Open Access
ARTICLE
Optimization of Animal-Glue Binders for Casting Applications
1
Mechanical and Electronic Engineering, Shandong Jiaotong University, Jinan, 250357, China
2
Chongqing Research Institute, Jilin University, Chongqing, 401123, China
3
School of Intelligent Transportation, Jiangsu Vocational College of Electronics and Information, Huai’an, 223003, China
* Corresponding Author: Yunwu Han. Email:
Fluid Dynamics & Materials Processing 2023, 19(11), 2923-2932. https://doi.org/10.32604/fdmp.2023.028740
Received 05 January 2023; Accepted 14 March 2023; Issue published 18 September 2023
Abstract
In typical metal foundry applications, sand casting is still the most used technology. The related binder plays a very important role as its performances can directly influence the quality of castings. Among many binders, glues of animal origin have attracted much attention in recent years due to their reduced environmental impact. However, they display some drawbacks such as the tendency to coagulate easily at room temperature and a relatively low strength. In this study, a novel gas-hardening casting binder was prepared using an animal glue and anhydrous potassium carbonate as a hydrolyzing agent to avoid undesired agglomeration. Moreover, sodium pyrophosphate and furfuryl alcohol were exploited as modifiers to obtain a binder with a high compressive strength. The best modification conditions, determined by means of an orthogonal design matrix approach, were 4 g of Na2CO3, sodium pyrophosphate, furfuryl alcohol and animal glue with a ratio of 4:12:100, at 85°C and with a duration of 115 min, respectively. The viscosity of the mixture obtained under these optimized conditions was 1250 mPa⋅s. The compressive strength of the binder, hardened by CO2 gas, was 4.00 MPa. Its gas evolution at 850°C was 15 ml⋅g−1 , and its residual strength after 10 min calculation at 800°C was 0.01 MPa, which is high enough to meet the requirement of core-making in foundry. Moreover, after hydrolysis and further modification, animal glue and modifiers displayed a grafting reaction and an esterification reaction, respectively, which made the adhesive network denser and improved its thermal stability.Keywords
As we all know, many kinds of organic binders, such as epoxy resin [1] and furan resin [2], are used in casting industry every year. Some components of organic adhesives do great harm to the environment and workers, so a variety of pollution-free binder is needed for casting. Therefore, binders obtained from natural materials attract a lot of attention. Clay, montmorillonoid and starch have the advantages of low cost and short molding cycle. However, their castings are prone to sand washing, sand inclusion, porosity and other defects, which restrict their application [3,4].
Animal glue is a kind of non-toxic, ecologically clean, and biodegradable natural polymer material, which is also abundant and inexpensive [5,6]. Nevertheless, animal glue binder applications are limited. Most of the research involving binders based on animal glue focused on preventing or eliminating its room temperature aggregation. One of the ways is to combine animal glue with other polymeric binders. Thus, Liu et al. used acrylic acid and glucose to prepare an animal glue binder with high tensile strength and apply it to aluminum alloy castings [7]. Miao et al. developed a liquid bone glue adhesive using epichlorohydrin [8]. Guo et al. proposed to chemically cross-link Al3+ with the bone glue. The surface of the resulting CA membrane was smoother and denser than of the original bone glue membrane [9]. Fan et al. used water-based polyurethane blends to modify animal glue and quickly cured it with compressed air [10]. The tensile strength of the molding sand could reach 2.60 MPa.
This paper reports synthesis method of a modified animal glue binder with high compressive strength and low viscosity prepared using sodium pyrophosphate and furfuryl alcohol. A thorough analysis of the resulting material by using Fourier transform infrared (FT-IR) spectroscopy and differential scanning calorimetry (DSC), provides insights into its formation, modification, and performance mechanism, which is discussed in details in this paper.
All initial materials (animal bone glue, anhydrous potassium carbonate, sodium pyrophosphate and furfuryl alcohol) were analytically pure. The sand ZGS-50/100(60) was purchased from Dalin Co. (China).
120 g water and 100 g animal glue were mixed at room temperature, for 30 min. Then, 1, 2, 3, 4, 5, 6 g Na2CO3 as the catalyst was added, respectively, after sodium pyrophosphate and furfuryl alcohol were added. The resulting mixture was heated under constant stirring, after 5 g anticoagulant was added. The mixture was stirred for another 30 min and then cooled down naturally to room temperature. The resulting brown liquid was a modified animal glue binder. The binder has a shelf life of 90 days at room temperature.
An orthogonal experimentation was used to determine the best modification conditions. Compressive strength and viscosity were tested as the important performance indicators of the binder. The orthogonal experiment of L9(43), which contains factors with three levels of sodium pyrophosphate, furfuryl alcohol, modification time and modification temperature, was designed as Table 1, and the test scheme was arranged as Table 2.
