloid-derived suppressor cells (MDSCs), which help CTCs escape from the immune attack.
Clustering enhances CTC survival
The clustering of CTCs has been observed in many cancer types (Schuster et al., 2021). For example, Chen et al. (2022) identified CTC clusters in 6 of the 8 hepatocellular carcinoma patients tested. Francescangeli et al. (2021) isolated large CTC clusters from metastatic colorectal cancer patients. There is evidence that such CTC clustering enhances the metastatic potential of CTCs (Piñeiro et al., 2020). Aceto et al. (2014) reported that CTC clusters have 23-50-fold higher metastatic activity than single CTCs. Aggregation of CTCs was found to induce anoikis resistance in the suspension culture with fluid shear stress (Maeshiro et al., 2021).
In addition to the formation of homo-clusters, CTCs can interact with immune cells such as neutrophils and macrophages to form hetero-clusters (Chen et al., 2018b; Osmulski et al., 2021). Osmulski et al. (2021) reported that the inclusion of macrophages in CTC clusters facilitated survival-promoting mechanical fitness through the induction of epithelial-mesenchymal plasticity. CTCs could also recruit and form heterotypic clusters with inflamed neutrophils, which then enhanced CTC extravasation (Chen et al., 2018b). Myeloid-derived suppressor cells (MDSCs) clustered with CTCs have been shown to promote tumor cell proliferation (Sprouse et al., 2019). Additionally, CTCs could interact with endothelial cells and cancer-associated fibroblasts (CAFs) (Sharma et al., 2021). Such cellular aggregation is believed to play an essential role in the maintenance of CTC survival in blood (Pereira-Veiga et al., 2022). Arnoletti et al. (2018) demonstrated that CTCs from patients with pancreatic ductal adenocarcinoma (PDAC), ampullary adenocarcinoma (AA), and distal cholangiocarcinoma (CC) could recruit multiple immune cell types to generate spheroid-like clusters, consequently promoting CTC survival and growth. Consistent with their biological roles, CTC clusters have important clinical values in cancer management. The detection of CTC clusters was of diagnostic and prognostic significance in patients with metastatic cancer (Manjunath et al., 2019; Costa et al., 2020; Lim et al., 2021; Wang et al., 2021a). In one study, CTC clusters could be used as a specific liquid biomarker to distinguish non-small cell lung cancer from high-risk subjects with benign nodules (Manjunath et al., 2019). In a real-world cohort of metastatic breast cancer patients, the presence of CTC clusters at baseline was significantly associated with reduced survival (Costa et al., 2020).
Most CTC clusters (about 80%) are associated with platelets (Lim et al., 2021). Heat shock protein 47 (Hsp47) was found to be involved in platelet recruitment and mediated CTC-platelet interaction (Xiong et al., 2020). Transcriptomic analysis of CTC samples isolated from breast cancer patients revealed an increased expression of Hsp47 in CTC clusters compared to single CTCs (Chen et al., 2020), suggesting the role of Hsp47 in CTC clustering. Cytoskeletal elements including α-tubulin, detyrosinated α-tubulin, and vimentin contribute to the interaction between CTCs and blood cells (Kallergi et al., 2018). Intercellular adhesion molecule 1 (ICAM1) was shown to be involved in homotypic breast cancer cell clustering, and its depletion impaired CTC cluster formation (Taftaf et al., 2021). Upregulation of desmocollin-2 (DSC2) and plakophilin-1 (PKP1) in CTCs led to increased formation of clusters and activation of PI3K/AKT/Bcl-2–mediated pathways, consequently promoting CTC survival in the circulation (Li et al., 2021). In one study, intercellular CD44-CD44 homophilic interactions could facilitate circulating breast cancer cell aggregation through the activation of focal adhesion kinase (FAK) signaling (Liu et al., 2019). Further, knockdown of plakoglobin resulted in the suppression of CTC cluster formation (Aceto et al., 2014). Fibronectin was also shown to participate in cell aggregate formation, and its knockdown blocked cancer cell clustering and reversed anoikis resistance (Han et al., 2021). Collectively, a number of genes contribute to the formation of homotypic or heterotypic CTC clusters, thus promoting CTC survival in circulation. Prevention of CTC clustering may thus represent a promising approach to treating metastatic cancers.
Induction of EMT and stemness contributes to CTC survival
Induction of EMT- and stemness-related genes has been shown to enhance anoikis resistance of cancer cells (Wang et al., 2021b; Shait Mohammed et al., 2022; Wang et al., 2022). Interestingly, CTCs can express multiple EMT and cancer stem cell markers (Chen et al., 2013; Werner et al., 2017). Pixberg et al. (2017) reported that aggregated CTCs have a global change in DNA methylation, leading to increased expression of genes involved in cell survival, proliferation, and stemness. Single-cell analysis of CTCs from patients with metastatic prostate cancer revealed the abundant expression of EMT-related genes including insulin like growth factor 1 (IGF1), insulin like growth factor 2 (IGF2), epidermal growth factor (EGFR), forkhead box P3 (FOXP3), and transforming growth factor beta 3 (TGFB3) (Chen et al., 2013; Werner et al., 2017). Further, CTCs with stem cell-like features documented overexpression of the pluripotency marker Krüppel-like factor 4 (Klf4) and activation of β-catenin signaling (Zhu et al., 2020). Clinically, the presence of CTCs expressing EMT markers (phosphatidylinositol-3-kinase α [PI3Kα] and Akt-2) and stem cell markers (aldehyde dehydrogenase 1 [ALDH1]) was associated with reduced survival in patients with metastatic colorectal cancer (Ning et al., 2018). Papadaki et al. (2019) reported that a subset of CTCs isolated from metastatic breast cancer patients expressed high levels of ALDH1, TWIST1, and cytokeratin and showed chemoresistant properties. These results suggest that the upregulation of EMT- and stemness-related genes confers an aggressive phenotype to CTCs.
Metabolic reprogramming supports CTC survival
Cells surviving in an anchorage-independent condition are characterized by metabolic reprogramming (Shi et al., 2014). For example, Dihydroceramide desaturase 1 acts as a mediator of HER2-driven glucose metabolic signals and enhances anchorage-independent survival (Linzer et al., 2022). Serum and glucocorticoid kinase-1 plays an essential role in ATP generation during the loss of extracellular matrix attachment, which is associated with the elevation of glucose uptake (Mason et al., 2021). Analysis of CTCs from patients with non-small cell lung cancer also reveals overexpression of multiple metabolism-related genes (Zafeiriadou et al., 2022). For example, an increased number of CTCs with aberrant expression of glucose metabolic genes (PGK1 and G6PD) was associated with advanced tumor stage and metastasis in prostate cancer patients (Chen et al., 2018b). These findings suggest the involvement of metabolic reprogramming in the regulation of CTC behaviors. In another study, single melanoma CTCs showed upregulation of lipogenesis and iron homeostasis pathways, which reduced reactive oxygen species and lipid peroxidation and thereby promoted resistance to ferroptosis (Hong et al., 2021). The PI3K/AKT pathway plays a pivotal role in cellular metabolism via either direct regulation of metabolic enzymes or modulation of metabolism-related transcription factors (Hoxhaj and Manning, 2020). In an anchorage-independent condition, AKT activation leads to the enhancement of cell survival through the induction of glycolysis (Joo et al., 2022). Moreover, the activation of the PI3K/AKT pathway promoted CTC survival in a study (Li et al., 2021). Therefore, the PI3K/AKT pathway may mediate the link between metabolism and survival of CTCs.
