Open Access
ARTICLE
Characterization of Flame Retardancy and Oil-Water Separation Capacity of Superhydrophobic Silylated Melamine Sponges
1 College of Chemistry and Chemical Engineering, FLUOBON Pilot Base of Chemical Engineering, Longdong University, Qingyang, 745000, China
2 Qingyang Solid and Hazardous Waste Pollution Prevention and Control Management Center, Qingyang, 745000, China
3 Gansu Qingluyuan Environmental Monitoring Co., Ltd., Qingyang, 745000, China
4 School of Chemistry and Chemical Engineering, Shanxi Key Laboratory of Low Metamorphic Coal Clean Utilization, Yulin University, Yulin, 719000, China
* Corresponding Author: Yongchun Liu. Email:
Fluid Dynamics & Materials Processing 2024, 20(2), 383-400. https://doi.org/10.32604/fdmp.2023.041928
Received 11 May 2023; Accepted 24 July 2023; Issue published 14 December 2023
Abstract
A silylated melamine sponge (SMS) was prepared by two simple steps, namely, immersion and dehydration of a melamine sponge coated with methyltrichlorosilane. The silylated structure of SMS was characterized by FT-IR (Fourier-transform infrared) spectroscopy, SEM (Scanning electron microscopy) and in terms of water contact angles. Its oil-water absorption and separation capacities were measured by FT-IR and UV-visible spectrophotometry. The experimental results have shown that oligomeric silanol covalently bonds by Si−N onto the surface of melamine sponge skeletons. SMS has shown superhydrophobicity with a water contact angle exceeding 150° ± 1°, a better separation efficiency with regard to diesel oil (by 99.31% (wt/wt%) in oil-water mixture and even up to 99.99% (wt/wt%) for diesel oil in its saturated aqueous solution. Moreover, SMS inherited the intrinsic flame retardancy of the melamine sponge. In general, SMS has shown superhydrophobicity, high porosity, excellent selectivity, remarkable recyclability, and better absorption capacity for various oils and organic solvents, and a high separation efficiency for oil in saturated aqueous solutions.Keywords
With the development of petroleum and chemical industry, oil products and chemical reagents will inevitably have leakage accidents and even combustion and explosion in production, storage and transportation, which has become one of the most serious environmental and ecological problems. The use of oil sorbents has been considered one of the most effective approaches for oil spill cleaning due to its propensity for oil collection and separation from water [1]. Recent studies of oil sorbents have focused on superhydrophobic sponges and sponge-like materials that exhibit superior oil sorption capacity [2–4]. Superhydrophobic material refers to the material with superhydrophobic surface whose water contact angle is greater than 150° and rolling angle is less than 10° [5]. This kind of material must have two conditions: the surface has micro-, nano-structure and low surface energy material. The preparation methods of superhydrophobic materials are mainly divided into two ways [6,7]: (i) micro- and nano-structures are constructed on the material surface, and then low surface energy substances are modified; (ii) micro-, nano-structure substances with low surface energy are constructed on the surface of materials. The materials with superhydrophobic properties prepared by the above methods are the most biomimetic interface and oil-water separation materials studied so far. These materials can be divided into high hydrophobic powder materials, superhydrophobic film materials and superhydrophobic three-dimensional porous materials [8]. Melamine sponges are open-cell foam structures, consisting of formaldehyde-melamine-sodium bisulfite copolymer, having low density (4−12 mg/cm3), high porosity (>99%), and excellent elasticity, all of which are ideal characteristics for sorption materials [4]. Fluorocarbons (such as polytetrafluoroethylene) and silicones (such as polydimethylsiloxane) are two kinds of well-known superhydrophobic materials with low surface energy. In addition, some organic materials (such as paraffinic hydrocarbons, polyamide, polycarbonate and alkylketene dimer) and inorganic materials (such as ZnO and TiO2) have also been made into superhydrophobic surfaces [9]. In recent years, a series of superhydrophobic sponges have been prepared by silylated melamine sponges, where alkylsilane compounds covalently bond onto the surface of the sponge skeletons through secondary amine groups, forming a self-assembled monolayer (SAM) [4,10,11]. Moreover, melamine sponge has ideal flame retardancy due to its high nitrogen content. When exposed to heat, it will degrade and release N2, which will replace the oxygen surrounding the material, thus preventing further burning [3]. On the other hand, most of the researches focus on the sorption and recovery of pure oil or oil mixed in water, while the separation of absorbers for oil dissolved in water is often ignored, which will subsequently cause water environmental and ecological pollution. In this study, a superhydrophobic silylated melamine sponge was prepared from melamine sponge coated by methyltrichlorosilane with low surface energy, and its chemical structure, surface morphology and hydrophobicity were investigated by FT-IR, SEM and static water contact angles. Importantly, its absorption capacity for common oils and organic solvents and its separation efficiency for oil dissolved in water were studied by gravimetric analysis, UV-vis and FT-IR spectrophotometry. Furthermore, its flame retardancy was investigated, too.
