Open Access
ARTICLE
Insight into Hydrolytic Stability and Tribological Properties of B-N Coordination Tung Oil-Based Lubricant Additive in Water
1 Institute of Ecological Conservation and Restoration, CAF, Beijing, 100091, China
2 Institute of Chemical Industry of Forest Products, CAF; National Engineering Laboratory for Biomass Chemical Utilization; Key Laboratory of Chemical Engineering of Forest Products, National Forestry and Grassland Administration; Key Laboratory of Biomass Energy and Material, Jiangsu Province; Jiangsu Co-Innovation Center of Efficient Processing and Utilization of Forest Resources, Nanjing, 210042, China
3 School of Chemical Engineering, Nanjing University of Science & Technology, Nanjing, 210094, China
* Corresponding Author: Haiyang Ding. Email:
(This article belongs to the Special Issue: Renewable Biomass as a Platform for Preparing Green Chemistry)
Journal of Renewable Materials 2023, 11(3), 1367-1381. https://doi.org/10.32604/jrm.2022.023411
Received 25 April 2022; Accepted 21 June 2022; Issue published 31 October 2022
Abstract
A tung oil-based boron-nitrogen coordination polymer (TWE-BN) was specially designed and synthesized as a highly efficient water-based lubricant additive, which has been beneficial to both energy conservation and conducive to environmental protection. Its hydrolysis stability and tribological properties in water were investigated. To better research the lubricating properties, and thus to understand the interaction between the surface and the lubricating additives. Herein, both experimental and theoretical computations based on density functional theory (DFT) were performed. The addition of TWE-BN reduces the water friction coefficient and wear scar diameter, and the maximum non-seizure load increased from 93 to 726 N. Moreover, the anti-corrosion ability on copper was classified as 1b level. The stainless-steel surface was analyzed using scanning electron microscopy (SEM) and X-ray photoelectron spectroscopy (XPS). In hydrolytic stability testing, TWE-BN was better than nitrogen-free tung oil-based lubricant additive (TWE-B) and remained non-hydrolyzed for at least 15 days, implying the feasibility of tung oil-based boron-nitrogen coordination as highly effective and hydrolytic stability lubricant additives.Graphical Abstract
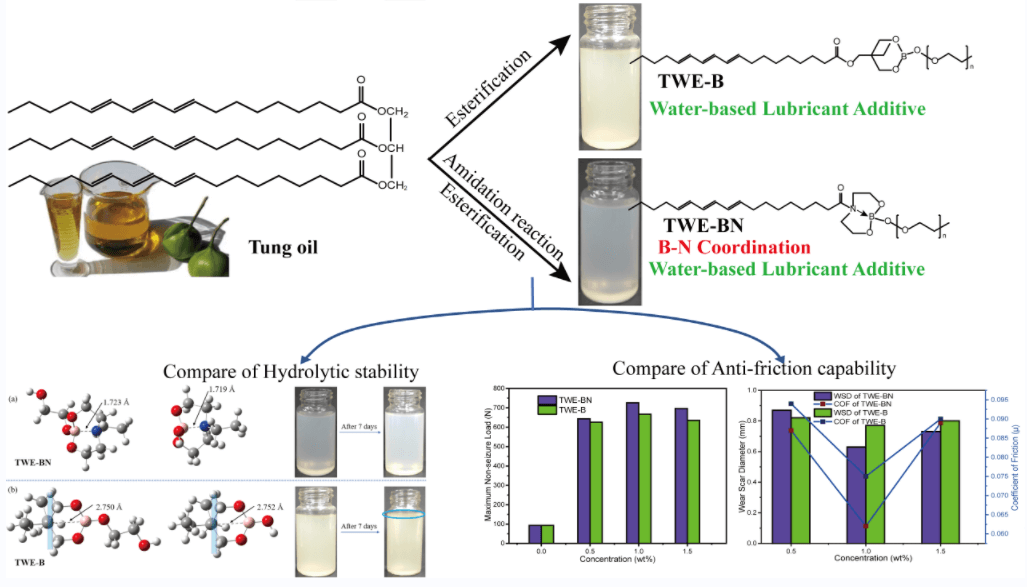
Keywords
In recent years, with the rapid increase in the global high-end equipment manufacturing capacity and the whole society attaching high attention to environmental protection issues, people have higher requirements for the high efficiency, degradability, harmless, and other operational performance for lubricating oil [1–3]. The traditional petroleum-based mineral oil has weakened cost advantages and limited biodegradation and service performance, causing it to face the pressure of resources and environmental protection [4–9]. Therefore, there is an urgent need for an alternative environmentally friendly and low-polluting lubricant. Water-based lubricants are a valid candidate for liquid lubricants because of their low cost, high fire resistance, good storage stability, and excellent biocompatibility [10–12].