1000 g of sand, 30 g of the binder, and 12.5 g of Ca(OH)2 (used as a hardening accelerator) were mixed in casting sand mixer (120 r/min) for 2 min, and then it was made a standard “Ф50 mm × Ф50 mm” sample, and hardened under a constant CO2 flow (0.6 m3/h air blowing amount and 60 s air blowing time). Then, the samples were taken out and cooled to room temperature for next testing. The compressive strength of the prepared samples was the average of the 5 samples.
2.4 Sand Sample Characterization
A scanning electron microscope (SEM) was used to characterize the bonding bridge and fracture morphology of the molding sand sample. Cut a 10 mm × 10 mm × 10 mm cube test block along the section of the molding sand sample, and place it on the gold spraying station to spray gold.
Collapsibility of sand: Heat the sand sample to different temperatures for 10 min, then cool it to room temperature, and test its strength. The test result is the average of 3 samples.
Gas emission: Use SFL gas emission tester to test the gas emission at 850°C with (1 ± 0.01) g sand sample.
2.5 Measurement of Binder Viscosity
The viscosity of binder was measured by a “NDJ-1” rotary bond meter. The 200 g binder sample, which was cooled to room temperature, was placed in a beaker, and after rotating 120 s at the 3# rotor (30 r/min speed) read value, this experiment repeated 5 times, the data averaged.
3.1 Hydrolysis Catalyst Optimization
The peptide groups (-NH-CO-) presence is the main reason for the animal glue aggregation at room temperature [11,12]. However, accumulations can be prevented by introducing hydrolysis catalysts such as acids, bases, and enzymes into the system during its synthesis process. These liquid additives do not affect the chemical reaction [13,14].
We used HCl, Na2CO3, and KOH as anticoagulants to study how they affected the final animal glue binder properties.
As seen in Table 3, the best combination of the binder viscosity and compressive strength of the hardened cast was obtained when Na2CO3 was used as the anticoagulating agen. Therefore, it was used for all further experiments as a hydrolysis catalyst.
We also performed experiments to optimize Na2CO3 quantity. When 1–3 g Na2CO3 was added, the viscosity of the binder decreased rapidly, and the compressive strength of the molding sand increased as the Na2CO3 amount increased (see Fig. 1). When over 4 g of Na2CO3 was added, the compressive strength of the molding sand decreased, and the viscosity increased proportionally. The lowest viscosity and the highest compressive strength were obtained when 4 g of Na2CO3 was used. This level was used in all further experiments.
Figure 1: Effect of anhydrous sodium carbonate content on properties of binders
The comparison of the FTIR spectrum of the hydrolyzed (by Na2CO3) animal glue with the unmodified sample (shown in Fig. 2A) showed that there is a wider absorption band at 3500–3200 cm−1 (which was attribute to the increased amount of OH groups), peaks at 1658, 1456, and 930 cm−1 (which were attributed to the carboxylic -COOH and -COO groups) and signals at 1558, 1147, and 3340.71 cm−1 (which were ascribed to the amino NH2 groups) (see Fig. 2B). Thus, peptide bonds of the animal glue were broken by Na2CO3 into hydroxyl, amino and carboxylic groups.
Figure 2: FT-IR spectra of (A) unmodified, (B) Na2CO3-treated, and (C) modified animal glue binder
After modifying the binder with sodium phosphate and furfuryl alcohol, a band at 3500–3000 cm−1 became weaker because of the decreased amount of the OH groups in the binder (see Fig. 2C). The peaks at ∼640 and 1025 cm−1 were assigned to the O-P-O bond bending and stretching, respectively. An absorption peak near 1250 cm−1 was interpreted as a P-O-C bond stretching, while the one at 1344 cm−1 was P=O stretching. No peaks attributable to the P-O-P bending vibrations were detected (typically located around 800–750 cm−1). These results indicate a cross-linking reaction completion between pyrophosphate and the -OH groups of the binder. The peaks at 1600–1500 cm−1 belonged to the C=O stretching vi-brations, while a band at 1045 cm−1 belonged to a C-O-C. These bands indicate a fatty anhydride for-mation during animal glue binder and furfuryl esteri-fication reaction.
As can be seen from Table 4, GPC data indicated that the average molecular weight of the untreated animal gum was 268160, the polydispersity was 2.73, and the molecular distribution was wide. Animal glue binder treated by Na2CO3 had a lower molecular weight, and its molecular weight distribution was relatively narrow. Moreover, the modified animal glue binder was higher than the unmodified binder, but the distribution was narrower. After modification, the molecular weight of the binder was increased, indicating that the molecules of the animal glue and the modifiers are grafted and cross-linked to form a larger molecule, and the smaller molecular component in the binder made the molecular weight distribution narrower.
