Open Access
ARTICLE
A Ring-Reinforced Right Ventricle to Pulmonary Artery Conduit is Associated with Better Regional Mechanics after Stage I Norwood Operation
1
Department of Cardiology, Boston Children’s Hospital, Boston, USA
2
Department of Pediatrics, Harvard Medical School, Boston, USA
3
Department of Cardiothoracic Surgery, Boston Children’s Hospital, Boston, USA
4
Department of Surgery, Harvard Medical School, Boston, USA
* Corresponding Author: Benjamin Zielonka. Email:
Congenital Heart Disease 2022, 17(5), 591-603. https://doi.org/10.32604/chd.2022.021509
Received 18 March 2022; Accepted 07 July 2022; Issue published 06 September 2022
Abstract
Background: The right ventricle to pulmonary artery conduit (RVPAC) may impair right ventricular (RV) function in patients with functional single right ventricles. Modification of the RVPAC using a ring-reinforced end with dunked insertion into the RV through a limited ventriculotomy may reduce the impact on RV function. We compared RV segmental strain between patients with a traditional RVPAC and ring-reinforced RVPAC using feature tracking cardiovascular magnetic resonance (CMR) imaging. Methods: Patients with CMR examinations after Stage I operation with RVPAC between 2000 and 2018 were reviewed. Ventricular mass, volumes, late gadolinium enhancement (LGE), and peak radial and circumferential strain of the 4 segments near the RVPAC insertion site were analyzed. Results: The study included 71 CMR examinations in 61 patients (30 traditional RVPAC, 31 ring-reinforced RVPAC). Prior to Stage II, the ring-reinforced RVPAC group had better peak radial strain and circumferential strain in 1 of 4 segments proximal to the RVPAC insertion site compared to the traditional RVPAC group. Prior to Stage III operation, the ring-reinforced group had better peak radial and circumferential strain in 2 of 4 segments. LGE at the RVPAC insertion site was observed in 97% of patients before Stage II and 95% of patients before Stage III. RVPAC type and regional strain were not associated with transplant-free survival during a median follow-up of 6.3 years. Conclusions: Compared to a traditional RVPAC, the ring-reinforced RVPAC is associated with improved regional mechanics at the conduit insertion site. Further investigation of long-term outcomes after ring-reinforced RVPAC is warranted.Keywords
The first stage of surgical palliation for patients with functional single ventricles is associated with significant morbidity and mortality [1–3]. The right ventricle to pulmonary artery conduit (RVPAC) provides pulmonary blood flow for patients with functional single right ventricles undergoing the Stage I Norwood operation with the Sano modification. The Single Ventricle Reconstruction Trial demonstrated that infants undergoing the Stage I Norwood operation had higher 1-year transplant-free survival with a right ventricle to pulmonary artery conduit (RVPAC) compared to a Blalock-Taussig shunt [3]. While the RVPAC has become the dominant shunt type at most institutions, subsequent studies have shown no difference in medium-term mortality between the two approaches [4]. In addition, the need for a ventriculotomy and muscular excision with traditional RVPAC placement has raised concerns regarding detrimental effects on right ventricular myocardial function [5–7].
Since 2010, our institution has performed a modified technique in which a ring-reinforced RVPAC is inserted or “dunked” transmurally into the myocardium through a limited ventriculotomy [8]. The use of a stiffer ring-reinforced conduit is associated with reduced proximal conduit obstruction and need for shunt-related interventions [9–11], as well as improved pulmonary artery growth at the time of Stage II operation [8]. The lack of a full ventriculotomy and muscular excision with the ring-reinforced RVPAC also carries theoretical advantages for myocardial performance, but the impact of this modification on right ventricular mechanics has not yet been studied.
The assessment of regional right ventricular deformation is typically a qualitative analysis. Small series have described quantitative regional ventricular function after Stage I operation using speckle-tracking echocardiography [12,13]. Cardiovascular magnetic resonance imaging (CMR) offers more comprehensive spatial resolution for assessment of regional function. The purpose of this study is to compare regional right ventricular myocardial function in patients who had a traditional versus ring-reinforced RVPAC using feature tracking CMR.
