Open Access
ARTICLE
A Rapid Parameter of Enzyme-Treated Cellulosic Material Revealed by Reducing Sugar Release
Departamento de Biotecnologia, Escola de Engenharia de Lorena, Universidade de São Paulo, Lorena, SP, 12602-810, Brazil
* Corresponding Author: Adriane Maria Ferreira Milagres. Email:
(This article belongs to the Special Issue: Renewable Materials and Advanced Technologies for Sustainability)
Journal of Renewable Materials 2024, 12(3), 539-551. https://doi.org/10.32604/jrm.2023.045726
Received 06 September 2023; Accepted 12 December 2023; Issue published 11 April 2024
Abstract
This study was conducted to evaluate the effectiveness of enzymes in purifying and reducing the degree of polymerization of cellulose for the production of dissolving pulp. Our goal was to determine the contributions of xylanase (X) and endoglucanase (EG) in the treatment of pulp, specifically by quantifying the formation of soluble and insoluble reducing sugars using the dinitrosalycilic acid (DNS) test. Predominantly, the release of soluble reducing sugars (RSSol) was enhanced after xylanase treatment, while endoglucanase (EG) treatment led to changes in insoluble reducing sugars (RSIns). The maximum synergism was observed for RSIns when a high ratio of endoglucanase to xylanase (320EG:5X/g pulp) was used. The relative contribution of endoglucanase to RSins was determined to be 15.6% of the total reducing sugar. The viscosity of pulps treated with xylanase decreased only by 7%, whereas endoglucanase treatment significantly reduced viscosity by 45%. Modifications in the particle size were observed after pulp treatment with the combination of endoglucanase and xylanase. In summary, the DNS test is a rapid and effective method for evaluating the efficiency of enzyme treatments on pulps. The measurement of RSIns correlates with changes in pulp viscosity to different extents, providing valuable insights into the effectiveness of enzyme treatments.Graphic Abstract
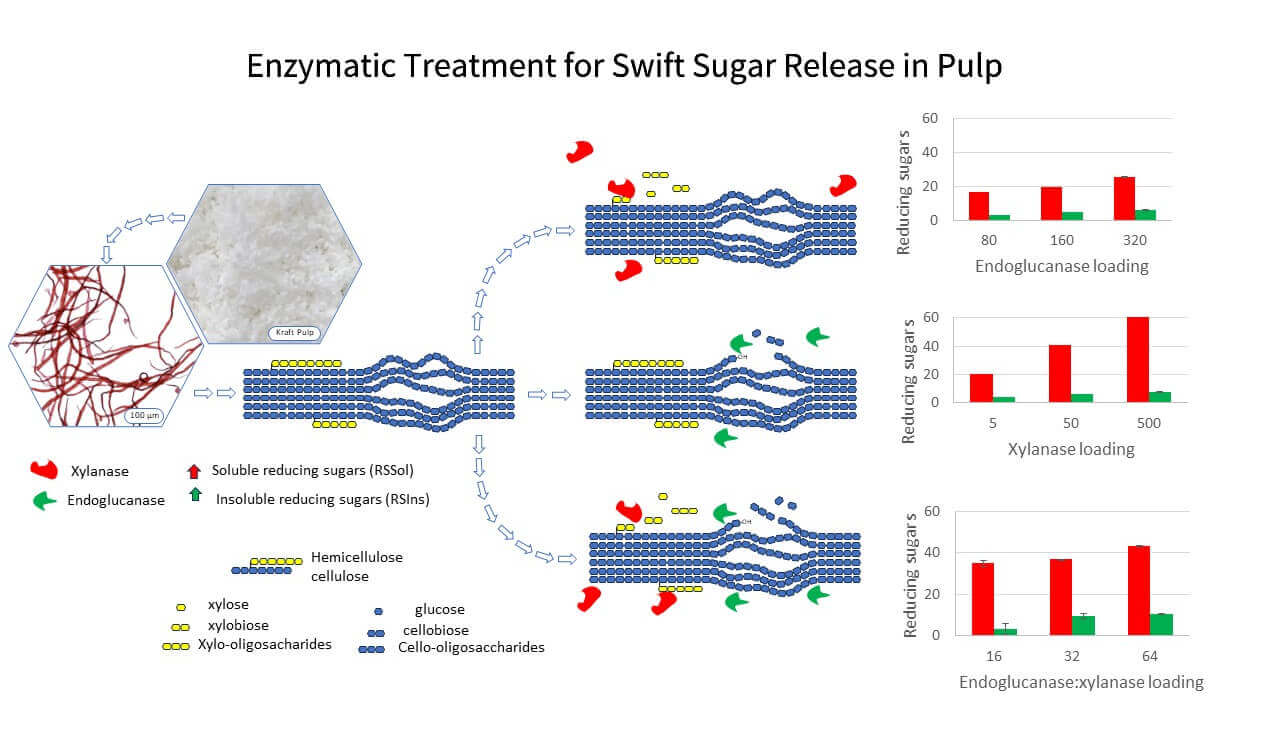
Keywords
Enzyme biotechnology is a viable and promising alternative to replace or improve traditional chemical methods for lignocellulose transformation, contributing to generating new alternative sustainable products. Enzymes are an alternative for industries that work with biomass since they allow the implementation of more substrate-specific, mild reactions, and lower CO2 emissions [1].
