Open Access
REVIEW
The effects of hormone-mediated PI3K/AKT signaling on spermatogenesis in Sertoli cells
The Sperm Laboratory, College of Life Sciences, Zhejiang University, Hangzhou, 310058, China
* Corresponding Author: WANXI YANG. Email:
(This article belongs to the Special Issue: Cellular Signal Transduction in Biological Activities)
BIOCELL 2023, 47(8), 1709-1725. https://doi.org/10.32604/biocell.2023.030379
Received 03 April 2023; Accepted 06 May 2023; Issue published 28 August 2023
Abstract
The phosphoinositide-3-kinase/Akt (PI3K/AKT) signaling pathway is crucial for Sertoli cell development and completing spermatogenesis. Its main role is to promote proliferation and inhibit apoptosis. Many factors activate the PI3K/AKT pathway, like hormones, such as follicle stimulating hormone (FSH), androgen, estrogen, insulin to name a few. Many of these factors have receptors inside or on the surface of Sertoli cells (SCs). This review summarizes how these hormones directly regulate the PI3K/AKT signaling pathway in SCs, which in turn affects SC proliferation and differentiation. Further, hormone-mediated PI3K/AKT signaling also stimulates SC secretion, which is essential for germ cell development, suggesting an indirect role of PI3K/AKT signaling during spermatogenesis. These functions include promoting spermatogonia proliferation and differentiation, meiosis of spermatocytes, sperm maturation, and their release. This review also provides potential hints for clinically treating male infertility issues like cryptorchidism and Sertoli cell-only syndrome.Keywords
The migration of primordial germ cells (PGCs) into the developing testis is the first step of seminiferous cord formation. At this point they begin to interact with SC progenitor cells called mesenchymal cells, after which seminiferous cords can be formed. Then PGCs transform into gonocytes, which are surrounded by the immature SCs making them migrate to the tubule basement membrane, where they acquire the ability to form type A spermatogonia (de Kretser et al., 1998). Type A spermatogonia are of three types: As, Apr, and Aal, which all retain stem cell properties and among the As type provides the spermatogonial stem cells (SSCs). Spermatogenesis originates from the self-renewal of SSCs (Lord et al., 2018). After undergoing non-mitotic differentiation, SSCs differentiate into type A1 spermatogonia, which then form A2, A3, A4, intermediate (Int), and B spermatogonia, the source of preleptotene spermatocytes and the initiation of meiosis. Subsequently, A1, A2, A3, A4, and B type spermatogonia undergo differentiation and lose their ability of self-renewal (Li et al., 2019). The passage across the blood-testis barrier (BTB) is a requirement for the development process of preleptotene and leptotene spermatocytes into pachytene spermatocytes (Russell, 1977; Lui et al., 2003). After the first and the second meiotic division, primary spermatocytes form secondary spermatocytes and subsequently into round spermatids. These round spermatids then transform into elongated spermatids during the process of spermiogenesis (Qian et al., 2014). Finally, spermatozoa are formed and released into the tubular lumen via a process called spermiation (Qian et al., 2014).
SCs are the dominant somatic cells in the seminiferous tubules that maintain and control spermatogenesis. This is mainly by providing a suitable microenvironment for GCs, ensuring the successful completion of spermatogenesis (Lim and Hwang, 1995). SCs also provide nutrients for germ cell development as they convert glucose to lactate, which then can be transported into germ cells (Rato et al., 2012), where lactate dehydrogenase (LDH) plays a crucial role. In addition, SCs secrete many factors necessary for spermatogenesis (Griswold, 1998), including cytokines and hormones, like stem cell factor (SCF), glial cell line-derived neurotrophic factor (GDNF), fibroblast growth factor (FGF), and anti-Müllerian hormone (AMH) along with transport proteins such as androgen binding protein (ABP) and transferrin (Griswold, 1988). Mature SCs also establish the blood-testis barrier (BTB) structure, which divides the seminiferous tubules into basal and abluminal compartments (Dym and Fawcett, 1970) and protects GCs from attack by the immune system (Meng et al., 2011). The BTB is composed of a variety of cell junction proteins, like tight junctions (TJ), gap junctions (GJ), desmosomes, and basal ectoplasmic specialization (ES) (Wang et al., 2022).
Given that SCs have so many documented functions, a better knowledge about them will be important for the control of spermatogenesis. It is known there are many hormone receptors inside or on the surface of SCs. Once ligands bind to these receptors, the receptors can mediate several essential signaling pathways, such as the PI3K/AKT pathway. PI3K is composed of a regulatory subunit (p85) and a catalytic subunit (p110) and at the membrane, it converts phosphoinositide (PIP) 2 lipids to PIP3. PIP3 recruits two kinases, PDK1 and PDK2 (mTORC2), thereby phosphorylating AKT at Thr308 and Ser473, and leading to its activation. After activation, AKT mediates cell growth and cell survival through various substrates, the major of which is the mammalian target of rapamycin (mTOR) (Ersahin et al., 2015).
Our previous review highlighted that some hormones like follicle-stimulating hormone (FSH), estrogen 2 (E2), and thyroid hormone 3 (TH3) can regulate the PI3K/AKT signaling in SCs (Chen et al., 2022). However, their detailed mechanisms and effects on spermatogenesis were not completely discussed. In this review, we summarize several essential hormones which can directly regulate the PI3K/AKT signaling pathway in SCs, as well as the consequent impacts on SC development and spermatogenesis. Additionally, we reason that this review can provide clinical hints for treating male infertility.
Methodology and search strategy
We first surveyed articles in the PubMed database with the terms of “hormone including” “follicle-stimulating hormone” OR “FSH”, “androgen” OR “testosterone”, “estrogen” OR “17β-estradiol”, “insulin”, “insulin-like growth factors” OR “IGF”, “relaxin”, “thyroid hormone”, “retinoic acid”, “PI3K/AKT”, “Sertoli cell”, “blood-testis barrier” OR “BTB”, “in testes”. We then selected articles that met the criteria that hormones exert influences on SC development by regulating the PI3K/AKT signaling (Table 1). Further, we collected articles by searching terms containing “Sertoli cell secretion” (including “SCF”, “GDNF”, “AMH”, “transferrin”, “ABP”, “connexin 43”, “ZO-1”, “occludin”, “claudin”, “N-cadherin”, “β-catenin”, “vimentin”), “germ cell development” (including “spermatogonia proliferation” OR “spermatogonia self-renewal”, “spermatogonia differentiation”, “spermatocyte meiosis”, “spermiogenesis”, “sperm maturation”, “sperm release” OR “spermiation”), and “spermatogenesis”. Articles that were aimed at exploring the role of SC secretions in spermatogenesis were collected (Table 2). The final screening was related to “male infertility”, “male fertility diseases” OR “male reproductive diseases” where we found two notable diseases cryptorchidism and the Sertoli cell-only syndrome (SCOS). Hence, we subsequently searched for “cryptorchidism”, “the Sertoli cell only syndrome” OR “SCOS” to gain insight into their mechanisms. More papers were selected from these review articles and their reference lists.
Hormone-mediated PI3K/AKT signaling directly regulates Sertoli cell proliferation, differentiation and BTB junction protein expression
In the fetal cells of mice and rats, immature SCs proliferate to increase their number (Lucas et al., 2014b). However, during the prepubertal period, SC proliferation stops and they start to differentiate to a mature state. During this process, SCs establish the BTB and acquire the ability to sustain spermatogenesis (Sharpe et al., 2003). It has been documented that several hormones participate in SC proliferation, maturation, and BTB junction protein expression or a part of these processes. We have summarized these hormones below (Fig. 1).
Figure 1: Hormones-mediated PI3K/AKT signaling pathways in Sertoli cells (SCs). The functions of different hormones are marked with different colors. FSH activates PI3K/AKT to promote activities of LDH and aromatase, the expression of SOX9, SF1, GATA4, and AMH. However, it suppresses TFEB nuclear translocation and lysosomal genes to stimulate GDNF, SCF, and ABP secretion (green). After binding to AR, testosterone (T) activates PI3K/AKT and enhances the expression of Ki67, CK-18, FSHR, WT1, SOX9 and occludin, and ZO-1. HIF and c-myc are also involved in this process (red). All the three estrogen receptors activate the PI3K/AKT pathway (orange). E2-ESR1 activates Src and HB-EGF phosphorylation to activate PI3K/AKT, which promotes NF-κB nuclear translocation and enhances CCND1 expression. E2-ESR2 directly activates the PI3K/AKT pathway to promote CREB nuclear translocation and increase the expression of CDKN1B, GATA-1, and DMRT1. Further, GPER activates Src, MMP, and HB-EGF phosphorylation successively to activate the PI3K/AKT signaling, which promotes the expression of apoptosis proteins BCL2 and BAX. Additionally, IGF/insulin stimulates calcium uptake, amino acid and glucose transport along with lactate secretion via PI3K/AKT signaling. Furthermore, the downstream signaling of AKT mTOR-p70S6K-rpS6 increases MMP9 production, which downregulates the expression of occludin, claudin, and ZO-1 (purple). By activating PI3K/AKT, relaxin promotes NF-κB nuclear translocation to promote SC proliferation (blue). RA also promotes SC proliferation and SC metabolism through PI3K/AKT (brown). However, only TH inhibits PI3K/AKT signaling to cause the upregulation of p21Cip1, p27Kip1, AR and connexin 43 while inducing the downregulation of cyclin A2, D1, E1, PCNA, Skp2 and KRT-18 (pink).
Follicle-stimulating hormone (FSH) belongs to the glycoprotein hormone family (Kumar et al., 1997). It is a crucial hormone in the endocrine regulation of spermatogenesis (Themmen and Huhtaniemi, 2000). After binding to FSH, the follicle-stimulating hormone receptor (FSHR) is activated which stimulates the downstream signaling cascades in SCs (Walker and Cheng, 2005).
Several studies have proven that FSH is required for immature SC proliferation (Griswold et al., 1977; Orth, 1984; Kumar et al., 1997; Abel et al., 2000; Crépieux et al., 2001; Wreford et al., 2001; Hayes et al., 2001; Johnston et al., 2004). Additional studies suggest that the level of p-AKT significantly increases after treatment with FSH. Moreover, reports showed that concurrent treatment with the PI3K inhibitor, LY294002/wortmannin, alone or combined with the mTOR inhibitor rapamycin, significantly decreases FSH-stimulated p-AKT levels (Meroni et al., 2002; McDonald et al., 2006; Musnier et al., 2009; Riera et al., 2012; Xi et al., 2022) and FSH-stimulated [3H] thymidine incorporation (Riera et al., 2012). In another study, FSH was also found to suppress the cytoplasm-to-nucleus translocation of transcription factor EB (TFEB) via PI3K/AKT/mTORC1 in cultured goat SCs. This further lower lysosomal biogenesis, inhibits autophagy, promotes cell survival, and enhances the expression of ABP, SCF, and GDNF (Xi et al., 2022). Besides, FSH was also found to be related to lactate production, lactate dehydrogenase (LDH) activity, and glucose uptake in a PI3K/AKT-dependent manner to provide nutrients to SCs and GCs in another report (Meroni et al., 2002). Another study showed that FSH also regulates PI3K/AKT downstream target gene c-myc, an essential factor in the regulation of cellular proliferation and the SC cycle. After treating primary SCs with FSH, there was an increase of mRNA levels of c-myc (Lim and Hwang, 1995). Similarly, another regulator of cell growth, Hypoxia Inducible Factor (HIF), which is involved in the regulation of rat SC proliferation by FSH (Gorga et al., 2018) was also shown to be related to PI3K/AKT signaling during spermatogenesis (Wang et al., 2021). Another report found that while FSH stimulates aromatase activity and estradiol production, a hormone playing a key role in the proliferation of SCs (Wu et al., 2015; Yang et al., 2015), these stimulatory effects of FSH on aromatase and estradiol are blocked by inhibiting PI3K/AKT signaling (McDonald et al., 2006). The expression of anti-Müllerian hormone (AMH), another SC-produced hormone, is triggered by SOX9 in immature SCs, and is regulated by SF1, GATA, FSH, and other factors (Rey et al., 2003). It has been documented that the expression and translocation of some of these genes are regulated mainly through the cAMP/PKA pathway in the SC line SMAT1, which possibly also involves the role of other kinases in this process, such as PI3K/AKT (Lasala et al., 2011). AMH was also shown to increase the expression of an important regulator of spermatogenesis, SCF (ur Rehman et al., 2017).