Commercial melamine sponge was purchased from Henan Derun New Material Technology Co., Ltd., Puyang, China (Bulk density measured was 7.60 mg/cm3). Methyltrichlorosilane (analytical reagent) was purchased from Shanghai Branch of Aladdin Biochemical Technology Co., Ltd., Shanghai, China. Petroleum standard stock solution (ρ = 1000 mg/L, n-hexane as solvent) was purchased from Tanmo Quality Inspection Technology Co., Ltd., Changzhou, China (Product Lot No. A2007023). NewPig® Absorbent Mat Pad was obtained from reserve materials of Changqing Oilfield Branch of China National Petroleum Corporation (CNPC), Qingyang, China. Activated carbon was obtained from Qingyang Zhongyuyuan Agriculture and Forestry Technology Co., Ltd., Qingyang, China (its average iodine value of 957.9 g/g was provided by the manufacturer). Crude oil after dehydrated and desalted electrostatically was obtained from Qingyang Petrochemical Branch of CNPC, Qingyang, China. Tetrafluoroethylene, n-hexane and n-hexadecane are of spectral pure reagents. Oils were commonly commercial products. Other organic solvents and reagents were analytical pure reagents. The experimental water was secondary deionized water (conductivity ≤ 0.5 μs/cm).
2.2 Silylation of Melamine Sponge
The silylation of melamine sponges was performed by two simple steps of immersion and dehydration as references [4,10,11]. The melamine sponges were cut into 2.0 cm3 × 2.0 cm3 × 2.0 cm3 pieces and cleaned by acetone and secondary deionized water in turn in ultrasonic cleaner for 30 min at 25°C. Then they were dried at 95°C for 8 h. Subsequently, they were immersed in a 0.5% (wt/wt%) solution of methyltrichlorosilane solved in n-hexane for 30 min. Thereafter, they were fished out from the solution and placed in an oven with weak exhaust system at 25°C and 30% relative humidity environment for 30 min. Finally, after squeezed to extract the absorbed solution, they were dried at 120°C for 1 h in an oven with weak exhaust system. The resultant silylated melamine sponges were denoted as SMS.
The surface morphologies of SMS were observed by SEM (ZEISS Sigma 500, Au used as coating target). Static water contact angles on the SMS were measured using a Drop Shape Analyzer (KRÜSS). A droplet of 4 μl water were gently deposited on the samples with a pipet, and the static water contact angle was measured using the goniometer optics. The measurements were repeated three times at different locations on each sample, and the mean static water contact angle value were reported. The infrared spectra of melamine sponge before and after silylation were recorded by FT-IR spectrometer (Nicolet nexus 670, KBr disc).
2.4 Absorption Capacity of SMS for Pure Oil and Organic Solvents
The absorption capacities of SMS for various pure oils and organic solvents were determined by dipping a piece of SMS into the target liquid (oil or organic solvent) until this liquid is adsorbed and saturated by SMS at a constant room temperature (25°C), except crude oil was heated to 40°C beforehand. And then taking SMS out for weight measurement with a balance with a sensitivity of 0.1 mg. The gravimetric absorption capacity (Qm/m), gravimetric/volumetric absorption capacity (Qm/v), and volumetric absorption capacity (Qv/v) were calculated according to the following equations [4]:
where, ms and ms,o are the masses of sponge before and after absorption, ds and do are the silylated bulk density of the sponge and the density of organic liquid, respectively.
The recyclability of SMS was evaluated by repeated absorption−squeezing processes.
The absorption efficiency of SMS for oil in oil-water mixture was calculated according to the following equation and denoted as AEm:
where, mo and mw were the masses of oil and water adsorbed by SMS of per gram, respectively, g/g.
Three small cleaned porcelain crucible were heated by alcohol blowtorch until the bottom were red and bright (up to 800°C) for 10 min so that they reached a constant weight, respectively. Then a certain quality of blank melamine sponge, SMS, and polypropylene foam used as a contrast materials were put into the crucible, respectively. The lasting time of degradation or combustion of these materials in the crucible was recorded and compared with each other.
2.6 Absorption Capacity of SMS for Oil in Oil-Water Mixture
Diesel oil (0#) and crude oil were selected as typical oils. Absorption capacity of SMS for diesel oil (0#) in oil-water mixture was measured by both gravimetric analysis and UV-vis spectrophotometry (Cary 60 UV-vis, Agilent Technologies) simultaneously. The standard regression curve of UV-vis spectrophotometry was established using petroleum standard stock solution (ρ = 1000 mg/L), measuring at the wavelength of 225 nm with 1 cm quartz cell, and taking n-hexane as the reference (National environmental protection standards of the people’s Republic of China, Water quality–Determination petroleum–Ultraviolet spectrophotometric method, HJ 970-2018). Before sample determination, experimental water was replaced the sample for blank determination.
Absorption capacity of SMS for crude oil in oil-water mixture was tested as the same process of diesel oil (0#), the difference was that the sufficient and definite mass of crude oil and water were preheated to 40°C.
