Open Access
ARTICLE
Insecticidal Activity and Physicochemical Characterization of Nanoparticles from Foliar Extract of Capsicum chinense
1
Division of Postgraduate Studies and Research, Tecnológico Nacional de México, Campus Conkal, Conkal, Yucatán, 97345, Mexico
2
Institute of Agricultural Sciences, Universidad Autónoma de Baja California, Ejido Nuevo León, Mexicali, Baja California, 21705,
Mexico
3
Instituto de Ingeniería, Universidad Autónoma de Baja California, Mexicali, Baja California, 21100, Mexico
4
Division of Postgraduate Studies and Research, Tecnológico Nacional de México, Campus Tuxtla Gutiérrez, Tuxtla Gutiérrez,
Chiapas, 29050, México
* Corresponding Author: Esaú Ruiz-Sánchez. Email:
Journal of Renewable Materials 2023, 11(11), 3933-3943. https://doi.org/10.32604/jrm.2023.031129
Received 16 May 2023; Accepted 10 July 2023; Issue published 31 October 2023
Abstract
This work was carried out to evaluate the insecticidal effect of mono, bi and trimetallic nanoparticles (NPs) from leaf extract of habanero pepper (Capsicum chinense Jacq.) on the red flour beetle (Tribolium castaneum herbst.) and cotton mealybug (Phenacoccus solenopsis Tinsley). The results showed that Cu NPs, Cu/Mn NPs and Zn/Cu NPs had high insecticidal effect against T. castaneum (63%–80% mortality after 96 h). The Cu NPs and Zn/Cu NPs showed also insecticidal effect against P. solenopsis, but to a lesser extent (20%–28% after 96 h). With regards to the physicochemical characterization, Cu NPs had a zeta potential of 21.1 mV, whereas Cu/Mn NPs and Zn/Cu NPs exhibited zeta potentials of 200 mV. The polydispersity index (PDI) values for Cu NPs, Cu/Mn NPs, and Zn/ Cu NPs were 10.1, 29.16, and 14.34, respectively. The TF-IR spectra of the NPs varied from 600 to 4000 cm−1 . The EDX weight percentages showed the presence of Cu (0.29%–2.4%), Mn (1.4%), and Zn (2.6%) in Cu/Mn NPs and Zn/Cu NPs, respectively.Graphical Abstract
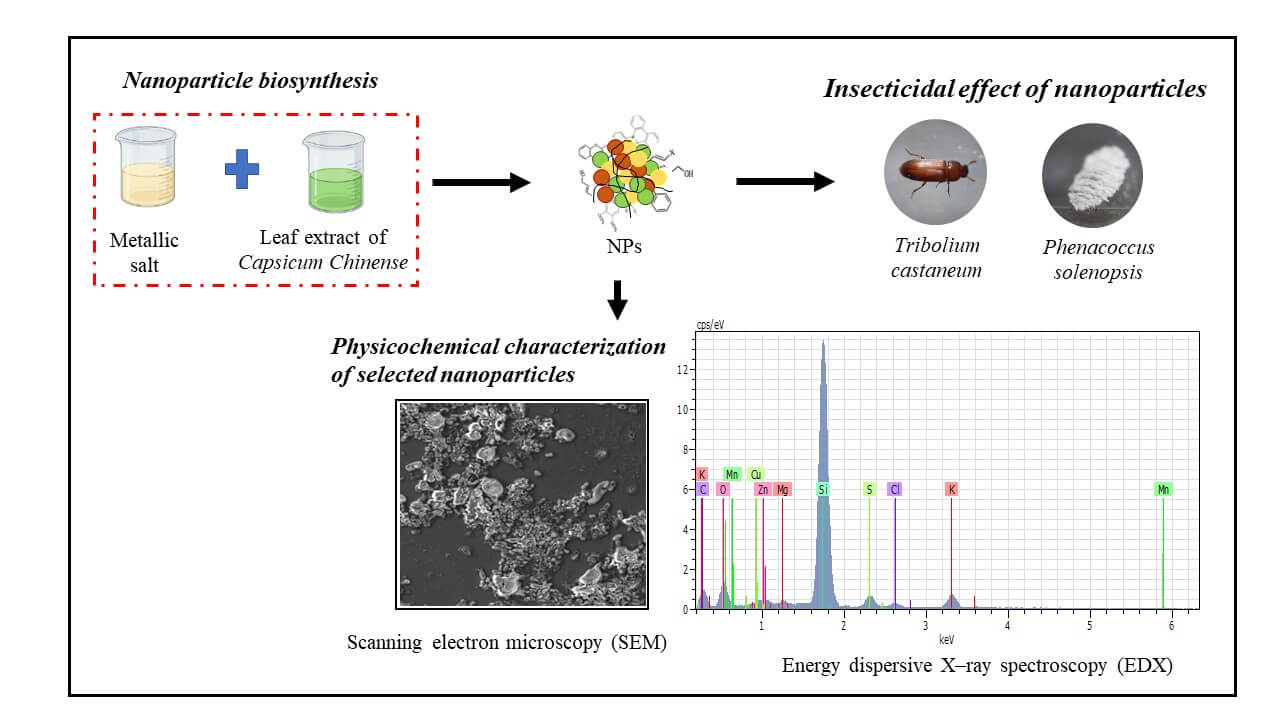
Keywords
Pepper (Capsicum spp.) is cultivated in temperate and warm climate zones around the world. Pepper fruits are rich in phytochemicals such as vitamins, capsaicinoids, carotenoids, and polyphenols, highly valued in human nutrition and the food industry [1,2]. Approximately 35 species have been reported in the genus Capsicum, but only Capsicum baccatum, C. chinense, C. frutescens, C. pubescens, and C. annuum have been cultivated [3,4]. Habanero pepper (C. chinense) was domesticated in South America and the Caribbean, where it is currently one of the most important pepper species [5,6]. This pepper is consumed directly or industrialized as powder, paste and sauce. In addition, its bioactive compounds and natural ingredients are also used for the agro-food, cosmetic and pharma industry [7–9].