At present, many types of research have shown that water-based lubricants were capable of forming lubricating films on metal surfaces and improving the friction properties of water-based lubricants. Fernando et al. synthesized a series of alkyl glyceryl ethers and it was demonstrated that these ethers have a good performance as an additive for water-based lubricants when formulated with Xanthan gum, and the coefficient friction decreased from 0.34 to 0.06 [13]. Hu et al. [14] prepared graphene-based material to be used as a nano additive a water-based lubricant. The results indicated that the nano additive could stably disperse in water for a long time and exhibit excellent friction-reducing and anti-wear properties. Zhang et al. [15] modified polytetrafluoroethylene micro powder with dopamine as a water-based lubricating additive, research shows that the additive exhibits good antiwear and antifriction properties. However, research on renewable bio-based additives as water-based lubricant additives is very scarce. Besides, the use of water-based lubricants that exist disadvantages of poor lubrication performance and corrosion equipment [16–18]. One of the effective ways to improve the performance of water-based lubricants is to add additives containing extreme pressure elements and anti-corrosion properties [19–22].
Tung oil is a non-edible oil, mainly grown in China, which primarily contain unsaturated fatty acids with the following average molar distribution, 77.2% α-eleostearic acid, 7.6% linoleic acid, 5.9% oleic acid, 4.2% palmitic acid, 2.3% stearic acid, and 0.4% linolenic acid. As an excellent drying oil, tung oil has long fatty acid chains and polar groups, and this amphiphilicity enables them to have a good film/force relationship, which can act as a boundary lubricant [23–26]. However, due to the high degree of unsaturation and low oxidation stability of tung oil, it is not suitable for direct use as lubricating oil [27]. Based on the improved performance and environmental consciousness of water-based lubricants, this work envisages the introduction of extreme pressure element boron into tung oil to prepare water-based extreme pressure lubricant additives. Despite the fact that boronic ester has been successfully used in the preparation of various lubrication additives, designing boronic ester with enhanced hydrolytic stability and superior extreme pressure performance remains extremely challenging due to the fact that the electron-deficient boron sites linked by boronate esters are vulnerable to attack by nucleophiles such as water molecules [28,29]. As a result, exposure to water, even vapor in the air, could potentially hydrolyze the boronate ester and compromise the integrity of lubricant additives [30,31]. Given the problem of easy hydrolysis of borate esters, we postulated a novel strategy for synthesizing stable nitrogen-coordinating cyclic boronic ester compounds by introducing an N→B coordination bond and then using them to construct a lubrication additive. Different from most studies on borate extreme pressure lubricant additives, the introduction of tung oil makes up for the shortcomings of water-based lubricants that are easy to corrode. In addition, explore through a combination of experimental and theoretical calculations design and characterization of the tung oil-based lubricant additive, and discuss the inherent law of the structure-function relationship between microscopic molecular structure and macroscopic properties. To provide a reference for pain point relief of water-based lubrication.
All chemicals we used were of analytical grade without further purification. The tung oil was purchased from the Luodian County Anling Vegetable Oil Plant (China). Methoxypolyethylene glycol (Relative molecular weight of 1000, Shanghai Aladdin Biochemical Co., Ltd., China), Diethanolamine (AR, ≥99%), Carbon tetrachloride (AR, ≥99%), Boric acid (AR, ≥99.5%), Trimethylolpropane (AR, 98%), ethylene glycol (AR, ≥99%) all purchased from the Sinopharm Chemical Reagent Co., Ltd. (China).
2.2 Preparation of Tung Oil-Based Waterborne Extreme Pressure Lubrication Additives
Tung oil-based waterborne extreme pressure lubrication additive (TWE-BN) was fabricated as illustrated in Scheme 1. In order to compare the effect of the nitrogen coordination on the properties of the lubrication additives, especially hydrolysis stability, the nitrogen-free lubrication additive (TWE-B) was also synthesized. Synthesis details are described below.
Scheme 1: Synthesis routes of TWE-BN and TWE-B
Preparation of Tung Oil Acid Diethanolamine (TODN)
Tung oil acid diethanolamine (TODN) was synthesized by the amidation reaction between tung oil and diethanolamine, following specific methods are adopted. Firstly, transferred tung oil, diethanolamine, and alkaline catalyst into four flasks with a mixer, thermometer, and condensate tube under nitrogen protection this mixed solution reacts 4 h, 90oC–120oC to prepare TODN. After this period, the resulting reaction product is a mixture containing glycerol, diethanolamine, tung oil, and TODN. After the product is purified and distilled, a pure TODN product can be obtained.
Preparation of Tung Oil-Based Nitrogen-Containing Borate (TO-BN)
Tung oil-based nitrogen-containing borate (TO-BN) was synthesized by the esterification method [32,33]. Details are as follows, methylbenzene as a water-carrying agent, TODN, boric acid, and a few catalysts placed on three flasks with 110oC condensation reflux reaction 5 h. After the product is purified and distilled, a pure TO-BN product can be obtained.
Preparation of Tung Oil-Based B-N Coordination Waterborne Extreme Pressure Lubrication Additives (TWE-BN)
TWE-BN was synthesized by the esterification method. Details are as follows, methoxypolyethylene glycol of 1000 relative molecular weight, TO-BN, and a few catalysts placed on three flasks with 110oC condensation reflux reaction 5 h. After the product is purified and distilled, a pure TWE-BN product can be obtained.
Preparation of Trihydroxymethyl Tung Oil Ester (TO-tOH)
Trihydroxymethyl tung oil ester (TO-tOH) was synthesized by the transesterification between tung oil and trimethylolpropane, following specific methods are adopted. Firstly, transferred tung oil, trimethylolpropane, and acid catalyst into four flasks with a mixer, thermometer, and condensate tube under nitrogen protection this mixed solution reacts 4 h, 90oC–120oC to prepare TO-tOH. After the product is purified and distilled, a pure TO-tOH product can be obtained.