2.7 Separation Efficiency of SMS for Oil in Its Saturated Aqueous Solution
Diesel oil (0#) and crude oil were also selected as typical oils. Separation efficiencies of SMS for diesel oil (0#) and crude oil in their saturated aqueous solution at 25°C were measured by Infrared oil-measuring spectrophotometer (D-18B, Hongyuankeyi, China), respectively. Before sample determination, the calibration coefficient of Infrared oil-measuring spectrophotometer (set with a correction factor in advance) was tested with petroleum standard solution. The relative error between the measured value and the standard value was less than ±10%. The concentration of total oil or petroleum in the sample shall was calculated according to the following formula (National environmental protection standards of the people’s Republic of China, Water quality–Determination petroleum, animal fats and vegetable oils–Infrared spectrophotometry, HJ 637-2018):
where, ρ is the concentration of total oil or petroleum in the sample, mg/L; ρ0 is the concentration of total oil or petroleum in blank sample, mg/L; X is the coefficient corresponding to the absorbance of C−H bond in CH2 group, mg/L/absorbance; Y is the coefficient corresponding to the absorbance of C−H bond in CH3 group, mg/L/absorbance; Z is the coefficient corresponding to the absorbance of C−H bond in aromatic ring, mg/L/absorbance; F is the correction factor for the influence of aliphatic hydrocarbons on aromatic hydrocarbons, that is the ratio of the absorbance of n-hexadecane at 2930 to 3030 cm−1; A2930, A2960 and A3030 are the absorbance values measured at each corresponding wave number, respectively; V0 is the volume of extraction solvent, ml; Vw is the volume of sample, ml; D is the dilution ratio of extract.
Before sample determination, experimental water was replaced the sample for blank determination. The concentration of polar substances containing animal fats and vegetable oils was calculated by the difference between total oil and petroleum in the sample.
3.1 Silylation of Melamine Sponge
It was reported that the optimum experimental conditions of silylation of the melamine sponges were 0.5% (wt/wt%) of methyltrichlorosilane, 25°C and 30% relative humidity and lasting for 30 min of immersion and hydrolysis [4,10,11]. In this study, the immersed concentration of methyltrichlorosilane solved in n-hexane vs. the static water contact angle and absorption capacity of SMS were investigated. It was found that when the immersed concentration of methyltrichlorosilane was lower than 0.5% (wt/wt%), the static water contact angle of SMS was lower than 150°, the minimum characteristic value of superhydrophobic materials [5]. In fact, when the immersed concentration of methyltrichlorosilane was selected as 0.25%, 0.5% and 1.0% (wt/wt%), the static water contact angles of SMS were 140° ± 2°, 150° ± 1° and 153° ± 2°, respectively. While melamine sponge was immersed by 1.0% (wt/wt%) of methyltrichlorosilane, the absorption capacity of SMS for diesel (0#) and crude oil decreased 4.6% and 7.3% (wt/wt%) compared with that immersed by 0.5% (wt/wt%) of methyltrichlorosilane, respectively, due to the porosity of the former (99.39%) was lower than that of the latter (99.46%), though the water contact angle of the former (153° ± 2°) was higher than that of the latter (150° ± 1°). The dehydration and drying temperature was selected as 120°C and lasting for 1 h so that the oligomeric silanol compounds covalently bonded onto the surface of the melamine sponge skeletons [4]. Therefore, the SMS was prepared by immersion concentration of 0.5% (wt/wt%) of methyltrichlorosilane solution, and its absorption capacity, separation efficiency and flame retardancy were investigated subsequently in this study.
The silylation of the melamine sponges is illustrated as Fig. 1. Methyltrichlorosilane was first hydrolyzed by water that had absorbed onto the surface of the melamine sponge’s skeleton (melamine sponges absorb 4.0% (wt/wt%) of H2O at room temperature due to its high hydrophilicity) to form silanol compounds [4]. Subsequently, they were further completely hydrolyzed by water at a constant humidity of 30% and temperature of 25°C for 30 min. Thereafter, condensation of the Si−OH formed oligomers. Finally, dehydration reaction occurred that resulted to form N−Si covalent bonds and thus oligomeric silanol compounds covalently bonded onto the surface of the skeletons [4].
Figure 1: The functional silylation of the melamine sponges from hydrophilicity to superhydrophobicity
Fig. 2 shows the SEM images of blank melamine sponge (a∼c) and SMS (d∼f). The magnified times of sponge morphologies are from 5.00 KX to 55.00 KX. The pore sizes of blank melamine sponges are in the range of 50–100 μm, and the sponge ligaments are ∼6 μm in diameter. Importantly, compared with the smooth and flawless surface of blank melamine sponge, the surface of SMS has constructed rough, papillary micro-, and nano-structures with low surface free energy, probably a hydrolytic self-aggregation product of methyltrichlorosilane, which is the fundamental reason for the special water-repellency of the surface [12,13].