The modified mechanism can be expressed by the reactions shown below (see Fig. 3).
Figure 3: The reaction equation of animal glue modification
DSC curves of the unmodified and modified binder samples were significantly different (see Fig. 4). The shrinkage temperature of the unmodified binder was 53°C (curve A), the double endothermic peaks appear at 180°C–200°C, which indicates the unmodified animal glue has multiple thermal transitions. After the modification, the thermal denaturation peak of the binder basically disappeared (curve B), an exothermic plateau appeared when the temperature was raised to 100°C. The heat release of it accelerated when the temperature was higher than 250°C. The molecular chain of the modified binder becomes longer, hydrogen bonding increases, intermolecular bonding becomes stronger, and thermal stability increases.
Figure 4: DSC curve of the (A) unmodified and (B) modified animal glue binder
3.3 Orthogonal Tests of the Best Parameters for the Binder Structure and Composition Optimization
To quantitatively evaluate how four factors affected final binder properties, and based on single factor test results. We designed an orthogonal experiment with four factors and three levels to analyze the corresponding data ranges (see Tables 4 and 5). All test results were the average of 5 samples.
Compressive strength was affected by these optimization factors in the following order: furfuryl alcohol > sodium pyrophosphate > modification temperature > modification time. Under T4 treatment (4 g sodium pyrophosphate, 10 g furfuryl alcohol, 115 min modification time and 85°C modification temperature), the highest tensile strength than other treatments were observed (see Fig. 5). However, according to the average value (k) of the compressive strength of each test factor, 4 g of sodium pyrophosphate, 12 g of furfuryl alcohol, a modification time of 115 min and a modification temperature of 85°C were selected for further testing (see Table 5). Under these conditions, it compared to T4, showed better effects not only on compressive strength (4.00 MPa) but also on viscosity (1250 mPa⋅s). Samples prepared under these conditions were used for the next round of tests.
Figure 5: Analysis of the modifier addition ranges
3.4 Stress-Strain Curve of Compressive Process of Sand Sample
As can be seen from Fig. 6, it was found that the stress-strain curve of the sand sample was identified as two steps, the plastic deformation stage (O-M) and the rupture stage (M-C), and compressive strength of M point was the peak in the σ-ε curve. Under the action of compressive stress, the animal film between sand particles is squeezed to become smaller and undergoes irreversible viscoplastic deformation until the film reaches the stress level of resisting the compressive deformation of the bonding bridge. Moreover, when the stress reaches the ultimate compressive strength, the sample enters the stage of extrusion fracture, which lasts for a short time. When the stress exceeds the compressive strength of the sample, the sample will be crushed.
Figure 6: Stress-strain curve of compressive process of sand sample
Compared to the unmodified binder molding sand (shown in Fig. 7a), the surface of the modified sand possessed more bonding bridges and nets (see Fig. 7b). Additionally, the fracture areas of the bonding bridges in the sand sample prepared using the modified binder were larger, which indicates higher strength.
Figure 7: The morphology of the unmodified and modified animal glue sand
The addition of sodium pyrophosphate and furfuryl alcohol increased the amount of the active molecular groups and polarity of the binder. With the reaction, the binding force between the sand and the binder increases. The bond bridge network of molding sand increases, too. All these factors contributed to the enhanced strength of the molding sand.
We also tested the collapsibility and gas generation of the modified binder molding sand (see results in Table 6 and Fig. 8, respectively).
Figure 8: Gas evolution from the sand used together with the modified binder as a function of time
Collapsibility means that sand is not easy to break after casting solidification. After touring at 800°C, the residual strength of the sample after annealing is only 0.01 MPa. Thus, the modified binder possessed excellent collapsibility, which is essential upon binder pouring into the molds. Gas evolution refers to the amount of gas emitted by the sand mold when encountering high-temperature metal solution during pouring. In this experiment, the gas evolution of the modified animal glue binder sand was 15 ml⋅g−1, which meets the casting requirements.
A novel liquid animal glue binder was prepared by hydrolysis reaction and modification. It was used for core making based on CO2 hardening. The samples prepared with this binder possesses high compressive strength (4.00 MPa) and suitable viscosity (1250 mPa⋅s). The main conclusions and results of this study are summarized as follows: Anhydrous potassium carbonate was the best hydrolysis catalyst. Its optimal level was 4 g per each 100 g of animal glue binder; The optimum sodium pyrophosphate/furfuryl alcohol/animal glue weight ratio was 4:12:100, while the best modification time and reaction temperature were 115 min and 85°C, respectively; FT-IR spectra confirmed that the chemical com-position and structure of the binder has changed after the hydrolysis and further modification due to the cross-linking and esterification reactions. DSC analysis proved that the modified animal glue binder possessed remarkably high thermal stability; The modified binder sand possessed denser bonding networks.