Escape from immune attack is important for CTC survival in circulation. It has been documented that CTCs can express multiple immune checkpoint proteins including CD47 and programmed death ligand 1 (PD-L1) (Chikamatsu et al., 2019; Papadaki et al., 2020; Zhang et al., 2021). The transmembrane protein, CD47, can interact with the myeloid inhibitory immunoreceptor signal-regulatory protein-α (SIRPα) to inhibit the phagocytic activity of activated immune cells (Barclay and van den Berg, 2014). Steinert et al. (2014) reported that CD47 was upregulated in CTCs from colorectal cancer patients compared to matched tumor tissues and contributed to CTC immune escape. The presence of CD47-positive CTCs has also been suggested to be responsible for tumor relapse in breast cancer patients (Nagahara et al., 2010). The PD-1/PD-L1 axis is another immune checkpoint regulator. The interaction between PD-L1 and PD-1 can halt T cell-mediated immune responses and help tumor cells escape from the surveillance of the immune system (Pantazaka et al., 2022). PD-L1 can also promote the expansion of regulatory T cells, thus enhancing immunosuppression (Dong et al., 2020). The PD-L1/PD-1 interaction is believed to enable CTCs to survive in circulation (Chikamatsu et al., 2019; Papadaki et al., 2020). For example, baseline PD-L1-positive CTCs were detected in 36.1% of 56 patients with metastatic breast cancer that correlated with patient survival (Jacot et al., 2020). In another study, Nicolazzo et al. (2016) monitored the PD-L1 expression status in CTCs from non-small lung cancer patients after treatment with the PD-1 inhibitor Nivolumab. They found that patients with PD-L1-positive CTCs developed progressive disease, suggesting that PD-L1-positive CTCs can escape from anti-cancer immunity. In a cohort of patients with advanced non-small cell lung cancer, survival was worse in the group with PD-L1-positive CTCs than in the group with PD-L1-negative CTCs (Sinoquet et al., 2021). In an animal model study, dual inhibition of both PD-L1 and CD47 led to a significant reduction of metastatic formation by CTCs (Lian et al., 2019).
The release of a variety of cytokines and chemokines by CTCs to counteract immune responses is documented (Sun et al., 2021a). For example, Sun et al. (2021a) reported that the chemokine C-C motif chemokine ligand 5 (CCL5) was abundantly produced by hepatocellular carcinoma CTCs, which consequently recruited regulatory T cells to suppress cytotoxic T cell immunity. Further, PDAC CTCs produced colony-stimulating factor 1 (CSF1), Interleukin (IL) 34, and IL8 that induced MDSC differentiation (Arnoletti et al., 2022). MDSCs are characterized by their T-cell immunosuppressive functions (Hegde et al., 2021). The induction of MDSCs has also been shown to inhibit anti-cancer immunity (Li et al., 2018). Hence, such interactions with MDSCs support CTC survival and immune evasion.
The enumeration and characterization of CTCs provide valuable information for cancer detection, prognostic evaluation, and treatment guidance. An example is the CellSearch system, which is designed for the enumeration of CTCs, has been approved by the Food and Drug Administration (FDA) for prognostic analysis in patients with breast, colorectal, and prostate cancers (Deng et al., 2022). A large number of clinical studies have indicated the prognostic value of CTC enumeration in cancer patients (Basso et al., 2021; Deng et al., 2022). In addition to enumeration, molecular phenotyping of CTCs provides an additional clinical relationship with the disease state and patient survival (Sun et al., 2021a; Abdulla et al., 2022; Khan et al., 2022). For example, PD-L1 expression in CTCs was significantly associated with progression-free survival of patients with triple-negative breast cancer (Jacot et al., 2020). Single-cell technologies have enabled the dynamic monitoring of heterogenous CTCs. The reduction in the percentage of PD-L1-positive CTCs reflects a beneficial response to PD-1/PD-L1 inhibitors in patients with advanced cancers (Tan et al., 2021), suggesting the significance of monitoring CTC dynamic changes. Recently, multiple microfluidic platforms have been developed to integrate CTC enrichment, identification, and molecular analysis, which strengthen the significance of CTCs as clinical biomarkers in cancer detection and treatment (Abdulla et al., 2022; Sajdik et al., 2022).
The enumeration of CTCs also serves as a promising blood test to screen for cancers. For example, the enumeration of EpCAM-positive CTCs yielded a sensitivity of 85.3% and specificity of 78.5% in differentiating colorectal cancers from the non-cancerous controls (Tsai et al., 2021). When combined with serum carcinoembryonic antigen (CEA) assay, CTC testing significantly improved colorectal cancer screening. Total CTC counting provided a potential diagnostic value in hepatocellular carcinoma (Cheng et al., 2019). A meta-analysis proposed CTCs to be used as a biomarker for lung cancer diagnosis given a sensitivity and specificity of 75% and 92%, respectively. The diagnostic potential of CTCs has also been observed in pancreatic cancer, with a sensitivity and specificity of 75.0% and 96.4%, respectively. Moreover, a cut-off of
The detection of CTCs also provides prognostic information in many cancers (Deng et al., 2022). For example, a systematic literature review on CTCs in pancreatic cancer suggested that CTCs are present in the peripheral blood of most patients with pancreatic cancer and predict shorter overall survival (Pang et al., 2021b). In patients with metastatic melanoma, CTC detection was associated with worse overall survival and progression-free survival (Aya-Bonilla et al., 2020). Total CTCs and CTC-white blood cell clusters have been identified as prognostic factors for the metastasis-free survival of renal cell carcinoma patients (Guan et al., 2021). Further, combining CTC cluster counts and cell-free DNA levels exhibited the potential to improve the prediction of progression-free survival in non-small cell lung cancer patients (Kapeleris et al., 2022). Taking heterogeneity into consideration, CTCs can be classified into distinct sub-populations, such as epithelial, mesenchymal, partial EMT, and stem cell-like CTCs. Although there is no significant correlation between the total CTC count and clinicopathological variables, the partial EMT sub-population of CTCs showed significant correlations with advanced disease and poor prognosis in pancreatic cancer patients (Semaan et al., 2021). These studies highlight the prognostic value of CTC biomarkers in cancers.
The counts of CTCs have been shown to change during anticancer treatment (Rossi et al., 2012; Pernot et al., 2017; Pang et al., 2021a). This provides a rationale for monitoring therapeutic response based on CTC analysis. CTC counts at baseline served as an independent predictor of treatment response in patients with head and neck cancer (Zhang et al., 2022b). For example, Chong et al. (2012) reported that CTCs were detected in 53 out of 94 breast cancer patients before chemotherapy. However, after 3 cycles of chemotherapy, CTC numbers were reduced to an undetectable level. Li et al. (2016) quantified CTCs in patients with advanced gastric cancer before and after chemotherapy. They found that elevated CTC numbers during therapy predict a poor prognosis. In contrast, conversion to a favorable CTC level after chemotherapy was associated with a good prognosis. Further, Wang et al. (2017) suggested that the assessment of CTC clusters provides additional prognostic values to CTC enumeration alone in metastatic breast cancer patients.
The molecular phenotypes of CTCs may change perceptibly in response to anticancer treatment. For example, CTCs from patients with ER+/HER2− (ER: estrogen receptor; HER: human epidermal growth factor receptor 2) breast cancer acquired a subpopulation of HER2+ CTCs after several courses of therapy, which is explained by the reciprocal transformation between HER2+ and HER2− CTCs (Jordan et al., 2016). Further, the reduced expression of Schlafen 11 in CTCs was associated with therapeutic response in small cell lung cancer patients receiving platinum treatment (Zhang et al., 2022a). The proportion of PD-L1-positive CTCs was reduced in cancer patients who benefited from PD-1/PD-L1 blockade therapies (Tan et al., 2021), suggesting that the PD-L1 level on CTCs is useful in monitoring response to PD-1/PD-L1 inhibitors. Additionally, Spiliotaki et al. (2022) examined the expression of PD-L1 in longitudinal CTC samples isolated from 47 advanced NSCLC patients receiving pembrolizumab. They found that the patients with decreased total and PD-L1low CTCs after first cycle treatment have significantly longer progression-free survival than those with increased CTCs. These observations necessitate that the dynamic analysis of CTC counts and phenotypes is important to monitor therapeutic response in cancer patients.