Fruit extracts of C. chinense have important properties for plant protection. Fruit extracts are toxic to a wide variety of phytopathogenic fungi and phytophagous insects. The antifungal activities of fruit extracts include the inhibition of growth of the phytopathogenic fungi Sclerotinia sclerotiorum, Rhizopus stolonifer, Colletotrichum gloeosporioides, Fusarium andiyazi, and Cochliobollus spp. [10–12]. Purified metabolites from fruit extracts have also demonstrated high antifungal activity against a wide range of fungi. For example, peptide fractions have activity against Fusarium oxysporum, Fusarium solani, Fusarium lateritium, Colletotrichum lindemuthianum, and Colletotrichum gloeosporioides [13,14]. Fruit extracts of C. chinense have been also shown to be effective against insect pests, such as Aphis cytisorum, Aphis craccivora, various biotypes of Bemisia tabaci, Trichopulsia ni, and Sitophilus zeamais [15–18].
In contrast to the study of fruit extracts of C. chinense, research on leaf extracts is limited, but there is evidence of antifungal effects against Botrytis spp. [19]. Leaf extracts of other species of Capsicum, mainly C. annuum, are effective against various species of phytopathogenic fungi, such as Alternaria alternata, Rhizoctonia solani, Sclerotinia minor and Verticillium dahliae [20,21]. In addition, high effects against phytophagous insects, such as Spodoptera littoralis, Callosobruchus maculatus, and Callosobruchus chinensis [22,23]. The activity of Capsicum spp. leaf extracts may be attributed to alkaloids, flavonoids, terpenoids, resins, phenols, steroids and tannins, which are the predominant metabolites in Capsicum spp. leaves [24–26].
The use of pepper leaves as a subproduct is a feasible option as in commercial pepper production, only the fruit is traded, while the rest of the plant is considered agricultural waste [27]. The dry weight of the leaves could be 50% approximate of the total weight of the plant [28], so the use of the leaves can be an option as a source of botanical pesticides in agriculture [29]. To increase the effectiveness of plant-derived extracts on plant pests, one of the alternatives is the green synthesis of phytonanoparticles from metal ions, where the metabolites of the plant extracts serve as reducing agents. In the present study, we synthesized phytonanoparticles (NPs) using mono, bi, and trimetallic ions and aqueous leaf extracts of C. chinense as a reductor agent. The insecticidal effects of NPs on T. castaneum and P. solenopsis were evaluated, and the most active NPs characterized.
2.1 Preparation of Plant Extracts
The leaves of C. chinense were collected in Conkal, Yucatan, Mexico, located at 21°04′24″N and 89°31′15″W and elevation of 10 m above sea level. Leaves of plants in fruiting stage (120 days after transplant) were rinsed with neutral detergent and afterwards with deionized distilled water. Leaves were dried in an oven for 6 days at 60°C and subsequently ground in a laboratory mill Wiley Thomas Scientific 4. The aqueous extract was prepared using 30 g of dried leaves in 300 ml of distilled water, heated at 50°C for 20 min and left stirring for 24 h at 28°C. Then, the extract was centrifuged at 5,000 g for 10 min to remove impurities and obtain a clear solution. This solution was stored at 4°C until use.
For the synthesis of metallic nanoparticles, the metallic salt copper sulfate, zinc sulfate and manganese sulfate were used for Cu, Zn and Mn NPs, respectively. The procedure was performed as follows: an aliquot of 10 mL aqueous extract of C. chinense leaves was mixed with 50 mL of distilled water that contained 10 mM of the metallic, bimetallic or trimetallic salt in 100 mL Erlenmeyer flask and heated at 60°C for 30 min. The bioreduction of metals and the formation of NPs were visually determined by observing the color change of the aqueous extract.
2.3 Insecticidal Effect of Nanoparticles
For bioassays, NPs were considered as the experimental treatments. For positive control and blank control the pure aqueous leaf extract and distilled water were used, respectively. To evaluate the insecticidal activity of the NPs, newly emerged female adults of P. solenopsis were collected from infected plants of C. chinense in the Mexicalli Valley located in the state of Baja California Mexico. A colony of T. castaneum were raised in wheat flour at the biotechnology laboratory of the Institute of Agricultural Sciences of the Autonomous University of Baja California at Mexicali. For bioassay, newly emerged adults were used. Both insect species were placed in Petri dishes (60 mm by 15 mm) and kept in the aforementioned laboratory, where 2 mL of a solution containing phytonanoparticles or distilled water (control) was then sprayed by hand using a 10 mL hand atomizer (Varienvases, Mexico). For P. solenopsis and T. castaneum, a completely randomized design with three and five replications was used, respectively, with the experimental unit consisting of a Petri dish containing 10 and 5 adult individuals, respectively. All Petri dishes were placed in a growth room at 27 ± 3°C and 16 h of light. The number of survivors of P. solenopsis and T. castaneum was reported up to 96 h after the initial application. On the basis of the muscle response of the insects to a modest needle prick, the insects’ mortality was determined.