Mature SCs cease to proliferate and begin to differentiate. They respond to FSH by stimulating cAMP production (Crépieux et al., 2001; Dupont et al., 2010; Nascimento et al., 2013; Nascimento et al., 2016; Bhattacharya et al., 2019; Bhattacharya et al., 2021) instead of PI3K/AKT. This is due to the inhibition of the PI3K/AKT pathway resulting from high level cAMP (Eskola et al., 1993; Nascimento et al., 2016) and enhanced PTEN activity (Dupont et al., 2010).
Testosterone is the major androgen in the testes, which is predominantly produced by Leydig cells and can pass through the plasma membrane to interact with the intracellular androgen receptor (AR) (Walker, 2021). In the testis, only Leydig cells, peritubular cells, and SCs express AR (Lyon et al., 1975). There are two types of AR signaling: the classical signaling pathway and the non-classical signaling pathway. It is known the PI3K/AKT signaling is involved in the non-classical signaling pathway of AR. Here, testosterone interacts with AR to activate the PI3K/AKT pathway directly by activating the PI3K subunit p85a. Phosphorylated AKT activates Src, a component of another non-classical AR signaling pathway type, and contributes to AR translocation from the cytoplasm to the plasma membrane (Deng et al., 2017).
Previous studies have reported that the androgen can regulate spermatogenesis and BTB junction proteins. This regulation can be the androgen alone (Meng et al., 2005; Zhang et al., 2005; Denolet et al., 2006; Wang et al., 2006; Kaitu’u-Lino et al., 2007; Yan et al., 2008; Willems et al., 2010; Chakraborty et al., 2014; Chojnacka et al., 2016b) by working with FSH synergistically (McLachlan et al., 1994; Haywood et al., 2003; Johnston et al., 2004; Abel et al., 2008; Bhattacharya et al., 2019). Other studies showed that several compounds have an impact on SC proliferation and BTB junction proteins through the AR/PI3K/AKT pathway. For example, Lycium barbarum polysaccharide (LBP) resists heat-stress-induced injury of SCs by increasing the expression of the androgen receptor and activates the AKT signaling pathway in rats. As a result, it restores the proliferation activity of SCs by resisting the induction of Ki67 expression. It reverses the dedifferentiation of SCs by decreasing CK-18 expression, while increasing the expression levels of junction proteins occludin and ZO-1 (Hu et al., 2021a). In another report, Wuzi Yanzong Pills (WYP) improved SC viability and proliferation, ameliorated dedifferentiation and the expression of junction proteins such as ZO-1 and occludin damaged by heat stress via AKT signaling (Hu et al., 2021b; Xu et al., 2021), which may result from T-level restoration (Xu et al., 2014; Hu et al., 2021b). Ouabain is an endogenous cardiotonic steroid (Cavalcante-Silva et al., 2017), and is known to participate in the non-classical AR pathway (Ni et al., 2020). Treatment of the SC line 93RS2 with ouabain activated c-Src/c-Raf/Erk1/2 signaling, which is radically triggered by testosterone (Konrad et al., 2011; Cavalcante-Silva et al., 2017; Rajamanickam et al., 2017). Moreover, another study showed that ouabain induces PI3K/AKT phosphorylation in primary rat SCs (Lucas et al., 2012), but it was not clear whether PI3K/AKT signaling also was triggered by testosterone. In addition, Testosterone treatment can increase c-Src phosphorylation. However, 2-hydroxyflutamide (HF), an anti-androgen compound, inhibits the increase of c-Src activity induced by testosterone in 20-day-old rat testes. At the same time, HF augments AKT phosphorylation by upregulating PTEN phosphorylation, indicating the activation of the PI3K/AKT pathway. This is probably because testosterone is unable to exert functions properly. Besides, HF also reduces the level of SC functional marker connexin 43, which results from the activated PI3K/AKT pathway (Chojnacka et al., 2016a). On the contrary, long-term exposure to phenanthrene (Phe), downregulates the GDNF/PI3K/AKT signaling pathway, reduces SC numbers, and inhibits the expression of SC markers such as FSHR, WT1, and Sox9. These observations may be related to the reduction of AR expression (Chen et al., 2021; Huang et al., 2021). In another study, treating TM4 cells (normal mouse testis Sertoli cell line) with just 20 μM of nonylphenol (NP) increased the p-AKT level (Liu et al., 2016). However, treating prepubertal SCs with 30 μM NP, triggers apoptosis related to oxidative stress, disturbs the PI3K/AKT/mTOR pathway and the functions of the hormone receptor and SC secretory function, inducing reproductive damage (Huang et al., 2016). These changes are possible because of the reduction of the androgen level or the androgen receptor (Huang et al., 2016; Jambor et al., 2016; Liu et al., 2016).
To summarize, androgen stimulates the proliferation of SCs and regulates BTB junction protein expression via the PI3K/AKT pathways. While previous research was focused on classical pathways and the EGFR/Src/Ras/CREB non-classical pathway, additional research is needed to elucidate the function of AR/PI3K/AKT signaling.
In young animals, estrogens are synthesized mainly by SCs, while in adult animals, Leydig and germ cells can also synthesize these hormones (Carreau et al., 2006; Carreau and Hess, 2010). The action of certain aromatase can transform testosterone into estrogen (Walker, 2021). This then functions in male reproduction and male fertility (Lazari et al., 2009). Estrogens perform their biological functions by interacting with the classical nuclear estrogen receptors (ESRs): ESR1 and ESR2 (also known as ERα and ERβ) and also the non-classical membrane receptor, a G protein-coupled estrogen receptor 1 (GPER, also known as GPR) (Aquila et al., 2004; Royer et al., 2012). All these three receptors have been shown to activate the PI3K/AKT pathway (Lucas et al., 2014b). Once bound to estrogen, ESR1, and GPER can activate Src and EGFR, which then activates PI3K/AKT signaling. However, ESR2 activates the PI3K/AKT pathway directly after binding to estrogen.
The main physiological estrogen for male germ cell survival is 17β-estradiol (E2) (Pentikäinen et al., 2000). Treatment of cultured immature boar Sertoli cell with E2 activates mTOR in a time dependent manner, whereas 10-DEBC (an inhibitor of AKT) treatment reduces E2-induced mTOR phosphorylation significantly, suggesting an activation of PI3K/AKT/mTOR signaling. Further, the mTOR inhibitor, rapamycin, reduces the mRNA levels of SKP2, CCND1, and CCNE1 mRNA and the protein levels of RB and EMI1 to suppress E2-induced SC proliferation (Yang et al., 2015).
Studies show that ESR1 stimulates SC proliferation by upregulating CCND1 expression (Royer et al., 2012; Lucas et al., 2014a; Macheroni et al., 2020). It is a vital gene of the cell cycle, and this effect depends on NF-κB activation (Lucas et al., 2014a). However, a study showed that E2 and DPN (the ESR2-selective agonist) increase the expression of CDKN1B, GATA-1, and DMRT1 in a PI3K and CREB-dependent manner (Lucas et al., 2014a). Similarly, E2 and G-1 (the GPER-selective agonist) rapidly activate PI3K/AKT and CREB, which may participate in the upregulation of anti-apoptotic proteins BCL2 and BCL2L2 (Royer et al., 2012). Further, both G-15 (a GPR30 antagonist) and PP2 (a Src inhibitor) inhibit E2-induced activation of PI3K, indicating both GPR30 and Src participate in E2-induced PI3K/AKT phosphorylation. Besides, the activation of PI3K/AKT also enhances Skp2 expression, which contributes to SC proliferation (Yang et al., 2017).
Some chemical treatments also confirm that estrogen acts through the PI3K/AKT signaling pathway. An example is zearalenone (ZEA) (Zheng et al., 2016; Wang et al., 2018a; Zheng et al., 2018; Rogowska et al., 2019), an exogenous compound that resembles the structure of natural estrogens in its chemical structure (Rogowska et al., 2019). It arrests the cell cycle in the G2/M phase to inhibit SC proliferation (Wang et al., 2018a) and triggers autophagy via inhibition of the PI3K/AKT/mTOR pathway (Wang et al., 2018a; Zheng et al., 2018). Further, studies have shown that the autophagy stimulated by ZEA leads to damage to cytoskeletal structures (F-actin, α-tubulin, and the nucleus structure) (Zheng et al., 2016, 2018). Besides the damage to the cytoskeletal structure, one of these studies also shows that ZEA treatment can affect the secretion of specific proteins of SCs like ABP, transferrin, vimentin, N-cadherin, and FSHR (Zheng et al., 2016). Another structural analog of estradiol, Bisphenol-A(BPA) (Chianese et al., 2018), an endocrine-disrupting chemical, can also compete with E2 for ESR. BPA treatment of cultured SCs increases the protein level of PTEN while decreasing the levels of p-AKT and procaspase-3, suggesting that the PTEN/AKT pathway is involved in the apoptotic effects of BPA on SCs (Wang et al., 2015). Another molecule, diosgenin induces ESR1 and ESR2 translocation from the nucleus to the plasma membrane, which is an SRC-dependent process. This process activates ERK/AKT signaling and induces the transcriptional activity of ESR, which subsequently directly regulates the cell cycle and apoptosis proteins, such as cyclin D and Bcl-2, leading to final cell proliferation (Wu et al., 2015). Treating TM4 cells with just 20 μM of nonylphenol(NP) increased the p-AKT level (Liu et al., 2016). However, treating prepubertal SCs with 30 μM NP, triggers apoptosis related to oxidative stress, disturbs the PI3K/AKT/mTOR pathway and induces reproductive damage (Huang et al., 2016). These changes are attributed to the targeting of estrogen receptors (Huang et al., 2016; Jambor et al., 2016; Liu et al., 2016).
Insulin family of growth factors
The insulin family of growth factors includes insulin, insulin-like growth factor I (IGF-1), insulin-like growth factor II (IGF-2), and relaxin. Though they are all structurally small polypeptides, they are key regulators of cell growth, cell metabolism, and male reproduction.
Insulin and insulin-like growth factors
Insulin and IGF1 exert biological functions by activating their receptors: the insulin receptor (IR, also known as INSR), and the IGF-1 receptor (IGF-1R), respectively which are tyrosine kinases (Meroni et al., 2019). Notably, insulin could activate both INSR and IGFR (Escott et al., 2014). This makes it sometimes difficult to affirm the effects induced whether by insulin or IGF-I when both receptors exist.
Both insulin and IGFs have been shown to regulate the PI3K/AKT pathway in SCs. For example, in prepubertal rat SCs, unlike FSH, insulin stimulation leads to an increase in PTEN phosphorylation, the PI3K major down-regulator. This activates the PI3K/AKT pathway to promote SC proliferation and resist the shift of SCs from proliferation to differentiation states induced by FSH (Dupont et al., 2010). Similarly, IGFs also promote immature SC proliferation through the IGF/PTEN/PI3K pathway (Neirijnck et al., 2019). Besides, they are also reported to regulate aspects of SC metabolism, such as calcium uptake, amino acid and glucose transport, and lactate secretion (Oliveira et al., 2012; Escott et al., 2013; Escott et al., 2014; Faure et al., 2016; Gan et al., 2022). These are all important in SC survival through the PI3K/AKT pathway (Escott et al., 2013; Gan et al., 2022). The downstream factor p-rpS6 of mTOR was shown to disrupt IGF-1/insulin-AKT signaling that leads to an increase of MMP-9 expression. This subsequently perturbs the BTB by downregulating tight junction proteins such as claudin-11, occludin and ZO-1 (Mok et al., 2014), suggesting a protective effect of IGF-1/insulin-AKT signaling on the BTB.
IGF acts synergistically with FSH to activate AKT (Froment et al., 2007; Musnier et al., 2009). In addition, it was shown that IGF1 is required for the activation of AKT by FSH in immature SCs. For example, pre-treatment of these cells with NVP-AEW541, an IGF1R inhibitor, inhibits FSH-dependent AKT308 phosphorylation (Cannarella et al., 2019). In SCs lacking INSR and IGF1R, The expression of the fshr gene and AKT activation are both decreased (Pitetti et al., 2013). FSH was shown to stimulate immature SCs to secrete IGF1 and amplify the IGF-mediated PI3K/AKT signaling pathway in immature SCs (Khan et al., 2002; Pitetti et al., 2013). However, another report showed that FSH activates PI3K/AKT signaling in 20-day-old SCs independently of IGF-1 (Meroni et al., 2004), implicating that the interaction between IGF and FSH depends on the developmental phase of SCs.
Interestingly, a study showed that knocking out follistatin-like 3 (FSTL3), a glycoprotein that binds and inhibits the action of TGFβ ligands increases SC numbers, which might arise from the increased IGF1/AKT signaling. This result demonstrates a cross-talk between TGF ligands and IGF/AKT signaling for cell survival (Oldknow et al., 2013).