CTCs have the potential to seed metastases in distant organs. Their survival in circulation is indispensable for successful metastasis. High heterogeneity, phenotypic plasticity, stem-like properties, and cell-cell cross-talk all contribute to CTC survival in the bloodstream microenvironment. Advances in single-cell technologies have enabled the scrutiny of individual CTCs and shed light on the complex mechanisms by which CTCs escape from anoikis and immune attack. An understanding of the molecular characteristics of CTCs will further promote their clinical implications in cancer diagnosis, prognosis, and therapy monitoring.
Funding Statement: This work was supported by the Science Foundation of Fujian Province of China (2019J01596).
Author Contributions: XFL, JYZ, JFZ, XH, HHZ, and BDC designed the study. XFL and BDC were responsible for manuscript drafting. All authors read and approved the final draft of the manuscript.
Availability of Data and Materials: The datasets generated during and/or analysed during the current study are available from the corresponding author on reasonable request.
Ethics Approval: Not applicable.
Conflicts of Interest: The authors declare that they have no conflicts of interest to report regarding the present study.
References
Abdulla A, Zhang T, Li S, Guo W, Warden AR, Xin Y, Maboyi N, Lou J, Xie H, Ding X (2022). Integrated microfluidic single-cell immunoblotting chip enables high-throughput isolation, enrichment and direct protein analysis of circulating tumor cells. Microsystems & Nanoengineering 8: 13. https://doi.org/10.1038/s41378-021-00342-2 [Google Scholar] [PubMed] [CrossRef]
Aceto N, Bardia A, Miyamoto DT, Donaldson MC, Wittner BS et al. (2014). Circulating tumor cell clusters are oligoclonal precursors of breast cancer metastasis. Cell 158: 1110–1122. https://doi.org/10.1016/j.cell.2014.07.013 [Google Scholar] [PubMed] [CrossRef]
Alves JM, Estévez-Gómez N, Valecha M, Prado-López S, Tomás L et al. (2022). Comparative analysis of capture methods for genomic profiling of circulating tumor cells in colorectal cancer. Genomics 114: 110500. https://doi.org/10.1016/j.ygeno.2022.110500 [Google Scholar] [PubMed] [CrossRef]
Ankeny JS, Court CM, Hou S, Li Q, Song M et al. (2016). Circulating tumour cells as a biomarker for diagnosis and staging in pancreatic cancer. British Journal of Cancer 114: 1367–1375. https://doi.org/10.1038/bjc.2016.121 [Google Scholar] [PubMed] [CrossRef]
Arnoletti JP, Fanaian N, Reza J, Sause R, Almodovar AJ et al. (2018). Pancreatic and bile duct cancer circulating tumor cells (CTC) form immune-resistant multi-cell type clusters in the portal venous circulation. Cancer Biology & Therapy 19: 887–897. https://doi.org/10.1080/15384047.2018.1480292 [Google Scholar] [PubMed] [CrossRef]
Arnoletti JP, Reza J, Rosales A, Monreal A, Fanaian N et al. (2022). Pancreatic ductal adenocarcinoma (PDAC) circulating tumor cells influence myeloid cell differentiation to support their survival and immunoresistance in portal vein circulation. PLoS One 17: e0265725. https://doi.org/10.1371/journal.pone.0265725 [Google Scholar] [PubMed] [CrossRef]
Aya-Bonilla CA, Morici M, Hong X, McEvoy AC, Sullivan RJ et al. (2020). Detection and prognostic role of heterogeneous populations of melanoma circulating tumour cells. British Journal of Cancer 122: 1059–1067. https://doi.org/10.1038/s41416-020-0750-9 [Google Scholar] [PubMed] [CrossRef]
Barclay AN, van den Berg TK (2014). The interaction between signal regulatory protein alpha (SIRPα) and CD47: Structure, function, and therapeutic target. Annual Review of Immunology 32: 25–50. https://doi.org/10.1146/annurev-immunol-032713-120142 [Google Scholar] [PubMed] [CrossRef]
Barnett ES, Schultz N, Stopsack KH, Lam ET, Arfe A et al. (2022). Analysis of BRCA2 copy number loss and genomic instability in circulating tumor cells from patients with metastatic castration-resistant prostate cancer. European Urology 83: 112–120. https://doi.org/10.1016/j.eururo.2022.08.010 [Google Scholar] [PubMed] [CrossRef]
Basso U, Facchinetti A, Rossi E, Maruzzo M, Conteduca V et al. (2021). Prognostic role of circulating tumor cells in metastatic renal cell carcinoma: A large, multicenter, prospective trial. Oncologist 26: 740–750. https://doi.org/10.1002/onco.13842 [Google Scholar] [PubMed] [CrossRef]
Burr R, Gilles C, Thompson EW, Maheswaran S (2020). Epithelial-mesenchymal plasticity in circulating tumor cells, the precursors of metastasis. Advances in Experimental Medicine and Biology 1220: 11–34. https://doi.org/10.1007/978-3-030-35805-1 [Google Scholar] [CrossRef]
Chen J, Cao S, Situ B, Zhong J, Hu Y et al. (2018a). Metabolic reprogramming-based characterization of circulating tumor cells in prostate cancer. Journal of Experimental & Clinical Cancer Research 37: 127. https://doi.org/10.1186/s13046-018-0789-0 [Google Scholar] [PubMed] [CrossRef]
Chen MB, Hajal C, Benjamin DC, Yu C, Azizgolshani H, Hynes RO, Kamm RD (2018b). Inflamed neutrophils sequestered at entrapped tumor cells via chemotactic confinement promote tumor cell extravasation. Proceedings of the National Academy of Sciences of the United States of America 115: 7022–7027. https://doi.org/10.1073/pnas.1715932115 [Google Scholar] [PubMed] [CrossRef]
Chen P, Hsu WH, Han J, Xia Y, DePinho RA (2021a). Cancer stemness meets immunity: From mechanism to therapy. Cell Reports 34: 108597. https://doi.org/10.1016/j.celrep.2020.108597 [Google Scholar] [PubMed] [CrossRef]
Chen VL, Huang Q, Harouaka R, Du Y, Lok AS, Parikh ND, Garmire LX, Wicha MS (2022). A dual-filtration system for single-cell sequencing of circulating tumor cells and clusters in HCC. Hepatology Communications 6: 1482–1491. https://doi.org/10.1002/hep4.1900 [Google Scholar] [PubMed] [CrossRef]
Chen Y, Li Y, Qi C, Zhang C, Liu D et al. (2021b). Dysregulated KRAS gene-signaling axis and abnormal chromatin remodeling drive therapeutic resistance in heterogeneous-sized circulating tumor cells in gastric cancer. Cancer Letters 517: 78–87. https://doi.org/10.1016/j.canlet.2021.06.002 [Google Scholar] [PubMed] [CrossRef]
Chen CL, Mahalingam D, Osmulski P, Jadhav RR, Wang CM et al. (2013). Single-cell analysis of circulating tumor cells identifies cumulative expression patterns of EMT-related genes in metastatic prostate cancer. Prostate 73: 813–826. https://doi.org/10.1002/pros.22625 [Google Scholar] [PubMed] [CrossRef]