Figure 2: The SEM images of blank melamine sponge (a, b and c) and SMS (d, e and f). The magnified times of sponge morphologies are from 5.00 KX to 55.00 KX
Porosity of SMS is determined by density method. The melamine sponge has bulk density of 7.60 mg/cm3, which accounts for a 99.52% porosity considering a specific density of 1.57 g/cm3 for melamine resin [4]. After silylation by 0.5% (wt/wt%) of methyltrichlorosilane, the bulk density increases from 7.60 to 8.40 mg/cm3 with the porosity of 99.46%, indicating that alkylsilane loading on the melamine sponge has a higher weight gain of 10.5% (wt/wt%) and a less porosity reduction of 0.06%. While after silylation by 1.0% (wt/wt%) of methyltrichlorosilane, the bulk density increases to 9.61 mg/cm3 with the porosity of 99.39%, a higher weight gain of 26.4% (wt/wt%) and a less porosity reduction of 0.13%. As the same preparation methods, silylation of melamine sponges by octadecyltrichlorosilane has a lower weight gain of 1.61% (wt/wt%) and a less porosity reduction of 0.01%, where the blank melamine sponge has bulk density of 8.07 mg/cm3 and porosity of 99.49% [4]. Moreover, after silylation of melamine sponges with bulk density of 8.0 and 9.6 mg/cm3 by polydimethylsiloxane, both of the porosity are more than 99.4% [10]. However, these silylated melamine sponges have higher porosities, special water-repellencies and better estimated absorption capacities for oils and organic solvents.
As shown in Supplementary Fig. S1, compared with the FT-IR spectrum of blank melamine sponge, in the wave number range of 1500∼4000 cm−1, the shape and position of the infrared absorption peak of the blank melamine sponge and SMS are almost the same, such as characteristic absorption of N−H at 3412 cm−1 and C = N at 1597 cm−1, except SMS shows a moderate intensity absorption peak at 2972 cm−1 that belongs to the C−H stretching vibration characteristic peak of Si-CH3. While, in the range of wave number 500∼1500 cm−1, there are significant differences in the shape and position of infrared absorption peaks between blank melamine sponge and SMS, that is, peaks of 1273 and 1126 and 1030 cm−1 belong to the characteristic absorption of Si−O−Si, peaks of 901 cm−1 changed from 893 cm−1 and additional 783 cm−1 belong to the characteristics of Si−N [14,15]. This further indicates that oligomeric silanol compounds have covalently bonded onto the surface of the SMS skeletons.
The hydrophobicity of the SMS was characterized by measurements of static water contact angle. As shown in Fig. 3a, the average water contact angle of SMS is 150° ± 1°, indicating the superhydrophobic characteristics of SMS. When SMS was immersed in water by applying an external force, a mirror-like surface of SMS was found, which was formed between entrapped air residing along the surface of SMS and the surrounding water as shown in Fig. 3b, this phenomenon is due to the Cassie−Baxter non-wetting behavior [16,17].
Figure 3: (a) The average water contact angle of SMS is 150° ± 1°. (b) A mirror-like surface on the SMS formed between entrapped air residing along the surface of the sponge and the surrounding water
3.3 Absorption Capacity of SMS for Pure Oil and Organic Solvents
Both the Qm/m and Qv/v were used to evaluate the overall absorption capacities of materials. The Qm/m greatly depends on the bulk density, much more than on the porosity of the absorbent material. Therefore, the sorption material that has low or ultralow bulk density typically exhibits very high Qm/m [4]. Fig. 4 shows the bar diagram of Qm/m of SMS for common oils and organic solvents at 25°C (except for crude oil at 40°C). Tables 1 and Supplementary Table S1 list the absorption capacities of SMS and the other types of functionalized melamine sponges for common oils and organic solvents, respectively [2,4,10,18–23].
Figure 4: The bar diagram of gravimetric absorption capacities of SMS for various organic solvents and oils at 25°C except for crude oil at 40°C
It is found that Qm/m of SMS for present organic solvents and oils changes from 62.7 to 172.2 g/g, and Qv/v changes from 0.6750 to 1.077 m3/m3, indicating a higher porosity and better absorption capacities of SMS for various oils and organic solvents. It is more interesting that only in terms of Qm/m of chloroform, SMS exhibits much superior adsorption property compared with the other types of functionalized melamine sponge except for mercapto-functionalized polydopamine coated melamine sponge (195 g/g), however, Qv/v of SMS is higher than the latter (0.9000 m3/m3). In addition, Octadecylphosphonic acid (ODP)-co-TiO2 melamine sponge has a much higher Qm/m of 211 g/g for chloroform, but its Qv/v has not been reported. Moreover, CW (candelilla wax)-coated Fe3O4@MS has higher Qv/v of 1.358∼1.432 m3/m3 for dichloromethane, isooctane and carbon tetrachloride, but its Qm/m of 55∼132 g/g is lower than that of SMS.
The recyclability of SMS for p-xylene as typical organic solvent and diesel oil (0#) as typical oil at a constant room temperature of 25°C were determined by absorption−squeezing gravimetric analysis. As shown in Fig. 5, after circulating absorption/extrusion of 30 times, the absorption capacity of SMS for diesel oil (0#) decreases 11.60% (wt/wt%), while after circulating 70 times, the absorption capacity of SMS for p-xylene decreases only 6.31% (wt/wt%), which may be due to a small amount of adhesion of diesel oil to SMS when circulating absorption/extrusion. From the linear fitting relationship, it can be concluded that the absorption capacity of SMS would decrease 50% (wt/wt%) after circulating absorption/extrusion of 600 times for p-xylene (Adsorptive ability (g/g) = −0.09111n + 109.4, R2 = 0.9480, n represents the absorption/extrusion times) or 115 times for diesel oil (Adsorptive ability (g/g) = −0.3756n + 90.10, R2 = 0.8575). However, SMS possesses a high recyclability of absorption/extrusion for various organic solvents and oils.