Relaxin (RLN/RLX) is another member of the insulin family of growth factors, the structure of which is similar to that of insulin. Relaxin binds to the relaxin family of peptide receptors (RXFP1 and RXFP2) (Hsu et al., 2002), which belong to the G-protein coupled receptor (GPCR) family, and stimulate several crucial signaling pathways (Bathgate et al., 2013).
Relaxin has been shown to stimulate SC proliferation (Cardoso et al., 2010; Nascimento et al., 2012; Pimenta et al., 2013, 2015; Nascimento et al., 2016). Further, relaxin increases the incorporation of [3H]-thymidine (Cardoso et al., 2010; Nascimento et al., 2012, 2016) and PCNA levels in SCs (Nascimento et al., 2012). Other studies show that relaxin stimulates SC proliferation due to the activation of the PI3K/AKT pathways (Nascimento et al., 2013). In SCs isolated from 15-day-old rats at a stage that is close to the transition between cell proliferation and cell differentiation, relaxin and FSH affect the same signaling pathways in opposite directions. While relaxin increases AKT phosphorylation, inhibits basal cAMP production, and impacts NF-κB nuclear translocation, FSH inhibits AKT phosphorylation and strongly increases cAMP production as well as CREB phosphorylation (Nascimento et al., 2013, 2016). To summarize, relaxin counteracts FSH signaling but does not block its pro-differentiation function triggered by FSH in 15-day-old rats.
Thyroid hormones (THs) are critical regulators of cell growth, cell development, and cell metabolism in almost all tissues (Oetting and Yen, 2007; Cheng et al., 2010; Brent, 2012). The thyroid gland mainly secretes two types of THs, levothyroxine (T4) and triiodothyronine (T3), where the latter is identified as the more biologically active form (Sinha and Yen, 2016). The expression of the thyroid hormone receptor (TR) within the seminiferous epithelium only occurs in SCs, and the Tαreceptor is its only form expressed (Jannini et al., 2000). There are two main pathways by which THs exert their functions, namely genomic and non-genomic. The former refers to the influences of gene expression, while the latter refers to the regulation of the intracellular signaling pathways, including PI3K/AKT (Geist et al., 2021).
T3 has been shown to inhibit SC proliferation via the PI3K/AKT signaling pathway (Sun et al., 2015; Wang et al., 2019). This is accomplished by downregulating cyclinA2, cyclinD1, cyclinE1, PCNA, and Skp2 (Holsberger and Cooke, 2005; Sun et al., 2015; Wang et al., 2019) and upregulating the cyclin-dependent kinase inhibitors (CDKI) p21Cip1, and p27Kip1 (Buzzard et al., 2003; Holsberger et al., 2003, 2005; Holsberger and Cooke, 2005; Sun et al., 2015; Wang et al., 2019). Therefore, T3 and the above-mentioned hormones have opposite effects on SC proliferation even though they can both affect the PI3K/AKT signaling.
It is worth noting that Cx43 could be an intermediate target for T3 inhibition of neonatal SC proliferation (Gilleron et al., 2006), which has been shown to be an inhibitor of Skp2 in a previous study (Zhang et al., 2003). This offers a new thought that T3 is capable to upregulate connexin 43 expression, one of the main components of the BTB under the control of the PI3K/AKT signaling pathway. Further, T3 also possibly promotes SC differentiation via PI3K/AKT signaling by upregulating AR and down-regulating KRT-18 (Wang et al., 2019).
Hormone-mediated PI3K/AKT signaling in Sertoli cells indirectly regulates spermatogenesis
The hormones summarized above have indirect effects on spermatogenesis by stimulating SC secretion through PI3K/AKT signaling. These secretions mainly include cytokines and hormones, like SCF, GDNF, and AMH and transport proteins, like ABP, and transferrin. They also include cell junction proteins, like ZO-1, occludin, claudin, connexin 43, β-catenin, and N-cadherin along with cellular metabolites, like amino acid, glucose, LDH, and HIF. Some SC secretions have been shown to play important roles in regulating spermatogenesis (Fig. 2).
Figure 2: The effects of Sertoli cell secretions produced by hormone-mediated PI3K/AKT signaling on spermatogenesis. SCs (the pink cells) secrete lactate to provide energy to germ cells and secrete many kinds of proteins essential for spermatogenesis. GDNF promotes spermatogonia self-renewal after binding to its receptor GFRA1, while SCF binds to KIT to promote differentiated spermatogonia proliferation. Connexin 43 (Cx43) regulates the expression of Sohlh1, Lhx8, and Sox3 in spermatogonia (the blue cell). Junction proteins allow preleptotene spermatocytes to go through the BTB structure while differentiating into leptotene spermatocytes. Subsequently, ABP assists AR to stimulate spermatocyte secretion. Transferrin binds to TFR to promote the meiosis process. Cx43 regulates the expression of Ovol1, Dazl, Stra8, Sox3, Sycp2, Piwi2, and Ddx25 in spermatocytes (the green cell). ABP works with androgen to regulate sperm-fertilizing ability and reduce nuclear chromatin condensation proteins during spermiogenesis. Further, Cx43 regulates the expression of the sperm genes Spag6, Zpbp2, Taf7l, and Tex11 (the purple cell).
Spermatogonia proliferation and differentiation
Spermatogenesis originates from the self-renewal of SSCs (Lord et al., 2018). They then differentiate into types A1–A4, intermediate (Int), and B spermatogonia successively (Li et al., 2019). This process is regulated by several cytokines secreted by SCs in a paracrine manner.
GDNF, produced by SCs, and its receptor GFRA1 is expressed on the surface of SSCs. The GDNF-GFRA1 complex interacts with RET tyrosine kinase, which is present on the surface of undifferentiated type A spermatogonia (Naughton et al., 2006; He et al., 2007). This promotes spermatogonial self-renewal in mice (Meng et al., 2000; Kubota et al., 2004; Hofmann et al., 2005; Wang et al., 2014).
SCF, specifically produced by SCs acts through its receptor KIT, which is encoded by c-kit. The stem cell factor (SCF)/KIT pathway is known to regulate type A spermatogonia proliferation and differentiation (Blume-Jensen et al., 2000; Feng et al., 2000; Ohta et al., 2000; Feng et al., 2002). Its effects on spermatogonia proliferation are induced by type A spermatogonial DNA synthesis (Feng et al., 2000). However, studies have shown that the SCF/KIT signaling also appears to be necessary for maintaining the proliferation of differentiated spermatogonia but not for the initiation of spermatogonial cell differentiation (Yoshinaga et al., 1991; Tajima et al., 1994; Hakovirta et al., 1999; Ohta et al., 2000, 2003). To elaborate, undifferentiated type A spermatogonia or SSCs are independent of KIT. Interestingly, a review states that KIT also regulates the effects of both bone morphogenetic protein 4 (BMP4) and retinoic acid (RA) on spermatogonial differentiation including SSCs (Hai et al., 2014). In cryptorchid patients, SCF and RA promote human SSCs differentiating into haploid spermatids, which can be a potential approach for treating this disease (Yang et al., 2014).
In SC specific connexin 43 knockout (SCCx43KO) mice, the germ cell numbers are significantly reduced, causing Sertoli-cell-only (SCO) syndrome, and spermatogenesis is arrested at the level of undifferentiated spermatogonia (Steger et al., 1999; Brehm et al., 2007; Rode et al., 2018). This suggests a defect of spermatogonia differentiation, which may be associated with the gene expression of Sohlh1, Lhx8, and Sox3 (Giese et al., 2012).
SCs play an active role in the transfer of spermatocytes to the adluminal compartment mainly because of the BTB structure (Russell, 1977).
The expression of the major components of the BTB, ZO-1, occludin, and claudin, plays an important role in the migration of spermatocytes through the BTB. Another cell junction protein, connexin 43 restores meiosis and the BTB structure under the situation of aspermatogenesis and barrier disruption caused by toxicant, but it fails to support round spermatids to enter spermiogenesis (Li et al., 2016). Further, in SCCx43KO the gene expression of Ovol1, Dazl, Stra8, Sox3, Sycp2, Piwil2, and Ddx25 are changed. These play pivotal roles in the initiation or progression of meiosis and controlling apoptosis in spermatocytes (Giese et al., 2012). Additionally, Cx43 is also required for the mitosis-meiosis switch (Hilbold et al., 2020).
Spermatogenesis is also a transferrin-dependent process, which is primarily secreted by SCs and can transport iron into the seminiferous tubule (Wauben-Penris et al., 1986; Morales et al., 1987). Transferrin receptors (TFRC) are localized mainly in spermatocytes and early spermatids (Sylvester and Griswold, 1984; Forti et al., 1989). Further, TFRC is essential for spermatocyte meiosis progression, particularly for DNA damage repair and chromosome synapsis (Gao et al., 2021).
Another SC secretion is androgen-binding protein (ABP), the receptor of which is expressed by spermatocytes (Gerard, 1995). It is also shown to increase the secretion of specific spermatocyte proteins individually (Sharpe et al., 1992).
Round spermatids differentiate into spermatozoa, which then are released into the tubular lumen at spermiation (Qian et al., 2014). SCs play an essential role during sperm maturation and release, mainly by forming Sertoli cell-spermatid adhesion. In the initial stages, round spermatids are linked to SCs by desmosomes before spermiogenesis occurs. Round spermatids then begin to elongate and apical ectoplasmic specialization (ES) replaces desmosome anchors gradually. This ES disassembles before sperm release (Wang et al., 2022). Additionally, cytokines secreted by SCs (such as EGF), also regulate sperm maturation (Naz et al., 1994).
SC-expressed Cx43 affects sperm development. For example, SCCx43KO changes the expression of Spag6, Zpbp2, Taf7l, and Tex11 which are essential genes for sperm count, sperm formation, and maturation (Giese et al., 2012).
Sperm production and maturation are also androgen-dependent processes. ABP works with androgen to regulate the sperm-fertilizing ability (Anthony et al., 1984b; Hermo et al., 1998). The hypophysectomized rat model, which maintains a low testosterone level with pregnenolone, also verifies this result (Anthony et al., 1984a). Further, ABP mRNA levels can be upregulated by estradiol, and this increase could upregulate the expression of transition protein 1 (TP1) involved in nuclear chromatin condensation in rodent spermatids in vitro (Caron et al., 2001; Kierszenbaum, 2001; Della-Maria et al., 2002; Aleem et al., 2006).
Hormones play important roles in the process of reproduction and are attracting increased attention from researchers. Studies show male infertility diseases, like cryptorchidism (Rodprasert et al., 2019; Aldahhan et al., 2021) and the Sertoli cell-only syndrome (SCOS) (Jain and Halder, 2012) usually document disrupted hormone levels. In cryptorchid testes, the FSH and androgen receptors of SCs are dramatically reduced (Agoulnik et al., 2012). Most SCOS testes have low inhibin B but high FSH levels (Jain and Halder, 2012). Hence, detecting the relevant hormone level change can be used as a means of diagnosis, and regulation of hormone levels. This offers a potentially feasible treatment method for male infertility diseases.
Many studies show that infertility in men is mainly (more than 90%) caused by low sperm counts, poor sperm quality, or both (Leaver, 2016). This indicates that the disruption of spermatogenesis is the major cause of male infertility. We have summarized that spermatogenesis is regulated by hormone-mediated signaling pathways, including PI3K/AKT, and its subsequent effects on SC secretions. Therefore, regulating the PI3K/AKT signaling in SCs (such as using their activators/inhibitors or changing the levels of related hormones) and SC secretions is also a good idea for regulating spermatogenesis and treating male infertility. For example, in cryptorchid testes, the ability of SCs to secrete inhibin B and AMH is affected (Cortes et al., 2016). Further, spermatogenesis is arrested at the undifferentiated type A spermatogonia stage and germ cell apoptosis increases significantly (Agoulnik et al., 2012). However, the SC secretions, SCF and RA promote the differentiation of human SSCs into haploid spermatids in cryptorchid patients (Yang et al., 2014). SCOS, also a major cause of male infertility is characterized by a lack of germ cells, and the seminiferous tubules contain only SCs (Talati and Sheikh, 1991). Most SCs in SCOS can neither organize cell-cell junctions nor stimulate SSCs to produce normal levels of growth factors, such as GDNF, FGF8, and BMP4 (Paduch et al., 2019). The failure of SCs to form normal CX43 junction protein is associated with impaired spermatogenesis in SCO testis (Defamie et al., 2003), including spermatogonia differentiation, spermatocyte meiosis, and sperm maturation (Giese et al., 2012). Additionally, the downregulation of GDNF is associated with reduced spermatogonial self-renewal (Meng et al., 2000; Kubota et al., 2004; Hofmann et al., 2005; Wang et al., 2014). Hence, detecting the level of SC secretions such as inhibin B, AMH, SCF, RA, GDNF, FGF8, BMP4, and CX43 can be used as a means of diagnosis. Further, the regulation of their levels may be a potential treatment method of male infertility diseases.