Chen J, Ye C, Dong J, Cao S, Hu Y et al. (2020). Metabolic classification of circulating tumor cells as a biomarker for metastasis and prognosis in breast cancer. Journal of Translational Medicine 18: 59. https://doi.org/10.1186/s12967-020-02237-8 [Google Scholar] [PubMed] [CrossRef]
Cheng Y, Luo L, Zhang J, Zhou M, Tang Y, He G, Lu Y, Wang Z, Pan M (2019). Diagnostic value of different phenotype circulating tumor cells in hepatocellular carcinoma. Journal of Gastrointestinal Surgery 23: 2354–2361. https://doi.org/10.1007/s11605-018-04067-y [Google Scholar] [PubMed] [CrossRef]
Chikamatsu K, Tada H, Takahashi H, Kuwabara-Yokobori Y, Ishii H, Ida S, Shino M (2019). Expression of immune-regulatory molecules in circulating tumor cells derived from patients with head and neck squamous cell carcinoma. Oral Oncology 89: 34–39. https://doi.org/10.1016/j.oraloncology.2018.12.002 [Google Scholar] [PubMed] [CrossRef]
Cho JH, Sim MH, Kim SY, Kim K, Lee T, Lee J, Kang WK, Kim ST (2021). Analysis of intrapatient heterogeneity of circulating tumor cells at the single-cell level in the cerebrospinal fluid of a patient with metastatic gastric cancer. Journal of Cancer Research and Therapeutics 17: 1047–1051. https://doi.org/10.4103/jcrt.JCRT_108_19 [Google Scholar] [PubMed] [CrossRef]
Cho EH, Wendel M, Luttgen M, Yoshioka C, Marrinucci D et al. (2012). Characterization of circulating tumor cell aggregates identified in patients with epithelial tumors. Physical Biology 9: 016001. https://doi.org/10.1088/1478-3975/9/1/016001 [Google Scholar] [PubMed] [CrossRef]
Chong MH, Zhao Y, Wang J, Zha XM, Liu XA, Ling LJ, Du Q, Wang S (2012). The dynamic change of circulating tumour cells in patients with operable breast cancer before and after chemotherapy based on a multimarker QPCR platform. British Journal of Cancer 106: 1605–1610. https://doi.org/10.1038/bjc.2012.157 [Google Scholar] [PubMed] [CrossRef]
Costa C, Muinelo-Romay L, Cebey-López V, Pereira-Veiga T, Martínez-Pena I et al. (2020). Analysis of a real-world cohort of metastatic breast cancer patients shows circulating tumor cell clusters (CTC-clusters) as predictors of patient outcomes. Cancers 12: 1111. https://doi.org/10.3390/cancers12051111 [Google Scholar] [PubMed] [CrossRef]
Deng Z, Wu S, Wang Y, Shi D (2022). Circulating tumor cell isolation for cancer diagnosis and prognosis. eBioMedicine 83: 104237. https://doi.org/10.1016/j.ebiom.2022.104237 [Google Scholar] [PubMed] [CrossRef]
Dive C, Brady G (2017). SnapShot: Circulating tumor cells. Cell 168: 742. https://doi.org/10.1016/j.cell.2017.01.026 [Google Scholar] [PubMed] [CrossRef]
Dong Y, Han Y, Huang Y, Jiang S, Huang Z, Chen R, Yu Z, Yu K, Zhang S (2020). PD-L1 is expressed and promotes the expansion of regulatory T cells in acute myeloid leukemia. Frontiers in Immunology 11: 1710. https://doi.org/10.3389/fimmu.2020.01710 [Google Scholar] [PubMed] [CrossRef]
Du L, Cheng Q, Zheng H, Liu J, Liu L, Chen Q (2022). Targeting stemness of cancer stem cells to fight colorectal cancers. Seminars in Cancer Biology 82: 150–161. https://doi.org/10.1016/j.semcancer.2021.02.012 [Google Scholar] [PubMed] [CrossRef]
Felici C, Mannavola F, Stucci LS, Duda L, Cafforio P, Porta C, Tucci M (2022). Circulating tumor cells from melanoma patients show phenotypic plasticity and metastatic potential in xenograft NOD.CB17 mice. BMC Cancer 22: 754. https://doi.org/10.1186/s12885-022-09829-1 [Google Scholar] [PubMed] [CrossRef]
Follain G, Osmani N, Azevedo AS, Allio G, Mercier L et al. (2018). Hemodynamic forces tune the arrest, adhesion, and extravasation of circulating tumor cells. Developmental Cell 45: 33–52. https://doi.org/10.1016/j.devcel.2018.02.015 [Google Scholar] [PubMed] [CrossRef]
Francescangeli F, Magri V, de Angelis ML, de Renzi G, Gandini O, Zeuner A, Gazzaniga P, Nicolazzo C (2021). Sequential isolation and characterization of single CTCs and large CTC clusters in metastatic colorectal cancer patients. Cancers 13: 6362. https://doi.org/10.3390/cancers13246362 [Google Scholar] [PubMed] [CrossRef]
Genna A, Vanwynsberghe AM, Villard AV, Pottier C, Ancel J, Polette M, Gilles C (2020). EMT-associated heterogeneity in circulating tumor cells: Sticky friends on the road to metastasis. Cancers 12: 1632. https://doi.org/10.3390/cancers12061632 [Google Scholar] [PubMed] [CrossRef]
Guan Y, Xu F, Tian J, Gao K, Wan Z, Wang Y, Gao M, Wang Z, Chong T (2021). The prognostic value of circulating tumour cells (CTCs) and CTC white blood cell clusters in patients with renal cell carcinoma. BMC Cancer 21: 826. https://doi.org/10.1186/s12885-021-08463-7 [Google Scholar] [PubMed] [CrossRef]
Han HJ, Sung JY, Kim SH, Yun UJ, Kim H et al. (2021). Fibronectin regulates anoikis resistance via cell aggregate formation. Cancer Letters 508: 59–72. https://doi.org/10.1016/j.canlet.2021.03.011 [Google Scholar] [PubMed] [CrossRef]
Hegde S, Leader AM, Merad M (2021). MDSC: Markers, development, states, and unaddressed complexity. Immunity 54: 875–884. https://doi.org/10.1016/j.immuni.2021.04.004 [Google Scholar] [PubMed] [CrossRef]
Ho DW, Tsui YM, Sze KM, Chan LK, Cheung TT, Lee E, Sham PC, Tsui SK, Lee TK, Ng IO (2019). Single-cell transcriptomics reveals the landscape of intra-tumoral heterogeneity and stemness-related subpopulations in liver cancer. Cancer Letters 459: 176–185. https://doi.org/10.1016/j.canlet.2019.06.002 [Google Scholar] [PubMed] [CrossRef]
Hong X, Roh W, Sullivan RJ, Wong KHK, Wittner BS et al. (2021). The lipogenic regulator SREBP2 induces transferrin in circulating melanoma cells and suppresses ferroptosis. Cancer Discovery 11: 678–695. https://doi.org/10.1158/2159-8290.CD-19-1500 [Google Scholar] [PubMed] [CrossRef]
Hoxhaj G, Manning BD (2020). The PI3K-AKT network at the interface of oncogenic signalling and cancer metabolism. Nature Reviews Cancer 20: 74–88. https://doi.org/10.1038/s41568-019-0216-7 [Google Scholar] [PubMed] [CrossRef]
Huang Q, Li S, Hu X, Sun M, Wu Q et al. (2020). Shear stress activates ATOH8 via autocrine VEGF promoting glycolysis dependent-survival of colorectal cancer cells in the circulation. Journal of Experimental & Clinical Cancer Research 39: 25. https://doi.org/10.1186/s13046-020-1533-0 [Google Scholar] [PubMed] [CrossRef]
