Open Access
ARTICLE
Addressing the Issues and Developments Correlated with Envisaged 5G Mobile Technologies: Comprehensive Solutions
Energy Engineering Department, Duhok Polytechnic University, Duhok, 42001, Iraq
* Corresponding Author: Idrees Sarhan Kocher. Email:
(This article belongs to the Special Issue: Artificial Intelligence, Big Data, Sensors and 5G for Smart Cities)
Computers, Materials & Continua 2023, 77(2), 2205-2223. https://doi.org/10.32604/cmc.2023.039392
Received 26 January 2023; Accepted 24 May 2023; Issue published 29 November 2023
Abstract
Fifth Generation (5G) communications are regarded as the cornerstone to household consumer experience improvements and smart manufacturing revolution from the standpoint of industries' objectives. It is anticipated that Envisaged 5G (E5G) mobile technology would be operational in certain developed countries by 2023. The Internet of Things (IoTs) will transform how humans live when combined with smart and integrated sensing devices, such as in-home sensing devices. Recent research is being carried out all over the world to produce a new technique that can be crucial in the success of the anticipated 5G mobile technology. High output, reduced latency, highly reliable, greater scalability, high performance, capacity, bandwidth efficiency, virtual open-air transmission, and efficient energy mobile wireless communications are all being investigated currently. In this work, a comprehensive path for addressing the difficulties and developments associated with 5G mobile technology is provided. The debate and description of a complete analysis of current situations, certain characteristics and prospective scenarios, important technologies, problems and advances, and spectrum allocation of envisioned 5G mobile technologies are provided. Furthermore, this paper analyzes the most notable elements of 5G mobile technology, such as Cognitive Radio (CR), flexibility, accessibility, and cloud-based service offers, which will assure 5G mobile technology’s dominance as the main protocol for international communication. Eventually, this paper provides a method for integrating CR with current wireless communication systems, the necessity for further evolution of the E5G network, and the need for comprehensive consideration of architecture evolution and function enhancement to enhance the E5G mobile technologies.Keywords
One of the technological advances that will transform the nature of engineering communication is 5G mobile communication. As a result, for the effective deployment of the Envisaged 5G (E5G), it is critical to analyze its demands, problems, benefits, and drawbacks, among other things. Over the preceding two decades, cellular communication technology has rapidly expanded over the world, moving from Global System for Mobile Communications (GSM) in 2G towards Long Term Evolution-Advanced (LTE-A) adopted by 4G. The main driving force is the requirement for larger capacity and less delay. Throughput is the real rate of data transport, whereas latency is mostly determined by the computational efficiency of each node that a data flow passes through. Along with throughput-related performance improvements, associated factors including inter-channel interference, jitter, connectivity, scalability, power efficiency, and network compatibility have been factored in when designing new mobile technologies. Higher network speeds and better download speeds enabled real-time video conversations as technology improved transferring from GSM in 2G towards Universal Mobile Telecommunication System (UMTS) considered in 3G. LTE and its follow-up LTE-A provided increased capacity of the network and reduced application–server access latency, allowing tri-traffic such as data, voice, and video to be accessed wirelessly at any time and from any location. The 4G is the true definition of mobile broadband. The 5G mobile technology is projected to improve mobile networks’ scalability, connection, and energy efficiency, as well as their data transmission speed. Mobile communication has fundamentally altered peoples’ lifestyles over the past few decades, but people have continued to seek higher-performance mobile communication devices. The smartphone communications network has begun a new phase of advancement, such as the E5G mobile communication system stage, to acclimate to the conceptual environments of the E5G technologies with ongoing wide support, large hotspots, and large links with low consumption, reduced latency and highly reliable [1–11].
The key limitations for the advancement of digital transceivers are now spectrum resources, energy consumption, and deployment costs as a result of the mobile communication system’s response to a variety of service requirements and increasing speed requirements. In addition, the E5G mobile communication system features traits like dynamic and heterogeneousness that together present significant difficulties for wireless resource management. So, the recent research focuses on the field of wireless communication is the problem of wireless resource management in the context of E5G technologies [12–17].
By 2023, over 49 billion objects are expected to be connected to the global IP network, posing a huge problem [18]. In a world that is fully connected, remote control of devices and essential business machinery would be available with minimal latency through a dependable 5G network. Mobile devices will be able to manage machinery in real-time, making the IoTs more accessible to everyone. Last but not least, to attain a sustainable green future, fewer energy-starving nodes in the network will be required.
Both 3G and 4G mobile communication systems use the current techniques, such as Code Division Multiple Access (CDMA) and Orthogonal Frequency Division Multiplexing (OFDM). Terms like excessive power consumption and Inter Symbol Interference (ISI) are two of CDMA’s drawbacks [19]. CDMA was employed in 3G systems before being superseded by OFDM, which has various advantages such as simplicity of installation, resistance to interference, and high data rate. However, OFDM has several drawbacks, such as the need for a Cyclic Prefix (CP) and a broadside lobe that restricts spectrum use. Furthermore, one of the biggest challenges associated with OFDM is thought to be the Peak Average Power Ratio (PAPR), reducing the performance and efficiency of non-linear OFDM amplifiers significantly. In 4G Wi-max, for example, the cyclic prefix causes a 10% reduction in bandwidth. As a result, OFDM is unlikely to be considered for the forthcoming E5G network. As a result, among several improved modulation schemes, Filter Band Multi Carrier (FBMC) is regarded as the most promising alternative for the E5G network. As a result of the employment of FBMC, the system’s spectral efficiency and capacity rise.
Nowadays, experts predict that combining 5G technologies with Cognitive Radio (CR) would enable communication to be accessed at anytime, anywhere, and by anybody. Furthermore, CR offers a dynamic spectrum-sharing technique that aids mostly in the resolution of the spectrum problem that much of the community is experiencing [20].
The spectral ranges 1 (FR1) and 2 (FR2) make up the 5G New Radio (NR) air interface of the E5G networks. The FR2 employs frequency bands in the millimeter (mm) wave range, whereas the FR1 employs sub-6 GHz frequency bands. The WLAN 5-GHz band (5150–5825 MHz) employs bands from 5 to 6 GHz. Bands n77 (3300–4200 MHz), n78 (3300–3800 MHz), and n79 (4400–5000 MHz) make up the prospective new spectrum for 5G NR (FR1) services (4400–5000 MHz). Several nations have allotted this new spectrum for 5G sub-6 GHz transmission as provided in [21].
As a result, the current study suggests that fast throughput, reduced latency, highly reliable, higher scalability, and energy efficiency are the most significant characteristics in the description of E5G technologies. Eventually, a complete analysis of possible alternatives for the anticipated 5G mobile technology is offered to improve wireless connection in rural regions and to create a truly wireless future free of existing wireless barriers.
The structure of this article is as follows: Section 2 provides previous works. Section 3 presents future and futuristic scenarios of E5G Mobil technologies. Section 4 proposes the E5G mobile key technologies. Developments towards E5G mobile technologies are presented in Section 5. While Section 6 proposes the E5G mobile technologies spectrum allocation, and finally, in Section 7 conclusions and possible future works are discussed.