Figure 5: The recyclability of absorption/extrusion of SMS for p-xylene and diesel oil (0#) at a constant room temperature of 25°C
On the other hand, Liu [11] prepared SMS (also named MTS) and OTS (0.5% octadecyltrichlorosilane, wt/wt%, toluene as a solvent) and their static water contact angles measured were 150.82° ± 0.82° and 150.7° ± 0.88°, respectively. Then, the OTS-MS-MTS was prepared by step-by-step synthesis method and its static water contact angles increased to 155.43° ± 0.94°. When the OTS-MS-MTS was immersed in hydrochloric acid aqueous solution (pH = 1) and sodium hydroxide aqueous solution (pH = 14) for 24 h, its static water contact angle decreased to 147.3° ± 1.3° and 149.7° ± 1.4°, respectively. It suggests that SMS prepared here will almost maintain a good hydrophobicity in strong acid or strong alkali, however, strong acid and alkali have a certain corrosive effect on the sponge skeleton, which in turn affects the wettability of SMS. In addition, superhydrophobic melamine sponges (SMS) were prepared by in situ growth of nanosilica on melamine sponge skeletons followed by surface modification with hexadecyltrimethoxysilane (HTMS). This prepared SMS not only exhibits superhydrophobicity with a water contact angle of 152°, but also has a strong adsorption capacity of 42–105 times its own weight for various oils and organic reagents [24]. In a strong acid and strong base environment, the contact angle of this SMS reduces to a minimum of 120°, but the hydrophobicity still maintains. This indicates that the sponge skeleton can be corroded by strong acids, and the modified nano-silica grown on the skeleton falls off. While in an alkaline environment, sodium hydroxide and nano-silica on the SMS framework reacts to produce water-soluble sodium silicate, which reduces the contact angle of the SMS.
According to the previous study of thermogravimetric analysis (TGA) for SMS [4], the ∼4.0 wt% mass loss in the 30°C − 100°C range can be attributed to the evaporation of the absorbed water on the hydrophilic surface of the sponge. The main mass loss of ∼27.0 wt% that occurred in the temperature regions of 370°C − 405°C can be ascribed to the breakdown of the methylene bridges. Mass losses at temperatures higher than 405°C were attributed to the thermal decomposition of the triazine ring. In the present experiments of flame retardancy, the same masses (0.084 g) of a polypropylene foam, blank melamine sponge and SMS were put into a porcelain crucible, respectively, and then heated by alcohol blowtorch with the temperature up to 800°C. It is found that a bright and vigorous flame is instantaneously observed on the polypropylene foam, and it continues to burn completely, leaving no ash after 16 s. However, no flame is observed but cyan smoke is observed from the beginning on the blank melamine sponge, and it continues to degrade and deform after 28 s, finally leaving no ash after 207 s. Similarly, no flame is observed but cyan smoke is observed from the beginning on the SMS, and it continues to degrade and deform after 35 s, but finally leaving a grayish white residue of 7.62% of the original weight. Supplementary Fig. S2 shows the degradation photos of blank melamine sponge (a) and SMS (b) for 35 s at ∼800°C. It is interesting that the silylated melamine sponge can inherit the inherent flame retardant characteristics of melamine sponge and has been enhanced, and it is thus expected to reduce the risk of fire and explosion when being used as an absorbent for flammable oils and organic compounds [3].
3.6 Absorption Capacity of SMS for Oil in Oil-Water Mixture
Absorption capacity of SMS for oil in oil-water mixture was carried out by both gravimetric analysis and UV-vis spectrophotometry. Supplementary Fig. S3 shows the UV-vis overlapping spectra for different concentrations of petroleum diluted by n-hexane and the UV-vis standard regression curve for petroleum. The relative errors of the absorption capacity of SMS for diesel oil (0#) and crude oil between both the methods calculated are ±1.9% and ±2.7% respectively; while the relative error of the absorption capacity of SMS between for pure diesel oil (0#) and for diesel oil (0#) in oil-water mixture by gravimetric analysis calculated is ±4.5%, and the relative error of the absorption capacity of SMS between for pure crude oil and for crude oil in oil-water mixture by gravimetric analysis calculated is ±3.1%. These indicates that both methods of gravimetric analysis and UV-vis spectrophotometry are accurate if the relative error is limited to ±10%.