Jacot W, Mazel M, Mollevi C, Pouderoux S, D’Hondt V et al. (2020). Clinical correlations of programmed cell death ligand 1 status in liquid and standard biopsies in breast cancer. Clinical Chemistry 66: 1093–1101. https://doi.org/10.1093/clinchem/hvaa121 [Google Scholar] [PubMed] [CrossRef]
Jerabkova-Roda K, Dupas A, Osmani N, Hyenne V, Goetz JG (2022). Circulating extracellular vesicles and tumor cells: Sticky partners in metastasis. Trends Cancer 8: 799–805. https://doi.org/10.1016/j.trecan.2022.05.002 [Google Scholar] [PubMed] [CrossRef]
Joo HJ, Chung GE, Han S, Ka HI, Soh SJ, Yang Y (2022). Adapted suspension tumor cells rewire metabolic pathways for anchorage-independent survival through AKT activation. Experimental Cell Research 411: 113005. https://doi.org/10.1016/j.yexcr.2021.113005 [Google Scholar] [PubMed] [CrossRef]
Jordan NV, Bardia A, Wittner BS, Benes C, Ligorio M et al. (2016). HER2 expression identifies dynamic functional states within circulating breast cancer cells. Nature 537: 102–106. https://doi.org/10.1038/nature19328 [Google Scholar] [PubMed] [CrossRef]
Kallergi G, Aggouraki D, Zacharopoulou N, Stournaras C, Georgoulias V, Martin SS (2018). Evaluation of α-tubulin, detyrosinated α-tubulin, and vimentin in CTCs: Identification of the interaction between CTCs and blood cells through cytoskeletal elements. Breast Cancer Research 20: 67. https://doi.org/10.1186/s13058-018-0993-z [Google Scholar] [PubMed] [CrossRef]
Kapeleris J, Müller Bark J, Ranjit S, Irwin D, Hartel G et al. (2022). Prognostic value of integrating circulating tumour cells and cell-free DNA in non-small cell lung cancer. Heliyon 8: e09971. https://doi.org/10.1016/j.heliyon.2022.e09971 [Google Scholar] [PubMed] [CrossRef]
Khan T, Becker TM, Po JW, Chua W, Ma Y (2022). Single-circulating tumor cell whole genome amplification to unravel cancer heterogeneity and actionable biomarkers. International Journal of Molecular Sciences 23: 8386. https://doi.org/10.3390/ijms23158386 [Google Scholar] [PubMed] [CrossRef]
Kim TM, Yoo JS, Moon HW, Hur KJ, Choi JB, Hong SH, Lee JY, Ha US (2020). Distinct mutation profiles between primary bladder cancer and circulating tumor cells warrant the use of circulating tumors cells as cellular resource for mutation follow-up. BMC Cancer 20: 1203. https://doi.org/10.1186/s12885-020-07684-6 [Google Scholar] [PubMed] [CrossRef]
Knoche SM, Larson AC, Sliker BH, Poelaert BJ, Solheim JC (2021). The role of tumor heterogeneity in immune-tumor interactions. Cancer Metastasis Reviews 40: 377–389. https://doi.org/10.1007/s10555-021-09957-3 [Google Scholar] [PubMed] [CrossRef]
Kondo Y, Hayashi K, Kawakami K, Miwa Y, Hayashi H, Yamamoto M (2017). KRAS mutation analysis of single circulating tumor cells from patients with metastatic colorectal cancer. BMC Cancer 17: 311. https://doi.org/10.1186/s12885-017-3305-6 [Google Scholar] [PubMed] [CrossRef]
Lee TK, Guan XY, Ma S (2022). Cancer stem cells in hepatocellular carcinoma—From origin to clinical implications. Nature Reviews Gastroenterology & Hepatology 19: 26–44. https://doi.org/10.1038/s41575-021-00508-3 [Google Scholar] [PubMed] [CrossRef]
Li Y, Gong J, Zhang Q, Lu Z, Gao J, Li Y, Cao Y, Shen L (2016). Dynamic monitoring of circulating tumour cells to evaluate therapeutic efficacy in advanced gastric cancer. British Journal of Cancer 114: 138–145. https://doi.org/10.1038/bjc.2015.417 [Google Scholar] [PubMed] [CrossRef]
Li W, Tanikawa T, Kryczek I, Xia H, Li G et al. (2018). Aerobic glycolysis controls myeloid-derived suppressor cells and tumor immunity via a specific CEBPB isoform in triple-negative breast cancer. Cell Metabolism 28: 87–103. https://doi.org/10.1016/j.cmet.2018.04.022 [Google Scholar] [PubMed] [CrossRef]
Li K, Wu R, Zhou M, Tong H, Luo KQ (2021). Desmosomal proteins of DSC2 and PKP1 promote cancer cells survival and metastasis by increasing cluster formation in circulatory system. Science Advances 7: eabg7265. https://doi.org/10.1126/sciadv.abg7265 [Google Scholar] [PubMed] [CrossRef]
Lian S, Xie R, Ye Y, Lu Y, Cheng Y, Xie X, Li S, Jia L (2019). Dual blockage of both PD-L1 and CD47 enhances immunotherapy against circulating tumor cells. Scientific Reports 9: 4532. https://doi.org/10.1038/s41598-019-40241-1 [Google Scholar] [PubMed] [CrossRef]
Lim M, Park S, Jeong HO, Park SH, Kumar S, Jang A, Lee S, Kim DU, Cho YK (2021). Circulating tumor cell clusters are cloaked with platelets and correlate with poor prognosis in unresectable pancreatic cancer. Cancers 13: 5272. https://doi.org/10.3390/cancers13215272 [Google Scholar] [PubMed] [CrossRef]
Linzer RW, Guida DL, Aminov J, Snider JM, Khalife G et al. (2022). Dihydroceramide desaturase 1 (DES1) promotes anchorage-independent survival downstream of HER2-driven glucose uptake and metabolism. FASEB Journal 36: e22558. https://doi.org/10.1096/fj.202200748R [Google Scholar] [PubMed] [CrossRef]
Liu X, Taftaf R, Kawaguchi M, Chang YF, Chen W et al. (2019). Homophilic CD44 interactions mediate tumor cell aggregation and polyclonal metastasis in patient-derived breast cancer models. Cancer Discovery 9: 96–113. https://doi.org/10.1158/2159-8290.CD-18-0065 [Google Scholar] [PubMed] [CrossRef]
Liu T, Xu H, Huang M, Ma W, Saxena D et al. (2018). Circulating glioma cells exhibit stem cell-like properties. Cancer Research 78: 6632–6642. https://doi.org/10.1158/0008-5472.CAN-18-0650 [Google Scholar] [PubMed] [CrossRef]
Maeshiro M, Shinriki S, Liu R, Nakachi Y, Komohara Y et al. (2021). Colonization of distant organs by tumor cells generating circulating homotypic clusters adaptive to fluid shear stress. Scientific Reports 11: 6150. https://doi.org/10.1038/s41598-021-85743-z [Google Scholar] [PubMed] [CrossRef]
Manjunath Y, Upparahalli SV, Suvilesh KN, Avella DM, Kimchi ET, Staveley-O’Carroll KF, Li G, Kaifi JT (2019). Circulating tumor cell clusters are a potential biomarker for detection of non-small cell lung cancer. Lung Cancer 34: 147–150. https://doi.org/10.1016/j.lungcan.2019.06.016 [Google Scholar] [PubMed] [CrossRef]
Martínez-Reyes I, Chandel NS (2021). Cancer metabolism: Looking forward. Nature Reviews Cancer 21: 669–680. https://doi.org/10.1038/s41568-021-00378-6 [Google Scholar] [PubMed] [CrossRef]
Mason JA, Cockfield JA, Pape DJ, Meissner H, Sokolowski MT et al. (2021). SGK1 signaling promotes glucose metabolism and survival in extracellular matrix detached cells. Cell Reports 34: 108821. https://doi.org/10.1016/j.celrep.2021.108821 [Google Scholar] [PubMed] [CrossRef]