In this retrospective study, a database search identified all patients with functional single right ventricles who underwent a Stage I Norwood operation with a RVPAC between 2000 and 2018 at Boston Children’s Hospital and had a CMR study performed prior to Fontan or biventricular conversion surgery. Patients were excluded if the CMR study was technically inadequate for feature tracking analysis. If a patient had multiple CMR studies within a single stage of palliation, only the most recent study was used for analysis. The study was approved by the Scientific Review Committee of the Department of Cardiology and by the Boston Children’s Hospital Committee on Clinical Investigation (Institutional Review Board protocol number P00034066).
Subjects were assigned to one of two groups based on the presence of a traditional RVPAC or ring-reinforced RVPAC as determined by review of the surgical operative note at time of Stage I operation. Baseline demographic and echocardiographic data were extracted from the medical records. Clinical parameters included underlying diagnoses, birth weight, gestational age at birth, fetal diagnosis and intervention, age at time of Stage I operation, age at time of CMR examination, need for RVPAC intervention, and need for extracorporeal membrane oxygenation support or cardiopulmonary resuscitation. Echocardiographic variables included the qualitative grade of right ventricular systolic function and tricuspid regurgitation at the time of Stage I operation.
The details of the surgical technique for traditional and ring-reinforced RVPAC conduit placement at our institution have been previously described [8]. Briefly, placement of the traditional RVPAC requires a right ventriculotomy in the infundibulum with muscular excision, followed by anastomosis of a non-reinforced Gore-Tex graft that is sewn flush by direct suture to the epicardium (Fig. 1A). In contrast, the ring-reinforced RVPAC is placed by a “dunked” technique in which the proximal end of a ring-reinforced expanded polytetrafluoroethylene graft is directly inserted into the right ventricle through a limited right ventriculotomy without muscular excision (Fig. 1B). The conduit is then secured with limited surgical fixing sutures and surgical glue into the right ventricular free wall.
Figure 1: Right ventricle to pulmonary artery conduit techniques
CMR studies were performed with a 1.5 Tesla scanner (GE Medical Systems, Milwaukee, Wisconsin; and Philips Healthcare, Best, the Netherlands). The CMR and late gadolinium enhancement (LGE) acquisitions protocols used at our institution for assessment of patients with congenital heart disease have been described [14,15]. ECG-gated steady-state free precession cine images in the ventricular short-axis plane were used for ventricular volume, mass, function, and feature tracking analysis. The ventricular short-axis plane was derived from two orthogonal planes drawn perpendicular from the tricuspid valve annulus to the right ventricular apex per our institutional lab protocol for patients with functional single right ventricles. The presence of LGE was assessed using inversion-recovery prepared, phase-sensitive, electrocardiogram-triggered, breath-hold segmented fast gradient echo pulse sequence in the ventricular short-axis planes acquired 15 to 20 min after injection of 0.2 mmol/kg of gadopentetate dimeglumine (Magnevist; Bayer HealthCare, Tarrytown, NY, USA).
Peak regional radial and circumferential strain were assessed using feature tracking analysis (CVI42, Calgary, Canada) on steady-state free precession ventricular images in the ventricular short-axis plane. Open contours of the endocardium and epicardium were manually drawn to exclude the conduit insertion site and adjusted as needed to ensure accurate tracking. The contour was automatically divided into 12 equal segments. Segments were labeled in a clockwise fashion with segment 1 directly anterior and superior to the conduit insertion site and segment 12 posterior and inferior to the conduit (Fig. 2A). For CMR studies performed after RVPAC takedown, a closed contour technique was used with reference points at the presumed site of RVPAC insertion to keep the labeled segments consistent. Peak segmental circumferential and radial strain measurements were calculated (Figs. 2B and 2C). Circumferential strain represents fiber shortening and has a negative value. Therefore, more negative circumferential strain indicates greater shortening and is characterized as “better” (vs. “worse”) circumferential strain throughout the article. More positive radial strain is consistent with greater myocardial thickening and is referred to as “better” (vs. “worse”) radial strain.
Figure 2: Myocardial contour and feature tracking techniques
Panel A: Open contours of endocardium and epicardium with segments labeled in a clockwise fashion. Segments 1, 2, 11, and 12 represent segments of interest for comparison between conduit types. Panel B: Feature tracking analysis with mesh overlay. Panel C: Peak circumferential strain (dark blue represents better strain).