Preparation of Tung Oil-Based Borate (TO-B)
Tung oil-based borate (TO-B) was synthesized by the esterification method. Details are as follows, methylbenzene as a water-carrying agent, TO-tOH, boric acid and a few catalysts placing to three flasks with 110oC condensation reflux reaction 5 h. After the product is purified and distilled, a pure TO-B product can be obtained.
Preparation of Tung Oil-Based Waterborne Extreme Pressure Lubrication Additives (TWE-B)
TWE-B was synthesized by the esterification method. Details are as follows, methoxy polyethylene glycol of 1000 relative molecular weight, TO-B, and a few catalysts placed on three flasks with 110oC condensation reflux reaction 5 h. After the product is purified and distilled, a pure TWE-B product can be obtained.
2.3 Characterization Methodologies
Characterizations of the obtained lubrication additives and steel ball obtained after friction include identification of chemical structure (by infrared spectroscopy (FTIR), 1H Nuclear magnetic resonance (1H NMR), X-ray photoelectron spectroscopy (XPS)), observation of microscopic morphology (by scanning electron microscope (SEM)). FTIR was recorded on the attenuated total reflection mode spectrometer. 1H NMR measurement was performed on an AV ANCE 400 Bruker, samples were first dissolved in CDCl3. Mestre Nova software (Santiago de Compostela, Spain) was used to handle the NMR data. Steel ball obtained after friction was analyzed by XPS (PHI 5000 Versa Probe. The tribological properties were analyzed in terms of coefficient of friction (COF), wear scar diameter (WSD) of the ball, and the worn track in the disk. The worn surface morphologies of the worn surface were investigated with SEM (FEI Co., Ltd., Hillsboro, USA).
Hydrolysis stability adopts the wet-heat treatment to accelerate the hydrolysis method (patent USP 4892670): add 150 g of sample (1.0% additive in water) into a 200 ml beaker and put it in a moist heat oven (temperature 50 ± 2oC, relative humidity greater than 95%). Monitor samples every hour and records the time. If precipitation is observed in the sample or the sample is no longer transparent, this means that the additive has been hydrolyzed [29].
2.5 Measurement of Tribology Properties
The maximum non-seize load (PB value), and friction performances of the samples were measured by the four-ball tribometer (MRS-10). Its measurement method is similar to ASTM D2783. The test conditions for PB were 556 mm/s (1450 r/min) for 10 s at room temperature, and the coefficient of friction and wear scar diameter tests conditions were 1450 rpm for 1800 s under an applied load of 200 N [10]. Each measurement was triplicated.
The anticorrosion performance test is carried out in accordance with the GB/T 5096–2017 standard for TWE-BN and TWE-B in water. The bright-treated copper bars were dipped into the prepared samples. After that, they were transferred to a constant temperature of 100 ± 2oC for 3 h. After the test, the copper strip is taken out for cleaning, dried at room temperature, and then compared with the corrosion standard color plate to determine the corrosion level [34].
2.7 Computational Details for DFT Simulations Studies
In order to thoroughly analyze the lubrication mechanism, we explore the lubrication mechanism using theoretical calculation as auxiliary instructions. Density Functional Theory (DFT) calculations were performed using the Gaussian16 software. All calculations were performed using the M06–2X functional. For geometric optimization and frequency calculations, using the def2-TZVP basis set. The optimal geometry for each compound was determined [35].
3.1 Characterization of Tung Oil-Based Waterborne Lubrication Additives
Fig. 1b displays the FTIR spectra of Tung oil, TWE-BN, and TWE-B. A series of similar strong and broad bands at approximately 3470 cm−1 are ascribed to the stretching vibrations of hydroxide groups (-OH) and/or H-bonding for TWE-BN, and TWE-B, while the peak did not appear in the tung oil. The characteristic peak of carbonyl stretching vibration appears at 1740 cm−1 attributable to the glycerides in the structure of tung oil. The peaks at 989 cm−1 were ascribed to the conjugate double bond of tung oil. These position peak also occurs on both TWE-BN and TWE-B. The symmetric stretching vibrations with a new peak of the B-O bond appear at 1348 and 1344 cm−1, and the formation of the B-O bond in borates indicates that the hydroxyl of methoxy polyethylene glycol has been connected by esterification reaction [36]. The peak at 1644 cm−1 represents C-N of TWE-BN, which is not shown on the spectrum of TWE-B. The 1H-NMR spectra of tung oil, TWE-BN, and TWE-B are shown in Fig. 1c and the attributions of the chemical shifts of the protons are labeled. Multiple peaks assigning to the protons of conjugated double bond appeared at 5.3–6.6 ppm, the protons attributing to the methyl appeared at 0.9 ppm, the signals ascribing to protons of methylene appeared at 1.2–1.5, 2.0–2.5 ppm. It also could be seen that the peaks associated with double bonds in the spectrum of TWE-BN and TWE-B, indicated that the double bonds remained after the esterification. The FTIR and 1H-NMR spectra validate the successful preparation of TWE-BN and TWE-B.