Massagué J, Obenauf AC (2016). Metastatic colonization by circulating tumour cells. Nature 529: 298–306. https://doi.org/10.1038/nature17038 [Google Scholar] [PubMed] [CrossRef]
Meng S, Tripathy D, Frenkel EP, Shete S, Naftalis EZ et al. (2004). Circulating tumor cells in patients with breast cancer dormancy. Clinical Cancer Research 10: 8152–8162. https://doi.org/10.1158/1078-0432.CCR-04-1110 [Google Scholar] [PubMed] [CrossRef]
Micalizzi DS, Maheswaran S, Haber DA (2017). A conduit to metastasis: Circulating tumor cell biology. Genes & Development 31: 1827–1840. https://doi.org/10.1101/gad.305805.117 [Google Scholar] [PubMed] [CrossRef]
Nagahara M, Mimori K, Kataoka A, Ishii H, Tanaka F, Nakagawa T, Sato T, Ono S, Sugihara K, Mori M (2010). Correlated expression of CD47 and SIRPA in bone marrow and in peripheral blood predicts recurrence in breast cancer patients. Clinical Cancer Research 16: 4625–4635. https://doi.org/10.1158/1078-0432.CCR-10-0349 [Google Scholar] [PubMed] [CrossRef]
Najafi M, Mortezaee K, Majidpoor J (2019). Cancer stem cell (CSC) resistance drivers. Life Sciences 234: 116781. https://doi.org/10.1016/j.lfs.2019.116781 [Google Scholar] [PubMed] [CrossRef]
Nicolazzo C, Raimondi C, Mancini M, Caponnetto S, Gradilone A et al. (2016). Monitoring PD-L1 positive circulating tumor cells in non-small cell lung cancer patients treated with the PD-1 inhibitor Nivolumab. Scientific Reports 6: 31726. https://doi.org/10.1038/srep31726 [Google Scholar] [PubMed] [CrossRef]
Ning Y, Zhang W, Hanna DL, Yang D, Okazaki S et al. (2018). Clinical relevance of EMT and stem-like gene expression in circulating tumor cells of metastatic colorectal cancer patients. Pharmacogenomics Journal 18: 29–34. https://doi.org/10.1038/tpj.2016.62 [Google Scholar] [PubMed] [CrossRef]
Ntzifa A, Strati A, Kallergi G, Kotsakis A, Georgoulias V, Lianidou E (2021). Gene expression in circulating tumor cells reveals a dynamic role of EMT and PD-L1 during osimertinib treatment in NSCLC patients. Scientific Reports 11: 2313. https://doi.org/10.1038/s41598-021-82068-9 [Google Scholar] [PubMed] [CrossRef]
Osmulski PA, Cunsolo A, Chen M, Qian Y, Lin CL et al. (2021). Contacts with macrophages promote an aggressive nanomechanical phenotype of circulating tumor cells in prostate cancer. Cancer Research 81: 4110–4123. https://doi.org/10.1158/0008-5472.CAN-20-3595 [Google Scholar] [PubMed] [CrossRef]
Pang S, Li H, Xu S, Feng L, Ma X, Chu Y, Zou B, Wang S, Zhou G (2021a). Circulating tumour cells at baseline and late phase of treatment provide prognostic value in breast cancer. Scientific Reports 11: 13441. https://doi.org/10.1038/s41598-021-92876-8 [Google Scholar] [PubMed] [CrossRef]
Pang TCY, Po JW, Becker TM, Goldstein D, Pirola RC, Wilson JS, Apte MV (2021b). Circulating tumour cells in pancreatic cancer: A systematic review and meta-analysis of clinicopathological implications. Pancreatology 21: 103–114. https://doi.org/10.1016/j.pan.2020.11.022 [Google Scholar] [PubMed] [CrossRef]
Pantazaka E, Ntzifa A, Roumeliotou A, Lianidou E, Georgoulias V, Kotsakis A, Kallergi G (2022). PD-L1/pS6 in circulating tumor cells (CTCs) during osimertinib treatment in patients with non-small cell lung cancer (NSCLC). Biomedicines 10: 1893. https://doi.org/10.3390/biomedicines10081893 [Google Scholar] [PubMed] [CrossRef]
Paoletti C, Cani AK, Larios JM, Hovelson DH, Aung K et al. (2018). Comprehensive mutation and copy number profiling in archived circulating breast cancer tumor cells documents heterogeneous resistance mechanisms. Cancer Research 78: 1110–1122. https://doi.org/10.1158/0008-5472.CAN-17-2686 [Google Scholar] [PubMed] [CrossRef]
Papadaki MA, Koutsopoulos AV, Tsoulfas PG, Lagoudaki E, Aggouraki D et al. (2020). Clinical relevance of immune checkpoints on circulating tumor cells in breast cancer. Cancers 12: 376. https://doi.org/10.3390/cancers12020376 [Google Scholar] [PubMed] [CrossRef]
Papadaki MA, Stoupis G, Theodoropoulos PA, Mavroudis D, Georgoulias V, Agelaki S (2019). Circulating tumor cells with stemness and epithelial-to-mesenchymal transition features are chemoresistant and predictive of poor outcome in metastatic breast cancer. Molecular Cancer Therapeutics 18: 437–447. https://doi.org/10.1158/1535-7163.MCT-18-0584 [Google Scholar] [PubMed] [CrossRef]
Perea Paizal J, Au SH, Bakal C (2021). Squeezing through the microcirculation: Survival adaptations of circulating tumour cells to seed metastasis. British Journal of Cancer 124: 58–65. https://doi.org/10.1038/s41416-020-01176-x [Google Scholar] [PubMed] [CrossRef]
Pereira-Veiga T, Schneegans S, Pantel K, Wikman H (2022). Circulating tumor cell-blood cell crosstalk: Biology and clinical relevance. Cell Reports 40: 111298. https://doi.org/10.1016/j.celrep.2022.111298 [Google Scholar] [PubMed] [CrossRef]
Pernot S, Badoual C, Terme M, Castan F, Cazes A et al. (2017). Dynamic evaluation of circulating tumour cells in patients with advanced gastric and oesogastric junction adenocarcinoma: Prognostic value and early assessment of therapeutic effects. European Journal of Cancer 79: 15–22. https://doi.org/10.1016/j.ejca.2017.03.036 [Google Scholar] [PubMed] [CrossRef]
Pixberg CF, Raba K, Müller F, Behrens B, Honisch E et al. (2017). Analysis of DNA methylation in single circulating tumor cells. Oncogene 36: 3223–3231. https://doi.org/10.1038/onc.2016.480 [Google Scholar] [PubMed] [CrossRef]
Piñeiro R, Martínez-Pena I, López-López R (2020). Relevance of CTC clusters in breast cancer metastasis. Advances in Experimental Medicine and Biology 1220: 93–115. https://doi.org/10.1007/978-3-030-35805-1 [Google Scholar] [CrossRef]
Ponomarev A, Gilazieva Z, Solovyeva V, Allegrucci C, Rizvanov A (2022). Intrinsic and extrinsic factors impacting cancer stemness and tumor progression. Cancers 14: 970. https://doi.org/10.3390/cancers14040970 [Google Scholar] [PubMed] [CrossRef]
Qi LN, Xiang BD, Wu FX, Ye JZ, Zhong JH et al. (2018). Circulating tumor cells undergoing EMT provide a metric for diagnosis and prognosis of patients with hepatocellular carcinoma. Cancer Research 78: 4731–4744. https://doi.org/10.1158/0008-5472.CAN-17-2459 [Google Scholar] [PubMed] [CrossRef]