2.6 Ventricular Size and Function
Ventricular volumes and function were analyzed by manual tracing of endocardial and epicardial borders on each short-axis steady-state free precession cine slice at end-diastole and end-systole. The Simpson method was applied to calculate end-diastolic volume (EDV), end-systolic volume (ESV), stroke volume (SV), ejection fraction, and ventricular mass. To account for variations in body size, EDV, ESV, SV, and ventricular mass were indexed to the body surface area (BSA) raised to the 1.3 power. This method was applied based on studies that demonstrate that volumetric parameters are best adjusted with this transformation due to a nonlinear relationship between ventricular volumes and BSA [16,17].
The primary outcomes were peak radial and circumferential strain of the four segments most proximal to the RVPAC (regions 1, 2, 11, and 12 in Fig. 2A). Additional secondary outcomes included the presence of LGE at the RVPAC site and transplant-free survival. For survival analysis, follow-up was measured from the date of Stage I operation to death, transplant, or the last known follow-up date.
Categorical data were summarized as number (frequency) and compared using Fisher exact test. Continuous data were summarized as median (25th percentile, 75th percentile) and compared using Mann-Whitney U test. Multivariable linear regression analysis was used to assess the association of clinical variables with peak segmental radial and circumferential strain. Cox regression analysis was used to identify independent predictors for transplant-free survival. Univariate predictors with a p value < 0.2 were included in the multivariable stepwise model selection. The multivariate model was determined using Akaike information criterion. A two-sided p value < 0.05 was considered statistically significant for all tests. Statistical analyses were performed using R version 4.0.3 (R Foundation for Statistical Computing, Vienna, Austria).
The analysis included 71 CMR examinations in 61 patients (30 patients with traditional RVPAC and 31 patients with ring-reinforced RVPAC). There were 45 CMR examinations performed prior to the Stage II operation and 26 CMR examinations prior to the Stage III operation. Ten patients had CMR examinations performed during both periods.
Baseline characteristics of the cohort are shown in Table 1. Patients underwent Stage I operation at a median age of 5 days (4, 7). Pre-Stage II CMR was performed at a median age of 4.6 months (4.0, 5.2) and post-Stage II CMR was performed at a median age of 27 months (22, 30). Prior to Stage I operation, 4 patients (7%) had moderate or greater tricuspid regurgitation and 5 patients (8%) had moderate or greater right ventricular systolic dysfunction by echocardiography. A total of 13 patients (21%) required RVPAC intervention. There was no significant difference in clinical or demographic characteristics between traditional and ring-reinforced RVPAC groups.
Table 2 compares CMR parameters between patients with traditional and ring-reinforced RVPAC. Prior to Stage II operation, patients that received a ring-reinforced RVPAC had better peak radial strain (23.6% [13.9, 39.2] vs. 13.3% [9.5, 18.4], p = 0.005) and better peak circumferential strain (−16.0% [−10.8, −22.0] vs. −10.5% [−7.9, −13.5], p = 0.004) in the superior segment most proximal to the RVPAC (segment 1) than patients who received a traditional RVPAC. On multivariable linear regression analysis, the presence of a ring-reinforced RVPAC was independently associated with better peak radial strain in segment 1 (coefficient 13.2, p = 0.001) and segment 2 (coefficient 6.4, p = 0.03) and better peak circumferential strain in segment 1 (coefficient −6.4, p < 0.001) and segment 2 (coefficient −3.1, p = 0.04). There was no difference in peak radial or circumferential strain between the two groups in the two most proximal segments inferior to the RVPAC (segments 11 and 12). A traditional RVPAC was associated with increased ventricular massi (86 vs. 67 g/BSA1.3, p = 0.02). There was no significant difference in EDVi, ESVi, SVi, or ejection fraction between the two groups. LGE data was available in 34 (76%) patients. Of these, 33 patients (97%) had LGE at the RVPAC site.
CMR parameters between patients with traditional and ring-reinforced RVPAC prior to the Stage III operation are displayed in Table 3. Patients that received a ring-reinforced RVPAC had better peak radial strain in segment 1 (24.6% [18.4, 30.2] vs. 13.4% [11.2, 18.0], p = 0.008) and segment 2 (18.6% [15.7, 27.8] vs. 10.4% [8.5, 12.0], p < 0.001) than patients that received a traditional RVPAC. Peak circumferential strain was also better in the patients that received a ring-reinforced RVPAC compared to those that received a traditional RVPAC in segment 1 (−16.3% [−13.3, −18.8] vs. −10.5% [−9.1, −13.1], p = 0.006) and segment 2 (−13.3% [−11.9, −17.7] vs. −8.6% [−7.1, −9.6], p < 0.001). On multivariable linear regression analysis, a ring-reinforced RVPAC was associated with better peak radial strain in segment 1 (coefficient 8.8, p = 0.006), segment 2 (coefficient 9.8, p = 0.002), and segment 11 (coefficient 7.8, p = 0.02) and better peak circumferential strain in segment 1 (coefficient 4.6, p = 0.006), segment 2 (coefficient −5.6, p = 0.001), and segment 11 (coefficient −3.8, p = 0.02). There was no difference in EDVi, ESVi, SVi, massi, or ejection fraction between the two groups. LGE data was available in all patients, with 25 patients (96%) demonstrating LGE at the prior RVPAC site.