2.4 Physicochemical Characterization of Selected Nanoparticles
The most effective NPs of the insecticide evaluations (Cu NPs, Cu/Mn NPs and Zn/Cu NPs) were selected for physicochemical characterization. The morphological characterization and elemental composition were carried out by scanning electron microscopy—energy dispersive spectra (SEM-EDS) in a JEOL-6010LA (MA, EU). The particle size distribution and the Z–potential of NPs in solution were analyzed by dynamic light scattering (DLS) in a Nanotrac Wave Instrument (PA, EU). Fourier transform infrared (FT-IR) spectrum measurements were performed to identify the molecules responsible for reducing and stabilizing the NPs, and energy-dispersive X–ray spectroscopy (EDX) was used to determine the chemical composition of NPs.
As the insect mortality data did not meet the conditions for conducting an analysis of variance, these data were analyzed by generalized linear models, using the Poisson family for all treatments with the sqrt link function. The Bonferroni test was used for the comparison of means.
The analysis of mortality showed that T. castaneum was found to be more sensitive than P. solenopsis to C. chinense NPs. In T. castaneum, the highest mortality (X2(13, 28) = 96.15, p < 0.01) was observed when applied Cu NPs, Cu/Mn NPs and Zn/Cu NPs. Insects treated with Cu NPs, Cu/Mn NPs, and Zn/Cu NPs displayed 63% to 80% mortality after 96 h of treatment. The crude extract caused 53.3% mortality (Fig. 1A). In P. solenopsis, the highest mortality (X2(13, 56) = 58.78, p < 0.01) was caused by Cu NPs and Zn/Cu NPs. Insects treated with Cu NPs and Zn/Cu NPs displayed 20% to 28% mortality after 96 h of treatment. The pure extract did not have insecticide activity (Fig. 1B).
Figure 1: Percentage mortality of (A) T. castaneum and (B) P. solenopsis caused by NPs synthesized from C. chinense leaf extract at 96 h after application. The bars are means ± standard error. Different letters above the bars indicate statistically significant difference (GML, p < 0.01)
3.2 Physical Characterization of Nanoparticles
The particle size distribution in colloidal solution and the Z potential of NPs were determined using dynamic light scattering (DLS) analysis for treatments (Cu NPs, Cu/Mn NPs and Zn/Cu NPs) that had the largest insecticide effect. The DLS results showed Z potentials of 21.1 for Cu NPs, and 200 mV for Cu/Mn NPs and Zn/Cu NPs (Fig. 2). Regarding the hydrodynamic size, Cu NPs, Cu/Mn NPs, and Zn/Cu NPs had polydispersity index (PDI) values of 10.1, 29.16 and 14.34, respectively, with size ranges of 28.1 to 4190 nm, 85.8 to 3110 nm and 17.74 to 5830 nm, respectively.
Figure 2: Particle size distribution in colloidal solution and Z potential of NPs using dynamic light scattering (DLS) in (A) Cu NPs; (B) Cu/Mn NPs, and (C) Zn/Cu NPs
The TF–IR spectra of the NPs ranged from 600 to 4000 cm−1 (Fig. 3). The infrared spectrum of pure C. chinense leaf extract revealed bands at 3743.7, 3291.84, 2930.3, 1625.65, 1396.5, 1121.1, and 616.96 cm−1 that corresponded to free alcohols, O–H stretching (alcohols/phenols), C–H stretching (alkanes), C=C stretching (alkenes), C=C stretching (aromatic rings), C–N stretching, and C–H bending (Fig. 3). All infrared spectra of NPs were comparable to that observed by the pure leaf extract; however, a new band corresponding to the C–N stretch appeared between 2319.8 and 2322.4 cm−1. Cu NPs and Zn/Cu NPs exhibited irregular shapes with sizes ranging from 1.3 to 1.9 µm, whereas Cu/Mn NPs exhibited an oval shape with diameters ranging from 1 to 1.9 µm (Fig. 4).
Figure 3: Infrared spectra (TF-IR) of (A) Crude leaf extract of Capsicum chinense; (B) Cu NPs; (C) Cu/Mn NPs; (D) Zn/Cu NPs
Figure 4: Analysis of scanning electron microscopy (SEM) of the NPs of (A) Cu NPs; (B) Cu/Mn NPs; (C) Zn/Cu NPs
The EDX analysis confirmed the presence of Cu in the three analyzed NPs (0.29%–2.4%), as well as Mn (1.4%) and Zn (2.65%) for Cu/Mn NPs and Zn/Cu NPs, respectively (Figs. 5A–5C). Si (46.76%–66.29%), O (11.42%–13.61%), Cl (0.93%–4.16%), and Mg (0.22%–0.55%) were the main elements in the three analyzed NPs. There was also presence of C in Cu NPs and Cu/Mn NPs, S in Cu NPs and Zn/Cu NPs, Na in Cu NPs, and Zn in Cu/Mn NPs.