Reference [22] described and discusses the E5G problems, covering hardware and software. Because the E5G technology is still an uncertain framework, the technology that will be employed in it is still up in the air, resulting in a variety of 5G advancements. Reference [23] went over the many important technologies that might be employed in the E5G.
A comprehensive millimeter wave hardware system for both frequencies of 28 and 38 GHz is proposed and built in this study [24], which may be processed in steerable directional antennas just in both base and mobile stations.
The relevance of cloud computing technologies in achieving a flexible E5G radio access network is discussed in this paper. Because of the growing number of wireless devices and sensors, it is considered one of the most difficult challenges [25].
This article plans and develops a cost-effective 5G network using a hybrid of green communication and programming, with an emphasis on cognitive signaling, energy-efficient design, full duplex radio, and invisible base stations [26].
The author of this research explores the many technological devices and networks that contribute to the advancement of E5G mobile technologies [27].
The authors suggest different network architectures for indoor and outdoor communication systems in this paper. Some of the important technologies are also mentioned in [28], such as visible light communication and enormous Multiple-input, Multiple-output (MIMO).
Device-centric architecture, millimeter wave, massive MIMO, smart devices, and Machine to Machine (M2M) communication are five technologies highlighted in a communication magazine article that might have a revolutionary influence on the E5G design and concept [29].
Various research organizations are developing E5G standards. The biggest Framework Program 7 (FP7) 5G project is Mobile and Wireless Communications Enablers for the Twenty-twenty Information Society (METIS). The Seventh Framework Programme (FP7) is the European Union’s research and innovation program.
On 30 April 2015, METIS released its project report (Deliverable 8.4), which included high-level architectural drawings, architecture, channel conditions, about 140 technical elements, and test bed assessments, as described in [30]. They demonstrated that the FBMC may be used to construct popular for its expansive functionality interfaces. The simulation research includes assessments of anticipated 5G Key Performance Indicators (KPIs) such as traffic volume per consumer, traffic volume per location, mean consumer data rate during busy hours, and real customer data rates. Surprisingly, the simulations revealed a latency of the Radio Access Network (RAN) of less than 1 ms. These groups are presently working on the METIS-II project, which calls for a comprehensive E5G RAN concept, interactive 5G RAN evaluation, and global building consensus across all standards organizations.
The European Commission, communications carriers, manufacturers, and academics launched the 5G infrastructure Public Private Partnership (5G-PPP). The E5G concept may be summarized as follows: in ten years, Information Technology (IT) and telecoms will be combined in a single, global, extraordinarily large infrastructure having merging abilities in both mobile and fixed services, as described in.
The 5th Generation Non-Orthogonal Waveforms for Asynchronous Signaling (5GNOW) has investigated ultra-low latency, integrated frame architectures, ultra-high resilience, and suitable waveforms for E5G. The latest launch depicts Gabor signaling, in which the enlarged signal is the sum of the prototype window’s scaled time–frequency transitions. The Gabor expands coefficients to determine the necessary weights. The Short Term Fourier Transform (STFT) as in [31] is also used to obtain time-frequency plane information of an underlying signal.
Enhanced Multicarrier Technology for Professional Ad-Hoc and Cell-Based Communications (EMPhAtiC) is investigating MIMO transmission, the construction of an extremely configurable filter bank, equalization, and multi-hop or relay-based data transmission with asynchronicity. In a recent project outcome [32], different MIMO transceiver approaches for FBMC within frequency-selective channels were suggested.
The Network of Excellence in Wireless Communications (NEWCOM#) group is focusing on high-end wireless networking alternatives such as identifying the tight maximum limit of wifi networks, adaptive multi-hop connections, and efficient energy communication. One of the project’s most recent outputs as in [33] is unique research on Cloud-RAN, wireless transmission, both 4G and 5G technology collaboration employing spectrum overlay, multi-hop code, and location via dispersed antennas.
Rappaport et al. at NYU WIRELESS are conducting Millimeter Wave (Mm-Wave) studies for E5G technologies, having tested the Mm-Wave propagation model and route losses in two towns: New York and Austin as in [24].
At 5G Innovation Center (5GIC), the UK’s sole research organization devoted to E5G research, finally launched a stunning milestone in wireless speed gain like a wireless Point-to-Point (P2P) transmission speed of 1 Tbps. For futuristic purposes, the staff of 5GIC is investigating ultra-low latency-sensitive application services as in [34].
As part of the GIGA 5G project, the Korean Electronics and Telecommunications Research Institute (ETRI) is working on telephony for Device to Device (D2D), improving dependability and the Mobile Hotspot Network (MHN) protocol stack as in [35]. The Republic of Korea’s 5Gforum is also looking for new ideas and market studies for the upcoming standard. The 4G-Americas is the Americas’ “voice of 5G” in some other areas of the globe. It successfully created white papers on E5G development and suggestions “In October 2015”, with a concentration on Information-Centric Networking (ICN).
Other research organizations exist in addition to the ones listed above. The author of this work briefly noted a handful of them and their contributions in his survey.
3 Features and Futuristic Scenarios of E5G Mobile Technologies
In the following subsections, the current roadmap contributes, proposes several expected features, and provides futuristic scenarios included within E5G mobile technologies.
3.1 Proposed Features of E5G Mobile Technologies
Exploring the expected features for E5G technologies are summarized in the following points [36]:
• Reliable Service in Crowded Areas: Users encounter Denial of Service (DoS) as a result of the network congestion caused by the high volume of traffic. As a result, 5G was designed to provide improved service and connection in congested areas like shopping centers and rail stations.
• Network Latency: The 4G wireless communication networks have a consumer data rate of 100 Mbps which is powerful but not fast enough to meet the ever-increasing needs of users, industries, and other consumers. A data transfer rate greater than or equal to 1Gbps will be available to users in 2023.
• Devices Integration: Even while 4G mobile technologies enable a large number of low-power devices, it still falls short for several applications. As a result, the E5G promises to accommodate a large number of low-power devices, which will be effortlessly linked into widely viable 5G mobile technologies.
• Remote Service: Remote meter reading for monitoring, billing, and e-health such as telehealth, sustainable city, and surveillance cameras are some of the applications for remote service. 5G mobile technologies were envisioned to enhance these capabilities in remote areas.
• Smart Handover: The current handover scenario is completely complicated, as the delay experienced by the handover process is significant, resulting in a call drop. As a result, in the future of 5G mobile technologies, the smart handover with the least amount of delay is expected with the process switching of network functionality.
• White Spectrum Utilization: One of the main goals of 5G here is to make use of the white band spectrum, which is currently underutilized and may help address the spectrum problem to the greatest extent feasible.
• High QoS and User Capacity: Wireless traffic consumption is anticipated to rise by 40% by 2023; hence, the 5G network must be able to support the growing number of clients while maintaining the highest QoS.