Therefore, the regulation of hormone levels, the PI3K/AKT signaling, SC secretions, and finally spermatogenesis may be promising methods for treating male infertility, like cryptorchidism and SCOS.
Conclusion and Future Perspectives
We discussed several hormones like FSH, androgen, estrogen, insulin/IGF, relaxin, thyroid hormone, and RA, which all directly mediate the PI3K/AKT signaling in SCs. In addition to the hormones we mentioned, studies show that prostaglandin D2 (PGD2) (Rossi et al., 2016) and leptin (Wang et al., 2018b) also directly stimulate PI3K/AKT signaling in SCs. However, this still requires further exploration. The cross-talk among these hormones in regulating the PI3K/AKT signaling is an interesting topic for future study.
Further, the effects of SC secretions under the control of hormone-mediated PI3K/AKT signaling on spermatogenesis have also been discussed. These include the effects on spermatogonia proliferation and differentiation, spermatocyte meiosis, sperm maturation, and release. The roles of other SC secretions during spermatogenesis however remain unclear.
Finally, we discussed two diseases-cryptorchidism and SCOS from the perspective of hormone regulation and spermatogenesis, hoping to provide clinical hints for their treatment. The deeper and broader effects of spermatogenesis and SC secretions on these diseases require further investigation (Fig. 3).
Figure 3: Schematic representation of the hormone-PI3K/AKT-SC secretions-spermatogenesis pathways. We pose a few questions here. Do other hormones also participate in mediating PI3K/AKT signaling? How do these hormones cooperate? What other roles may SC secretions have in regulating spermatogenesis? From the perspective of spermatogenesis, what new ideas can be provided for male fertility disease prevention and treatment?
Acknowledgement: The authors thank all members of the Sperm Laboratory of Zhejiang University for their help. We are grateful for the experimental platform provided by Zhejiang University. Our appreciation also goes to the College Students Creative Research Training Center, National Biological Demonstration Experimental Center, Zhejiang University.
Funding Statement: This work was supported in part by the National Natural Science Foundation of China (Nos. 32270555 and 32072954).
Author Contributions: The authors confirm their contribution to the paper as follows: Zhao conceived, wrote, revised the manuscript, and prepared all the figures. Yang was constructed the outline of the manuscript, participated in the revision, and provided the funds for this research.
Ethics Approval: Not applicable.
Conflicts of Interest: The authors declare that they have no conflicts of interest to report regarding the present study.
References
Abel MH, Baker PJ, Charlton HM, Monteiro A, Verhoeven G, de Gendt K, Guillou F, O’Shaughnessy PJ (2008). Spermatogenesis and Sertoli cell activity in mice lacking Sertoli cell receptors for follicle-stimulating hormone and androgen. Endocrinology 149: 3279–3285. https://doi.org/10.1210/en.2008-0086 [Google Scholar] [PubMed] [CrossRef]
Abel MH, Wootton AN, Wilkins V, Huhtaniemi I, Knight PG, Charlton HM (2000). The effect of a null mutation in the follicle-stimulating hormone receptor gene on mouse reproduction. Endocrinology 141: 1795–1803. https://doi.org/10.1210/endo.141.5.7456 [Google Scholar] [PubMed] [CrossRef]
Agoulnik AI, Huang Z, Ferguson L (2012). Germline development-chapter 11: Spermatogenesis in cryptorchidism. Methods in Molecular Biology 825: 127–147. https://doi.org/10.1007/978-1-61779-436-0 [Google Scholar] [CrossRef]
Aldahhan RA, Stanton PG, Ludlow H, de Kretser DM, Hedger MP (2021). Experimental cryptorchidism causes chronic inflammation and a progressive decline in Sertoli cell and Leydig cell function in the adult rat testis. Reproductive Sciences 28: 2916–2928. https://doi.org/10.1007/s43032-021-00616-0 [Google Scholar] [PubMed] [CrossRef]
Aleem M, Padwal V, Choudhari J, Balasinor N, Parte P, Gill-Sharma MK (2006). Estradiol affects androgen-binding protein expression and fertilizing ability of spermatozoa in adult male rats. Molecular and Cellular Endocrinology 253: 1–13. https://doi.org/10.1016/j.mce.2006.01.014 [Google Scholar] [PubMed] [CrossRef]
Anthony CT, Danzo BJ, Orgebin-Crist MC (1984a). Investigations on the relationship between sperm fertilizing ability and Androgen-Binding Protein in the hypophysectomized, pregnenoloneenolone-injected rat. Endocrinology 114: 1419–1425. https://doi.org/10.1210/endo-114-4-1419 [Google Scholar] [PubMed] [CrossRef]
Anthony CT, Danzo BJ, Orgebin-Crist MC (1984b). Investigations on the relationship between sperm fertilizing ability and Androgen-Binding Protein in the restricted rat. Endocrinology 114: 1413–1418. https://doi.org/10.1210/endo-114-4-1413 [Google Scholar] [PubMed] [CrossRef]
Aquila S, Sisci D, Gentile M, Middea E, Catalano S, Carpino A, Rago V, Ando S (2004). Estrogen receptor (ER)α and ERβ are both expressed in human ejaculated spermatozoa: Evidence of their direct interaction with phosphatidylinositol-3-OH kinase/Akt pathway. The Journal of Clinical Endocrinology & Metabolism 89: 1443–1451. https://doi.org/10.1210/jc.2003-031681 [Google Scholar] [PubMed] [CrossRef]
Bathgate RAD, Halls ML, van der Westhuizen ET, Callander GE, Kocan M, Summers RJ (2013). Relaxin family peptides and their receptors. Physiological Reviews 93: 405–480. https://doi.org/10.1152/physrev.00001.2012 [Google Scholar] [PubMed] [CrossRef]
Bhattacharya I, Basu S, Pradhan BS, Sarkar H, Nagarajan P, Majumdar SS (2019). Testosterone augments FSH signaling by upregulating the expression and activity of FSH-Receptor in Pubertal Primate Sertoli cells. Molecular and Cellular Endocrinology 482: 70–80. https://doi.org/10.1016/j.mce.2018.12.012 [Google Scholar] [PubMed] [CrossRef]
Bhattacharya I, Sharma SS, Sarkar H, Gupta A, Pradhan BS, Majumdar SS (2021). FSH mediated cAMP signalling upregulates the expression of Gα subunits in pubertal rat Sertoli cells. Biochemical and Biophysical Research Communications 569: 100–105. https://doi.org/10.1016/j.bbrc.2021.06.094 [Google Scholar] [PubMed] [CrossRef]
Blume-Jensen P, Jiang G, Hyman R, Lee KF, O’Gorman S, Hunter T (2000). Kit/stem cell factor receptor-induced activation of phosphatidylinositol 3′-kinase is essential for male fertility. Nature Genetics 24: 157–162. https://doi.org/10.1038/72814 [Google Scholar] [PubMed] [CrossRef]
Brehm R, Zeiler M, Rüttinger C, Herde K, Kibschull M et al. (2007). A Sertoli cell-specific knockout of connexin43 prevents initiation of spermatogenesis. The American Journal of Pathology 171: 19–31. https://doi.org/10.2353/ajpath.2007.061171 [Google Scholar] [PubMed] [CrossRef]
Brent GA (2012). Mechanisms of thyroid hormone action. The Journal of Clinical Investigation 122: 3035–3043. https://doi.org/10.1172/JCI60047 [Google Scholar] [PubMed] [CrossRef]
Buzzard JJ, Wreford NG, Morrison JR (2003). Thyroid hormone, retinoic acid, and testosterone suppress proliferation and induce markers of differentiation in cultured rat Sertoli cells. Endocrinology 144: 3722–3731. https://doi.org/10.1210/en.2003-0379 [Google Scholar] [PubMed] [CrossRef]
Cannarella R, Arato I, Condorelli RA, Luca G, Barbagallo F et al. (2019). The IGF1 receptor is involved in follicle-stimulating hormone signaling in porcine neonatal Sertoli cells. Journal of Clinical Medicine 8: 577. https://doi.org/10.3390/jcm8050577 [Google Scholar] [PubMed] [CrossRef]
Cardoso LC, Nascimento AR, Royer C, Porto CS, Lazari MFM (2010). Locally produced relaxin may affect testis and vas deferens function in rats. Reproduction 139: 185–196. https://doi.org/10.1530/REP-09-0146 [Google Scholar] [PubMed] [CrossRef]
Caron N, Veilleux S, Boissonneault G (2001). Stimulation of DNA repair by the spermatidal TP1 protein. Molecular Reproduction and Development 58: 437–443. https://doi.org/10.1002/1098-2795(20010401)58:4<437::AID-MRD12>3.0.CO;2-Q [Google Scholar] [CrossRef]
Carreau S, Delalande C, Silandre D, Bourguiba S, Lambard S (2006). Aromatase and estrogen receptors in male reproduction. Molecular and Cellular Endocrinology 246: 65–68. https://doi.org/10.1016/j.mce.2005.11.021 [Google Scholar] [PubMed] [CrossRef]
Carreau S, Hess RA (2010). Oestrogens and spermatogenesis. Philosophical Transactionsof the Royal Society B: Biology Sciences 365: 1517–1535. https://doi.org/10.1098/rstb.2009.0235 [Google Scholar] [PubMed] [CrossRef]
Cavalcante-Silva LHA, Lima EA, Carvalho DCM, de Sales-Neto JM, Alves AKA, Galvao JGFM, da Silva JSF, Rodrigues-Mascarenhas S (2017). Much more than a cardiotonic steroid: Modulation of inflammation by ouabain. Frontiers in Physiology 8: 895. https://doi.org/10.3389/fphys.2017.00895 [Google Scholar] [PubMed] [CrossRef]
Chakraborty P, Buaas FW, Sharma M, Smith BE, Greenlee AR, Eacker SM, Braun RE (2014). Androgen-dependent Sertoli cell tight junction remodeling is mediated by multiple tight junction components. Molecular Endocrinology 28: 1055–1072. https://doi.org/10.1210/me.2013-1134 [Google Scholar] [PubMed] [CrossRef]
Chen KQ, Wei BH, Hao SL, Yang WX (2022). The PI3K/AKT signaling pathway: How does it regulate development of Sertoli cells and spermatogenic cells? Histology and histopathology 37: 621–636. https://doi.org/10.14670/HH-18-457 [Google Scholar] [PubMed] [CrossRef]
Chen Y, Zhang Y, Yu Z, Guan Y, Chen R, Wang C (2021). Early-life phenanthrene exposure inhibits reproductive ability in adult zebrafish and the mechanism of action. Chemosphere 272: 129635. https://doi.org/10.1016/j.chemosphere.2021.129635 [Google Scholar] [PubMed] [CrossRef]
Cheng SY, Leonard JL, Davis PJ (2010). Molecular aspects of thyroid hormone actions. Endocrine Reviews 31: 139–170. https://doi.org/10.1210/er.2009-0007 [Google Scholar] [PubMed] [CrossRef]
Chianese R, Troisi J, Richards S, Scafuro M, Fasano S, Guida M, Pierantoni R, Meccariello R (2018). Bisphenol A in reproduction: Epigenetic effects. Current Medicinal Chemistry 25: 748–770. https://doi.org/10.2174/0929867324666171009121001 [Google Scholar] [PubMed] [CrossRef]
Chojnacka K, Hejmej A, Zarzycka M, Tworzydlo W, Bilinski S, Pardyak L, Kaminska A, Bilinska B (2016b). Flutamide induces alterations in the cell-cell junction ultrastructure and reduces the expression of Cx43 at the blood-testis barrier with no disturbance in the rat seminiferous tubule morphology. Reproductive Biology and Endocrinology 14: 14. https://doi.org/10.1186/s12958-016-0144-2 [Google Scholar] [PubMed] [CrossRef]