In the production of dissolving pulp, enzymes can provide an efficient approach for the development of cellulose derivatives in a more environmentally friendly process [2–4]. Dissolving pulps consist of high-purity cellulose, with low amounts or complete absence of hemicelluloses and traces of lignin and other impurities [5]. These pulps can be used to manufacture various cellulose-derived products, such as regenerated fibers and films [6–8].
Kraft pulp from eucalyptus species is one of the most important fiber sources for the generation of dissolving pulp, however, the kraft process has been reported to yield cellulose with low reactivity because this treatment collapses the fibers, leading to fibrillar aggregations [9]. Increasing the reactivity and accessibility of pulps is not easy as cellulose exhibits an extremely compact structure.
However, several concepts have been adopted for improving the accessibility and reactivity of cellulose fibers, such as opening or expanding the pores, shortening the cellulose chains, and disrupting the highly ordered and tightly packed crystalline regions [10].
Xylanase treatment has been proposed to remove residual hemicelluloses in the structure of the fiber and enhance the accessibility of cellulose [2,11], while endoglucanases improve pulp reactivity by hydrolyzing cellulose and increasing the specific area of fibers [12,13]. The enzymatic hydrolysis of cellulose by endoglucanase starts with the production of chain ends, reducing the degree of polymerization and increasing the specific surface area of fibers [14–16]. Studies have shown a correlation between increased reactivity and decreased intrinsic viscosity of dissolving pulp during enzymatic treatment, whose efficiency depends on several factors, such as temperature, pH, enzyme dosage, reaction time, and pulp consistency [10]. One can cite works with enzymes such as endoglucanase [8,12,17] and even combining xylanase and cellulase treatment [11,14], improving the properties of pulp reactivity and accessibility by the adjustment of pulp viscosity.
Several methods to evaluate the accessibility and reactivity of cellulose fibers have been reported which include dissolution techniques such as the Fock test although this method can be laborious and time-consuming [18]. Alternatively, rheological, microscopy analysis and fiber analyzers are also employed to infer pulp susceptibility to chemical and enzymatic degradation [13,19]. However, such methods require advanced instrumentation and specialized expertise that restrict the accessibility and widespread. Besides, most reactivity measurements cannot differentiate between specific functional groups, hindering their application for understanding the detailed reactivity of cellulose [20,21]. This becomes an even greater challenge when the objective is to define adequate enzymes to purify and activate cellulose for its conversion into dissolving-grade pulp. Considering the benefits of using enzymes, such as their specificity and their environmentally friendly nature, the development of a rapid parameter of enzyme treatment would provide comparable measurements of efficient enzymatic hydrolysis.