• Pseudo-Outside Information Exchange: A study has shown that approximately 50% of phone traffic and 70% of message transmission originate from interior places, however net availability with functionality by interior regions is inferior to outdoor ones. As a result, E5G mobile systems are focused on pseudo-outdoor communication, in which network coverage, data rate, and other services in the inside environment are equal to those in the outdoors.
3.2 Futuristic Scenarios of E5G Mobile Technologies
Exploring the expected futuristic scenarios for E5G technologies are summarized in the following points [37]:
• It appears as today's civilization will be an Internet of Things (IoTs) linked society. The Internet of Things, the conjunction of smart and integrated wireless sensor networks with IoTs, can transform how people live. People who live in smart homes will need continual and ubiquitous mobile network access to post personal user activities with an IoT framework set, resulting in a vast reported uplink flow of information.
• In the delivery of services and commercial operations, the vast M2M interaction and critical M2M communication become important. Using technologies of Vehicular Ad-hoc Networks (VANETs) are rapidly evolving. VANETs connected with the mobile network can be worked under the VANET cloud by 2023, resulting in a good and safe transport system.
• Because as a range of Internet-connected appliances grows into the hundreds of billions in decades ahead, dumping connected traffic into unlicensed spectrum will become increasingly important for network load balancing, ensuring assured bit rates and reducing control signals. As a result, it’s critical that envisioned 5G mobile technologies function easily with crowded hybrid nets to fulfill the rising level of real data while also guaranteeing that clients have a stable network connection.
4 Proposed E5G Mobile Key Technologies
In the following subsections, the numerous projected advanced features that could perform a significant part in the development of the E5G technologies with mobile networks are reviewed.
The bandwidth is split into several sub-carriers that are perpendicular to one another in this technique. At the transmitter and receiver sides, the technology of Fast Fourier Transform (FFT) and Inverse Fast Fourier Transform (IFFT) are used, respectively. High capacity, increased information rate, and immunity to Inter Symbol Interference (ISI) are all advantages of this approach. However, OFDM is not a feasible alternative for the E5G network due to several limitations, like Peak Average Power Ratio (PAPR) and CP. Because there is a risk of noise when the symbols are so close together, CP is added between them to reduce ISI. However, the inclusion of CP leads to a reduction of bandwidth, with roughly 10% of bandwidth being wasted, resulting in a fall in spectral efficiency. The PAPR also drastically lowered the system’s performance, resulting in data addition in sub-carriers. Whereas if the system’s PAPR is 10 dB, that indicates it takes 10 watts of power to transmit 1 dB of signal, which effectively affects the system’s performance. As a result, OFDM is not a good modulating option for the E5G mobile network. As a result, researchers everywhere in the world are shopping for a suitable modulation technique that can meet the expectations of 5G mobile [38].
It is a more improved version of existing OFDM that uses filter arrays at the transmission and reception instead of CP. This method has the advantage of being more effective and providing greater efficiency over OFDM without the need for a CP. As a result, this is among the most appealing modulation formats for the next 5G mobile technology. A bank of filters is employed in this technique to convey a series of parallel data. FBMC is more suited for Television White Spectrum (TVWS) and spectrum utilization due to its frequency domain adaptability. An adequate prototype filter can be used to manage adjacent frequency leakage and localization. FBMC can readily handle channel delay spread, and fragmented spectrum accessing is also possible [39].
4.3 Universal Filtered Multi-Carrier (UFMC) Technology
This technology is built on FBMC and OFDM modulation system, which is thought to be the strongest and most innovative modulation technique for E5G. For FBMC, where each subcarrier is filtered individually, and the process of filtering conducts upon groups of sub-carriers with this approach. The method effectively decreases the side lobe, improving the system’s performance. Because it had such a short filter length, it was typically used for short burst transmission. In this process, the band is split into several sub-band then assigned to several subcarriers. At the transmitter, the signal is converted from the time domain to the frequency domain through the use of an N-point FFT process. At the receiver, IFFT is employed to transform the frequency domain to the time domain [21].
It may lead to greater usage of available bandwidth and increased system robustness. This method divides the bandwidth into many orthogonal subcarriers, similar to OFDM, and it does not need the CP. When this technology is combined with Space Time Block Codes (STBC), Spatial Division Multiplexing (SDM), and Space-Time Trellis Codes (SPTC), performance is enhanced by lowering some flaws including ISI. With MIMO methods, the co-channel interference impact is more pronounced; as a result, OFBMC has piqued researchers’ interest [22].
The capacity of this strategy is increased by delivering additional information at the temporal range. This term employs linear model approaches, in which the pulse can be delivered at a much faster rate in the temporal range (domain), resulting in orthogonal loss, ISI, and other issues, however, the information still can be retrieved using an upgraded recognition system [40].
4.6 The Technology of Cognitive Radio (CR)
The CR is known as a smart type of wireless connection that is intelligent and makes decisions based on the approach of comprehending the environment. This approach of CR can be linked to a wireless communication network for better usage. The E5G, generally termed WISDOM, integrates and links all wireless techniques, while CR adapts to and connects to all wireless techniques. When the primary user is ideal, CR successfully exploited spectral performance by allowing unlicensed (CR users) to use the free spectral range without causing any interference. This technology is a radio that examines the environment and makes judgments based on that data; in other words, it adds intelligence to the network. This technology is affected by several factors, including the idle channel, the type of data to be carried, channel occupancy, and the modulation technique used. As a result, it is vital to use software that reconfigures itself to meet the user’s requirements or a variety of criteria. A Software Defined Radio (SDR) is used by cognitive radio as in [41].
4.7 Matched Filter Detection (MFD)
In the Gaussian case, this approach is ideal since it optimizes the received signal’s SNR, providing a platform for recognition. In non-Gaussian circumstances, however, this is not ideal. In general, the matching filter prioritizes spectrum elements with the highest SNR. This necessitates a non-flat frequency response; however, in applications such as digital communications and radar, in which the fundamental shape is recorded and thus the goal is always to indicate the existence of such a signal vs. background noises, the distortion is irrelevant. The worst part about a matched filter would be that it requires knowledge of the signal being detected; else, it is indeed useless. The linear filter is responsible to increase the SNR of just input data. When the secondary user is aware of the channel information for him, the matching filter can be used. Similar to co-relation, this scheme involves convolving an unidentified signal with a filter for whom the impulse response is a mirror and time-shifted form of a standard wave [42]. Complex conjugate reverse signal defines the coefficient of matching filter detection. There are two forms of receivers:
• Non-Coherent Receiver: In this type, the received signal is a clone of the standard signal with unpredictable phase inaccuracy. The signal’s power or amplitude is used for matching filter detection.
• Coherent Receiver: When the secondary user understands the amplitude and phase of the signal source, this receiver can be used.
One of the motivations for the development of matching filter detection is the examination of the Pu signal in a specific spectrum over time. The primary matched filter is most useful in radar, intelligent radio systems, wireless communication, sonar, and other applications.
4.8 Challenges in Cognitive Radio (CR)
This section discusses the anticipated obstacles in CR. Despite the introduction and proposal of new techniques for spectrum access, spectrum access remains challenging due to the following procedure:
• Detecting the optimal spectrum is regarded as one of the most challenging processes.