Additional comparison was made to assess differences in strain between segments within each group. Segment 1 was chosen as the reference for this comparison because it was the segment with the largest difference in strain between segments. Table S1 demonstrates the peak radial and peak circumferential strain for all segments in patients prior to the Stage II operation. Patients that had a traditional RVPAC had significantly worse peak radial strain in segment 1 than in segment 4. Patients that had a ring-reinforced RVPAC had worse peak radial and peak circumferential strain in segment 1 than in segment 10 and significantly better peak radial and circumferential strain in segment 1 compared to segment 12. There were no other differences in strain among either group. Table S2 shows the peak radial and peak circumferential strain in each segment in patients prior to the Stage III operation. Patients that received a traditional RVPAC had significantly worse peak radial and peak circumferential strain in segment 1 compared to segments 5, 6, 7, 8, 9, and 10. Patients that underwent placement of a ring-reinforced RVPAC had significantly worse peak radial and circumferential strain in segment 1 compared to segment 10 and significantly better peak radial and circumferential strain in segment 1 than in segment 12.
Over a median follow-up period of 6.3 years (4.1, 12.4) after the Stage I operation, 12 patients (19.7%) had the composite outcome, with 7 deaths and 5 patients undergoing heart transplantation. Table 4 shows independent predictors of the composite outcome on multivariate Cox regression analysis. Moderate or greater tricuspid regurgitation prior to Stage I operation, history of extracorporeal membrane oxygenation support or cardiopulmonary resuscitation, and need for RVPAC intervention were associated with decreased transplant-free survival after Stage I operation. RVPAC type and peak segmental radial and circumferential strain were not associated with death or transplant. The remaining clinical and CMR parameters were not significantly associated with the composite outcome.
This study compared regional right ventricular mechanics in patients with functional single right ventricles after the Stage I operation with either a traditional or ring-reinforced RVPAC. We found that a ring-reinforced RVPAC was associated with better peak radial and circumferential strain in segments adjacent to the RVPAC insertion site prior to both the Stage II and Stage III operations. LGE was present at the RVPAC insertion site in almost all patients regardless of conduit type. RVPAC type and regional myocardial deformation parameters were not associated with medium-term transplant-free survival. To our knowledge, this study describes the first analysis of regional myocardial deformation using feature tracking CMR in patients with functional single ventricles.
Previous analyses of the ring-reinforced RVPAC have largely focused on its impact on the need for conduit-related interventions. Baird et al. described lower rates of conduit complications and interventions with a ring-reinforced RVPAC [9]. A later study from Bentham et al. failed to re-demonstrate a reduction in conduit interventions but showed decreased interventions of any type at 12 months of age and improved pulmonary artery growth [8]. Our study similarly did not find any difference in rates of RVPAC intervention between the two groups (p = 0.53), although we observed lower rates of conduit intervention in patients with a traditional RVPAC compared to the two prior studies (17% vs. 41% and 36%) [8,9].
The improved regional right ventricular myocardial deformation in segments adjacent to the RVPAC site in patients with the ring-reinforced RVPAC may carry important implications for understanding the relationship between RVPAC insertion and right ventricular function. The need for a right ventriculotomy at the time of RVPAC insertion has elicited concerns regarding the potential for dysfunction of the right ventricular anterior wall. Impaired regional deformation after Stage 1 Norwood with RVPAC has been previously described by echocardiographic indices. Menon et al. described decreased strain in the anterior wall by velocity-vector imaging at the time of the Stage II operation and Fontan [18]. Meanwhile, an analysis by Pediatric Heart Network of 275 patients in the Single Ventricle Reconstruction Trial demonstrated a trend towards worse circumferential strain rate in the anterior wall (p = 0.07) in patients with an RVPAC using speckle-tracking echocardiography [19]. We found that patients with a ring-reinforced RVPAC had improved peak radial and circumferential strain in segments adjacent to the RVPAC site and that the strain in these segments were not as significantly reduced compared to segments further from the RVPAC site prior to the Stage III operation. These findings suggest that the dunked insertion technique with a limited ventriculotomy may mitigate the degree of regional ventricular dysfunction, which may progress over time. These regional differences may also represent early changes that precede declines in global right ventricular function. Additional follow-up is needed to evaluate the progression of right ventricular mechanics in this population after Fontan palliation.