Chojnacka K, Zarzycka M, Hejmej A, Mruk DD, Gorowska E, Kotula-Balak M, Klimek M, Bilinska B (2016a). Hydroxyflutamide affects connexin 43 via the activation of PI3K/Akt-dependent pathway but has no effect on the crosstalk between PI3K/Akt and ERK1/2 pathways at the Raf-1 kinase level in primary rat Sertoli cells. Toxicology in Vitro 31: 146–157. https://doi.org/10.1016/j.tiv.2015.09.027 [Google Scholar] [PubMed] [CrossRef]
Cortes D, Clasen-Linde E, Hutson JM, Li R, Thorup J (2016). The Sertoli cell hormones inhibin-B and anti müllerian hormone have different patterns of secretion in prepubertal cryptorchid boys. Journal of Pediatric Surgery 51: 475–480. https://doi.org/10.1016/j.jpedsurg.2015.08.059 [Google Scholar] [PubMed] [CrossRef]
Crépieux P, Marion S, Martinat N, Fafeur V, Vern YL, Kerboeuf D, Guillou F, Reiter E (2001). The ERK-dependent signalling is stage-specifically modulated by FSH, during primary Sertoli cell maturation. Oncogene 20: 4696–4709. https://doi.org/10.1038/sj.onc.1204632 [Google Scholar] [PubMed] [CrossRef]
de Kretser DM, Loveland KLL, Meinhardt A, Simorangkir D, Wreford N (1998). Spermatogenesis. Human Reproduction 13: 1–8. https://doi.org/10.1093/humrep/13.suppl_1.1 [Google Scholar] [PubMed] [CrossRef]
Defamie N, Berthaut I, Mograbi B, Chevallier D, Dadoune JP, Fenichel P, Segretain D, Pointis G (2003). Impaired gap junction connexin43 in Sertoli cells of patients with secretory azoospermia: A marker of undifferentiated Sertoli cells. Laboratory Investigation 83: 449–456. https://doi.org/10.1097/01.LAB.0000059928.82702.6D [Google Scholar] [PubMed] [CrossRef]
Della-Maria J, Gerard A, Franck P, Gerard H (2002). Effects of androgen-binding protein (ABP) on spermatid Tnp1 gene expression in vitro. Molecular and Cellular Endocrinology 198: 131–141. https://doi.org/10.1016/S0303-7207(02)00376-3 [Google Scholar] [PubMed] [CrossRef]
Deng Q, Zhang Z, Wu Y, Yu WY, Zhang J, Jiang ZM, Zhang Y, Liang H, Gui YT (2017). Non-genomic action of androgens is mediated by rapid phosphorylation and regulation of androgen receptor trafficking. Cellular Physiology and Biochemistry 43: 223–236. https://doi.org/10.1159/000480343 [Google Scholar] [PubMed] [CrossRef]
Denolet E, De Gendt K, Allemeersch J, Engelen K, Marchal K et al. (2006). The effect of a Sertoli cell-selective knockout of the androgen receptor on testicular gene expression in prepubertal mice. Molecular Endocrinology 20: 321–334. https://doi.org/10.1210/me.2005-0113 [Google Scholar] [PubMed] [CrossRef]
Dupont J, Musnier A, Decourtye J, Boulo T, Lécureuil C et al. (2010). FSH-stimulated PTEN activity accounts for the lack of FSH mitogenic effect in prepubertal rat Sertoli cells. Molecular and Cellular Endocrinology 315: 271–276. https://doi.org/10.1016/j.mce.2009.09.016 [Google Scholar] [PubMed] [CrossRef]
Dym M, Fawcett DM (1970). The blood-testis barrier in the rat and the physiological compartmentation of the seminiferous epithelium. Biology of Reproduction 3: 308–326. https://doi.org/10.1093/biolreprod/3.3.308 [Google Scholar] [PubMed] [CrossRef]
Ersahin T, Tuncbag N, Cetin-Atalay R (2015). The PI3K/AKT/mTOR interactive pathway. Molecular Biosystems 11: 1946–1954. https://doi.org/10.1039/C5MB00101C [Google Scholar] [PubMed] [CrossRef]
Escott GM, de Castro AL, Jacobus AP, Loss ES (2014). Insulin and IGF-I actions on IGF-I receptor in seminiferous tubules from immature rats. Biochimica et Biophysica Acta 1838: 1332–1337. https://doi.org/10.1016/j.bbamem.2014.02.002 [Google Scholar] [PubMed] [CrossRef]
Escott GM, Jacobus AP, Loss ES (2013). PI3K-dependent actions of insulin and IGF-I on seminiferous tubules from immature rats. Pflügers Archiv-European Journal of Physiology 465: 1497–1505. https://doi.org/10.1007/s00424-013-1287-z [Google Scholar] [PubMed] [CrossRef]
Eskola V, Nikula H, Huhtaniemi I (1993). Age-related variation of follicle-stimulating hormone-stimulated cAMP production, protein kinase C activity and their interactions in the rat testis. Molecular and Cellular Endocrinology 93: 143–148. https://doi.org/10.1016/0303-7207(93)90117-3 [Google Scholar] [PubMed] [CrossRef]
Faure M, Guibert E, Alves S, Pain B, Rame C, Dupont J, Brillard JP, Froment P (2016). The insulin sensitiser metformin regulates chicken Sertoli and germ cell populations. Reproduction 151: 527–538. https://doi.org/10.1530/REP-15-0565 [Google Scholar] [PubMed] [CrossRef]
Feng LX, Chen Y, Dettin L, Pera RAR, Herr JC, Goldberg E, Dym M (2002). Generation and in vitro differentiation of a spermatogonial cell line. Science 297: 392–395. https://doi.org/10.1126/science.1073162 [Google Scholar] [PubMed] [CrossRef]
Feng LX, Ravindranath N, Dym M (2000). Stem cell factor/c-kit up-regulates cyclin D3 and promotes cell cycle progression via the phosphoinositide 3-kinase/p70 S6 kinase pathway in spermatogonia. The Journal of Biological Chemistry 275: 25572–25576. https://doi.org/10.1074/jbc.M002218200 [Google Scholar] [PubMed] [CrossRef]
Forti G, Barni T, Vannelli BG, Balboni GC, Orlando C, Serio M (1989). Sertoli cell proteins in the human seminiferous tubule. Journal of Steroid Biochemistry 32: 135–144. https://doi.org/10.1016/0022-4731(89)90154-4 [Google Scholar] [PubMed] [CrossRef]
Froment P, Vigier M, Negre D, Fontaine I, Beghelli J, Cosset FL, Holzenberger M, Durand P (2007). Inactivation of the IGF-I receptor gene in primary Sertoli cells highlights the autocrine effects of IGF-I. Journal of Endocrinology 194: 557–568. https://doi.org/10.1677/JOE-07-0258 [Google Scholar] [PubMed] [CrossRef]
Gan L, Huang S, Hu Y, Zhang JJ, Wang XZ (2022). Heat treatment reduced the expression of miR-7-5p to facilitate insulin-stimulated lactate secretion by targeting IRS2 in boar Sertoli cells. Theriogenology 180: 161–170. https://doi.org/10.1016/j.theriogenology.2021.12.029 [Google Scholar] [PubMed] [CrossRef]
Gao T, Lin M, Wu Y, Li K, Liu C, Zhou Q, Shen C, Zheng B, Huang X (2021). Transferrin receptor (TFRC) is essential for meiotic progression during mouse spermatogenesis. Zygote 29: 169–175. https://doi.org/10.1017/S0967199420000659 [Google Scholar] [PubMed] [CrossRef]
Geist D, Hönes GS, Gassen J, Kerp H, Kleinbongard P, Heusch G, Führer D, Moeller LC (2021). Noncanonical thyroid hormone receptor α action mediates arterial vasodilation. Endocrinology 162: 1–10. https://doi.org/10.1210/endocr/bqab099 [Google Scholar] [PubMed] [CrossRef]
Gerard A (1995). Endocytosis of androgen-binding protein (ABP) by spermatogenic cells. The Journal of Steroid Biochemistry and Molecular Biology 53: 533–542. https://doi.org/10.1016/0960-0760(95)00109-D [Google Scholar] [PubMed] [CrossRef]
Giese S, Hossain H, Markmann M, Chakraborty T, Tchatalbachev S, Guillou F, Bergmann M, Failing K, Weider K, Brehm R (2012). Sertoli-cell-specific knockout of connexin 43 leads to multiple alterations in testicular gene expression in prepubertal mice. Disease Models & Mechanisms 5: 895–913. https://doi.org/10.1242/dmm.008649 [Google Scholar] [PubMed] [CrossRef]
Gilleron J, Nebout M, Scarabelli L, Senegas-Balas F, Palmero S, Segretain D, Pointis G (2006). A potential novel mechanism involving connexin43 gap junction for control of Sertoli cell proliferation by thyroid hormones. Journal of Cellular Physiology 209: 153–161. https://doi.org/10.1002/jcp.20716 [Google Scholar] [PubMed] [CrossRef]
Gorga A, Rindone G, Regueira M, Riera MF, Pellizzari EH, Cigorraga SB, Meroni SB, Galardo MN (2018). HIF involvement in the regulation of rat Sertoli cell proliferation by FSH. Biochemical and Biophysical Research Communications 502: 508–514. https://doi.org/10.1016/j.bbrc.2018.05.206 [Google Scholar] [PubMed] [CrossRef]
Griswold MD (1988). Protein secretions of Sertoli cells. International Review of Cytology 110: 133–156. https://doi.org/10.1016/S0074-7696(08)61849-5 [Google Scholar] [PubMed] [CrossRef]
Griswold MD (1998). The central role of Sertoli cells in spermatogenesis. Cell & Developmental Biology 9: 411–416. https://doi.org/10.1006/scdb.1998.0203 [Google Scholar] [PubMed] [CrossRef]
Griswold MD, Solari A, Tung PS, Fritz IB (1977). Stimulation by follicle-stimulating hormone of DNA synthesis and of mitosis in cultured Sertoli cells prepared from testes of immature rats. Molecular and Cellular Endocrinology 7: 151–165. https://doi.org/10.1016/0303-7207(77)90064-8 [Google Scholar] [PubMed] [CrossRef]
Hai Y, Hou J, Yun Liu, Yang Liu, Yang H, Li Z, He Z (2014). The roles and regulation of Sertoli cells in fate determinations of spermatogonial stem cells and spermatogenesis. Seminars in Cell & Developmental Biology 29: 66–75. https://doi.org/10.1016/j.semcdb.2014.04.007 [Google Scholar] [PubMed] [CrossRef]
Hakovirta H, Yan W, Kaleva M, Zhang F, Vänttinen K, Morris PL, Söder M, Parvinen M, Toppari J (1999). Function of stem cell factor as a survival factor of spermatogonia and localization of messenger ribonucleic acid in the rat seminiferous epithelium. Endocrinology 140: 1492–1498. https://doi.org/10.1210/endo.140.3.6589 [Google Scholar] [PubMed] [CrossRef]
Hayes FJ, Pitteloud N, DeCruz S, CrowleyJr. WF, Boepple PA (2001). Importance of inhibin B in the regulation of FSH secretion in the human male. The Journal of Clinical Endocrinology & Metabolism 86: 5541–5546. https://doi.org/10.1210/jcem.86.11.8031 [Google Scholar] [PubMed] [CrossRef]
Haywood M, Spaliviero J, Jimemez M, King NJC, Handelsman DJ, Allan CM (2003). Sertoli and germ cell development in hypogonadal (hpg) mice expressing transgenic follicle-stimulating hormone alone or in combination with testosterone. Endocrinology 144: 509–517. https://doi.org/10.1210/en.2002-220710 [Google Scholar] [PubMed] [CrossRef]
He Z, Jiang J, Hofmann MC, Dym M (2007). Gfra1 silencing in mouse spermatogonial stem cells results in their differentiation via the inactivation of RET tyrosine kinase. Biology of Reproduction 77: 723–733. https://doi.org/10.1095/biolreprod.107.062513 [Google Scholar] [PubMed] [CrossRef]
Hermo L, Barin K, Oko R (1998). Androgen binding protein secretion and endocytosis by principal cells in the adult rat epididymis and during postnatal development. Journal of Andrology 19: 527–541. https://doi.org/10.1002/j.1939-4640.1998.tb02054.x [Google Scholar] [CrossRef]
Hilbold E, Distl O, Hoedemaker M, Wilkening S, Behr R et al. (2020). Loss of CX43 in murine Sertoli cells leads to altered prepubertal Sertoli cell maturation and impairment of the mitosis-meiosis switch. Cells 9: 676. https://doi.org/10.3390/cells9030676 [Google Scholar] [PubMed] [CrossRef]