• In frequency ranges where transmission and reception are dynamic, determining the primary user is exceedingly challenging, necessitating the use of a highly sensitive detector.
• When noise is mistaken for a signal, which happens all the time in the real world, false alarm detection occurs.
• Detecting the principal subscriber spectrum in a short amount of time is one of the most difficult aspects of CR.
• The hidden terminal problem is one of the most difficult problems to solve in spectrum sensing.
The CR is expected to be critical for next-generation wireless communication and should be implemented into the E5G communication system. E5G network is being rolled out across the country, to increase speed to 1 Gbps, doubling capacity by 10, and connecting everyone at any time and from anywhere. The communication system’s slogan, on the other hand, cannot be fulfilled without the introduction of the CR.
According to research, the interior area accounts for more than 50% of voice traffic and 70% of data traffic, however, the connectivity in the interior area is significantly lower than in the outside area, which is a significant issue in today’s environment where the majority of users spend their time indoors. FC is a home node base station that receives a signal from a macro cell and transmits it to a mobile device attached to it. Two varieties of FC are commercially available [43]:
• Home-Based FC: For this type, which links 6–8 mobiles with it.
• Industrial-Based FC: For this type, which links up to 15 mobiles with it.
This cell has a range of 10–50 m. FC’s back-haul connection is made using an Advance Digital Subscriber Line (ADSL) connection to an Internet service provider. This technique has several advantages, including a high data rate due to the proximity of the transmitter and receiver, enhanced battery life, and improved service quality; nevertheless, interference between the macro cell and FC, a primary concern is FC to end devices and user terminals to a macro cell. Femto Forum was founded in 2008, and its members include numerous European countries, as well as China, Japan, and India [43]. Fig. 1 depicts the FC installation.
Figure 1: The FC installation
4.10 Quadrature Amplitude Modulation (QAM) 256
Earlier, modulation was explained as the method of transmitting a signal via a carrier, but today, modulation has a different concept. The choice of modulation system affects the efficiency and performance of data transmission. As a result, a high speed can be achieved by using a dependable modulation and a better filtration technique. Each symbol in QAM-256 represents an 8-bit that is transferred, and it can create a mobility speed of 40 Mbps while also providing 33% more efficiency than QAM-64. This indicates that when QAM is used, the data rate is greatly boosted. The distortion between the two symbols, also known as ISI, is one of the drawbacks of high data rates. However, ISI can be lowered by adding an equalizer but contributes to increasing the communication system’s transmitter and receiver parts [44].
4.11 Technology of Mobile Femto Cell (MFC)
Signal coverage is occasionally missing in numerous areas or regions, resulting in poor network service. As a result, MFC that can connect to the E5G mobile network can fix this issue. The MFC is based on the FC and relay concepts used in mobile communication. MFC is a compact device that integrates easily with the operator. MFC technology may be installed on trains, cars, and other vehicles with weak signal coverage. This approach has several advantages, including increased spectrum efficiency, longer battery life, and lower signal overhead. This technology allows all data to be delivered to the macro cell over a single channel, then the data from the macro cell is subsequently added and transmitted to the FC through the downlink.
4.12 Visible Light Communication (VLC)
Data is conveyed using a light frequency of 400 to 800 Thz in this technology. VLC is a technology that combines luminosity and connectivity. In this technique, the light was employed as a signal transmitter, airflow as a channel form of media, and a photo-diode as a receiver. The light is modulated with a data stream using rapid switching Light Emitting Diodes (LEDs).
5 Developments Towards E5G Mobile Technologies
With a few tweaks, several very well ideas/plans, such as distributed computing, radio access techniques, and modulation techniques, could also be used in the E5G, in addition to several other newly invented and advanced solutions. Some of these concepts are presented in the following subsections.
First, a carrier frequency in the mm-wave spectrum in the 3–300 GHz range is used, and opportunist congestion offloading into an uncontrolled envisaged 5 GHz Wi-Fi band, to obtain a thousand-fold performance gain. The frequency range for the currently authorized cellular service is between 750 and 2600 MHz. As a result, the design of the mm-wave spectrum’s generally underutilized PHY layers is necessary. Data flow will be sped up and availability ensured with the help of MIMO Systems, traffic offloading to unlicensed spectral, cloudification of radio resources, and beam shaping. The authors of [24] investigated many ideas like the penetration characteristics, propagation behavior, and route loss of 28 and 38 GHz carriers due to urban buildings in their work. The findings of their research will be extremely beneficial in building the E5G PHY layer for mm-wave deployment. The authors of [45] devised a 5G mm-wave communication system with ultra-low latency in communication.
For E5G mobile technologies, the core network and RAN will be well-connected. High-bandwidth cable connections should be used by the networking BS, and the network structure may move from fiber to wireless mm-wave communication. As the number of linked devices increases, a typical macro-cell may become heavily overburdened with managing overheads to maintain connectivity with a sizable number of devices, such as 10,000 per cell. As a result, to support increased communications and package overhead, the architecture must be streamlined and updated. The Giga KOREA 5G proposal has reported on the effectiveness of a prospective E5G architecture using mm-wave RAN. Additionally, the authors of the study highlighted how beam methodology enables seamless transitions between various beams and included graphs and charts of various antenna array configurations for 3-dimensional beamforming. The 3-dimensional beam formation is produced by a 2D array of patch antennas. High-definition radio transmission signals produced in three dimensions and emanating from a 2D array of patch antennae enable Space Division Multiple Access (SDMA). The technical term for this is Beam Division Multiple Access (BDMA). In end devices, they used patch antenna arrays with a 2-dimensional NXM array of the radiating patches. The radio access mechanism is durable, secure, and highly dependable because it allows for rapid handoff between multiple beams. Furthermore, relay transmission is employed to overcome the mm-wave RAN’s limited coverage, and the handoff procedure needs not to be managed in the BS node instead of the core node. This resource allocation task is handled by the BS or eNB in 4G LTE. For higher QoS in LTE, many scheduling techniques have been proposed. Based on game-theoretic simulations, an intelligent resource allocation system for CR connections was suggested in [46].
5.3 Modulation Technique Better Than OFDM
The multiple access mechanism and modulation strategy utilized have a big impact on spectral efficiency. In 4G-LTE, the modulation technique and multiple access strategy are OFDM and OFDMA. The old CDMA utilized in 3G telecommunications was replaced by OFDMA. OFDMA should be able to manage higher PAPRs and the necessity for CPs to provide prevention from Inter Block Interference (IBI) in the future. Furthermore, the usability of OFDM on wide band mm-wave with the needed hardware arrangement is unknown. A comparison of FBMC, OFDM, and UFMC modulation algorithms for anticipated 5G technologies was reported in [47].