Figure 1: FTIR (a) and 1H-NMR (b) spectra of tung oil, TWE-BN, and TWE-B
3.2 Evaluation of Hydrolytic Stability
To investigate the impact of B→N coordination on the electronic structures of the lubricant additives, the density functional theory calculations were carried out using a suite of Gaussian 16 programs [37–39]. Since the hydrolytic process of borate ester has little relationship with the structure of tung oil and methoxy polyethylene glycol. A simplified model was chosen for two lubricant additives (TWE-BN and TWE-B) by comprehensively considering computational cost and accuracy. The TWE-BN and TWE-B molecule full geometry optimization of the transition state (TS) was carried out. Reactant and product complex geometries were found by the IRC descend in the B3LYP method and reactant complexes were subsequently optimized Fig. 2 shows the calculated energy profiles of hydrolytic processes of TWE-BN and TWE-B in the presence of water molecule. The hydrolysis of the boronate ester linkage is a three-step reaction. In the first step, one H2O molecule reacts with the B-O bond outside the ring in TWE-BN, the coordination between boron and nitrogen alleviates the occurrence of this hydrolysis process. In the following step, another H2O molecule reacts with the hydrolysis with one of the B-O bonds on the seven rings. These two steps have energy barriers of 166.45 and 170.31 kJ/mol, respectively. It shows that the resistance of the second step of the hydrolysis process is larger. On the one hand, it needs to destroy the coordination between B and N even the ring structure also needs to be destroyed. Last step, an additional H2O molecule is found to greatly assist the hydrolysis by lowering the reaction barriers to 134.94 kJ/mol. This is attributed to the seven rings being completely destroyed, and the coordination between B and N almost disappears, which makes the hydrolysis reaction easier. For TWE-B, the total energy barrier for the second hydrolysis process is below TWE-BN by approximately 22.46 kJ/mol. This indicates that the presence of N and B can alleviate the hydrolysis of borate lubrication additives, TWE-BN showed higher hydrolysis stability than TWE-B because of the intramolecular coordination of N→B in the TWE-BN linkages, which is probably easier to maintain extreme pressure performance. Lubrication performance will be discussed in detail in the following section. Fig. 3 shows the changes in the ring where the B element is located before and after the two lubrication additives bind to the water to demonstrate the contribution of the N in the hydrolysis process. As shown in Fig. 3a, the distance between B and N is 1.723 Å before water molecules attack borate, which forms a strong coordination effect. After the water molecules attack, the distance between B and N is further shortened and the coordination effect becomes stronger, that is, N exhibits further hydrolysis inhibition of organic borate.
Figure 2: DFT-calculated hydrolytic process of TWE-BN and TWE-B in the presence of water molecule
Figure 3: Most stable conformations of TWE-BN (a) and TWE-B (b) according to DFT calculation results, appearance change of TWE-BN and TWE-B (additive concentration: 1.0 wt%, normal pressure and temperature)
In contrast to TWE-BN, the distance between the B atom and its para-atom is large for TWE-B. Para-atom of B has a similar position to N in the TWE-BN and the interatomic distance is further increased after the water molecules attack. It can also be seen from the comparison figure before and after 7 days that there is a turbid precipitate in TWE-B, but there is almost no change in TWE-BN. This further indicates that the hydrolysis stability of TWE-BN is stronger than that of TWE-B. In addition, we performed hydrolysis stability monitoring for the two additives. The experimental results are listed in Table 1. TWE-BN remained unhydrolyzed for at least 15 days compared to TWE-B, suggesting that the formation of inter-molecular-coordination of nitrogen to boron N→B (TWE-BN) that could decrease the possibility of the attack on water.
The load-carrying properties with varying concentrations of TWE-BN and TWE-B additives are marked with the maximum non-seizure load (PB), and the experimental results are shown in Fig. 4. The PB value of water is 93 N, indicating it is difficult to form an effective lubrication film on a metal surface. Nevertheless, the addition of TWE-BN and TWE-B significantly improved the load-carrying capacity of water, which is related to the formation of protective film on the friction surface. This may be due to long-chain molecules, complex structures, and polar groups, which can be firmly adsorbed on the metal surface to form an aligned molecular layer. As the content of additives increases, the mono-molecular layer changes to the multi-molecular layer. Furthermore, the intermolecular Van der Waals force makes the molecular layers tightly combined to form a physical adsorption film. Under the conditions of high-speed friction and instant high temperature, it is easy to form on the metal surface with low shear strength and good thermal stability, and the chemical adsorption membrane with strong bearing capacity. It is noteworthy that the TWE-BN and TWE-B copolymer concentration exceeds 1.0 wt%, and it is found that in maximum non-seizure loads the PB value does not change much. Further, the higher PB value of TWE-BN than TWE-B indicates that the coordination effect between N and B alleviates the hydrolysis of boric acid ester, making the lubrication film more stable and having a higher PB.