In addition, the average static absorption capacity of SMS for water measured is 0.7098 g/g at 25°C, which may be largely due to surface adhesion of water to SMS. However, the static absorption capacity of SMS for pure diesel oil (0#) is 101.9 g/g at 25°C, and the AEm of SMS in the process of absorbing diesel oil (0#) in oil-water mixture was calculated as 99.31% at 25°C; while the static absorption capacity of SMS for pure crude oil is 98.1 g/g at 40°C, and the AEm of SMS in the process of absorbing crude oil in oil-water mixture was calculated as 99.27% at 40°C. For solvents and oils investigated here, the AEm of SMS for them changes from 98.88% to 99.59% (wt/wt%), which is related to the polarity and density of solvents and oils. Moreover, the average static absorption capacity of SMS prepared by 1.0% (wt/wt%) of methyltrichlorosilane for water is 0.4122 g/g, and its absorption capacity for pure diesel oil (0#) is 97.2 g/g at 25°C, accordingly, the AEm for diesel oil (0#) in oil-water mixture calculated is 99.58% (wt/wt%), which is 0.27% higher than that of SMS prepared by 0.5% (wt/wt%) of methyltrichlorosilane. Similarly, its absorption capacity for crude oil is 90.9 g/g at 40°C, accordingly, the AEm for crude oil in oil-water mixture calculated is 99.55% (wt/wt%), which is 0.28% higher than that of SMS prepared by 0.5% (wt/wt%) of methyltrichlorosilane. In industrial production of SMS, ranges of 0.5%∼1.0 (wt/wt%) of methyltrichlorosilane will be recommended and selected. The data of gravimetric absorption capacity and AEm of SMS for oils are listed in Table 2. It is presumed that in the absorbent process for oil in oil-water mixture, a small amount of water adhered to SMS may be pushed away and replaced by oil dynamically so that an actually dynamic absorption efficiency is greater than the static absorption capacity calculated here. It should be noted that the absorption capacity can be measured by using a coulometer to test the moisture content in collected oil. In the experiment of PDMS-functionalized melamine sponge absorbing silicon oil, motor oil, octadecene and so on, the water content in the collected oils is measured by a coulometer and the purity of the collected samples is greater than 99.98% (the same purity as the unused liquids as purchased) [10].
3.7 Separation Efficiency of SMS for Saturated Aqueous Solution of Oil Dissolved in Water
NewPig® Absorbent Mat Pad composed of polypropylene is a commercial emergency material reserved by some Oilfield branches for oil-leakage absorption. Its Qm/m for crude oil at 40°C and for diesel oil at 25°C measured in our laboratory are 13.25 and 10.19 g/g, respectively (absorption data for other oils and solvents listed in Supplementary Table S1). Activated carbon is also a commonly commercial emergency material for oil-leakage absorption. The two materials are introduced into this experiment to compare their oil-absorption efficiency with SMS.
The UV-vis spectrophotometry is not very stable in measurement for oil content in its saturated aqueous solution, therefore, infrared spectrophotometry is introduced in this study. The spectra and the standard regression curve of infrared spectrophotometry for petroleum solved in tetrachloroethylene are shown as Supplementary Figs. S4a and S4b.
Figs. 6a and 6b show the separation efficiency of selected three absorbent materials for total oil and petroleum in 250 ml saturated aqueous solution of crude oil dissolved in water and saturated aqueous solution of diesel oil (0#) dissolved in water at a constant temperature of 25°C, respectively, and the separation efficiency data are listed in Supplementary Table S2. The initial concentrations of total oil and petroleum in saturated aqueous solution of crude oil are measured by infrared oil-measuring spectrophotometry as 17.24 and 16.93 mg/L, respectively; and the initial concentrations of total oil and petroleum in saturated aqueous solution of diesel oil (0#) are 59.76 and 55.87 mg/L, respectively, at 25°C. These differences between total oil and petroleum may be attributed to the fact that non-petroleum additives come from exploitation and refining processes.
Figure 6: The separation efficiency of selected three absorbent materials for total oil and petroleum in 250 ml saturated aqueous solution of crude oil solved in water (a) and saturated aqueous solution of diesel oil (0#) solved in water (b) at 25°C
It is interesting that SMS shows more superior performance of separation efficiency than the other two absorbent materials investigated here. In the present conditions (0.474 g SMS, 250 ml saturated aqueous solution of oil), separation efficiency of SMS for total oil or for petroleum is higher than 99.93%, and even reaches to 99.99%. From the trends of separation efficiency curve, it is presumed that the content of petroleum would be less than 0.5 mg/L (Standard limits of class IV of surface water environmental quality, National environmental protection standards of the people’s Republic of China, Environmental quality standards for surface water, GB 3838-2002), or the separation efficiency for petroleum would be more than 97.05% (wt/wt%) in the 250 ml saturated aqueous solution of crude oil, when the 1.0 g NewPig® Absorbent Mat Pad, 0.44 g (or 52.4 cm3) SMS or 9.6 g activated carbon is added in it, where the gravimetric absorption capacities are 4.1, 9.3 and 0.43 mg/g for NewPig® Absorbent Mat Pad, SMS and activated carbon, respectively; while the separation efficiency for petroleum would be more than 99.11% (wt/wt%) in the 250 ml saturated aqueous solution of diesel oil (0#), when the 3.3 g NewPig® Absorbent Mat Pad, 0.45 g (or 53.6 cm3) SMS or 5.6 g activated carbon is added in it, and where the gravimetric absorption capacities are 4.2, 30.8 and 2.5 mg/g for NewPig® Absorbent Mat Pad, SMS and activated carbon, respectively. Compared with the absorption capacity of SMS for pure crude oil and diesel oil (0#), this separation efficiency in saturated aqueous solution is much lower, especially in the conditions of small amount of absorbing material added, which is may be attributed to the phenomenon of O/W (oil/water) micelle state of the aqueous solution. In practical application, this phenomenon can be broken through demulsification, air flotation and adding a large amount of absorbents. However, these three absorbent materials can absorb and separate oils in their saturated aqueous solution well, and SMS shows better performance.