Figure 5: Chemical composition of the selected metallic NPs synthesized from C. chinense leaf extract. The analysis was performed by energy dispersive X–ray spectroscopy (EDX) on (A) Cu NPs; (B) Cu/Mn NPs and (C) Zn/Cu NPs
Ours results showed that T. castaneum was more susceptible than P. solenopsis when exposed to metallic nanoparticles from C. chinense leaf extract. This difference may be the result of the wax layer covering the body of P. solenopsis, which prevents the penetration of xenobiotics trough the cuticle [30]. In other studies, NPs have had differential effects, for example, the Cu/Zn NPs from leaf extract of Crotalaria longirostrata produced 65% mortality of P. solenopsis [31], whereas the Cu NPs from leaf extract of Prosopis juliflora and Pluchea sericea caused 14% and 20% mortality, respectively [32]. This suggests that the effects of the NPs from plant leaves depend on the plant species and the metallic salt used for the synthesis. In the present study, the EDX analysis showed that Cu was present within the NPs of the most effective treatments against both, T. castaneum and P. solenopsis. This is well known that Cu salts and derived products have effects against plant pathogens and phytophagous arthropods such as mites. The mode of action of Cu has been related to permeabilization and disruption of the plasma membrane, alteration of enzymatic active sites and interference with the energy transport system [33,34]. This is important to note that the effect of the Cu NPs in this case depended also on the number of metal ions in the synthesis. We observed a trend of higher insecticidal activity of mono- and bimetallic NPs compared to that of trimetallic NPs, suggesting that there were no synergistic effects of the metal ions on the bioactivity of the plant metabolites as observed against other target organisms [35–37]. Bimetallic and trimetallic nanoparticles have gained great attention from a toxicological point of view. Synergistic effects of two metals in bimetallic and three metal ions in trimetallic nanoparticles enable certain functions which are otherwise not possible with monometallic nanoparticles alone. Bimetallic and trimetallic nanoparticles may show different properties and consequently biological applications due to their peculiar mixing patterns [38,39].
4.2 Characterization of Nanoparticles
The most effective NPs in the insecticidal evaluation (Cu NPs, Cu/Mn NPs and Zn/Cu NPs) were selected for physicochemical characterization. The Z-potential is an analytical technique that predicts the stability of the NPs in colloidal solution by their surface charge. The Z-potential calculated for the selected NPs showed that the Cu/Mn NPs and Zn/Cu NPs were highly stable (200 mV), whereas the Cu NPs had low stability (21.1 mV). NPs with Z-potential values of ±30 mV have high repulsive forces that prevent their aggregation [40]. In addition, the polydispersity index (PDI) was high in all NPs, with values of 10–30. In this sense, values higher to 1 indicate high polydispersity [41]. Taken together, these results suggest that the Cu/Mn NPs and Zn/Cu NPs were highly stable. The stability of the NPs is given in part by the biomolecules present in the plant extract, which serve as reducing agents at the time of synthesis, in addition to the interaction of the extract metabolites with the metal ions, where it is suggested that a better stability is usually achieved when two different metals are combined [42,43]. Our results are lined up with this finding as the bimetallic NPs presented higher stability (200 mV) than the monometallic one (21 mV).
Fourier transform infrared spectroscopy (FT-IR) analysis was performed to identify the active functional groups responsible for stabilizing the NPs synthesized. For pure leaf extract and the NPs, clear visible peaks showed the presence of free alcohols (alcohols/phenols), alkanes, alkenes and aromatic rings in the pure extract. In addition to aliphatic amines (alkaloids) present in the NPs. These active phytochemicals reduced the metallic salts into NPs and stabilized the synthesized NPs. In agreement with these findings, previous studies have found that C. chinense leaf extracts contain a wide range of phytochemicals, mainly phenolic compounds, and flavonoids [44]. In particular, various phytosterol, like phytol, ergosterol, caryophyllene, and also fatty acid ethyl esters have been identified in leaf extracts of species of Capsicum [26].
Regarding the size and shape of the NPs, we found irregular and oval shapes with sizes ranging from 1 to 1.9 µm. These NPs were larger compared to those found by Lomelí-Rosales et al. [27] using C. chinense extracts for the synthesis of Ag NPs and Au NPs, where the sizes ranged 15 to 16.7 nm with oval shapes. However, using bimetallic NPs, Mendez-Trujillo et al. [31,45] found that Cu/Zn NPs based on P. juliflora and C. longirostrata had sizes from 0.41 to 0.74 µm and spherical shape. Thus, this is the first report using copper in green synthesis NPs from C. chinense.
The elemental composition of the NPs determined by EDX analysis confirmed the presence of Cu in the three analyzed NPs. In addition, Mn and Zn were also found in Cu/Mn NPs and Cu/Zn NPs, respectively. This suggests that these elements participated in the synthesis of the NPs. The presence of Si, O, K, S, Cl and Mg in all the characterized NPs may be due to the mineral composition of the C. chinense extract. The presence of some minerals, such as Na, K and Mg in leaf extracts of Capsicum spp. has been reported [46].