The high prevalence of LGE at the RVPAC insertion site in our study supports the hypothesis that the right ventriculotomy leads to myocardial fibrosis that serves as a substrate for regional dysfunction. We observed similar prevalence of LGE at the RVPAC insertion site regardless of RVPAC type, which likely reflects that the more limited ventriculotomy involved in placement of the ring-reinforced RVPAC still incurs fibrosis on the local myocardium. Quantitative assessment of the extent of LGE has been shown to correlate with myocardial performance in patients with functional single ventricles [14] and may be helpful to further clarify the relationship between the right ventriculotomy, myocardial fibrosis and regional ventricular mechanics. The incidence of ventricular arrhythmias in this population may also serve as an important indirect clinical marker of myocardial fibrosis in longitudinal follow-up.
Our analysis did not find any difference in peak strain along the anterior wall segments inferior to the RVPAC (segments 11 and 12). These results run counter to the expectation that there would be symmetrically impacted myocardial function around the RVPAC insertion site. The presence of sternal wires is known to compromise the imaging quality of anterior structures and create metal artifact in steady-state free precession sequences [20]. We hypothesize that metal artifact from sternal wires immediately adjacent to these segments impeded the ability to accurately measure strain using feature tracking and resulted in underestimated peak radial and circumferential strain. Feature tracking by cine computed tomography or echocardiography may be less sensitive to this type of artifact.
Moderate or greater tricuspid regurgitation prior to the Stage I operation, history of extracorporeal membrane oxygenation support or cardiopulmonary resuscitation, and need for RVPAC intervention were identified as independent predictors of decreased transplant-free survival after Stage I operation. These findings are consistent with previously published data from the Single Ventricle Reconstruction Trial [4]. Notably, no CMR parameters were associated with mortality following Stage I operation. These data stand in contrast to analyses in the post-Fontan population in which CMR-derived ventricular end-diastolic volume and global circumferential strain predict survival [15,21]. This difference likely reflects different causes of mortality in infants in the interstage and perioperative periods when compared to adults that have already completed Fontan palliation.
Our study introduces feature tracking CMR as a potential tool for the assessment of regional ventricular function in patients with functional single ventricles. As CMR has emerged as a widely adopted mode of noninvasive surveillance in patients with functional single ventricles, feature tracking will enable clinicians to quantify regional ventricular function. These data may compliment standard measurements of global ventricular function and provide subtle clues to early deleterious changes that originate from the right ventriculotomy. Future study is needed to determine the long-term impact of RVPAC conduit type on the progression of regional dysfunction.
The single-center, retrospective study design limits the ability to make generalizable conclusions about the impact of RVPAC type on regional ventricular strain. Our study population of patients that underwent CMR may not be representative of all patients with functional single ventricles. For example, the presence of a pacemaker or implantable cardiac defibrillator remains a relative contraindication for CMR [22]. Patients with ventricular dysfunction may also undergo more frequent CMR surveillance and be overrepresented in the cohort. This study only included patients with functional single right ventricles and may not be generalizable to patients with single left ventricles given the different mechanics of left ventricular deformation. Finally, artifact from sternal wires may have impacted the ability to accurately measure regional strain in the anterior regions of interest in this study.
In conclusion, a ring-reinforced RVPAC is associated with better peak regional radial and circumferential strain along the anterior free wall after the Stage I operation in patients with functional single right ventricles. Additional longitudinal study is needed to assess the impact of the ring-reinforced RVPAC on long-term regional function and clinical outcomes in patients following Fontan palliation.
Authorship: The authors confirm contribution to the paper as follows: study conception and design: B Zielonka, RH Rathod; data collection: B Zielonka, RH Rathod; analysis and interpretation of results: B Zielonka, EG Elia, RH Rathod; draft manuscript preparation: B Zielonka, RH Rathod; critical manuscript revision: B Zielonka, DM Harrild, SJ Ghelani, EG Elia, CW Baird, AJ Powell, RH Rathod. All authors reviewed the results and approved the final version of the manuscript.