Quantification of enzyme treatment of pulp typically involves analyzing the water-soluble fragments that are released. Methods like HPLC are commonly used for this purpose. However, when studying changes in the reactivity of pulp during enzyme treatment, it is also important to consider the alterations in the insoluble part of the pulp [22–24]. The research conducted by Silveira et al. [25], focused on the assessment of the enzymatic hydrolysis profile of cellulosic substrates based on reducing sugar release. The methodology was employed to determine the action of cellobiohydrolases in the production of soluble reducing sugars and endoglucanases for insoluble reducing sugars from the Whatman #1 filter paper. Different classes of reducing sugars were evaluated by the DNS assay and the authors concluded that this method can be performed for other substrates. More specifically, the analysis is based on the detection of reducing sugar ends in the substrate caused by enzyme application.
A progression of this work was to evaluate the application of enzymes in the cellulosic pulp based on solubilized and residual reducing sugars produced. The ability of xylanase to increase the soluble reducing sugars and of endoglucanase to increase the insoluble reducing sugars in bleached kraft pulp is proposed to determine the enzymatic treatments in terms of enzyme dosage and possible synergistic effects. The proof of concept of this approach is successfully demonstrated by changes in viscosity, fiber length, and residual xylan for pulp samples. The results suggest that insoluble reducing sugars offer advantages over typical methods regarding the relative contribution of enzymes to the transformation of the pulp into the final product.
Bleached kraft pulp (BKP) from eucalyptus was provided by Suzano S.A (Jacareí, Brazil) and contained 76% glucan, 14.1% xylan, and 1.2% lignin in % dry matter. Enzymes utilized were Fibercare (Novozymes A/S, Denmark), a commercial endoglucanase, and Luminase PB-200 (BASF, San Diego, Ca) a recombinant xylanase. Luminase formulation exhibited xylanase activity at 23.600 U.ml −1 at 50°C, pH 6, while the endoglucanase activity found for Fibercare formulation was 548 U.ml−1. The protein concentrations of Luminase and Fibercare were 43.7 and 10.9 mg/ml, respectively.
2.1 Determination of Enzymatic Activities and Protein Concentration
Endoglucanase activity was determined by the method of Ghose [26]. Xylanase activity was measured by reducing sugar production from beechwood xylan [27]. The protein concentration of the samples was determined according to Bradford [28].
Trial experiments were carried out on a micro-scale with 50 mg pulp (dry matter) in 5 ml micro centrifugation tubes under agitation (20 rpm) in a hybridization incubator (Hybrigene, Techne) at 50°C for 3 h. The pulp was treated at 2.5% (w/v) solids, with enzyme solution prepared in deionized water with the pH adjusted to 6.0, with HCl 1M. Samples were produced with Fibercare (endoglucanase) or Luminase (xylanase) and with binary combinations at different enzyme concentrations. Enzyme activity loadings varied between experiments as indicated in Fig. 1 in the results and discussion section. The amount of endoglucanase added was 0.15−0.6 mg of protein/g pulp and xylanase 0.01−1.0 mg protein/g of pulp. Each treatment was performed in six replicates and a control without enzymes was run at the same time. After 3 h incubation, 3 mL of the DNS reagent was added to the pulp suspension test tubes [29]. Total reducing sugars (RSTot), soluble reducing sugars (RSSol) and insoluble reducing sugars (RSIns) were reported to evaluate the action of enzymes on pulp as follows:
Figure 1: Reducing sugars (-o- RSTot; -- RSSol, ….•… RSIns) released as glucose equivalent from bleached kraft pulp by individual and combined Fibercare (endoglucanase) and Luminase (xylanase) at different enzyme concentrations. (A) isolated Fibercare, (B) isolated Luminase, (C) binary combination of enzymes with a fixed concentration of 5 U/g Luminase and varied concentration of Fibercare, (D) binary combination of enzymes with a fixed concentration of 80 EGU/g Fibercare and varied concentration of Luminase
For RSTot, test tubes were transferred to a boiling water bath for 5 min for color development and 20 ml distilled water was added and mixed by inversion. The supernatant was collected after centrifugations and absorbance was read at 540 nm in a Shimadzu spectrophotometer. The total reducing sugars (RSTot) of kraft pulp was expressed as glucose equivalent (mg.L−1).