In our study, we observed that the synthesized metallic NPs of C chinense leaf extracts had insecticidal effects against T. castaneum, and to a lesser extent to P. solenopsis. The most effective NPs (Cu NPs, Cu/Mn NPs and Zn/Cu NPs) were selected for physicochemical characterization. The SEM analysis showed that Cu NPs and Zn/Cu NPs exhibited irregular shapes with sizes ranging from 1.3 to 1.9 µm, whereas Cu/Mn NPs exhibited an oval shape with diameters ranging from 1 to 1.9 µm. The infrared spectra showed varios peaks at the range of 600 to 4000 cm−1. The EDX analysis revealed the presence of Cu (0.29%–2.4%), Mn (1.4%) and Zn (2.60%) in Cu NPs, Cu/Mn NPs and Zn/Cu NPs. The green synthesis of NPs from C. chinense leaf extracts is a feasible alternative to search for new strategies to manage the insect pests T. castaneum and P. solenopsis. However, more studies are needed to determine the impact of NPs on non-target insects.
Acknowledgement: The authors thank the Mexican Council for Science and Technology (CONACYT) for the PhD scholarship awarded to Arnoldo E. Alfaro-Corres.
Funding Statement: This work was funded by Tecnológico Nacional de México (TecNM), research call 2023, Specific Grant Numer 17932.23-P. The grant was received by Esaú Ruiz-Sánchez to carry out the research project “Efecto insecticida y caracterización físicoquímica de fitonanopartículas metálicas sintetizadas a partir de extractos vegetales acuosos”.
Author Contributions: The authors confirm contribution to the paper as follows: study conception and design: Arnoldo E. Alfaro-Corres, Daniel González-Mendoza, Esaú Ruiz-Sánchez; data collection: Arnoldo E. Alfaro-Corres, Daniel González-Mendoza, Carlos Ail-Catzin, Benjamín Valdez-Salas; analysis and interpretation of results: Esaú Ruiz-Sánchez, Federico Gutiérrez-Miceli, Arturo Reyes-Ramírez; draft manuscript preparation: Arnoldo E. Alfaro-Corres Esaú Ruiz-Sánchez, Jacques Fils Pierre. All authors reviewed the results and approved the final version of the manuscript.
Availability of Data and Materials: The data that support the findings of this study are available from the corresponding author [Esaú Ruiz-Sánchez, Email: esau.ruiz@itconkal.edu.mx], upon reasonable request.
Conflicts of Interest: The authors declare that they have no conflicts of interest to report regarding the present study.
References
1. De Aguiar, A. C., Viganó, J., da Silva Anthero, A. G., Dias, A. L. B., Hubinger, M. D. et al. (2022). Supercritical fluids and fluid mixtures to obtain high–value compounds from Capsicum peppers. Food Chemistry: X, 13(2), 100228. https://doi.org/10.1016/j.fochx.2022.100228 [Google Scholar] [PubMed] [CrossRef]
2. Pappalardo, I., Santarsiero, A., de Luca, M., Acquavia, M. A., Todisco, S. et al. (2021). Exploiting the anti-inflammatory potential of white Capsicum extract by the nanoformulation in phospholipid vesicles. Antioxidants, 10(11), 1683. https://doi.org/10.3390/antiox10111683 [Google Scholar] [PubMed] [CrossRef]
3. Olatunji, T. L., Afolayan, A. J. (2019). Evaluation of genetic relationship among varieties of Capsicum annuum L. and Capsicum frutescens L. in West Africa using ISSR markers. Heliyon, 5(5), e01700. https://doi.org/10.1016/j.heliyon.2019.e01700 [Google Scholar] [PubMed] [CrossRef]
4. Rudrapal, M., Sarwa, K. K. (2020). Capsicum: Chemistry and medicinal properties of indigenous Indian varieties. In: Dekebo, A. (Ed.Capsicum, pp. 1–9. London, UK: IntechOpen. [Google Scholar]
5. Ramjattan, R., Umaharan, P. (2021). Interrelationships between yield and its components in hot pepper (Capsicum chinense Jacq.). Scientia Horticulturae, 287(3), 110254. https://doi.org/10.1016/j.scienta.2021.110254 [Google Scholar] [CrossRef]
6. Ramjattan, R., Umaharan, P. (2022). Selection criteria for yield in hot pepper (Capsicum chinense Jacq.). New Zealand Journal of Crop and Horticultural Science, 50(2), 1–17. https://doi.org/10.1080/01140671.2022.2058966 [Google Scholar] [CrossRef]
7. Kumar, R., Malik, A., Kumar, S. (2022). Chilli (Capsicum chinense Jacq.) production and post-harvest management: An overview. The Pharma Innovation Journal, 11(5), 752–756. [Google Scholar]
8. Von Hagen, R. L., Norris, P., Schulte, B. A. (2020). Quantifying capsaicinoids from chili pepper and motor oil mixtures used in elephant deterrent fences. Chromatographia, 83(9), 1153–1157. https://doi.org/10.1007/s10337-020-03934-8 [Google Scholar] [CrossRef]