Availability of Data and Materials: The data and materials used in this study are available on reasonable request to the corresponding author.
Funding Statement: This study was supported by Evan’s Heart Fund (RH Rathod).
Conflicts of Interest: The authors declare that they have no conflicts of interest to report regarding the present study.
References
1. Rychik, J., Szwast, A., Natarajan, S., Quartermain, M., Donaghue, D. D. et al. (2010). Perinatal and early surgical outcome for the fetus with hypoplastic left heart syndrome: A 5-year single institutional experience. Ultrasound in Obstetrics and Gynecology, 36(4), 465–470. DOI 10.1002/uog.7674. [Google Scholar] [CrossRef]
2. Zielonka, B., Snarr, B. S., Liu, M. Y., Zhang, X., Mascio, C. E. et al. (2019). Resource utilization for prenatally diagnosed single-ventricle cardiac defects: A Philadelphia fetus-to-Fontan cohort study. Journal of the American Heart Association, 8(11), e011824. DOI 10.1161/JAHA.118.011284. [Google Scholar] [CrossRef]
3. Ohye, R. G., Sleeper, L. A., Mahony, L., Newburger, J. W., Pearson, G. D. et al. (2010). Comparison of shunt types in the Norwood procedure for single-ventricle lesions. New England Journal of Medicine, 362(21), 1980–1992. DOI 10.1056/NEJMoa0912461. [Google Scholar] [CrossRef]
4. Newburger, J. W., Sleeper, L. A., Gaynor, W. J., Hollenbeck-Pringle, D., Frommelt, P. C. et al. (2018). Transplant-free survival and interventions at 6 years in the SVR trial. Circulation, 137(21), 2246–2253. DOI 10.1161/CIRCULATIONAHA.117.029375. [Google Scholar] [CrossRef]
5. Graham, E. M., Atz, A. M., Bradley, S. M., Scheurer, M. A., Bandisode, V. M. et al. (2007). Does a ventriculotomy have deleterious effects following palliation in the Norwood procedure using a shunt placed from the right ventricle to the pulmonary arteries? Cardiology in the Young, 17(2), 145–150. DOI 10.1017/S1047951107000133. [Google Scholar] [CrossRef]
6. Newburger, J. W., Sleeper, L. A., Frommelt, P. C., Pearson, G. D., Mahle, W. T. et al. (2014). Transplantation-free survival and interventions at 3 years in the single ventricle reconstruction trial. Circulation, 129(20), 2013–2020. DOI 10.1161/CIRCULATIONAHA.113.006191. [Google Scholar] [CrossRef]
7. Sano, S., Huang, S. C., Kasahara, S., Yoshizumi, K., Kotani, Y. et al. (2009). Risk factors for mortality after the Norwood procedure using right ventricle to pulmonary artery shunt. Annals of Thoracic Surgery, 87(1), 178–186. DOI 10.1016/j.athoracsur.2008.08.027. [Google Scholar] [CrossRef]
8. Bentham, J. R., Baird, C. W., Porras, D. P., Rathod, R. H., Marshall, A. C. (2015). A reinforced right-ventricle-to-pulmonary-artery conduit for the stage-1 Norwood procedure improves pulmonary artery growth. Journal of Thoracic and Cardiovascular Surgery, 149(6), 1502–1508. DOI 10.1016/j.jtcvs.2015.02.046. [Google Scholar] [CrossRef]
9. Baird, C. W., Myers, P. O., Borisuk, M., Pigula, F. A., Emani, S. M. (2015). Ring-reinforced Sano conduit at Norwood stage I reduces proximal conduit obstruction. Annals of Thoracic Surgery, 99(1), 171–179. DOI 10.1016/j.athoracsur.2014.08.078. [Google Scholar] [CrossRef]
10. Myers, P. O., Emani, S. M., Baird, C. W. (2016). Ring-reinforced Sano right ventricular to pulmonary artery conduit at Norwood stage I. Multimedia Manual of Cardiothoracic Surgery, 2016, mmv038. DOI 10.1093/mmcts/mmv038. [Google Scholar] [CrossRef]
11. Schreiber, C., Kasnar-Samprec, J., Hörer, J., Eicken, A., Cleuziou, J. et al. (2009). Enforced right ventricle-to-pulmonary artery conduit in Norwood stage I reduces proximal conduit stenosis. Annals of Thoracic Surgery, 88(5), 1541–1545. DOI 10.1016/j.athoracsur.2009.07.081. [Google Scholar] [CrossRef]