For FBMC, inherent asynchronicity here between the transmitter and the receiver poses no issues. Based on the work of [48], the group of 5GNOW is currently evaluating four wave patterns for 5G, including GFDM, UFMC, FBMC, and Bi-orthogonal Frequency Division Multiplexing (BFDM). Here is a quick comparison of the 5G waveforms based on operation and prototype points of view:
• GFDM: It can be adjusted and is adaptable, similar to Single Carrier Frequency Division Multiplexing (SCFDM) and OFDM, allowing the available bandwidth to be split into a greater number of subcarriers with narrow band frequencies or a smaller number of subcarriers with broader individual bandwidths. A block-based modulation technique is GFDM. Due to the simplicity of the transmitter and receiver, the GFDM signal is intended to reduce equalization and synchronization difficulties.
• UFMC: It is defined as a multi-carrier signaling style with orthogonal subcarriers which solves the orthogonal subcarrier loss issue at the receiving side. It is a generalization of filtered OFDM and FBMC. Subband filters are used instead of a CP. Such short-duration filters perform better for short-burst transmission than FBMC.
• FBMC: To provide flexible resource allocation for both the time and frequency domains, FBMC utilizes a preliminary burst approach. Synchronization and channel estimation will be accomplished via training sequences.
• BFDM: It replaces the orthogonality requirement (as in OFDM) of a set of transmitters and receivers with the concept of bi-orthogonality, a flexible form of orthogonality. In this system, the time-frequency characterizations of the transmitted and received pulses should be pairwise orthogonal as opposed to an individual. Compared to OFDM, it is more resilient to frequency offset in transmission.
5.4 Cloud-Based Radio Access Network RAN-as-a-Service (RANaaS)
The term RAN refers to the front haul network component. The E5G communication network may have a special ability: it’s going to be able to transmit high-definition real-time video to IoT control signals with incredibly little capacity. Though RAN includes fundamental physical aspects like massive MIMO, coding scheme, and modulation, this subsection primarily focuses on Cloud-RAN, a rapidly evolving and revolutionary topic. The whole new use of cloud services in RAN deployment is the most important component of the anticipated 5G network. The work of [49] provided evidence of the benefits of RANaaS in the areas of network longevity and power efficiency. Sustaining RAN ability on a centralized server and making it available to customers as needed is the aim of RANaaS in the cloud. To provide it, the BSs must be split into an access network unit and a basic unit resource pool, with the latter serving any cell with high traffic. To conserve energy and make reserved system capacity for the cell when needs it owing to a sudden surge in traffic, a limited number of small cells must be placed. Researchers have shown that computation energy consumption and power efficiency will be further optimized with the launch of newer cloud computing platforms and upcoming data-center servers. In addition to the RAN, the core and backbone networks can be virtual by employing cloud-based resource availability, as shown depicted schematically in Fig. 2 in [50].
Figure 2: Schematic diagram of network cloudification [50]
5.5 Energy Efficiency for E5G Technologies
The use of energy is a significant component in the widespread adoption of modern networks. Nowadays, cellular networks use much more than 0.5% of all the energy produced worldwide. As a result, one of the most significant aspects of E5G research is a decrease in energy usage from both an environmental and network maintenance perspective. A network that has been made denser by reduced cell size is surely going to need more energy. The biggest energy consumers will indeed be the retrieval of data electricity and sitting idle so the network will have a greater number of smaller cells. An E5G framework that combines software-defined MAC and network functions virtualization has been introduced very well in the literature. By combining these techniques, some approaches envision a 5G network with reduced delay and low energy consumption. In a research project, known as the 5GrEEn Project, this research concurred with the previously existing work that the conceptual separation of the data and control planes would result in a substantial contribution to an energy-flexible and effective E5G architecture. By adopting an energy-efficient and efficient Heterogeneous Network (HetNet) framework for various load circumstances and scenarios, the 5GrEEn project seeks to provide better capacity. Cloud-based services also need to be allocated carefully. As stated in [51], Anchor seems to be a cloud-based resource systematic methodology that has been suggested, implemented, and assessed.
A multilayer protocol stack is the foundation of any communication system. Reference [52] described the overall protocol stack in their 5G study. The authors proposed a global communication standard. The stack’s most notable feature is its ability to work better with an existing open-source protocol. The term Open Transport Protocol (OTP) which can be used by every user device whenever necessary was discussed by the authors. It is possible to link user equipment to many BSs. In reality, BSs might be a part of various networking.
5.7 3GPP Device-to-Device (D2D) Proximity Services
To boost cell capacity and provide various Proximity Services (ProSe), such as bus or cab availability, neighborhood retail/restaurant marketing, social network searches in a public crowd, and so on. In a cellular system, D2D connectivity is critical. Within the release-12, the 3GPP standardized LTE-Direct (LTE-D). LTE-D is a new concept that uses a licensed frequency to discover “always-on” devices in a 500-m radius in an energy-efficient and secure environment. Despite Wi-Fi Direct and Bluetooth being the most commonly used P2P near-network protocols, they have drawbacks in terms of energy usage. As a result, LTE-D has been standardized, and Mm-wave D2D connection is required for E5G technologies. In reference [53], capable mobile devices equipped with electrically steerable antennae and beam-forming technology demonstrated mm-wave D2D communication. Concurrent transmission between D2D users and the base station will not cause much interference thanks to highly directed antennae and beam-shaping technology. A network might get crowded when multiple neighbors share multimedia files. Crowd-sensing media recovery mechanisms are needed to handle this type of situation. Accessibility in rural areas, at sea, or on-air services 35000 feet over the earth’s surface is an exciting application of 5G; the above needs may be met by a satellite. For onboard beam-forming, a research endeavor minimized the delay in non-GEO satellite systems and power allocation in multi-beam satellite schemes. Reference [54] proposed that an FBMC architecture might be reused via satellite communication, making the transition between ground cellular infrastructure and satellite coverage faster and smoother.
In general, the idea as in [55,56] presented the predicted idea of the envisioned 5G network having attributes of IoT networks. Fig. 3 depicts a schematic design of the system for E5G network technologies.
Figure 3: Simplified network for E5G technology [55]
5.8 Combination of OFDM into Massive Multiple-Input, Multiple-Output (MIMO)
The outcome of combining massive MIMO with OFDM is depicted in Fig. 4. Each user transmits data to an IFFT-N points block during the uplink, which prefixes the data with a CP before sending it across the channel. CP is an end-of-block component that is appended to the beginning of the block. After the CP is eliminated, each BS antenna’s received signal is sent to the BS via an FFT N points block, and the resulting signals are then subjected to detection methods such as zero forcing or Minim Square Error (MMSE) [57].
Figure 4: OFDM massive MIMO system’s block diagram in the uplink [57]
5.9 Software-Defined Netwok (SDN)
Software-Defined Networking (SDN) can be used in IoT networks to increase quality-of-service (QoS), minimize complexity related to IoT, and obtain the best possible results [58].
6 Proposed E5G Mobile Technologies Spectrum Allocation
Customers may access the internet and other multimedia very quickly with 5G thanks to the newest technology. The LTE network is anticipated to be upgraded to a 5G network in the future. The 5G technology employs millimeter waves and an unresolved data transmission spectrum to enable faster data throughput [59]. The terminal should have the option to integrate different streams from multiple upgrades because the 5G mobiles will approach various remote innovations at the same time. For enthusiastic cell phone shoppers, 5G advancement delivers great expectations [60,61].