9. Cirlini, M., Luzzini, G., Morini, E., Folloni, S., Ranieri, R. et al. (2019). Evaluation of the volatile fraction, pungency and extractable color of different Italian Capsicum annuum cultivars designed for food industry. European Food Research and Technology, 245(12), 2669–2678. https://doi.org/10.1007/s00217-019-03378-x [Google Scholar] [CrossRef]
10. Santos, L. S., Fernandes, C. C., Santos, L. S., Dias, A. L. B., Souchie, E. L. et al. (2022). Phenolic compounds and antifungal activity of ethyl acetate extract and methanolic extract from Capsicum chinense Jacq. ripe fruit. Brazilian Journal of Biology, 84(1), e258084. https://doi.org/10.1590/1519-6984.258084 [Google Scholar] [PubMed] [CrossRef]
11. Toigo, S. E. M., Fernandes, C. C., Miranda, M. L. D. (2022). Promising antifungal activity of two varieties of Capsicum chinense against Sclerotinia sclerotiorum, Rhizopus stolonifer and Colletotrichum goleosporoides. Food Science and Technology, 42(3), e52722. https://doi.org/10.1590/fst.52722 [Google Scholar] [CrossRef]
12. López-Muñoz, N. R., Romero-Bastidas, M., Arce-Amézquita, P. M., Hernández-Rubio, J. S. (2019). Actividad antifúngica de antioxidantes derivados de cuatro cultivares de Capsicum spp. Contra hongos fitopatógenos. Ecosistemas y Recursos Agropecuarios, 6(18), 487–498. https://doi.org/10.19136/era.a6n18.2174 [Google Scholar] [CrossRef]
13. Resende, L. M., de Oliveira Mello, É., de Lima Aguieiras, M. C., Shiniti Nagano, C., Pinheiro chaves, R. et al. (2021). Inhibition of serine protease, α–amylase and growth of phytopathogenic fungi by antimicrobial peptides from Capsicum chinense fruits. Probiotics and Antimicrobial Proteins, 15(3), 502–515. https://doi.org/10.1007/s12602-021-09865-6 [Google Scholar] [PubMed] [CrossRef]
14. de Azevedo Dos Santos, L., Taveira, G. B., da Silva, M. S., da Silva Gebara, R., da Silva Pereira, L. et al. (2020). Antimicrobial peptides from Capsicum chinense fruits: Agronomic alternatives against phytopathogenic fungi. Bioscience Reports, 40(8), BSR20200950. https://doi.org/10.1042/BSR20200950 [Google Scholar] [PubMed] [CrossRef]
15. Diallo, A., Sakho, A., Keita, N., Bangoura, L., Bah, A. et al. (2022). Ethnobotanical investigation of insecticidal plants and evaluation of the insecticidal activity of Capsicum chinense on Sitophilus zeamaïs of maize and Aphis craccivora of groundnuts: Case of Boffa, Boké Region (Republic of Guinea). Journal of Agricultural Chemistry and Environment, 11(2), 132–142. https://doi.org/10.4236/jacen.2022.112009 [Google Scholar] [CrossRef]
16. Claros Cuadrado, J. L., Pinillos, E. O., Tito, R., Mirones, C. S., Gamarra Mendoza, N. N. (2019). Insecticidal properties of capsaicinoids and glucosinolates extracted from Capsicum chinense and Tropaeolum tuberosum. Insects, 10(5), 132. https://doi.org/10.3390/insects10050132 [Google Scholar] [PubMed] [CrossRef]
17. Castillo-Sánchez, L. E., Jiménez-Osornio, J. J., Delgado-Herrera, M. A. (2012). Actividad biológica in vitro del extracto de Capsicum chinense Jacq. contra Bemisia tabaci Genn. Revista Chapingo Serie Horticultura, 18(3), 345–356. https://doi.org/10.5154/r.rchsh.2011.04.016 [Google Scholar] [CrossRef]
18. Antonious, G. F., Meyer, J. E., Rogers, J. A., Hu, Y. H. (2007). Growing hot pepper for cabbage looper, Trichopulsia ni (Hübner) and spider mite, Tetranychus urticae (Koch) control. Journal of Environmental Science and Health, Part B, 42(5), 559–567. https://doi.org/10.1080/03601230701389512 [Google Scholar] [PubMed] [CrossRef]
19. Ahmed, F. A., Sipes, B. S., Alvarez, A. M. (2016). Natural products to control postharvest gray mold of tomato fruit—Possible mechanisms. Journal of Plant Pathology & Microbiology, 7(7), 1000367. https://doi.org/10.4172/2157-7471.1000367 [Google Scholar] [CrossRef]
20. Pane, C., Fratianni, F., Caputo, M., Parisi, M., Nazzaro, F. et al. (2015). Antifungal activity of wild Capsicum foliar extracts containing polyphenols against the phytopathogens Alternaria alternata, Rhizoctonia solani, Sclerotinia minor and Verticillium dahliae. In: Mendez-Vilas, A. (Ed.Multidisciplinary approach for studying and combating microbial pathogens, pp. 34–38. Boca Raton: Brown Walker Press. [Google Scholar]