Hofmann MC, Braydich-Stolle L, Dym M (2005). Isolation of male germ-line stem cells; influence of GDNF. Developmental Biology 279: 114–124. https://doi.org/10.1016/j.ydbio.2004.12.006 [Google Scholar] [PubMed] [CrossRef]
Holsberger DR, Buchold GM, Leal MC, Kiesewetter SE, O’Brien DA, Hess RA, Franca LR, Kiyokawa H, Cooke PS (2005). Cell-cycle inhibitors p27Kip1 and p21Cip1 regulate murine Sertoli cell proliferation. Biology of Reproduction 72: 1429–1436. https://doi.org/10.1095/biolreprod.105.040386 [Google Scholar] [PubMed] [CrossRef]
Holsberger DR, Cooke PS (2005). Understanding the role of thyroid hormone in Sertoli cell development: A mechanistic hypothesis. Cell Tissue Research 322: 133–140. https://doi.org/10.1007/s00441-005-1082-z [Google Scholar] [PubMed] [CrossRef]
Holsberger DR, Jirawatnotai S, Kiyokawa H, Cooke PS (2003). Thyroid hormone regulates the cell cycle inhibitor p27Kip1 in postnatal murine Sertoli cells. Endocrinology 144: 3732–3738. https://doi.org/10.1210/en.2003-0389 [Google Scholar] [PubMed] [CrossRef]
Hsu SY, Nakabayash K, Nishi S, Kumagai J, Kudo M, Sherwood OD, Hsueh AJW (2002). Activation of orphan receptors by the hormone relaxin. Science 295: 671–674. https://doi.org/10.1126/science.1065654 [Google Scholar] [PubMed] [CrossRef]
Hu SQ, Liu DL, Li CR, Xu YH, Hu K, Cui LD, Guo J (2021b). Wuzi-Yanzong prescription alleviates spermatogenesis disorder induced by heat stress dependent on Akt, NF-κB signaling pathway. Scientifc Reports 11: 18824. https://doi.org/10.1038/s41598-021-98036-2 [Google Scholar] [PubMed] [CrossRef]
Hu S, Liu D, Liu S, Li C, Guo J (2021a). Lycium barbarum polysaccharide ameliorates heat-stress-induced impairment of primary sertoli cells and the blood-testis barrier in rat via androgen receptor and akt phosphorylation. Evidence-Based Complementary and Alternative Medicine 2021: 5574202. https://doi.org/10.1155/2021/5574202 [Google Scholar] [PubMed] [CrossRef]
Huang J, Fang L, Zhang S, Zhang Y, Ou K, Wang C (2021). Long-term exposure to environmental levels of phenanthrene disrupts spermatogenesis in male mice. Environmental Pollution 285: 117488. https://doi.org/10.1016/j.envpol.2021.117488 [Google Scholar] [PubMed] [CrossRef]
Huang W, Quan C, Duan P, Tang S, Chen W, Yang K (2016). Nonylphenol induced apoptosis and autophagy involving the Akt/mTOR pathway in prepubertal Sprague-Dawley male rats in vivo and in vitro. Toxicology 373: 41–53. https://doi.org/10.1016/j.tox.2016.11.006 [Google Scholar] [PubMed] [CrossRef]
Jain M, Halder A (2012). Sertoli cell only syndrome: Status of Sertoli cell maturation and function. Indian Journal of Endocrinology and Metabolism 16: S512–513. https://doi.org/10.4103/2230-8210.104154 [Google Scholar] [PubMed] [CrossRef]
Jambor T, Lukáčová J, Tvrdá E, Kňažická Z, Forgács Z, Lukáč N (2016). The impact of 4-nonylphenol on the viability and hormone production of mouse Leydig cells. Folia Biologica (Praha) 62: 34–39. https://fb.cuni.cz/file/5800/fb2016a0004.pdf [Google Scholar]
Jannini EA, Crescenzi A, Rucci N, Screponi E, Carosa E, de Matteis A, Macchia E, D’amati G, D’armiento M (2000). Ontogenetic pattern of thyroid hormone receptor expression in the human testis. The Journal of Clinical Endocrinology & Metabolism 85: 3453–3457. https://doi.org/10.1210/jcem.85.9.6803 [Google Scholar] [PubMed] [CrossRef]
Johnston H, Baker PJ, Abel M, Charlton HM, Jackson G, Fleming L, Kumar TR, O’Shaughnessy PJ (2004). Regulation of Sertoli cell number and activity by follicle-stimulating hormone and androgen during postnatal development in the mouse. Endocrinology 145: 318–329. https://doi.org/10.1210/en.2003-1055 [Google Scholar] [PubMed] [CrossRef]
Kaitu’u-Lino TJ, Sluka P, Foo CFH, Stanton PG (2007). Claudin-11 expression and localisation is regulated by androgens in rat Sertoli cells in vitro. Reproduction 133: 1169–1179. https://doi.org/10.1530/REP-06-0385 [Google Scholar] [PubMed] [CrossRef]
Khan SA, Ndjountche L, Pratchard L, Spicer LJ, Davis JS (2002). Follicle-stimulating hormone amplifies insulin-like growth factor I-mediated activation of AKT/protein kinase B signaling in immature rat Sertoli cells. Endocrinology 143: 2259–2267. https://doi.org/10.1210/endo.143.6.8838 [Google Scholar] [PubMed] [CrossRef]
Kierszenbaum AL (2001). Transition nuclear proteins during spermiogenesis: Unrepaired DNA breaks not allowed. Molecular Reproduction and Development 58: 357–358. https://doi.org/10.1002/1098-2795(20010401)58:4<357::AID-MRD1>3.0.CO;2-T [Google Scholar] [PubMed] [CrossRef]
Konrad L, Dietze R, Kirch U, Kirch H, Eva A, Scheiner-Bobis G (2011). Cardiotonic steroids trigger non-classical testosterone signaling in Sertoli cells via the α4 isoform of the sodium pump. Biochimica et Biophysica Acta 1813: 2118–2124. https://doi.org/10.1016/j.bbamcr.2011.07.012 [Google Scholar] [PubMed] [CrossRef]
Kubota H, Avarbock MR, Brinster RL (2004). Growth factors essential for self-renewal and expansion of mouse spermatogonial stem cells. Proceedings of the National Academy of Sciences of the United States of America 101: 16489–16494. https://doi.org/10.1073/pnas.0407063101 [Google Scholar] [PubMed] [CrossRef]
Kumar TR, Wang Y, Lu N, Matzuk MM (1997). Follicle stimulating hormone is required for ovarian follicle maturation but not male fertility. Nature Genetics 15: 201–204. https://doi.org/10.1038/ng0297-201 [Google Scholar] [PubMed] [CrossRef]
Lasala C, Schteingart HF, Arouche N, Bedecarrás P, Grinspon RP, Picard JY, Josso N, di Clemente N, Rey RA (2011). SOX9 and SF1 are involved in cyclic AMP-mediated upregulation of anti-Müllerian gene expression in the testicular prepubertal Sertoli cell line SMAT1. American Journal of Physiology-Endocrinology and Metabolism 301: E539–E547. https://doi.org/10.1152/ajpendo.00187.2011 [Google Scholar] [PubMed] [CrossRef]
Lazari MFM, Lucas TFG, Yasuhara F, Games GRO, Siu ER, Royer C, Fernandes SAF, Porto CS (2009). Estrogen receptors and function in the male reproductive system. Arquivos Brasileiros de Endocrinologia & Metabologia 53: 923–933. https://doi.org/10.1590/S0004-27302009000800005 [Google Scholar] [PubMed] [CrossRef]
Leaver RB (2016). Male infertility-an overview of causes and treatment options. British Journal of Nursing 25: S35–S40. https://doi.org/10.12968/bjon.2016.25.18.S35 [Google Scholar] [PubMed] [CrossRef]
Li X, Long XY, Xie YJ, Zeng X, Chen X, Mo ZC (2019). The roles of retinoic acid in the differentiation of spermatogonia and spermatogenic disorders. Clinica Chimica Acta 497: 54–60. https://doi.org/10.1016/j.cca.2019.07.013 [Google Scholar] [PubMed] [CrossRef]
Li N, Mruk DD, Mok KW, Li MWM, Wong CKC, Lee WM, Han D, Silvestrini B, Cheng CY (2016). Connexin 43 reboots meiosis and reseals blood-testis barrier following toxicant-mediated aspermatogenesis and barrier disruption. The FASEB Journal 30: 1436–1452. https://doi.org/10.1096/fj.15-276527 [Google Scholar] [PubMed] [CrossRef]
Lim K, Hwang BD (1995). Follicle-stimulating hormone transiently induces expression of protooncogene c-myc in primary Sertoli cell cultures of early pubertal and prepubertal rat. Molecular and Cellular Endocrinology 111: 51–56. https://doi.org/10.1016/0303-7207(95)03543-G [Google Scholar] [PubMed] [CrossRef]
Liu X, Nie S, Yu Q, Wang X, Huang D, Xie M (2016). Downregulation of steroid hormone receptor expression and activation of cell signal transduction pathways induced by a chiral nonylphenol isomer in mouse Sertoli TM4 cells. Environmental Toxicology 32: 469–476. https://doi.org/10.1002/tox.22251 [Google Scholar] [PubMed] [CrossRef]
Lord T, Oatley MJ, Oatley JM (2018). Testicular architecture is critical for mediation of retinoic acid responsiveness by undifferentiated spermatogonial subtypes in the mouse. Stem Cell Reports 10: 538–552. https://doi.org/10.1016/j.stemcr.2018.01.003 [Google Scholar] [PubMed] [CrossRef]
Lucas TFG, Amaral LS, Porto CS, Quintas LEM (2012). Na+/K+-ATPase α1 isoform mediates ouabain-induced expression of cyclin D1 and proliferation of rat Sertoli cells. Reproduction 144: 737–745. https://doi.org/10.1530/REP-12-0232 [Google Scholar] [PubMed] [CrossRef]
Lucas TFG, Lazari MFM, Porto CS (2014a). Differential role of the estrogen receptors ESR1 and ESR2 on the regulation of proteins involved with proliferation and differentiation of Sertoli cells from 15-day-old rats. Molecular and Cellular Endocrinology 382: 84–96. https://doi.org/10.1016/j.mce.2013.09.015 [Google Scholar] [PubMed] [CrossRef]
Lucas TFG, Nascimento AR, Pisolato R, Pimenta MT, Lazari MFM, Porto CS (2014b). Receptors and signaling pathways involved in proliferation and differentiation of Sertoli cells. Spermatogenesis 4: e28138. https://doi.org/10.4161/spmg.28138 [Google Scholar] [PubMed] [CrossRef]
Lui WY, Mruk D, Lee WM, Cheng CY (2003). Sertoli cell tight junction dynamics: Their regulation during spermatogenesis. Biology Reproduction 68: 1087–1097. https://doi.org/10.1095/biolreprod.102.010371 [Google Scholar] [PubMed] [CrossRef]
Lyon MF, Glenister PH, Lamoreux ML (1975). Normal spermatozoa from androgen-resistant germ cells of chimaeric mice and the role of androgen in spermatogenesis. Nature 258: 620–622. https://doi.org/10.1038/258620a0 [Google Scholar] [PubMed] [CrossRef]
Macheroni C, Lucas TFG, Porto CS (2020). The role of estrogen receptors in rat Sertoli cells at different stages of development. Heliyon 6: e05363. https://doi.org/10.1016/j.heliyon.2020.e05363 [Google Scholar] [PubMed] [CrossRef]
McDonald CA, Millena AC, Reddy S, Finlay S, Vizcarra J, Khan SA, Davis JS (2006). Follicle-stimulating hormone-induced aromatase in immature rat Sertoli cells requires an active phosphatidylinositol 3-kinase pathway and is inhibited via the mitogen-activated protein kinase signaling pathway. Molecular Endocrinology 20: 608–618. https://doi.org/10.1210/me.2005-0245 [Google Scholar] [PubMed] [CrossRef]
McLachlan RI, Wreford NG, Tsonis C, de Kretser DM, Robertson DM (1994). Testosterone effects on spermatogenesis in the gonadotropin-releasing hormone-immunized rat. Biology of Reproduction 50: 271–280. https://doi.org/10.1095/biolreprod50.2.271 [Google Scholar] [PubMed] [CrossRef]
Meng J, Greenlee AR, Taub CJ, Braun RE (2011). Sertoli cell-specific deletion of the androgen receptor compromises testicular immune privilege in mice. Biology of Reproduction 85: 254–260. https://doi.org/10.1095/biolreprod.110.090621 [Google Scholar] [PubMed] [CrossRef]