The superhydrophobic silylated melamine sponge (SMS) was prepared by melamine sponge coated by 0.5% (wt/wt%) of methyltrichlorosilane, where oligomeric silanol compounds covalently bonded by Si−N onto the surface of the melamine sponge skeletons. The surface of SMS has rough and papillary micro-, and nano-structures constructed by materials with low surface free energy, probably a hydrolytic self-aggregation product of methyltrichlorosilane. Its water contact angle is more than 150° ± 1°. The absorption capacity of SMS would decrease 50% (wt/wt%) after circulating absorption/extrusion of 600 times for p-xylene or 115 times for diesel oil (0#). The gravimetric absorption capacities (Qm/m) of SMS for common organic solvents and oils change from 62.7 to 172.2 g/g, exhibiting much superior adsorption property compared with the other types of functionalized melamine sponge except for Mercapto-functionalized polydopamine coated melamine sponge and Octadecylphosphonic acid (ODP)-co-TiO2 melamine sponge. However, the volumetric absorption capacity (Qv/v) of SMS is higher than that of Mercapto-functionalized polydopamine coated melamine sponge. More importantly, SMS shows more superior performance of separation efficiency than NewPig® Absorbent Mat Pad and activated carbon, where separation efficiency of SMS for total oil or petroleum is higher than 99.93%, and even reaches to 99.99% for oil in its saturated aqueous solution. In general, SMS exhibits excellent absorption performances, including a high porosity, excellent selectivity, high absorption capacity, better separation efficiency and remarkable recyclability. Additionally, it inherits the intrinsic flame-retardant nature of the melamine sponge, and is thus expected to reduce the risk of fire and explosion. Furthermore, the preparation of SMS is convenient and very suitable for large-scale industrial production.
Acknowledgement: We thank Northwestern University and Fanrui Yunzhi Technology (Zhengzhou) Co., Ltd., China, for their support in water contact angle measurement and scanning electron microscopy testing, respectively.
Funding Statement: This work was funded by Qingyang Science and Technology Support Project (KT2019-03), and Y. C. Liu, Y. C. Li, C. X. He and S. Y. Wang as authors received the grant for this study.
Author Contributions: The authors confirm contribution to the paper as follows: study conception and design: Y. C. Liu, W. W. Gao; data collection: Y. C. Liu, Y. L. Yang, C. C. Liu, R. X. Lei; analysis and interpretation of results: Y. C. Liu, N. Qiao, Y. C. Li, C. X. He, S. Y. Wang, W. Li; draft manuscript preparation: Y. C. Liu, Y. L. Yang, C. C. Liu, R. X. Lei. All authors reviewed the results and approved the final version of the manuscript.
Availability of Data and Materials: The authors confirm that the data supporting the findings of this study are available within the article and its supporting information. In addition, the data that support the findings of this study are available on request from the corresponding author, Y. C. Liu, upon reasonable request.
Conflicts of Interest: The authors declare that they have no conflicts of interest to report regarding the present study.
References
1. Adebajo, M. O., Frost, R. L., Kloprogge, J. T., Carmody, O., Kokot, S. (2003). Porous materials for oil spill cleanup: A review of synthesis and absorbing properties. Journal of Porous Materials, 10, 159–170. [Google Scholar]
2. Nguyen, D. D., Tai, N. H., Lee, S. B., Kuo, W. S. (2012). Superhydrophobic and superoleophilic properties of graphene-based sponges fabricated using a facile dip coating method. Energy & Environmental Science, 5, 7908–7912. [Google Scholar]
3. Ruan, C., Ai, K., Li, X., Lu, L. (2014). A superhydrophobic sponge with excellent absorbency and flame retardancy. Angewandte Chemit-International Edition, 53, 5556–5560. [Google Scholar] [PubMed]
4. Pham, V. H., Dickerson, J. H. (2014). Superhydrophobic silanized melamine sponges as high efficiency oil absorbent materials. ACS Applied Materials & Interfaces, 6, 14181–14188. [Google Scholar]
5. Miwa, M., Nakajima, A., Fujishima, A., Hashimoto, K., Watanabe, T. (2000). Effects of the surface roughness on sliding angles of water droplets on superhydrophobic surfaces. Langmuir, 16, 5754–5760. [Google Scholar]
6. Nosonovsky, M., Bhushan, B. (2007). Biomimetic superhydrophobic surfaces: Multiscale approach. Nano Letters, 7, 2633–2637. [Google Scholar] [PubMed]