21. Pane, C., Fratianni, F., Parisi, M., Nazzaro, F., Zaccardelli, M. (2016). Control of Alternaria post-harvest infections on cherry tomato fruits by wild pepper phenolic-rich extracts. Crop Protection, 84, 81–87. https://doi.org/10.1016/j.cropro.2016.02.015 [Google Scholar] [CrossRef]
22. Ahmed, W. H., Atwa, W. A., Elshaier, M. E., Abdullah, G. E. (2021). Toward efficient and safe control strategy against cotton leaf worm Spodoptera littoralis (Boisd.) (Lepidoptera: Noctuidae) applying onion and pepper extracts and their oils. Al-Azhar Bulletin of Science: Section C, 32(2), 9–15. https://doi.org/10.21608/absb.2021.89834.1129 [Google Scholar] [CrossRef]
23. Rajapakse, R. H. S., Ratnasekera, D. (2008). Pesticidal potential of some selected tropical plant extracts against Callosobruchus maculates (F.) and Callosobruchus chinensis (L.) (Coleoptera: Bruchidae). Tropical Agricultural Research and Extension, 11, 69–71. https://doi.org/10.4038/tare.v11i0.1793 [Google Scholar] [CrossRef]
24. Adepoju, A. O., Macaulay, M. M. R., Otomoso, I. O., Adewumi, A. G., Osundara, O. A. (2023). Compararative phytochemical, mineral, proximate and organic compound study of foliar extracts of Capsicum species with their antimicrobial potentials. Acta Scientifica Malaysia (ASM), 7(1), 23–30. https://doi.org/10.26480/asm.01.2023.23.30 [Google Scholar] [CrossRef]
25. Rosulu, H. O., Oni, M. O., Ofuya, T. I., Adebayo, R. A. (2022). Bioefficacy of chilli pepper Capsicum frutescens (L.) and it’s intercropping on the growth, yield and insect pest management of cowpea Vigna unguiculata (L.) walp in the rain forest area of Nigeria. Journal of Global Agriculture and Ecology, 13(2), 7–18. [Google Scholar]
26. Lobo-Echeverri, T., Salazar, L. C., Hernández, A., Ortiz-Reyes, A. (2016). Effects of Capsicum baccatum and C. frutescens against Atta cephalotes (Hymenoptera: Formicidae) and the symbiotic fungus Leucoagaricus gongylophorus. Revista Colombiana de Entomología, 42(2), 137–145. https://doi.org/10.25100/socolen.v42i2.6684 [Google Scholar] [CrossRef]
27. Lomelí-Rosales, D. A., Zamudio-Ojeda, A., Reyes-Maldonado, O. K., López-Reyes, M. E., Basulto-Padilla, G. C. et al. (2022). Green synthesis of gold and silver nanoparticles using leaf extract of Capsicum chinense plant. Molecules, 27(5), 1692. https://doi.org/10.3390/molecules27051692 [Google Scholar] [PubMed] [CrossRef]
28. Nawrin, K. S., Uddin, M. J., Ali, A. H. M. Z., Rahman, M. K. (2020). Effects of boron and vermicompost on growth, yield and nutrient content of chilli (Capsicum annum L.). Journal of Biodiversity Conservation and Bioresource Management, 6(1), 31–36. https://doi.org/10.3329/jbcbm.v6i1.51329 [Google Scholar] [CrossRef]
29. Amoabeng, B. W., Johnson, A. C., Gurr, G. M. (2019). Natural enemy enhancement and botanical insecticide source: A review of dual use companion plants. Applied Entomology and Zoology, 54, 1–19. https://doi.org/10.1007/s13355-018 [Google Scholar] [CrossRef]
30. Ulusoy, S., Kahya, D., Bilgin, M. G., Apalak, A. (2022). The effectiveness of wax secretion on chemical control in some mealybug species. Journal of Asia-Pacific Entomology, 25(3), 101954. https://doi.org/10.1016/j.aspen.2022.101954 [Google Scholar] [CrossRef]
31. Mendez-Trujillo, V., Valdez-Salas, B., Carrillo-Beltran, M., Curiel-Alvarez, M. A., Beltran-Partida, E. et al. (2022). Insecticidal effect of green bimetallic nanoparticles from Crotalaria longirostrata on Cotton Mealybug, Phenacoccus solenopsis. Journal of Renwable Materials, 10(10), 2543–2552. https://doi.org/10.32604/jrm.2022.020965 [Google Scholar] [CrossRef]
32. León-Jimenez, E., Valdéz-Salas, B., González-Mendoza, D., Tzintzun-Camacho, O. (2019). Synthesis and insecticide activity of Cu-nanoparticles from Prosopis juliflora (Sw) DC and Pluchea sericea (Nutt.) on Phenacoccus solenopsis Tinsley (Hemiptera: Pseudococcidae). Revista de la Sociedad Entomológica Argentina, 78(2), 12–21. https://doi.org/10.25085/rsea.780202 [Google Scholar] [CrossRef]
33. Arendsen, L. P., Thakar, R., Sultan, A. H. (2019). The use of copper as an antimicrobial agent in health care, including obstetrics and gynecology. Clinical Microbiology Reviews, 32(4), e00125–18. https://doi.org/10.1128/cmr.00125-18 [Google Scholar] [PubMed] [CrossRef]