Meng J, Holdcraft RW, Shima JE, Griswold MD, Braun RE (2005). Androgens regulate the permeability of the blood-testis barrier. Proceedings of the National Academy of Sciences of the United States of America 102: 16696–16700. https://doi.org/10.1073/pnas.0506084102 [Google Scholar] [PubMed] [CrossRef]
Meng X, Lindahl ML, Hyvönen M E, Parvinen M, de Rooij D G et al. (2000). Regulation of cell fate decision of undifferentiated spermatogonia by GDNF. Science 287: 1489–1493. https://doi.org/10.1126/science.287.5457.1489 [Google Scholar] [PubMed] [CrossRef]
Meroni SB, Galardo MN, Rindone G, Gorga A, Riera MF, Cigorraga SB (2019). Molecular mechanisms and signaling pathways involved in sertoli cell proliferation. Front Endocrinol 10: 224. https://doi.org/10.3389/fendo.2019.00224 [Google Scholar] [PubMed] [CrossRef]
Meroni SB, Riera MF, Pellizzari EH, Cigorraga SB (2002). Regulation of rat Sertoli cell function by FSH: Possible role of phosphatidylinositol 3-kinase/protein kinase B pathway. Journal of Endocrinology 174: 195–204. https://doi.org/10.1677/joe.0.1740195 [Google Scholar] [PubMed] [CrossRef]
Meroni SB, Riera MF, Pellizzari EH, Galardo MN, Cigorraga SB (2004). FSH activates phosphatidylinositol 3-kinase/protein kinase B signaling pathway in 20-day-old Sertoli cells independently of IGF-I. Journal of Endocrinology 180: 257–265. https://doi.org/10.1677/joe.0.1800257 [Google Scholar] [PubMed] [CrossRef]
Mok KW, Mruk DD, Cheng CY (2014). rpS6 regulates blood-testis barrier dynamics through Akt-mediated effects on MMP-9. Journal of Cell Science 127: 4870–4882. https://doi.org/10.1242/jcs.152231 [Google Scholar] [PubMed] [CrossRef]
Morales C, Sylvester SR, Griswold MD (1987). Transport of iron and transferrin synthesis by the seminiferous epithelium of the rat in vivo. Biology of Reproduction 37: 995–1005. https://doi.org/10.1095/biolreprod37.4.995 [Google Scholar] [PubMed] [CrossRef]
Musnier A, Heitzler D, Boulo T, Tesseraud S, Durand G, Lecureuil C, Guillou H, Poupon A, Reiter E, Crepieux P (2009). Developmental regulation of p70 S6 kinase by a G protein-coupled receptor dynamically modelized in primary cells. Cellular and Molecular Life Sciences 66: 3487–3503. https://doi.org/10.1007/s00018-009-0134-z [Google Scholar] [PubMed] [CrossRef]
Nascimento AR, Macheroni C, Lucas TFG, Porto CS, Lazari MFM (2016). Crosstalk between FSH and relaxin at the end of the proliferative stage of rat Sertoli cells. Reproduction 152: 613–628. https://doi.org/10.1530/REP-16-0330 [Google Scholar] [PubMed] [CrossRef]
Nascimento AR, Pimenta MT, Lucas TFG, Porto CS, Lazari MFM (2013). Relaxin and Sertoli cell proliferation. Italian Journal of Anatomy and Embryology 118: 26–28, https://oajournals.fupress.net/index.php/ijae/article/view/3220 [Google Scholar] [PubMed]
Nascimento AR, Pimenta MT, Lucas TFG, Royer C, Porto CS, Lazari MFM (2012). Intracellular signaling pathways involved in the relaxin-induced proliferation of rat Sertoli cells. European Journal of Pharmacology 691: 283–291. https://doi.org/10.1016/j.ejphar.2012.07.021 [Google Scholar] [PubMed] [CrossRef]
Naughton CK, Jain S, Strickland AM, Gupta A, Milbrandt J (2006). Glial cell-line derived neurotrophic factor-mediated RET signaling regulates spermatogonial stem cell fate. Biology of Reproduction 74: 314–321. https://doi.org/10.1095/biolreprod.105.047365 [Google Scholar] [PubMed] [CrossRef]
Naz RK, Chaturvedi MM, Aggarwal BB (1994). Role of cytokines and proto-oncogenes in sperm cell function: Relevance to immunologic infertility. American Journal of Reproductive Immuuology 32: 26–37. https://doi.org/10.1111/j.1600-0897.1994.tb00875.x [Google Scholar] [PubMed] [CrossRef]
Neirijnck Y, Kühne F, Mayere C, Pavlova E, Sararols P, Foti M, Atanassova N, Nef S (2019). Tumor suppressor PTEN regulates negatively Sertoli cell proliferation, testis size, and sperm production in vivo. Endocrinology 160: 387–398. https://doi.org/10.1210/en.2018-00892 [Google Scholar] [PubMed] [CrossRef]
Ni FD, Hao SL, Yang WX (2020). Molecular insights into hormone regulation via signaling pathways in Sertoli cells: With discussion on infertility and testicular tumor. Gene 753: 144812. https://doi.org/10.1016/j.gene.2020.144812 [Google Scholar] [PubMed] [CrossRef]
Oetting A, Yen PM (2007). New insights into thyroid hormone action. Best Practice & Research Clinical Endocrinology & Metabolism 21: 193–208. https://doi.org/10.1016/j.beem.2007.04.004 [Google Scholar] [PubMed] [CrossRef]
Ohta H, Tohda A, Nishimune Y (2003). Proliferation and differentiation of spermatogonial stem cells in the W/Wv mutant mouse testis. Biology of Reproduction 69: 1815–1821. https://doi.org/10.1095/biolreprod.103.019323 [Google Scholar] [PubMed] [CrossRef]
Ohta H, Yomogida K, Dohmae K, Nishimune Y (2000). Regulation of proliferation and differentiation in spermatogonial stem cells: The role of c-kit and its ligand SCF. Development 127: 2125–2131. https://doi.org/10.1242/dev.127.10.2125 [Google Scholar] [PubMed] [CrossRef]
Oldknow KJ, Seebacher J, Goswami T, Villen J, Pitsillides AA, O’Shaughnessy PJ, Gygi SP, Schneyer AL, Mukherjee A (2013). Follistatin-like 3 (FSTL3) mediated silencing of transforming growth factor beta (TGFβ) signaling is essential for testicular aging and regulating testis size. Endocrinology 154: 1310–1320. https://doi.org/10.1210/en.2012-1886 [Google Scholar] [PubMed] [CrossRef]
Oliveira PF, Alves MG, Rato L, Laurentino S, Silva J et al. (2012). Effect of insulin deprivation on metabolism and metabolism-associated gene transcript levels of in vitro cultured human Sertoli cells. Biochimica et Biophysica Acta 1820: 84–89. https://doi.org/10.1016/j.bbagen.2011.11.006 [Google Scholar] [PubMed] [CrossRef]
Orth JM (1984). The role of follicle-stimulating hormone in controlling Sertoli cell proliferation in testes of fetal rats. Endocrinology 115: 1248–1255. https://doi.org/10.1210/endo-115-4-1248 [Google Scholar] [PubMed] [CrossRef]
Paduch DA, Hilz S, Grimson A, Schlegel PN, Jedlicka AE, Wright WW (2019). Aberrant gene expression by Sertoli cells in infertile men with Sertoli cell-only syndrome. PLoS One 14: e0216586. https://doi.org/10.1371/journal.pone.0216586 [Google Scholar] [PubMed] [CrossRef]
Pentikäinen V, Erkkilä K, Suomalainen L, Parvinen M, Dunkel L (2000). Estradiol acts as a germ cell survival factor in the human testis in vitro. The Journal of Clinical Endocrinology & Metabolism 85: 2057–2067. https://doi.org/10.1210/jcem.85.5.6600 [Google Scholar] [PubMed] [CrossRef]
Pimenta MT, Francisco RAR, Silva RP, Porto CS, Lazari MFM (2015). Relaxin affects cell organization and early and late stages of spermatogenesis in a coculture of rat testicular cells. Andrology 3: 772–786. https://doi.org/10.1111/andr.12056 [Google Scholar] [PubMed] [CrossRef]
Pimenta MT, Porto CS, Lazari MFM (2013). Effects of relaxin in a co-culture of Sertoli and germ cells. Italian Journal of Anatomy and Embryology 118: 29–31, https://oajournals.fupress.net/index.php/ijae/article/view/3223 [Google Scholar] [PubMed]
Pitetti JL, Calvel P, Zimmermann C, Conne B, Papaioannou MD et al. (2013). An essential role for insulin and IGF1 receptors in regulating Sertoli cell proliferation, testis size, and FSH action in mice. Molecular Endocrinology 27: 814–827. https://doi.org/10.1210/me.2012-1258 [Google Scholar] [PubMed] [CrossRef]
Qian X, Mruk DD, Cheng YH, Tang EI, Han D, Lee WM, Wong EWP, Cheng CY (2014). Actin binding proteins, spermatid transport and spermiation. Seminars in Cell & Developmental Biology 30: 75–85. https://doi.org/10.1016/j.semcdb.2014.04.018 [Google Scholar] [PubMed] [CrossRef]
Rajamanickam GD, Kastelic JP, Thundathil JC (2017). The ubiquitous isoform of Na/K-ATPase (ATP1A1) regulates junctional proteins, connexin 43 and claudin 11 via Src-EGFR-ERK1/2-CREB pathway in rat Sertoli cells. Biology of Reproduction 96: 456–468. https://doi.org/10.1095/biolreprod.116.141267 [Google Scholar] [PubMed] [CrossRef]
Rato L, Alves MG, Socorro S, Duarte AI, Cavaco JE, Oliveira PF (2012). Metabolic regulation is important for spermatogenesis. Nature Reviews Urology 9: 330–338. https://doi.org/10.1038/nrurol.2012.77 [Google Scholar] [PubMed] [CrossRef]
Rey R, Lukas-Croisier C, Lasala C, Bedecarras P (2003). AMH/MIS: What we know already about the gene, the protein and its regulation. Molecular and Cellular Endocrinology 211: 21–31. https://doi.org/10.1016/j.mce.2003.09.007 [Google Scholar] [PubMed] [CrossRef]
Riera MF, Regueira M, Galardo MN, Pellizzari EH, Meroni SB, Cigorraga SB (2012). Signal transduction pathways in FSH regulation of rat Sertoli cell proliferation. American Journal of Physiology-Endocrinology and Metabolism 302: E914–E923. https://doi.org/10.1152/ajpendo.00477.2011 [Google Scholar] [PubMed] [CrossRef]
Rode K, Weider K, Damm OS, Wistuba J, Langeheine M, Brehm R (2018). Loss of connexin 43 in Sertoli cells provokes postnatal spermatogonial arrest, reduced germ cell numbers and impaired spermatogenesis. Reproductive Biology 18: 456–466. https://doi.org/10.1016/j.repbio.2018.08.001 [Google Scholar] [PubMed] [CrossRef]
Rodprasert W, Virtanen HE, Makela JA, Toppari J (2019). Hypogonadism and cryptorchidism. Frontiers in Endocrinology 10: 906. https://doi.org/10.3389/fendo.2019.00906 [Google Scholar] [PubMed] [CrossRef]
Rogowska A, Pomastowski P, Sagandykova G, Buszewski B (2019). Zearalenone and its metabolites: Effect on human health, metabolism and neutralisation methods. Toxicon 162: 46–56. https://doi.org/10.1016/j.toxicon.2019.03.004 [Google Scholar] [PubMed] [CrossRef]
Rossi SP, Windschüttl S, Matzkin ME, Rey-Ares V, Terradas C et al. (2016). Reactive oxygen species (ROS) production triggered by prostaglandin D2 (PGD2) regulates lactate dehydrogenase (LDH) expression/activity in TM4 Sertoli cells. Molecular and Cellular Endocrinology 434: 154–165. https://doi.org/10.1016/j.mce.2016.06.021 [Google Scholar] [PubMed] [CrossRef]
Royer C, Lucas TFG, Lazari MFM, Porto CS (2012). 17beta-estradiol signaling and regulation of proliferation and apoptosis of rat Sertoli cells. Biology of Reproduction 86: 1–13. https://doi.org/10.1095/biolreprod.111.096891 [Google Scholar] [PubMed] [CrossRef]
Russell L (1977). Movement of spermatocytes from the basal to the adluminal compartment of the rat testis. American Journal of Anatomy 148: 313–328. https://doi.org/10.1002/aja.1001480303 [Google Scholar] [PubMed] [CrossRef]