Spectrum allocation is among the key problems for 5G mobile networks. As a result, an alternative spectrum of E5G mobile systems is being investigated. Alternatives frequency ranges are currently being sought up to 275 GHz. Both frequencies spanning 40 and 90 GHz which are higher than 6 GHz are debated and considered. Furthermore, the E5G phone includes CR, which aids in accessing the white spectrum as in [62]. The American Voice for 5G produced a 28-page article detailing the spectrum that could be used in E5G technologies. Some of the recommended frequency ranges like 27.5–29.5 GHz, 37–40.5 GHz, 47.2–50.2 GHz, 50.4–52.6 GHz, and 59.3–71 GHz are also proposed for E5G technologies.
Based on Federal Communication Commission (FCC) has indicated the following bands that may be licensed in E5G technologies:
• Bands of 24 GHz: 24.25–24.45 GHz, 25.05–25.25 GHz.
• Bands of LMDS: 27.5–28.35 GHz, 29.1–29.25 GHz, 31–31.3 GHz.
• Bands of 39 GHz: 38.64–40 GHz.
• Bands of 37/42 GHz: 37–38.6 GHz, 42–42.5 GHz.
• Bands of 60 GHz: 57–64 GHz, 64–711 GHz.
• Bands of 70/80 GHz: 71–76 GHz, 81–86 GHz, 92–95 GHz.
The 3GPP (3rd Generation Partnership Project) has recently developed the 5G new radio (NR) air interface of 5G networks. This new radio access technology has very low latency (<1 ms) and a high data rate, 100 times faster than 4G-LTE. The spectral ranges 1 (FR1) and 2 (FR2) make up the 5G New Radio (NR) air interface of 5G networks. The FR2 employs frequency bands in the millimeter (mm) wave range, whereas the FR1 employs sub-6 GHz frequency bands. The WLAN 5-GHz band (5150–5825 MHz) employs bands from 5 to 6 GHz. Bands n77 (3300–4200 MHz), n78 (3300–3800 MHz), and n79 (4400–5000 MHz) make up the prospective new spectrum for 5G NR (FR1) services (4400–5000 MHz). Several nations have allotted this new spectrum for 5G sub-6 GHz transmission [21].
7 Conclusions and Future Works
This article presents a comprehensive assessment and roadmap of several recent existing projects in the literature aimed at developing a flexible, green, and largely dominating E5G technologies standard. In this context, this paper presents a complete assessment of future 5G mobile technologies, including important technologies, current developments in future 5G mobile technologies, future wireless communication requirements, and a new spectrum allocation for 5G wireless communication systems. The paper also discusses bandwidth shortage, which is a key worry in the telecommunications industry, and proposes an FBMC modulation technology, radio cognitive approach, and E5G mobile technologies for addressing the problem.
Validation of novel concepts offered by various academics and investigation of unused spectrums are considered the two main challenges in E5G mobile technologies. Essentially, the fundamental goal of the proposed E5G mobile technologies was to create a new wireless communication world free of current impediments, which could only be achieved if considering the integration of all existing technologies with the future technologies for the next 5G. Furthermore, a variety of essential topics were briefly covered, ranging from an upgraded OFDMA replacement to energy-efficient Device to device (D2D) research efforts. Finally, this study focuses on the 5G-advanced oriented network architecture, important technologies, and the next stage of 5G network evolution. To satisfy the demands of quick deployment of network functions and on-demand iteration, the network design will evolve with the concepts of cloud-native, edge network, and network as a service. The original internet design aims to maintain the network as straightforward as possible and provide additional peripheral capabilities that will be used in the mobile networks of the following generation.
According to this investigation, the year 2023 will usher in a revolution in the field of 5G mobile technologies and communication systems, as everyone will be able to connect from anywhere, at any time. It is also planned to give reference scenarios, requirements, and technological directions for the development of 5G-advanced networks, to motivate industry consensus and collaboratively promote the development of anticipated 5G mobile technologies.
The security of the 5G network also needs a lot of attention. In the future, several security issues that could develop in a 5G network will be examined. Shortly, soon there will also need to be the consideration and discussion of issues like energy management, quality, data management, and caching. Last but not least, numerous research projects are needed to develop simulators, testbeds, and analytical models for the 5G network.
Acknowledgement: The researcher would like to thank the Deanship of Scientific Research, Duhok Polytechnic University for funding the publication of this work.
Funding Statement: The author received no specific funding for this study.
Conflicts of Interest: The author declares that he has no conflicts of interest to report regarding the present study.
References
1. F. N. Al-Wesabi, I. Khan, S. L. Mohammed, H. F. Jameel, M. Alamgeer et al., “Optimal resource allocation method for device-to-device communication in 5G networks,” Computers, Materials & Continua, vol. 71, no. 1, pp. 1–15, 2022. [Google Scholar]
2. M. Waqas, Y. Niu, Y. Li, M. Ahmed, D. Jin et al., “A comprehensive survey on mobility-aware D2D communications: Principles, practice, and challenges,” IEEE Communications Surveys & Tutorials, vol. 22, no. 3, pp. 1863–1886, 2020. [Google Scholar]
3. M. Ahmed, Y. Li, M. Waqas, M. Sheraz, D. Jin et al., “A survey on socially aware device-to-device communications,” IEEE Communications Surveys & Tutorials, vol. 20, no. 3, pp. 2169–2197, 2018. [Google Scholar]
4. F. S. Shaikh and R. Wismuller, “Routing in multi-hop cellular device-to-device (D2D) networks: A survey,” IEEE Communications Surveys & Tutorials, vol. 22, no. 3, pp. 2622–2657, 2018. [Google Scholar]
5. M. Haus, M. Waqas, A. Y. Ding, Y. Li, S. Tarkoma et al., “Security and privacy in device-to-device (D2D) communication: A review,” IEEE Communications Surveys & Tutorials, vol. 19, no. 2, pp. 1054–1079, 2017. [Google Scholar]