Figure 4: Maximum non-seizure loads (PB values) of the TWE-BN and TWE-B in water (four-ball tester, rotation velocity 1450 rpm, 10 s)
3.3.2 Wear Resistance and Frictional Properties
The coefficient of friction (COF) and wear scar diameter (WSD) of TWE-BN and TWE-B were carefully studied to examine the lubrication effect. Under the same test conditions, we also tested the friction coefficient of water, but only 141 s before the steel ball stopped moving due to a serious seizure. The test was forced to stop and cannot continue, which indicates that the carrying capacity of water is very weak. It is clearly seen from Fig. 5a that COF decreases with the addition of TWE-BN, while the regular pattern of the friction coefficient of TWE-B is not obvious and fluctuates greatly. As TWE-BN copolymer content increases, the COF decreases insignificantly. The lowest COF values (0.062) are obtained at 1.0 wt% TWE-BN, which is below the value TWE-B (0.075, 1.0 wt%). Fig. 5b illustrates the WSD of the balls after friction with TWE-BN and TWE-B (four-ball tester; load: 200 N, speed: 1450 rpm, duration: 1800 s). It can be seen that the introduction of TWE-BN and TWE-B in water leads to a sharp decrease of WSD when the concentration is less than or equal to 1.0 wt%. When the concentration of TWE-BN and TWE-B additive is greater than 1.0 wt%, the WSD varies slightly increased. And the smallest wear scar diameter of 0.63 mm was obtained at a concentration of 1.0 wt% for TWE-BN. This indicates that the addition of TWE-BN and TWE-B at a concentration of 1.0 wt% makes the lubricant produce a stable protective lubricating film on the contacting metal surfaces and improves the water carrying capacity.
Figure 5: Variation of coefficient of friction (COF) and wear scar diameter (WSD) with the additive concentration of TWE-BN and TWE-B (four-ball tester; load: 200 N, speed: 1450 rpm, duration: 1800 s)
3.4 Mechanism Analysis of as-Synthesized Borate Ester
The worn surface of steel ball was studied by SEM. SEM micrographs of the worn steel surface TWE-BN and TWE-B (1.0 wt%) are shown in Fig. 6. The size of the wear scar corroborates the order of tribological performance obtained experimentally. In the steel surface lubricated by TWE-B, the surface was damaged and deformed due to grain wear, indicating that severe wear and abrasion occurred during the test, which was due to the poor strength of the lubricating film formed by TWE-B. However, the wear surface of TWE-BN is smoother with no signs of severe wear along the sliding direction, and the wear scar is relatively narrow and shallow when compared to the surface with TWE-B lubrication. The observations indicate that TWE-BN reduces steel ball wear to some extent, in relative agreement with the corresponding friction wear test data in Fig. 5.
Figure 6: SEM images of the worn surfaces lubricated at concentrations 1.0 wt% for TWE-BN and TWE-B
A comparative analysis of the worn surfaces of the TWE-BN 1.0 wt% and TWE-B 1.0 wt% lubricated steel balls was performed with XPS to obtain more information on the mechanism of action in the lubrication process. As shown in Fig. 7, the XPS spectrum of the wear-out steel surface was lubricated with TWE-BN 1.0 wt%. The B1s peaks of 187.5 and 189.7 eV belong to B-N and Fe-O-B, respectively, suggesting that borates react with nitrogen on the friction surface during friction. This suggests that boron is involved in the frictional chemical reaction during sliding. The N1 s peak appears at 397.8 and 398.6 eV the former corresponding to B-N and the latter corresponding to the organic nitrogen compound of diethanolamine. Furthermore, these compounds were also supported by the binding energy of B1s at around 187.5 eV. This suggests that N also participates in frictional chemical reactions during its physical and/or chemical adsorption on frictional metal surfaces. The C1s peaks at 284.7, 285.8, and 288.1 correspond to the C-H, C-C, and C-O bonds of organic compounds, suggesting that TWE-BN is adsorbed on the steel surface. Combining the binding energies of 710.6 and 709.8 eV in the O1 s and Fe2p spectra can further confirm that iron oxide should be the component of the friction film [40]. The binding energies of the O1 s were 530.1, 531.8, and 532.6 eV, which can be attributed to ferrous oxide, C-O bonds, and Fe-O-B, respectively, further confirming that the TWE-BN additive underwent frictional chemical reactions in this process. Fig. 8, the XPS spectrum of the worn steel surface is lubricated with 1.0 wt% TWE-B. The B1s peak at 189.8 eV belongs to the B-OH or B-O bond, indicating that the TWE-B has been decomposed to form the B-O and B-OH groups during the friction process. The peaks of the B1s spectra are not obvious, indicating that the XPS is less detectable of the boron elements adsorbed on the worn surface, possibly due to the hydrolysis of the borate interfering with the B1s spectra. Spectra of the other elements are similar to the TWE-BN.
Figure 7: XPS spectra of worn steel surface lubricated with TWE-BN 1.0 wt% (four-ball friction and wear tester)
Figure 8: XPS spectra of worn steel surface lubricated with TWE-B 1.0 wt% (four-ball friction and wear tester)
For the above reason, it can be deduced that TWE-BN 1.0 wt% and TWE-B 1.0 wt% easily form lubricating films containing various compounds of carbon, oxygen, iron, and boron elements on the surface of steel balls through adsorption and/or friction reactions. The tribological properties of water can be significantly improved.