Rossi E, Fassan M, Aieta M, Zilio F, Celadin R et al. (2012). Dynamic changes of live/apoptotic circulating tumour cells as predictive marker of response to sunitinib in metastatic renal cancer. British Journal of Cancer 107: 1286–1294. https://doi.org/10.1038/bjc.2012.388 [Google Scholar] [PubMed] [CrossRef]
Ruan H, Zhou Y, Shen J, Zhai Y, Xu Y et al. (2020). Circulating tumor cell characterization of lung cancer brain metastases in the cerebrospinal fluid through single-cell transcriptome analysis. Clinical and Translational Medicine 10: e246. https://doi.org/10.1002/ctm2.246 [Google Scholar] [PubMed] [CrossRef]
Sajdik C, Schuster E, Holzer B, Krainer M, Deutschmann C, Peter S, Marhold M, Zeillinger R, Obermayr E (2022). Comparison of microfluidic platforms for the enrichment of circulating tumor cells in breast cancer patients. Breast Cancer Research and Treatment 196: 75–85. https://doi.org/10.1007/s10549-022-06717-x [Google Scholar] [PubMed] [CrossRef]
Schuster E, Taftaf R, Reduzzi C, Albert MK, Romero-Calvo I, Liu H (2021). Better together: Circulating tumor cell clustering in metastatic cancer. Trends Cancer 7: 1020–1032. https://doi.org/10.1016/j.trecan.2021.07.001 [Google Scholar] [PubMed] [CrossRef]
Semaan A, Bernard V, Kim DU, Lee JJ, Huang J et al. (2021). Characterisation of circulating tumour cell phenotypes identifies a partial-EMT sub-population for clinical stratification of pancreatic cancer. British Journal of Cancer 124: 1970–1977. https://doi.org/10.1038/s41416-021-01350-9 [Google Scholar] [PubMed] [CrossRef]
Shait Mohammed MR, Zamzami M, Choudhry H, Ahmed F, Ateeq B, Khan MI (2022). The histone H3K27me3 demethylases KDM6A/B resist anoikis and transcriptionally regulate stemness-related genes. Frontiers in Cell and Developmental Biology 10: 780176. https://doi.org/10.3389/fcell.2022.780176 [Google Scholar] [PubMed] [CrossRef]
Sharma U, Medina-Saenz K, Miller PC, Troness B, Spartz A, Sandoval-Leon A, Parke DN, Seagroves TN, Lippman ME, El-Ashry D (2021). Heterotypic clustering of circulating tumor cells and circulating cancer-associated fibroblasts facilitates breast cancer metastasis. Breast Cancer Research and Treatment 189: 63–80. https://doi.org/10.1007/s10549-021-06299-0 [Google Scholar] [PubMed] [CrossRef]
Shi Z, Wang B, Chihanga T, Kennedy MA, Weber GF (2014). Energy metabolism during anchorage-independence. Induction by osteopontin-c. PLoS One 9: e105675. https://doi.org/10.1371/journal.pone.0105675 [Google Scholar] [PubMed] [CrossRef]
Sinoquet L, Jacot W, Gauthier L, Pouderoux S, Viala M, Cayrefourcq L, Quantin X, Alix-Panabières C (2021). Programmed cell death ligand 1-expressing circulating tumor cells: A new prognostic biomarker in non-small cell lung cancer. Clinical Chemistry 67: 1503–1512. https://doi.org/10.1093/clinchem/hvab131 [Google Scholar] [PubMed] [CrossRef]
Spiliotaki M, Neophytou CM, Vogazianos P, Stylianou I, Gregoriou G, Constantinou AI, Deltas C, Charalambous H (2022). Dynamic monitoring of PD-L1 and Ki67 in circulating tumor cells of metastatic non-small cell lung cancer patients treated with pembrolizumab. Molecular Oncology 8: 13083. https://doi.org/10.1002/1878-0261.13317 [Google Scholar] [PubMed] [CrossRef]
Sprouse ML, Welte T, Boral D, Liu HN, Yin W et al. (2019). PMN-MDSCs enhance CTC metastatic properties through reciprocal interactions via ROS/Notch/Nodal signaling. International Journal of Molecular Sciences 20: 1916. https://doi.org/10.3390/ijms20081916 [Google Scholar] [PubMed] [CrossRef]
Steinert G, Schölch S, Niemietz T, Iwata N, García SA et al. (2014). Immune escape and survival mechanisms in circulating tumor cells of colorectal cancer. Cancer Research 74: 1694–1704. https://doi.org/10.1158/0008-5472.CAN-13-1885 [Google Scholar] [PubMed] [CrossRef]
Stewart CA, Gay CM, Xi Y, Sivajothi S, Sivakamasundari V et al. (2020). Single-cell analyses reveal increased intratumoral heterogeneity after the onset of therapy resistance in small-cell lung cancer. Nature Cancer 1: 423–436. https://doi.org/10.1038/s43018-019-0020-z [Google Scholar] [PubMed] [CrossRef]
Stock C (2022). Circulating tumor cells: Does ion transport contribute to intravascular survival, adhesion, extravasation, and metastatic organotropism? Reviews of Physiology, Biochemistry and Pharmacology 182: 139–175. https://doi.org/10.1007/978-3-030-99800-4 [Google Scholar] [CrossRef]
Su Z, Wang Z, Ni X, Duan J, Gao Y et al. (2019). Inferring the evolution and progression of small-cell lung cancer by single-cell sequencing of circulating tumor cells. Clinical Cancer Research 25: 5049–5060. https://doi.org/10.1158/1078-0432.CCR-18-3571 [Google Scholar] [PubMed] [CrossRef]
Sun YF, Guo W, Xu Y, Shi YH, Gong ZJ et al. (2018). Circulating tumor cells from different vascular sites exhibit spatial heterogeneity in epithelial and mesenchymal composition and distinct clinical significance in hepatocellular carcinoma. Clinical Cancer Research 24: 547–559. https://doi.org/10.1158/1078-0432.CCR-17-1063 [Google Scholar] [PubMed] [CrossRef]
Sun YF, Wu L, Liu SP, Jiang MM, Hu B et al. (2021a). Dissecting spatial heterogeneity and the immune-evasion mechanism of CTCs by single-cell RNA-seq in hepatocellular carcinoma. Nature Communications 12: 4091. https://doi.org/10.1038/s41467-021-24386-0 [Google Scholar] [PubMed] [CrossRef]
Sun Y, Zhou J, Fan J, Yang X (2021b). Single-cell RNA sequencing reveals spatial heterogeneity and immune evasion of circulating tumor cells. Cancer Biology & Medicine 18: 934–936. https://doi.org/10.20892/j.issn.2095-3941.2021.0466 [Google Scholar] [PubMed] [CrossRef]
Suvilesh KN, Nussbaum YI, Radhakrishnan V, Manjunath Y, Avella DM et al. (2022). Tumorigenic circulating tumor cells from xenograft mouse models of non-metastatic NSCLC patients reveal distinct single cell heterogeneity and drug responses. Molecular Cancer 21: 73. https://doi.org/10.1186/s12943-022-01553-5 [Google Scholar] [PubMed] [CrossRef]
Taftaf R, Liu X, Singh S, Jia Y, Dashzeveg NK et al. (2021). ICAM1 initiates CTC cluster formation and trans-endothelial migration in lung metastasis of breast cancer. Nature Communications 12: 4867. https://doi.org/10.1038/s41467-021-25189-z [Google Scholar] [PubMed] [CrossRef]