6. O. Hayat, R. Ngah, S. Z. M. Hashim, M. H. Dahri, R. F. Malik et al., “Device discovery in D2D communication: A survey,” IEEE Access, vol. 7, pp. 131114–131134, 2019. [Google Scholar]
7. A. Asadi, Q. Wang and V. Mancuso, “A survey on device-to-device communication in cellular networks,” IEEE Communications Surveys & Tutorials, vol. 16, no. 4, pp. 1801–1819, 2014. [Google Scholar]
8. B. M. Elhalawany, R. Ruby and K. Wu,“ “D2D communication for enabling internet-of-things: Outage probability,” IEEE Transactions on Vehicular Technology, vol. 68, no. 3, pp. 2332–2345, 2019. [Google Scholar]
9. I. Loannou, V. Vassiliou, C. Christophorou and A. Pitsillides, “Distributed artificial intelligence solution for D2D communication in 5G networks,” IEEE Systems Journal, vol. 14, no. 3, pp. 4232–4241, 2020. [Google Scholar]
10. P. Pawar, A. Trivedi and M. K. Mishra, “Outage and ASE analysis for power controlled D2D communication,” IEEE Systems Journal, vol. 14, no. 2, pp. 2269–2280, 2020. [Google Scholar]
11. F. Qamar, M. U. A. Siddiqui, M. H. D. Hinidia, R. Hassan and Q. N. Nguyen, “Issues, challenges, and research trends in spectrum management: A comprehensive overview and new vision for designing 6G networks,” Electronics, vol. 9, no. 9, pp. 1–124, 2019. [Google Scholar]
12. Y. R. B. Al-Mayouf, N. F. Abdullah, O. A. Mahdi, S. Khan, M. Ismail et al., “Real-time intersection based segment aware routing algorithm for urban vehicular networks,” IEEE Transactions on Intelligent Transportation Systems, vol. 19, no. 7, pp. 2125–2141, 2018. [Google Scholar]
13. M. K. Hasan, M. M. Ahmad, A. H. A. Hashim, A. RAzzaque, S. Islam et al., “A novel artificial intelligence- based timing synchronization scheme for smart grid applications,” Wireless Personal Communications, vol. 114, no. 2, pp. 1067–1087, 2019. [Google Scholar]
14. S. N. Makhadmeh, A. T. Khader, M. A. Al-Betar, S. Naim, A. K. Abasi et al., “A novel hybrid grey wolf optimizer with a min-conflict algorithm for power scheduling problem in a smart home,” Swarm and Evolutionary Computation, vol. 60, no. 2, pp. 1–17, 2021. [Google Scholar]
15. N. Nurelmadina, M. K. Hasan, I. Memon, R. A. Saeed, K. A. Z. Ariffin et al., “A systematic review on cognitive radio in low power wide area network for industrial IoT applications,” Sustainability, vol. 13, no. 1, pp. 1–21, 2021. [Google Scholar]
16. S. Alemaishat, O. A. Saraereh, I. Khan, S. H. Affes, X. Li et al., “An efficient precoding scheme for millimeter-wave massive MIMO systems,” Electronics, vol. 8, no. 9, pp. 1–15, 2019. [Google Scholar]
17. A. Al-Nimrat, M. Smadi, O. A. Saraereh and I. Khan, “An efficient channel estimation scheme for mm-wave massive MIMO systems,” in IEEE Int. Conf. on Communication, Networks and Satellite, Makassar, Indonesia, pp. 1–8, 2019. [Google Scholar]
18. Internet Resource, Ericsson. 2022. [Online]. Available: https://www.ericsson.com/en [Google Scholar]
19. M. Danneberg, R. Datta, A. Festag and G. Fetteis, “Experimental testbed for 5G cognitive radio access in 4 G LTE cellular systems,” in Proc. of 2014 IEEE 8th Sensor Array and Multi-Channel Signal Processing Workshop, A Coruna, Spain, pp. 321–324, 2014. [Google Scholar]
20. A. Kumar and M. Gupta, “A novel modulation technique for 5G mobile communication system,” American Journal of Applied Sciences, vol. 12, no. 9, pp. 601–605, 2015. [Google Scholar]
21. H. Askari, N. Hussain, D. Choi, M. Abu Sufian, A. Abbas et al., “An AMC-based circularly polarized antenna for 5G sub-6 Ghz communications,” Computers, Materials & Continua, vol. 69, no. 3, pp. 2997–3013, 2021. [Google Scholar]
22. S. Chen and J. Zhao, “The requirements, challenges, and technologies for 5G of terrestrial mobile telecommunication,” IEEE Communications Magazine, vol. 52, no. 5, pp. 36–43, 2014. [Google Scholar]
23. M. Peng, Y. Li, Z. Zhao and C. Wang, “System architecture and key technologies for 5G heterogeneous cloud radio access networks,” IEEE Network: The Magazine of Global Internetworking, vol. 29, no. 2, pp. 6–14, 2015. [Google Scholar]
24. T. S. Rappaport, S. Sun, R. Mayzus, H. Zhao, Y. Azar et al., “Millimeter wave mobile communications for 5G cellular: It will work,” IEEE Access, vol. 1, pp. 335–349, 2013. [Google Scholar]
25. P. Rost, C. Bernardos, A. Domenico, M. Girolamo, M. Lalam et al., “Cloud technologies for flexible 5G radio access networks,” IEEE Communications Magazine, vol. 52, no. 5, pp. 68–76, 2014. [Google Scholar]
26. I. Chih-Lin, C. Rowell, S. Han, Z. Xu, G. Li et al., “Toward green and soft: A 5G perspective,” IEEE Communications Magazine, vol. 52, no. 2, pp. 66–73, 2014. [Google Scholar]
27. B. Bangerter, S. Talwar, R. Arefi and K. Stewart, “Networks and devices for the 5G era,” IEEE Communications Magazine, vol. 52, no. 2, pp. 90–96, 2014. [Google Scholar]
28. C. Xiang, F. Haider, X. Gao, X. H. You, Y. Yang et al., “Cellular architecture and key technologies for 5G wireless communication networks,” IEEE Communications Magazine, vol. 52, no. 5, pp. 122–128, 2014. [Google Scholar]
29. B. reviea, F. Boccardi, R. W. Heath, A. Lozano, T. L. Marzetta et al., “Five disruptive technology directions for 5G,” IEEE Communications Magazine, vol. 52, no. 2, pp. 74–80, 2014. [Google Scholar]
30. P. Goyal and A. K. Sahoo, “A roadmap towards connected living: 5G mobile technology,” International Journal of Innovative Technology and Exploring Engineering (IJITEE), vol. 9, no. 1, pp. 1670–1685, 2019. https://doi.org/10.35940/ijitee.A4742.119119 [Google Scholar] [CrossRef]
31. G. Wunder, P. Jung, M. Kasparick, T. Wild, F. Schaich et al., “5GNOW: Non-orthogonal, asynchronous waveforms for future mobile applications,” IEEE Communications Magazine, vol. 52, no. 2, pp. 97–105, 2014. [Google Scholar]
32. G. Wunder, M. Kasparick, T. Wild, F. Schaich, Y. Chen et al., “5GNOW: Intermediate frame structure and transceiver concepts,” in Processing of 2014 IEEE Globecom Workshops (GC Wkshps), Austin, TX, USA, pp. 565–570, 2014. [Google Scholar]
33. Internet Resource, NEWCOM Deliverables 23.3. 2022. [Online]. Available: http://www.newcom-project.eu/images/Delivarables/D23.3-Secondreportontoolsandtheirintegrationontheexperimentalsetups.pdf [Google Scholar]
34. Internet Resource, 5GIC. 2022. [Online]. Available: http://www.surrey.ac.uk/5gic [Google Scholar]