12. Khoo, N. S., Smallhorn, J. F., Kaneko, S., Myers, K., Kutty, S. et al. (2011). Novel insights into RV adaptation and function in hypoplastic left heart syndrome between the first 2 stages of surgical palliation. JACC: Cardiovascular Imaging, 4(2), 128–137. DOI 10.1016/j.jcmg.2010.09.022. [Google Scholar] [CrossRef]
13. Petko, C., Uebing, A., Furck, A., Rickers, C., Scheewe, J. et al. (2011). Changes of right ventricular function and longitudinal deformation in children with hypoplastic left heart syndrome before and after the Norwood operation. Journal of the American Society of Echocardiography, 24(11), 1226–1232. DOI 10.1016/j.echo.2011.08.016. [Google Scholar] [CrossRef]
14. Rathod, R. H., Prakash, A., Powell, A. J., Geva, T. (2010). Myocardial fibrosis identified by cardiac magnetic resonance late gadolinium enhancement is associated with adverse ventricular mechanics and ventricular tachycardia late after Fontan operation. Journal of the American College of Cardiology, 55(16), 1721–1728. DOI 10.1016/j.jacc.2009.12.036. [Google Scholar] [CrossRef]
15. Rathod, R. H., Prakash, A., Kim, Y. Y., Germanakis, I. E., Powell, A. J. et al. (2014). Cardiac magnetic resonance parameters predict transplantation-free survival in patients with Fontan circulation. Circulation: Cardiovascular Imaging, 7(3), 502–509. [Google Scholar]
16. Sluysmans, T., Colan, S. D. (2005). Theoretical and empirical derivation of cardiovascular allometric relationships in children. Journal of Applied Physiology, 99(2), 445–457. DOI 10.1152/japplphysiol.01144.2004. [Google Scholar] [CrossRef]
17. Gutgesell, H. P., Rembold, C. M. (1990). Growth of the human heart relative to body surface area. The American Journal of Cardiology, 65(9), 662–668. DOI 10.1016/0002-9149(90)91048-B. [Google Scholar] [CrossRef]
18. Menon, S. C., Minch, L., Casper, C., Puchalski, M., Hawkins, J. A. et al. (2011). Regional myocardial dysfunction following Norwood with right ventricle to pulmonary artery conduit in patients with hypoplastic left heart syndrome. Journal of the American Society of Echocardiography, 24(8), 826–833. DOI 10.1016/j.echo.2011.05.008. [Google Scholar] [CrossRef]
19. Hill, G. D., Frommelt, P. C., Stelter, J., Campbell, M. J., Cohen, M. S. et al. (2015). Impact of initial Norwood shunt type on right ventricular deformation: The single ventricle reconstruction trial. Journal of the American Society of Echocardiography, 28(5), 517–521. DOI 10.1016/j.echo.2015.01.018. [Google Scholar] [CrossRef]
20. Olivieri, L. J., Cross, R. R., O’Brien, K. E., Ratnayaka, K., Hansen, M. S. (2015). Optimized protocols for cardiac magnetic resonance imaging in patients with thoracic metallic implants. Pediatric Radiology, 45(10), 1455–1464. DOI 10.1007/s00247-015-3366-0. [Google Scholar] [CrossRef]
21. Ghelani, S. J., Colan, S. D., Azcue, N., Keenan, E. M., Harrild, D. M. et al. (2018). Impact of ventricular morphology on fiber stress and strain in Fontan patients. Circulation Cardiovascular Imaging, 11(7), e006738. DOI 10.1161/CIRCIMAGING.117.006738. [Google Scholar] [CrossRef]
22. Levine, G. N., Gomes, A. S., Arai, A. E., Bluemke, D. A., Flamm, S. D. et al. (2007). Safety of magnetic resonance imaging in patients with cardiovascular devices: An American Heart Association scientific statement from the committee on diagnostic and interventional cardiac catheterization, council on clinical cardiology, and the council on cardiovascular radiology and intervention. Circulation, 116(24), 2878–2891. [Google Scholar]
Cite This Article
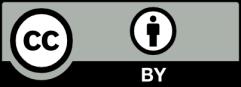