Tan Z, Yue C, Ji S, Zhao C, Jia R et al. (2021). Assessment of PD-L1 expression on circulating tumor cells for predicting clinical outcomes in patients with cancer receiving PD-1/PD-L1 blockade therapies. Oncologist 26: e2227–e2238. https://doi.org/10.1002/onco.13981 [Google Scholar] [PubMed] [CrossRef]
Tang Y, Zhang Z, Chen Y, Qin S, Zhou L, Gao W, Shen Z (2022). Metabolic adaptation-mediated cancer survival and progression in oxidative stress. Antioxidants 11: 1324. https://doi.org/10.3390/antiox11071324 [Google Scholar] [PubMed] [CrossRef]
Teng T, Kamal M, Iriondo O, Amzaleg Y, Luo C et al. (2021). N-Acetyl-L-cysteine promotes ex vivo growth and expansion of single circulating tumor cells by mitigating cellular stress responses. Molecular Cancer Research 19: 441–450. https://doi.org/10.1158/1541-7786.MCR-20-0482 [Google Scholar] [PubMed] [CrossRef]
Tsai WS, Hung WS, Wang TM, Liu H, Yang CY, Wu SM, Hsu HL, Hsiao YC, Tsai HJ, Tseng CP (2021). Circulating tumor cell enumeration for improved screening and disease detection of patients with colorectal cancer. Biomedical Journal 44: S190–S200. https://doi.org/10.1016/j.bj.2020.09.006 [Google Scholar] [PubMed] [CrossRef]
Wang C, Mu Z, Chervoneva I, Austin L, Ye Z et al. (2017). Longitudinally collected CTCs and CTC-clusters and clinical outcomes of metastatic breast cancer. Breast Cancer Research and Treatment 161: 83–94. https://doi.org/10.1007/s10549-016-4026-2 [Google Scholar] [PubMed] [CrossRef]
Wang W, Wan L, Wu S, Yang J, Zhou Y, Liu F, Wu Z, Cheng Y (2018). Mesenchymal marker and LGR5 expression levels in circulating tumor cells correlate with colorectal cancer prognosis. Cellular Oncology 41: 495–504. https://doi.org/10.1007/s13402-018-0386-4 [Google Scholar] [PubMed] [CrossRef]
Wang C, Zhang Z, Chong W, Luo R, Myers RE et al. (2021a). Improved prognostic stratification using circulating tumor cell clusters in patients with metastatic castration-resistant prostate cancer. Cancers 13: 268. https://doi.org/10.3390/cancers13020268 [Google Scholar] [PubMed] [CrossRef]
Wang D, Zhang L, Hu A, Wang Y, Liu Y, Yang J, Du N, An X, Wu C, Liu C (2021b). Loss of 4.1N in epithelial ovarian cancer results in EMT and matrix-detached cell death resistance. Protein & Cell 12: 107–127. https://doi.org/10.1007/s13238-020-00723-9 [Google Scholar] [PubMed] [CrossRef]
Wang LN, Zhang ZT, Wang L, Wei HX, Zhang T, Zhang LM, Lin H, Zhang H, Wang SQ (2022). TGF-β1/SH2B3 axis regulates anoikis resistance and EMT of lung cancer cells by modulating JAK2/STAT3 and SHP2/Grb2 signaling pathways. Cell Death & Disease 13: 472. https://doi.org/10.1038/s41419-022-04890-x [Google Scholar] [PubMed] [CrossRef]
Wang YW, Li LL, Lu M, Li H, Hu KW (2022). Stem cell-like circulating tumor cells indicate poor prognosis in gastric cancer. Archives of Medical Science 18: 1297–1307. https://doi.org/10.1155/2014/981261 [Google Scholar] [PubMed] [CrossRef]
Werner S, Stenzl A, Pantel K, Todenhöfer T (2017). Expression of epithelial mesenchymal transition and cancer stem cell markers in circulating tumor cells. Advances in Experimental Medicine and Biology 994: 205–228. https://doi.org/10.1007/978-3-319-55947-6 [Google Scholar] [CrossRef]
Wu F, Fan J, He Y, Xiong A, Yu J et al. (2021). Single-cell profiling of tumor heterogeneity and the microenvironment in advanced non-small cell lung cancer. Nature Communications 12: 2540. https://doi.org/10.1038/s41467-021-22801-0 [Google Scholar] [PubMed] [CrossRef]
Xin Y, Li K, Yang M, Tan Y (2020). Fluid shear stress induces EMT of circulating tumor cells via JNK signaling in favor of their survival during hematogenous dissemination. International Journal of Molecular Sciences 21: 8115. https://doi.org/10.3390/ijms21218115 [Google Scholar] [PubMed] [CrossRef]
Xiong G, Chen J, Zhang G, Wang S, Kawasaki K et al. (2020). Hsp47 promotes cancer metastasis by enhancing collagen-dependent cancer cell-platelet interaction. Proceedings of the National Academy of Sciences of the United States of America 117: 3748–3758. https://doi.org/10.1073/pnas.1911951117 [Google Scholar] [PubMed] [CrossRef]
Yu M, Bardia A, Wittner BS, Stott SL, Smas ME et al. (2013). Circulating breast tumor cells exhibit dynamic changes in epithelial and mesenchymal composition. Science 339: 580–584. https://doi.org/10.1126/science.1228522 [Google Scholar] [PubMed] [CrossRef]
Zafeiriadou A, Kollias I, Londra T, Tsaroucha E, Georgoulias V, Kotsakis A, Lianidou E, Markou A (2022). Metabolism-related gene expression in circulating tumor cells from patients with early stage non-small cell lung cancer. Cancers 14: 3237. https://doi.org/10.3390/cancers14133237 [Google Scholar] [PubMed] [CrossRef]
Zhang T, Agarwal A, Almquist RG, Runyambo D, Park S et al. (2021). Expression of immune checkpoints on circulating tumor cells in men with metastatic prostate cancer. Biomarker Research 9: 14. https://doi.org/10.1186/s40364-021-00267-y [Google Scholar] [PubMed] [CrossRef]
Zhang X, Ekanayake WC, Hughes BGM, Vasani S, Liu ZY et al. (2022). Application of circulating tumour cells to predict response to treatment in head and neck cancer. Cellular Oncology (Dordrecht) 45: 543–555. https://doi.org/10.1007/s13402-022-00681-w [Google Scholar] [PubMed] [CrossRef]
Zhang L, Guo X, Zhang D, Fan Y, Qin L, Dong S, Zhang L (2017). Upregulated miR-132 in Lgr5+ gastric cancer stem cell-like cells contributes to cisplatin-resistance via SIRT1/CREB/ABCG2 signaling pathway. Molecular Carcinogenesis 56: 2022–2034. https://doi.org/10.1002/mc.22656 [Google Scholar] [PubMed] [CrossRef]
Zhang B, Stewart CA, Wang Q, Cardnell RJ, Rocha P et al. (2022a). Dynamic expression of Schlafen 11 (SLFN11) in circulating tumour cells as a liquid biomarker in small cell lung cancer. British Journal of Cancer 127: 569–576. https://doi.org/10.1038/s41416-022-01811-9 [Google Scholar] [PubMed] [CrossRef]
Zhang R, Xia J, Wang Y, Cao M, Jin D, Xue W, Huang Y, Chen H (2020). Co-expression of stem cell and epithelial mesenchymal transition markers in circulating tumor cells of bladder cancer patients. OncoTargets and Therapy 13: 10739–10748. https://doi.org/10.2147/OTT.S259240 [Google Scholar] [PubMed] [CrossRef]
Zhu L, Hissa B, Győrffy B, Jann JC, Yang C, Reissfelder C, Schölch S (2020). Characterization of stem-like circulating tumor cells in pancreatic cancer. Diagnostics 10: 305. https://doi.org/10.3390/diagnostics10050305 [Google Scholar] [PubMed] [CrossRef]
Cite This Article
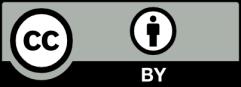