Fig. 9 shows the photographs of the copper sheet after the immersion. It is clearly seen that the TWE-BN and TWE-B sample had slightly tarnished the copper sheet surface, and all samples of different concentrations were at the 1b level of anti-corrosion ability. The process of corrosion inhibition involved releasing the tung oil chain segment which protects the opened copper sheet surface from the surrounding environment through protective film formation by oxidative polymerization of tung oil. This could be explained by the presence of a thicker protecting layer on the copper sheet surface during the test, which impedes the corrosion process. Therefore, the addition of a suitable amount of Tong oil-based modified lubricant additives into the water has successfully improved the corrosion behavior of the water-based lubricant.
Figure 9: Photographs of the corrosion test of the lubricant samples on copper sheets. From left: Blank copper sheet, TWE-BN 0.5 wt%∼2.0 wt%, TWE-B 0.5 wt%∼2.0 wt%
Based on developing high-performance and environmentally friendly lubricant additives. We synthesize tung oil-based heterocyclic boron-nitrogen coordination polymer (TWE-BN) as highly effective and hydrolytic stability extreme pressure lubricant additives in the water. When the TWE-BN concentration was 1.0 wt%, the PB value reached 726 N, which was 6.8 times that of water. The hydrolytic stability and lubrication performance of TWE-BN are higher than that of without nitrogen (TWE-B) because of the coordination effect between N-containing heterocyclic rings and borate in TWE-BN acting an important role. Furthermore, DFT studies gave the conformation of coordination B and N on the adsorbing surface, the energy required for TWE-BN to destroy the boronic ester on the ring is larger in the three-step hydrolysis simulation reaction and the distance between B and N is further shortened (from 1.723 to 1.719) after water molecules attack the boronate ester, which indicates the formation of a strong coordination effect. The XPS analysis results show that TWE-BN reacts with the metal surface to form a chemical adsorption film during the friction process, which plays a crucial role in reducing friction and anti-wear. Paving the way to an understanding of the lubrication and hydrolysis mechanism of water-based lubricant.
Funding Statement: This work was supported by Special Fund of Chinese Central Government for Basic Scientific Research Operations in Commonweal Research Institutes [No. CAFYBB2019SY037] and National Natural Science Foundation of China [No. 31901260].
Conflicts of Interest: The authors declare that they have no conflicts of interest to report regarding the present study.
References
1. Liu, H., Liu, H. J., Zhu, C. C., Parker, R. G. (2020). Effects of lubrication on gear performance: A review. Mechanism and Machine Theory, 145, 1–14. DOI 10.1016/j.mechmachtheory.2019.103701. [Google Scholar] [CrossRef]
2. Hamran, N. N. N., Ghani, J. A., Ramli, R., Haron, C. H. C. (2020). A review on recent development of minimum quantity lubrication for sustainable machining. Journal of Cleaner Production, 268, 1–18. DOI 10.1016/j.jclepro.2020.122165. [Google Scholar] [CrossRef]
3. He, Z. B., Min, C. Y., Yang, Y. Z., Zhang, K., Dong, C. K. et al. (2020). Synthesis by partial oxygenation of graphite-like carbon nitride (OCN) decorated with oleic diethanolamide borate (ODAB) for oil-based lubricant additives and its tribological properties. New Journal of Chemistry, 44, 5377–5385. DOI 10.1039/D0NJ00726A. [Google Scholar] [CrossRef]
4. Wu, H. X., Yin, S. C., Du, Y., Wang, L. P., Yang, Y. et al. (2020). Alkyl-functionalized boron nitride nanosheets as lubricant additives. ACS Applied Nano Materials, 3(9), 9108–9116. DOI 10.1021/acsanm.0c01767. [Google Scholar] [CrossRef]
5. Moreno, K. J., Hernandez-Sierra, M. T., Baez, J. E., Rodriguez-deLeon, E., Aguilera-Camacho, L. D. et al. (2021). On the tribological and oxidation study of xanthophylls as natural additives in castor oil for green lubrication. Materials, 14(18), 1–17. DOI 10.3390/ma14185431. [Google Scholar] [CrossRef]
6. Shetty, P., Mu, L. W., Shi, Y. J. (2020). Polyelectrolyte cellulose gel with PEG/water: Toward fully green lubricating grease. Carbohydrate Polymers, 230, 1–8. DOI 10.1016/j.carbpol.2019.115670. [Google Scholar] [CrossRef]
7. Nagendramma, P., Kaul, S. (2012). Development of ecofriendly/biodegradable lubricants: An overview. Renewable and Sustainable Energy Reviews, 16, 764–774. DOI 10.1016/j.rser.2011.09.002. [Google Scholar] [CrossRef]