To determine RSSol, test tubes were centrifuged to remove all solids substrates, and a representative 1mL aliquot of each digested mixture was withdrawn, boiled for 5 min to color development, and further diluted with 4 ml distilled water before reading the absorbance at 540 nm in a Shimadzu spectrophotometer. The soluble reducing sugars (RSSol) of kraft pulp were expressed as glucose equivalent (mg.L−1).
Insoluble sugar (RSIns) was indirectly calculated by the difference between the amount of RSTot and RSSol determined after the enzymatic treatments.
The degree of synergism was calculated by dividing the reducing sugar production of xylanase/endoglucanase mixtures by the sum of the productions in parallel digestion containing equivalent amounts of the xylanase and endoglucanase separately [30].
Enzymatic treatments established from trial experiments with 50 mg pulp to assess reducing sugar production were checked with 2 g pulp in 125 mL Erlenmeyer flasks, prepared with 2.5% consistency in water with pH adjusted to 6.0. Pulp was subjected to enzymatic treatment using Fibercare (endoglucanase in the range of 80 to 320 U/g of pulp) and Luminase (xylanase from 5 to 500 U/g of pulp). The effect of a binary mix of xylanases and endoglucanase during the hydrolysis of pulp was studied. The treatment was performing 3 h at 50°C in an orbital shaker. At the end of each treatment, the enzyme was inactivated by water bath (100°C) immersion of flasks for 5 min. A sample was taken from the supernatant for the determination of sugars obtained from enzymatic pulp hydrolysis.
The composition of glucose and xylose in the supernatant after enzymatic treatment of the pulps was analyzed by HPLC before and after a post-hydrolysis procedure [31]. Hydrolysates were filtered through a 0.45 μm filter into glass vials and applied to an HPLC system, equipped with a BioRad Aminex HPX-87H column for the separation of sugars [32].
2.3 Conventional Pulp Analysis
The moisture content of the pulp was determined as per the Tappi Test Method T210 cm-03.
Intrinsic viscosity was determined as per Tappi test methods T 230 om-08.
The particle size was measured with a laser diffraction analyzer (Mastersizer 3000, Malvern Instruments) with sample sonication as described in Dias et al. [33]. Size analyses are the average of two supplicates of each treatment. The percentage of the peak height after enzymatic treatment was compared with the control pulp, considering that 90% of the size of the particles is lower than the displayed value.
Microscopy observation of the pulps was performed to visualize changes in their morphology. The wet pulps and Congo red solution (1% w/v) were mixed in a 1:1 (v:v) ratio and sonicated for 1 min [34]. Dyed samples were visualized by contrast phase microscopy Olympus BX53 equipped with cell sense dimension.
3.1 Determination of Enzymatic Treatments on Pulp Based on the Reducing Sugars
The activities of Fibercare and Luminase on bleached kraft pulp were compared, either separately or combined. The only activity found in Luminase was xylanase, while the main activity in the Fibercare preparation was endoglucanase. Both extracts are broadly used in enzymatic hydrolysis experiments that focus on xylan extraction [35] and dissolving pulp production [36,37].
To explore the susceptibility of kraft pulp to the hydrolytic action of endoglucanase and xylanase, the pulp was treated with different enzyme doses and subjected to analysis of total (RSTot), soluble (RSSol), and insoluble (RSIns) reducing sugars (Fig. 1). The addition of endoglucanase to the pulp resulted in the release of sugars during hydrolysis (Fig. 1A), but the levels of soluble reducing sugars were relatively low. Treatment with 160 EG/g pulp led to a slight increase in the concentration of RSIns. At this enzyme loading, insoluble reducing ends accounted for 15.6% of the total reducing sugars released from the pulp. This increase in insoluble reducing ends is attributed to the disruption of loose cellulose chains in the pulp structure by endoglucanase activity, leading to the formation of new reducing ends [23,38]. This result agreed with degradation trends previously reported by Ceccherini et al. [13,39]. They have studied the effect of endoglucanase on the solubilization of sugars and concluded that endoglucanase can reduce the degree of cellulose polymerization without generating significant losses in pulp yield. Increasing the dose of endoglucanase further did not have an impact on RSIns, as its effectiveness is limited to the amorphous sites on cellulose. However, small RSSol can be detected in hydrolysates with high endoglucanase dosage (320 EG/g). The maximum RSSol (25.45 mg.L−1) was achieved at this dosage, indicating that the Fibercare preparation is capable of degrading sites beyond amorphous cellulose. This is due to the presence of additional enzymes in the Fibercare preparation, such as cellobiohydrolase, xylanase, and β-glucosidase [38]. It is also possible that certain endoglucanases have activity on hemicelluloses, which could contribute to an increase in RSSol [38,40].