35. J. S. Bae, Y. S. Choi, J. S. Kim and M. Young, “Architecture and performance evaluation of MmWave based 5G mobile communication system,” in Proc. of IEEE ICTC 2014, Busan, Korea (Southpp. 847–851, 2014. [Google Scholar]
36. A. Kumara and M. Gupta, “A review on activities of fifth generation mobile communication system,” Alexandria Engineering Journal, vol. 57, no. 2, pp. 1125–1135, 2018. [Google Scholar]
37. R. N. Mitra and P. Dharma Agrawal, “5G mobile technology: A survey,” ICT Express, vol. 1, no. 3, pp. 132–137, 2015. [Google Scholar]
38. A. Kumar and M. Gupta, “Design of OFDM and PAPR reduction using clipping method,” Artificial Intelligence and Network Security, vol. 1, no. 1, pp. 221–229, 2015. [Google Scholar]
39. J. Fang, Z. You, T. Lu, J. Li and R. Yang, “Comparisons of filter bank multicarrier systems,” in Proc. of Systems, Applications and Technology Conf. (LISAT), New York, USA, pp. 1–6, 2013. [Google Scholar]
40. L. B. Le, V. Lau, E. Jorswieck, N. D. Dao, A. Haghighat et al.,“ “Enabling 5G mobile wireless technologies,” EURASIP Journal on Wireless Communications and Networking, vol. 218, pp. 1–14, 2015. [Google Scholar]
41. N. Prasad, V. Croitoru, R. Prasad and C. Badoi, “5G based on cognitive radio,” Wireless Personal Communications, vol. 57, no. 3, pp. 441–464, 2011. [Google Scholar]
42. P. K. Verma, S. Taluja and R. L. Dua, “Performance analysis of energy detection, matched filter detection & cyclostationary feature detection spectrum sensing techniques,” International Journal of Computer Engineering Research, vol. 2, no. 5, pp. 1296–1301, 2014. [Google Scholar]
43. V. Chandrasekhar, J. G. Andrews, T. Muharemovic, Z. Shen and A. Gatherer, “Power control in two-tier femtocell networks,” IEEE Transactions on Wireless Communications, vol. 8, no. 8, pp. 1–28, 2009. [Google Scholar]
44. M. Petr, J. Misurec and M. Koutny, “Noise modeling for power line communication model,” in IEEE 2012 35th Int. Conf. on Telecommunications and Signal Processing (TSP), Prague, Czech Republic, pp. 282–286, 2012. [Google Scholar]
45. T. Levanen, J. Pirskanen and M. Valkama, “Radio interface design for ultra-low latency millimeter-wave communications in 5G era,” in Proc. of GLOBECOM 2014, IEEE Global Communications Conf., Austin, TX, USA, pp. 1524–1530, 2014. [Google Scholar]
46. L. Baochun and X. Hong, “Resource allocation with flexible channel cooperation in cognitive radio networks,” IEEE Transactions on Mobile Computing, vol. 12, no. 5, pp. 957–970, 2013. [Google Scholar]
47. F. Schaich and T. Wild, “Waveform contenders for 5G—OFDM vs. FBMC vs. UFMC,” in Proc. of 6th Int. Symp. on Communications, Control and Signal Processing, Athens, Greece, pp. 457–460, 2014. [Google Scholar]
48. “Internet Resource,” 2015. [Online]. Available: https://api.is-wireless.com/wp-content/uploads/2015/07/5GNOW_Deliverables-5G-Waveform-Candidate-Selection-part-2.pdf [Google Scholar]
49. D. Sabella, A. D. Domenico, E. Katranaras, M. A. Imran, M. D. Girolamo et al., “Energy efficiency benefits of RAN-as-a-service concept for a cloud-based 5G mobile network infrastructure,” IEEE Access, vol. 2, pp. 1586–1597, 2014. [Google Scholar]
50. V. Sahu and P. Dutta, “Performance enhancement in 5G the future of wireless communication: Review,” International Journal of Advanced Research in Computer and Communication Engineering, vol. 5, no. 3, pp. 65–68, 2016. [Google Scholar]
51. B. Li and H. Xua, “Anchor: A versatile and efficient framework for resource management in the cloud,” IEEE Transactions on Parallel and Distributed Systems, vol. 24, no. 6, pp. 1066–1076, 2013. [Google Scholar]
52. A. Gohil, H. Modi and S. K. Patel, “5G technology of mobile communication: A survey,” in Proc. of Int. Conf. on Intelligent Systems and Signal Processing ISSP, Vallabh Vidyanagar, India, pp. 288–292, 2013. [Google Scholar]
53. J. Qiao, X. S. Shen, J. W. Mark, Q. Shen, Y. He et al., “Enabling device-to-device communications in millimeter wave 5G cellular networks,” IEEE Communications Magazine, vol. 53, no. 1, pp. 209–215, 2015. [Google Scholar]
54. B. G. Evans, “The role of satellite in 5G,” in Proc. of 2014 7th Advanced Satellite Multimedia Systems Conf. and the 13th Signal Processing for Space Communications Workshop (ASMS/SPSC), Livorno, Italy, pp. 197–202, 2014. [Google Scholar]
55. N. Tuan Le, M. Arif Hossain, A. Islam, D. Y. Kim, Y. J. Choi et al., “Survey of promising technologies for 5G networks,” Mobile Information Systems, vol. 2016, 2676589, pp. 1–25, 2016. [Google Scholar]
56. I. S. Kocher, “An experimental simulation of addressing auto-configuration issues for wireless sensor networks,” Computers, Materials & Continua, vol. 71, no. 2, pp. 3821–3838, 2022. [Google Scholar]
57. L. Kansal, S. Berra, M. Mounir, R. Miglani, R. Dinis et al., “Performance analysis of massive MIMO-OFDM system incorporated with various transforms for image communication in 5G systems,” Electronics, vol. 11, no. 4, pp. 621, 2022. [Google Scholar]
58. J. Ali, R. H. Jhaveri, M. Alswailim and B. H. Roh, “ESCALB: An effective slave controller allocation-based load balancing scheme for multi-domain SDN-enabled-IoT networks,” Journal of King Saud University-Computer and Information Sciences, vol. 35, no. 6, pp. 1–11, 2023. [Google Scholar]
59. N. Raghavendra and M. P. S. Kumar, “Revolution of 5G wireless technology-future direction,” IOSR Journal of Computer Engineering (IOSR-JCE), vol. 21, no. 4, pp. 36–42, 2019. [Google Scholar]
60. V. Pujari, R. Patil and S. Motlekar, “5G technology of mobile communication: A survey,” Contemporary Research in India: Special Issue, pp. 1–8, 2021. [Google Scholar]
61. V. Pujari, R. Patil and K. Tambe, “Research paper on future of 5G wireless system,” Contemporary Research in India: Special Issue, pp. 252–256, 2021. [Google Scholar]
62. K. Haneda, L. Tian, H. Asplund, J. Li, Y. Wang et al., “Indoor 5G 3GPP-like channel models for office and shopping mall environments,” in Proc. of 2016 IEEE Int. Conf. on Communications (ICC), Kuala Lumpur, Malaysia, pp. 694–699, 2016. https://doi.org/10.1109/ICCW.2016.7503868 [Google Scholar] [CrossRef]
Cite This Article
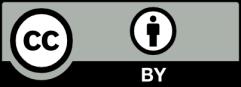