8. Polmear, R., Stark, J. S., Roberts, D., McMinn, A. (2015). The effects of oil pollution on antarctic benthic diatom communities over 5 years. Marine Pollution Bulletin, 90, 33–40. DOI 10.1016/j.marpolbul.2014.11.035. [Google Scholar] [CrossRef]
9. Ding, H. Y., Yang, X. H., Xu, L. N., Li, M., Li, S. H. et al. (2019). Tribological behavior of plant oil-based extreme pressure lubricant additive in water-ethylene glycol liquid. Journal of Renewable Materials, 7(12), 1392–1401. DOI 10.32604/jrm.2019.07207. [Google Scholar] [CrossRef]
10. Ding, H. Y., Wang, M., Li, M., Huang, K., Li, S. H. et al. (2018). Synthesis of a water-soluble, rubber seed oil-based sulfonate and its tribological properties as a water-based lubricant additive. Applied Polymer Science, 135(15), 1–7. DOI 10.1002/app.46119. [Google Scholar] [CrossRef]
11. Morshed, A., Wu, H., Jiang, Z. Y. (2021). A comprehensive review of water-based nanolubricants. Lubricants, 9(9), 2–62. DOI 10.3390/lubricants9090089. [Google Scholar] [CrossRef]
12. Mu, L. W., Shi, Y. J., Hua, J., Zhuang, W., Zhu, J. H. (2017). Engineering hydrogen bonding interaction and charge separation in Bio-polymers for green lubrication. Journal of Physical Chemistry B, 121(22), 5669–5678. DOI 10.1021/acs.jpcb.7b03194. [Google Scholar] [CrossRef]
13. de Paula, F. H. M., de Freitas, F. A., Nunes, D. G., Iglauer, S., Gramatges, A. P. (2022). Alkyl glyceryl ethers as water-based lubricant additives in mixtures with xanthan gum. Colloids and Surfaces A–Physicochemical and Engineering Aspects, 634, 1–8. DOI 10.1016/j.colsurfa.2021.127881. [Google Scholar] [CrossRef]
14. Hu, Y. W., Wang, Y. X., Zeng, Z. X., Zhao, H. C., Li, J. L. (2019). BLG-RGO: A novel nanoadditive for water-based lubricant. Tribology International, 135, 277–286. DOI 10.1016/j.triboint.2019.03.008. [Google Scholar] [CrossRef]
15. Zhang, H., Hu, H. Y., Ye, W., Zhou, F. (2011). Conferring polytetrafluoroethylene micropowders with hydrophilicity using dopamine chemistry and the application as water-based lubricant additive. Journal of Applied Polymer Science, 122(5), 3145–3151. DOI 10.1002/app.34330. [Google Scholar] [CrossRef]
16. Wang, Y. R., Yu, Q. L., Ma, Z. F., Huang, G. W., Cai, M. R. et al. (2017). Significant enhancement of anti-friction capability of cationic surfactant by phosphonate functionality as additive in water. Tribology International, 112, 86–93. DOI 10.1016/j.triboint.2017.03.034. [Google Scholar] [CrossRef]
17. Ji, H. R., Zhang, X., Tan, T. W. (2017). Preparation of a water-based lubricant from lignocellulosic biomass and its tribological properties. Industrial & Engineering Chemistry Research, 56(27), 7858–7864. DOI 10.1021/acs.iecr.7b01665. [Google Scholar] [CrossRef]
18. Zheng, G. L., Zhang, G. Q., Ding, T. M., Xiang, X. Z., Li, F. et al. (2017). Tribological properties and surface interaction of novel water-soluble ionic liquid in water-glycol. Tribology International, 116, 440–448. DOI 10.1016/j.triboint.2017.08.001. [Google Scholar] [CrossRef]
19. Wang, Y. R., Yu, Q. L., Cai, M. R., Shi, L., Zhou, F. et al. (2017). Ibuprofen-based ionic liquids as additives for enhancing the lubricity and antiwear of water-ethylene glycol liquid. Tribology Letters, 65, 1–13. DOI 10.1007/s11249-017-0840-x. [Google Scholar] [CrossRef]
20. Shariatzadeh, M., Grecov, D. (2019). Aqueous suspensions of cellulose nanocrystals as water-based lubricants. Cellulose, 26(7), 4665–4677. DOI 10.1007/s10570-019-02398-w. [Google Scholar] [CrossRef]
21. Rahman, M. H., Warneke, H., Webbert, H., Rodriguez, J., Austin, E. et al. (2021). Water-based lubricants: Development, properties, and performances. Lubricants, 9(8), 1–25. DOI 10.3390/lubricants9080073. [Google Scholar] [CrossRef]
22. Lv, T., Xu, X. F., Yu, A. B., Hu, X. D. (2021). Oil mist concentration and machining characteristics of SiO2 water-based nano-lubricants in electrostatic minimum quantity lubrication-EMQL milling. Journal of Materials Processing Technology, 290, 1–15. DOI 10.1016/j.jmatprotec.2020.116964. [Google Scholar] [CrossRef]
23. Zhao, H. R., Feng, J. X., Zhu, J., Yu, H. B., Liu, Y. J. et al. (2020). Synthesis and application of highly efficient multifunctional vegetable oil additives derived from biophenols. Journal of Cleaner Production, 242, 1–10. DOI 10.1016/j.jclepro.2019.118274. [Google Scholar] [CrossRef]
24. Souza, M. C. D., Gonçalves, J. F. D. S., Lutif, S. Y. S., Gomes, J. D. O. (2019). Tribological evaluation of the jatropha and tung-based oils as bio-lubricants on Al-7050-T7451 alloy. Journal of the Brazilian Society of Mechanical Sciences and Engineering, 41 (6), 1–11. DOI 10.1007/s40430-019-1746-3 [Google Scholar] [CrossRef]