Significant increases in reducing sugars were observed when increasing xylanase loading was used to treat pulp (Fig. 1B). At high xylanase loadings (500 U/g pulp) was solubilized approximately 60 mg.L−1 reducing sugars, which accounted for about 3% of the substrate. This mainly resulted in solubilized pulp sugars, while insoluble sugars remained constant even at high doses of xylanase. This result was to be expected since this xylanase acts on xylan rather than cellulose. These observations are well in line with earlier reports by Hu et al. [41].
The combined action of endoglucanase and xylanase had different effects on reducing sugar production [20,36,42]. To investigate the influence of minimal xylanase addition on increasing dosages of endoglucanase, the pulp was treated with xylanase (5 U/g pulp) in combination with endoglucanases (80 to 320 EG/g pulp) (Fig. 1C). Both RSSol and RSIns increased from using the mixture of xylanase and endoglucanase. Significant differences were detected in EG:X-treated pulp relative to the starting pulp. This finding supports the idea that xylanase activity removes some xylan from the outer part of the fiber, enhancing endoglucanase access to fibers. Additionally, high endoglucanase activity generates new reducing chain ends from the pulps, resulting in an increase in RSIns from 3.4 to 10.5 mg.L−1 at the highest endoglucanase loading.
In Fig. 1D, it is evident that the addition of xylanase at varying concentrations (ranging from 0.5 to 50 U/g pulp), along with a fixed concentration of endoglucanase (80 EG/g pulp), leads to a significant increase in RSSol. When both enzymes work together, there is a 1.5-fold increase in RSSol compared to the action of xylanase alone. Moreover, the enzyme mixture reported in Fig. 1D exhibited the best performance in this hydrolysis mode, reaching the highest levels of soluble reducing sugars production and showing saturation behavior at lower enzyme loadings than those shown in Figs. 1A–1C. However, the enzyme mixture D does not have any impact on the production of RSIns in the pulp.
This implies that the presence of low endoglucanase activity is a limiting factor for the production of insoluble reducing ends [36].
A thorough analysis of the production of RSSol and RSIns from bleached kraft pulp by endoglucanase and xylanase may help to explain the reported synergistic effects [41,42]. Fig. 1 shows that when a large dose of xylanase is used, it results in a high concentration of RSSol. Although endoglucanase also contributes to sugar release, its maximal hydrolysis achievement is only half of that achieved by xylanase. Moreover, endoglucanase has a more prominent effect on the production of insoluble sugars. Thus, combining xylanase and endoglucanase allows for the maximum rate of hydrolysis that could be expected after removing impediments caused by xylan, exposing more surface area for endoglucanase to act upon [10,42]. Therefore, it is important to thoroughly analyze the quantitative production of RSSol and RSIns production along with synergism effects.
3.2 Synergistic Actions of Endoglucanase and Xylanase
Investigation of the synergism between xylanase and endoglucanase during pulp hydrolysis requires knowledge of their individual and combined actions. Because for xylanase and endoglucanase a nonlinear relation of hydrolysis rate and enzyme concentration was found, the theoretical sum of the individual actions is nonlinear too. Hence analysis of synergism data was determined as described by Jeoh et al. [30]. This approach considers a degree of synergism greater than 1 if the interaction of the possible binary combinations surpasses the sum of their actions.
Table 1 shows results from the experiments in which xylanase and endoglucanase were added together to assess the possible synergistic interaction for improved hydrolysis of pulp. Different amounts of xylanase and endoglucanase were added to reaction mixtures. An endoglucanase loading of 80 U/g pulp was used to ensure minimal production of RSIns in the pulp within 3 h. The degree of synergism based on RSSol remained nearly constant throughout the entire measured range, with values close to 1.0. Conversely, the values for RSIns exhibited lower degrees of synergism.