7. Patankar, N. A. (2004). Mimicking the lotus effect: Influence of double roughness structures and slender pillars. Langmuir, 20, 8209–8213. [Google Scholar] [PubMed]
8. Zhu, Q. (2014). Fabrication and oil-water separation performance of superhydrophobic polyurethane (PU) sponges. Harbin Institute of Technology, Harbin, China (In Chinese). [Google Scholar]
9. Ma, M., Hill, R. M. (2006). Superhydrophobic surfaces. Current Opinion in Colloid & Interface Science, 11, 193–202. [Google Scholar]
10. Chen, X., Weibel, J. A., Garimella, V. (2016). Continuous oil-water separation using polydimethylsiloxane functionalized melamine sponge. Industrial & Engineering Chemistry Research, 55, 3596–3602. [Google Scholar]
11. Liu, S. H. (2021). Preparation and superhydrophobic bis-silane modified melamine sponge and its oil slick removal performance, pp. 25–49. Shandong University, Jinan, China (In Chinese). [Google Scholar]
12. Barthlott, W., Neinhuis, C. (1997). Purity of the sacred lotus, or escape from contamination in biological surfaces. Planta, 202, 1–8. [Google Scholar]
13. Neinhuis, C., Barthlott, W. (1997). Characterization and distribution of water-repellent, self-cleaning plant surfaces. Annals of Botany, 79, 667–677. [Google Scholar]
14. Shirgholami, M. A., Shateri-Khalilabad, M., Yazdanshenas, M. E. (2013). Effect of reaction duration in the formation of superhydrophobic polymethylsilsesquioxane nanostructures on cotton fabric. Textile Research Journal, 83, 100–110. [Google Scholar]
15. Wang, W., Sui, L. L., Li, Z. Q., Li, Y., Yu, H. W. (2016). Study on the stretching vibration of silicon nitride (Si-N) molecule by ATR-FTIRS. Physical Testing and Chemical Analysis Part B: Chemical Analysis, 52, 1241–1245 (In Chinese). [Google Scholar]
16. Bi, H., Yin, Z., Cao, X., Xie, X., Tan, C. et al. (2013). Carbon fiber aerogel made from raw cotton: A novel, efficient and recyclable sorbent for oils and organic solvents. Advanced Materials, 25, 5916–5921. [Google Scholar] [PubMed]
17. Zhou, X., Zhang, Z., Xu, X., Men, X., Zhu, X. (2013). Facile fabrication of superhydrophobic sponge with selective absorption and collection of oil from water. Industrial & Engineering Chemistry Research, 52, 9411–9416. [Google Scholar]
18. Xu, Z., Miyazaki, K., Hori, T. (2015). Dopamine-induced superhydrophobic melamine foam for oil/water separation. Advanced Materials Interfaces, 2, 1500255. [Google Scholar]
19. Zhou, J., Guo, J., Yan, H., Xiao, J., Wang, J. (2021). Reversible wettability switching of melamine sponges for oil/water separation. Materials Chemistry and Physics, 257, 123772. [Google Scholar]
20. Hong, P., Liu, Z., Gao, Y., Chen, Y., Zhuang, M. et al. (2019). Fabricated of superhydrophobic silanized melamine sponge with photochromic properties for efficiency oil/water separation. Advances in Polymer Technology, 2019, 1–8. [Google Scholar]
21. Yin, Z., Li, Y., Song, T., Bao, M., Li, Y. et al. (2020). An environmentally benign approach to prepare superhydrophobic magnetic melamine sponge for effective oil/water separation. Separation and Purification Technology, 236, 116308. [Google Scholar]
22. Xue, J., Zhu, L., Zhu, X., Li, H., Ma, C. et al. (2021). Tetradecylamine-MXene functionalized melamine sponge for effective oil/water separation and selective oil adsorption. Separation and Purification Technology, 259, 118106. [Google Scholar]
23. Zhou, J., Zhang, Y., Yang, Y., Chen, Z., Jia, G. et al. (2019). Silk fibroin-graphene oxide functionalized melamine sponge for efficient oil absorption and oil/water separation. Applied Surface Science, 497, 143762. [Google Scholar]
24. Li, Y., Zhou, M., Li, C., Han, H. C., Tu, H. J. (2023). In situ construction of green multiscale nanosilicon-based sponges for stable oil-water separation. Environmental Technology. https://doi.org/10.1080/09593330.2022.2161948 [Google Scholar] [PubMed] [CrossRef]
Supporting Materials
Figure S1: The FT-IR spectra of blank melamine sponge (a) and SMS (b)
Figure S2: The degradation photos of blank melamine sponge (a) and SMS (b) for 35 s at ∼800°C
Figure S3: The UV-vis overlapping spectra for different concentrations of petroleum diluted by n-hexane and the UV-vis standard regression curve for petroleum. The concentration of petroleum ranges from 2.0 to 36.0 mg/L
Figure S4: The spectra (a) and the standard regression curve (b) of infrared spectrophotometry for standard petroleum dissolved in tetrachloroethylene. The scanning wave number ranges from 3400 to 2400 cm−1
Cite This Article
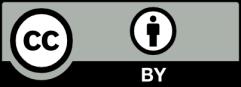