25. Li, H. Y., Cui, Y. X., Wang, H. Y., Zhu, Y. J., Wang, B. H. (2017). Preparation and application of polysulfone microcapsules containing tung oil in self-healing and self-lubricating epoxy coating. Colloids and Surfaces A–Physicochemical and Engineering Aspects, 518, 181–187. DOI 10.1016/j.colsurfa.2017.01.046. [Google Scholar] [CrossRef]
26. Woma, T. Y., Lawal, S. A., Abdulrahman, A. S., Olutoye, M. A., Ojapah, M. M. (2019). Vegetable oil-based lubricants: Challenges and prospects. Tribology Online, 14(2), 60–70. DOI 10.2474/trol.14.60. [Google Scholar] [CrossRef]
27. Ju, Y. H., Sari, N. N. F., Go, A. W., Wang, M. J., Agapay, R. C. et al. (2020). Preparation of epoxidized fatty acid ethyl ester from tung Oil as a Bio-lubricant base-stock. Waste and Biomass Valorization, 11(8), 4145–4155. DOI 10.1007/s12649-019-00749-z. [Google Scholar] [CrossRef]
28. Yang, G. B., Zhao, J. H., Cui, L., Song, S. Y., Zhang, S. M. et al. (2017). Tribological characteristic and mechanism analysis of borate ester as a lubricant additive in different base oils. RSC Advances, 7(13), 7944–7953. DOI 10.1039/C6RA26896J. [Google Scholar] [CrossRef]
29. Li, J. C., Fan, B. J., Ren, T. H., Zhao, Y. D. (2015). Tribological study and mechanism of B-N and B-S-N triazine borate esters as lubricant additives in mineral oil. Tribology International, 88, 1–7. DOI 10.1016/j.triboint.2015.03.002. [Google Scholar] [CrossRef]
30. Lanni, L. M., Tilford, R. W., Bharathy, M., Lavigne, J. J. (2011). Enhanced hydrolytic stability of self-assembling alkylated two-dimensional covalent organic frameworks. Journal of the American Chemical Society, 133(35), 13975–13983. DOI 10.1021/ja203807h. [Google Scholar] [CrossRef]
31. Kavita, Kuntail, J., Verma, D. K., Kumar, B., Rastogi, R. B. (2020). Theoretical and experimental studies of pyranopyrazoles and their tribological compatibility with a borate ester. Colloids and Surfaces A–Physicochemical and Engineering Aspects, 606, 1–12. DOI 10.1016/j.colsurfa.2020.125497. [Google Scholar] [CrossRef]
32. Palanisamy, A., Rao, B. S., Mehazabeen, S. (2011). Diethanolamides of castor oil as polyols for the development of water-blown polyurethane foam. Journal of Polymers and the Environment, 19(3), 698–705. DOI 10.1007/s10924-011-0316-2. [Google Scholar] [CrossRef]
33. Yang, W. Q., Chen, B. S., Fang, J. H., Wang, J. (2016). Synthesis of boric acid ester containing nitrogen from methyl oleate and its tribological performance. Petroleum Processing Section, 32(1), 82–87. [Google Scholar]
34. Chen, B., Fang, J., Wu, J., Huang, W., Wang, J. et al. (2013). The influence of amino acids on biodegradability, oxidation stability, and corrosiveness of lubricating oil. Petroleum Science and Technology, 31(2), 185–191. DOI 10.1080/10916466.2010.525578. [Google Scholar] [CrossRef]
35. Jaiswal, V., Kalyani, Rastogi, R. B., Kumar, R. (2014). Tribological studies of some SAPS-free schiff bases derived from 4-aminoantipyrine and aromatic aldehydes and their synergistic interaction with borate ester. Journal of Materials Chemistry A, 2(27), 10424–10434. DOI 10.1039/C4TA01191K. [Google Scholar] [CrossRef]
36. Yang, S. L., Huang, L. C., Luo, Q. Q., Zhang, C. X., Zhang, G. J. (2017). Novel N-containing heterocyclic borate ester with hydrolytic stability as lubricant additive. Petroleum Chemistry, 57(8), 722–727. DOI 10.1134/S0965544117080163. [Google Scholar] [CrossRef]
37. Li, H. F., Li, H. Y., Dai, Q. Q., Li, H., Bredas, J. L. (2018). Hydrolytic stability of boronate ester-linked covalent organic frameworks. Advanced Theory and Simulations, 1(2), 1–31. DOI 10.1002/adts.201700015. [Google Scholar] [CrossRef]
38. Blanck, S., Loehle, S., Steinmann, S. N., Michel, C. (2020). Adhesion of lubricant on aluminum through adsorption of additive head-groups on gamma-alumina: A DFT study. Tribology International, 145, 1–10. [Google Scholar]
39. Monasse, B., Montmitonnet, P. (2008). DFT-Modeling of the reaction of a polysulfur extreme-pressure lubricant additive on iron surface. International Journal of Material Forming, 1, 1251–1254. DOI 10.1007/s12289-008-0169-y. [Google Scholar] [CrossRef]
40. Jin, F. J., Yang, G. B., Peng, Y., Zhang, S. M., Yu, L. G. et al. (2016). Preparation of borate ester and evaluation of its tribological properties as an additive in different base oils. Lubrication Science, 28(8), 505–519. DOI 10.1002/ls.1343. [Google Scholar] [CrossRef]
Cite This Article
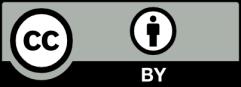