34. Giachino, A., Waldron, K. J. (2020). Copper tolerance in bacteria requires the activation of multiple accessory pathways. Molecular Microbiology, 114(3), 377–390. https://doi.org/10.1111/mmi.14522 [Google Scholar] [PubMed] [CrossRef]
35. Murthy, H. C. A., Desalegn, T., Kassa, M., Abebe, B., Assefa, T. (2020). Synthesis of green copper nanoparticles using medicinal plant Hagenia abyssinica (Brace) JF. Gmel. leaf extract: Antimicrobial properties. Journal of Nanomaterials, 2020, 3924081. https://doi.org/10.1155/2020/3924081 [Google Scholar] [CrossRef]
36. Riaz, T., Mughal, P., Shahzadi, T., Shahid, S., Abbasi, M. A. (2020). Green synthesis of silver nickel bimetallic nanoparticles using plant extract of Salvadora persica and evaluation of their various biological activities. Materials Research Express, 6(12), 1250k3. https://doi.org/10.1088/2053-1591/ab74fc [Google Scholar] [CrossRef]
37. Sharmila, G., Thirumarimurugan, M., Muthukumaran, C. (2018). Green synthesis of ZnO nanoparticles using Tecoma castanifolia leaf extract: Characterization and evaluation of its antioxidant, bactericidal and anticancer activities. Microchemical Journal, 145, 578–587. https://doi.org/10.1016/j.microc.2018.11.022 [Google Scholar] [CrossRef]
38. Gonzalez-Mendoza, D., Valdez-Salas, B., Bernardo-Mazariegos, E., Tzintzun-Camacho, O., Gutiérrez-Miceli, F. et al. (2019). Influence of monometallic and bimetallic phytonanoparticles on physiological status of mesquite. Open Life Sciences, 14(1), 62–68. https://doi.org/10.1515/biol-2019-0008 [Google Scholar] [PubMed] [CrossRef]
39. Mazhar, T., Shrivastava, V., Tomar, S. R. (2017). Green synthesis of bimetallic nanoparticles and its applications: A review. Journal of Pharmaceutical Science and Research, 9(2), 102–110. [Google Scholar]
40. Zielińska, A., Ferreira, N. R., Feliczak-Guzik, A., Nowak, I., Souto, E. B. (2020). Loading, release profile and accelerated stability assessment of monoterpenes-loaded solid lipid nanoparticles (SLN). Pharmaceutical Development and Technology, 25(7), 832–844. https://doi.org/10.1080/10837450.2020.1744008 [Google Scholar] [PubMed] [CrossRef]
41. Danaei, M., Dehghankhold, M., Ataei, S., Davarani, F. H., Javanmard, R. et al. (2020). Impact of particle size and polydispersity index on the clinical applications of lipidic nanocarrier systems. Pharmaceutics, 10(2), 57. https://doi.org/10.3390/pharmaceutics10020057 [Google Scholar] [PubMed] [CrossRef]
42. Reddy, N. V., Li, H., Hou, T., Bethu, M. S., Ren, Z. et al. (2021). Phytosynthesis of silver nanoparticles using Perilla frutescens leaf extract: Characterization and evaluation of antibacterial, antioxidant, and anticancer activities. International Journal of Nanomedicine, 16, 15–29. https://doi.org/10.2147/IJN.S265003 [Google Scholar] [PubMed] [CrossRef]
43. Tee, S. Y., Ye, E. (2021). Recent advancements in coinage metal nanostructures and bio-applications. Materials Advances, 2(5), 1507–1529. https://doi.org/10.1039/D0MA00829J [Google Scholar] [CrossRef]
44. Herrera-Pool, E., Ramos-Díaz, A. L., Lizardi-Jiménez, M. A., Pech-Cohuo, S., Ayora-Talavera, T. et al. (2021). Effect of solvent polarity on the ultrasound assisted extraction and antioxidant activity of phenolic compounds from habanero pepper leaves (Capsicum chinense) and its identification by UPLC-PDA-ESI-MS/MS. Ultrasonics Sonochemistry, 76, 105658. https://doi.org/10.1016/j.ultsonch.2021.105658 [Google Scholar] [PubMed] [CrossRef]
45. Mendez-Trujillo, V., Valdez-Salas, B., Carrillo-Beltran, M., Curiel-Alvarez, M. A., Tzintzun-Camacho, O. et al. (2019). Green synthesis of bimetallic nanoparticles from Prosopis juliflora (Sw) DC., and its effect against cotton mealybug, Phenacoccus solenopsis (Hemiptera: Pseudococcidae). Phyton-International Journal of Experimental Botany, 88(3), 269–275. https://doi.org/10.32604/phyton.2019.07316 [Google Scholar] [CrossRef]
46. Adepoju, A. O., Omotoso, I. O., Femi-Adepoju, A. G., Karim, A. B. (2020). Comparative studies on the antimicrobial, chemical and biochemical contents of the foliar extracts of Capsicum fructescens L. varieties. African Journal of Biotechnology, 19(12), 836–845. https://doi.org/10.5897/ajb2020.17258 [Google Scholar] [CrossRef]
Cite This Article
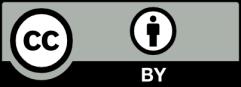