Its gas evolution at 850°C was 15 ml⋅g−1. Its residual strength after 10 min calculation at 800°C was 0.01 MPa, which can meet the requirements of aluminum alloy sand castings. In this experiment, it is found that a large amount of waste sand produced during the experiment has caused waste of resources. Then, with the characteristics of good water solubility and biocompatibility of animal glue, we suggest that the waste sand can be regenerated by heating, water-machinery combination and other methods in the subsequent treatment of casting waste sand, and the regenerated sand can be used as new sand to realize waste recycling.
Funding Statement: This research was supported by the National Natural Science Foundation of China (51275313); Shandong Province Transportation Science and Technology Project (2021B115); Shandong Jiaotong University School Fund (Z2019036).
Conflicts of Interest: The authors declare that they have no conflicts of interest to report regarding the present study.
References
1. Knapíková, L., Behúnová, A. (2020). Research of casting moulding of epoxy resin composites reinforced with high-strength fibres during the manufacturing operations. TEM Journal, 9(4), 1488–1493. https://doi.org/10.18421/TEM [Google Scholar] [CrossRef]
2. Zhang, S. Y., Qin, Y., Li, T., Luo, W. G. (2018). Experimental analysis of cast-bonded technique by separate casting with clay mould. Foundry, 67(9), 818–822. [Google Scholar]
3. Lachance, A. M., Hou, Z., Farooqui, M. M., Carr, S. A., Serrano, J. M. et al. (2022). Doctor-blade-assisted casting for forming thin composite coatings of montmorillonite and poly(vinyl alcohol). Industrial & Engineering Chemistry Research, 61(10), 3766–3774. https://doi.org/10.1021/acs.iecr.1c04381 [Google Scholar] [CrossRef]
4. Giorleo, L., Bonaventi, M. (2021). Casting of complex structures in aluminum using gypsum molds produced via binder jetting. Rapid Prototyping Journal, 27(11), 13–23. https://doi.org/10.1108/RPJ-03-2020-0048 [Google Scholar] [CrossRef]
5. Wang, T. S., Zhang, Z., Zhang, S. J., Wang, M. Y. (2021). Hydrolysis optimization and application of animal glue binder for casting. Journal of Physics: Conference Series, 1948(1), 012217. https://doi.org/10.1088/1742-6596/1948/1/012217 [Google Scholar] [CrossRef]
6. Fan, C. C., Tang, Q. (2020). Allyl glycidyl ether-modified animal glue binder for improved water resistance and bonding strength in sand casting. Organic Polymer Material Research, 2, 1–7. https://doi.org/10.30564/opmr.v2i2.2384 [Google Scholar] [CrossRef]
7. Liu, W. H., Zhang, Y. L., Li, Y. M. (2012). Process optimization of new air hardening animal glue for core sand binder. Foundry, 7, 718–721. [Google Scholar]
8. Miao, Z. C., Wang, F., Deng, D. (2012). Preparation of bone glue adhesives using epichlorohydrin modification. Advanced Materials Research, 6, 557–559. https://doi.org/10.4028/www.scientific.net/AMR.557-559.1005 [Google Scholar] [CrossRef]
9. Guo, M., Su, X., Zhou, L. (2015). Synthesis mechanism and water tolerance of bone glue adhesive material modified by Al3+. Journal of Functional Materials, 12, 12039–12043. [Google Scholar]
10. Fan, C. C., Gong, C. B., Peng, J. D. (2020). Study on the preparation process of air hardening waterborne polyurethane modified animal adhesive. Shandong Chemical Industry, 49, 33–38. [Google Scholar]
11. Oh, S. M., Ji, A. Y., Na, R. L. (2019). A study on characteristics of animal glue depending on pretreatment and evaluation condition. Journal of Conservation Science, 6, 701–708. https://doi.org/10.12654/JCS.2019.35.6.13 [Google Scholar] [CrossRef]
12. Esteban, M. B., García, A. J., Ramos, P. (2010). Sub-critical water hydrolysis of hog hair for amino acid production. Bioresource Technology, 7, 2472–2476. https://doi.org/10.1016/j.biortech.2009.11.054 [Google Scholar] [PubMed] [CrossRef]
13. Randle, S. B. (2020). Report on Fat in cooked animal feeds containing cereals (acid hydrolysis). Journal of Association of Official Agricultural Chemists, 4, 864–866. [Google Scholar]
14. Wang, L. C., Chen, S. Y. (2012). A novel hydrolytic product from flesh of mactra veneriformis and Its bioactivities in calcium supplement. Journal of Ocean University of China, 3, 389–396. https://doi.org/10.1007/s11802-012-1960-4 [Google Scholar] [CrossRef]
Cite This Article
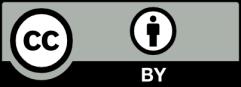