Sharpe RM, Maddocks S, Millar M, Kerr JB, Saunders PTK, McKinnell C (1992). Testosterone and spermatogenesis-identification of stage-specific, androgen-regulated proteins secreted by adult rat seminiferous tubules. Journal of Andrology 13: 172–184. https://doi.org/10.1002/j.1939-4640.1992.tb01653.x [Google Scholar] [CrossRef]
Sharpe RM, McKinnell C, Kivlin C, Fisher JS (2003). Proliferation and functional maturation of Sertoli cells, and their relevance to disorders of testis function in adulthood. Reproduction 125: 769–784. https://doi.org/10.1530/rep.0.1250769 [Google Scholar] [PubMed] [CrossRef]
Sinha RA, Yen PM (2016). Thyroid hormone disruption and neurodevelopment. In: Thyroid Hormone Disruption and Neurodevelopment, vol. 881, pp. 3–21. https://doi.org/10.1007/978-1-4939-3737-0_1 [Google Scholar] [CrossRef]
Steger K, Tetens F, Bergmann M (1999). Expression of connexin 43 in human testis. Histochemistry and Cell Biology 112: 215–220. https://doi.org/10.1007/s004180050409 [Google Scholar] [PubMed] [CrossRef]
Sun Y, Yang WR, Luo HL, Wang XZ, Chen ZQ, Zhang JJ, Wang Y, Li XM (2015). Thyroid hormone inhibits the proliferation of piglet Sertoli cell via PI3K signaling pathway. Theriogenology 83: 86–94. https://doi.org/10.1016/j.theriogenology.2014.08.003 [Google Scholar] [PubMed] [CrossRef]
Sylvester SR, Griswold MD (1984). Localization of transferrin and transferrin receptors in rat testes. Biology of Reproduction 31: 195–203. https://doi.org/10.1095/biolreprod31.1.195 [Google Scholar] [PubMed] [CrossRef]
Tajima Y, Sawada K, Morimoto T, Nishimune Y (1994). Switching of mouse spermatogonial proliferation from the c-kit receptor-independent type to the receptor-dependent type during differentiation. Journals of Reproduction and Fertility 102: 117–122. https://doi.org/10.1530/jrf.0.1020117 [Google Scholar] [PubMed] [CrossRef]
Talati J, Sheikh H (1991). Sertoli cell only syndrome (SECOSLessons from case studies. Journal of the Pakistan Medical Association 41: 219–223, https://jpma.org.pk/article-details/5322?article_id=5322 [Google Scholar] [PubMed]
Themmen APN, Huhtaniemi IT (2000). Mutations of gonadotropins and gonadotropin receptors: Elucidating the physiology and pathophysiology of pituitary-gonadal function. Endocrine Reviews 21: 551–583. https://doi.org/10.1210/edrv.21.5.0409 [Google Scholar] [PubMed] [CrossRef]
ur Rehman Z, Worku T, Davis JS, Talpur HS, Bhattarai D et al. (2017). Role and mechanism of AMH in the regulation of Sertoli cells in mice. Journal of Steroid Biochemistry and Molecular Biology 174: 133–140. https://doi.org/10.1016/j.jsbmb.2017.08.011 [Google Scholar] [PubMed] [CrossRef]
Walker WH (2021). Molecular mechanisms in spermatogenesis. In: Advances in Experimental Medicine and Biology, vol. 1381, pp. 175–203. https://doi.org/10.1007/978-3-030-77779-1 [Google Scholar] [CrossRef]
Walker WH, Cheng J (2005). FSH and testosterone signaling in Sertoli cells. Reproduction 130: 15–28. https://doi.org/10.1530/rep.1.00358 [Google Scholar] [PubMed] [CrossRef]
Wang C, Fu W, Quan C, Yan M, Liu C, Qi S, Yang K (2015). The role of Pten/Akt signaling pathway involved in BPA-induced apoptosis of rat Sertoli cells. Environmental Toxicology 30: 793–802. https://doi.org/10.1002/tox.21958 [Google Scholar] [PubMed] [CrossRef]
Wang JM, Li ZF, Yang WX (2022). What does androgen receptor signaling pathway in Sertoli cells during normal spermatogenesis tell us? Frontiers in Endocrinology 13: 838858. https://doi.org/10.3389/fendo.2022.838858 [Google Scholar] [PubMed] [CrossRef]
Wang P, Suo LJ, Wang YF, Shang H, Li GX, Hu JH, Li QW (2014). Effects of GDNF and LIF on mouse spermatogonial stem cells proliferation in vitro. Cytotechnology 66: 309–316. https://doi.org/10.1007/s10616-013-9574-2 [Google Scholar] [PubMed] [CrossRef]
Wang RS, Yeh S, Chen LM, Lin HY, Zhang C et al. (2006). Androgen receptor in Sertoli cell is essential for germ cell nursery and junctional complex formation in mouse testes. Endocrinology 147: 5624–5633. https://doi.org/10.1210/en.2006-0138 [Google Scholar] [PubMed] [CrossRef]
Wang X, Zhang X, Hu L, Li H (2018b). Exogenous leptin affects sperm parameters and impairs blood testis barrier integrity in adult male mice. Reproductive Biology and Endocrinology 16: 55. https://doi.org/10.1186/s12958-018-0368-4 [Google Scholar] [PubMed] [CrossRef]
Wang D, Zhao W, Liu J, Wang Y, Yuan C, Zhang F, Jin G, Qin Q (2021). Effects of HIF-1α on spermatogenesis of varicocele rats by regulating VEGF/PI3K/Akt signaling pathway. Reproductive Sciences 28: 1161–1174. https://doi.org/10.1007/s43032-020-00395-0 [Google Scholar] [PubMed] [CrossRef]
Wang C, Zheng P, Adeniran SO, Ma M, Huang F, Adegoke EO, Zhang G (2019). Thyroid hormone (T3) is involved in inhibiting the proliferation of newborn calf Sertoli cells via the PI3K/Akt signaling pathway in vitro. Theriogenology 133: 1–9. https://doi.org/10.1016/j.theriogenology.2019.04.025 [Google Scholar] [PubMed] [CrossRef]
Wang BJ, Zheng WL, Feng NN, Wang T, Zou H, Gu JH, Yuan Y, Liu XZ, Liu ZP, Bian JC (2018a). The effects of autophagy and PI3K/AKT/m-TOR signaling pathway on the cell-cycle arrest of rats primary Sertoli cells induced by Zearalenone. Toxins 10: 398. https://doi.org/10.3390/toxins10100398 [Google Scholar] [PubMed] [CrossRef]
Wauben-Penris PJJ, Strous GJ, van der Donk HA (1986). Transferrin receptors of isolated rat seminiferous tubules bind both rat and human transferrin. Biology of Reproduction 35: 1227–1234. https://doi.org/10.1095/biolreprod35.5.1227 [Google Scholar] [PubMed] [CrossRef]
Willems A, Batlouni SR, Esnal A, Swinnen JV, Saunders PTK, Sharpe RM, Franca LR, Gendt KD, Verhoeven G (2010). Selective ablation of the androgen receptor in mouse Sertoli cells affects Sertoli cell maturation, barrier formation and cytoskeletal development. PLoS One 5: e14168. https://doi.org/10.1371/journal.pone.0014168 [Google Scholar] [PubMed] [CrossRef]
Wreford NG, Kumar TR, Matzuk MM, de Kretser DM (2001). Analysis of the testicular phenotype of the follicle-stimulating hormone β-subunit knockout and the activin type ii receptor knockout mice by stereological analysis. Endocrinology 142: 2916–2920. https://doi.org/10.1210/endo.142.7.8230 [Google Scholar] [PubMed] [CrossRef]
Wu L, Dong H, Zhao J, Wang Y, Yang Q, Jia C, Ma J (2015). Diosgenin stimulates rat TM4 cell proliferation through activating plasma membrane translocation and transcriptional activity of estrogen receptors. Biology of Reproduction 92. https://doi.org/10.1095/biolreprod.114.124206 [Google Scholar] [PubMed] [CrossRef]
Xi H, Ren F, Li Y, Xian M, Wang L, Hu J (2022). FSH inhibits autophagy and lysosomal biogenesis to regulate protein degradation in cultured goat Sertoli cells. Molecular and Cellular Endocrinology 540: 111505. https://doi.org/10.1016/j.mce.2021.111505 [Google Scholar] [PubMed] [CrossRef]
Xu YH, Li Y, Hu SQ, Li CR, Liu DL, Hu K, Cui LD, Guo J (2021). Effect of Wuzi Yanzong Pills on Sertoli cells and blood-testis barrier in heat-stressed rats based on Akt signalling pathway. Andrologia 53: e14169. https://doi.org/10.1111/and.14169 [Google Scholar] [PubMed] [CrossRef]
Xu YP, Liu BX, Zhang XP, Yang CW, Wang CH (2014). A Chinese herbal formula, Wuzi Yanzong pill, improves spermatogenesis by modulating the secretory function of Sertoli cells. Chinese Journal of Integrative Medicine 20: 194–199. https://doi.org/10.1007/s11655-014-1743-4 [Google Scholar] [PubMed] [CrossRef]
Yan HHN, Mruk DD, Lee WM, Cheng CY (2008). Blood-testis barrier dynamics are regulated by testosterone and cytokines via their differential effects on the kinetics of protein endocytosis and recycling in Sertoli cells. The FASEB Journal 22: 1945–1959. https://doi.org/10.1096/fj.06-070342 [Google Scholar] [PubMed] [CrossRef]
Yang S, Ping P, Ma M, Li P, Tian R et al. (2014). Generation of haploid spermatids with fertilization and development capacity from human spermatogonial stem cells of cryptorchid patients. Stem Cell Reports 3: 663–675. https://doi.org/10.1016/j.stemcr.2014.08.004 [Google Scholar] [PubMed] [CrossRef]
Yang WR, Yong Wang, Yi Wang, Zhang JJ, Zhang JH, Lu C, Wang XZ (2015). mTOR is involved in 17β-estradiol-induced, cultured immature boar Sertoli cell proliferation via regulating the expression of SKP2, CCND1, and CCNE1. Molecular Reproduction & Development 82: 305–314. https://doi.org/10.1002/mrd.22473 [Google Scholar] [PubMed] [CrossRef]
Yang WR, Zhu FW, Zhang JJ, Wang Y, Zhang JH, Lu C, Wang XZ (2017). PI3K/Akt activated by GPR30 and Src regulates 17β-estradiol-induced cultured immature boar Sertoli cells proliferation. Reproductive Sciences 24: 57–66. https://doi.org/10.1177/1933719116649696 [Google Scholar] [PubMed] [CrossRef]
Yoshinaga K, Nishikawa S, Ogawa M, Hayashi SI, Kunisada T, Fujimoto T, Nishikawa SI (1991). Role of c-kit in mouse spermatogenesis: Identification of spermatogonia asa specific site of c-kit expression and function. Development 113: 689–699. https://doi.org/10.1242/dev.113.2.689 [Google Scholar] [PubMed] [CrossRef]
Zhang YW, Kaneda M, Morita I (2003). The gap junction-independent tumor-suppressing effect of connexin 43. The Journal of Biological Chemistry 278: 44852–44856. https://doi.org/10.1074/jbc.M305072200 [Google Scholar] [PubMed] [CrossRef]
Zhang J, Wong CH, Xia W, Mruk DD, Lee NPY, Lee WM, Cheng CY (2005). Regulation of Sertoli-germ cell adherens junction dynamics via changes in protein-protein interactions of the N-cadherin-beta-catenin protein complex which are possibly mediated by c-Src and myotubularin-related protein 2: An in vivo study using an androgen suppression model. Endocrinology 146: 1268–1284. https://doi.org/10.1210/en.2004-1194 [Google Scholar] [PubMed] [CrossRef]
Zheng W, Pan SY, Wang G, Wang YJ, Liu Q, Gu JH, Yuan Y, Liu XZ, Liu ZP, Bian JC (2016). Zearalenone impairs the male reproductive system functions via inducing structural and functional alterations of Sertoli cells. Environmental Toxicology and Pharmacology 42: 146–155. https://doi.org/10.1016/j.etap.2016.01.013 [Google Scholar] [PubMed] [CrossRef]
Zheng W, Wang B, Si M, Zou H, Song R et al. (2018). Zearalenone altered the cytoskeletal structure via ER stress-autophagy-oxidative stress pathway in mouse TM4 Sertoli cells. Scientific Reports 8: 3320. https://doi.org/10.1038/s41598-018-21567-8 [Google Scholar] [PubMed] [CrossRef]
Cite This Article
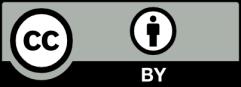