Concerning the interaction between xylanase at 5 U/g pulp and different doses of endoglucanase, the synergism was most evident on RSIns. As the amount of endoglucanase was increased, the degree of synergism of RSIns also increased. The combination of endoglucanase at a dosage of 320 U/g pulp and xylanase at 5 U/g pulp demonstrated the highest degree of synergism for RSIns.
Based on the information presented in Table 1 and Fig. 1, it is likely that the variations in the type of reducing sugar production are due to the relative quantities of endoglucanase and xylanase loaded. The degradation of xylan in the pulp and increase in RSSol can be achieved by supplementing the endoglucanase with varying amounts of xylanase. This finding supports the belief that xylanase can improve the purification of cellulosic pulp [20]. However, it is worth noting that the increase in RSSol was not due to synergistic effects of the enzymes, but rather an additive effect. Additionally, the RSIns results demonstrated a strong synergism effect at low xylanase loading and with a high endoglucanase ratio, as evidenced by the highest DSE (1.23). This suggests that there is a relative abundance of specific sites for endoglucanase in the pulp. Therefore, the degree of synergism between xylanase and endoglucanase may depend on the composition and accessibility of the substrate [24,42]. Although synergism was observed for some xylanase-to-cellulase ratios tested, it was also clear that the degree of synergism towards RSIns was not influenced by the xylanase loading. The low content of accessible xylan in the pulp is likely the reason for this. Previous research with a similar enzyme pair also supports this conclusion, stating that equal proportions of endoglucanase and xylanase resulted in negligible pulp hydrolysis due to the recalcitrant nature of the remaining substrate [43].
Overall, it can be inferred that xylanase dose may increase RSSol by solubilizing xylan on the pulp surface, independently of endoglucanase action, but the same is not true for RSIns.
Another interesting comparison can be made regarding the production of glucose, xylose, and soluble oligomers in connection with endoglucanase and xylanase hydrolysis of cellulosic pulp (Fig. 2). When enzymes were added individually to the pulp, xylanase did not hydrolyze cellulose and endoglucanase did not hydrolyze xylan, indicating their selectivity. However, when endoglucanase and xylanase were combined, a significant increase in the production of cellooligomers and xylooligomers was observed. This finding aligns with the results shown in Fig. 1D, which suggest that the optimal molar ratio in the combination of xylanase and endoglucanase is a 6-fold excess of xylanase over endoglucanase to remove residual xylan and amorphous cellulose in the pulp.
Figure 2: Effect of individual and combined endoglucanase and xylanase on cellulosic pulp hydrolysis, for 3 h. The dissolved monosaccharides and oligosaccharides concentration in each component was determined by HPLC
3.3 Influence of Enzymic Treatment on Pulp Structure
The characteristics of the fiber were assessed to better understand the correlation of RSSol and RSIns with enzymatic action on pulp. Fig. 3 displays optical microscopy images (A) and the particle size distribution of the fiber dimensions (B) of pulp treated with xylanase and endoglucanase, as well as with binary mixtures at different enzyme concentrations (B). The optical microscopy images provided insight into the visual appearance of the pulp samples, showing that treatments with individual enzymes did not appear to modify the fiber integrity, compared to the control. However, after the enzymatic treatment with xylanase and endoglucanase, shorter fiber fragments and fines were observed (Fig. 3A). Furthermore, it was observed that the particle size distribution of fiber remained unchanged when treated with xylanase and endoglucanase separately, compared to the control (Fig. 3B). However, when the enzymes were combined, a decrease in peak height was observed after treating the pulp with 500X:80EG, facilitating the process of fibrillation to the production of cellulose nanofibrils [44].
Figure 3: (A) Optical microscopy images of bleached kraft pulp control and after enzyme treatments. (B) The distribution of the size of fibrils from bleached kraft pulp without enzyme (control) and with xylanase (500U), endoglucanase (80U), and combinations of enzyme treatments (500X:80EG)
The effect of the enzymatic process on pulp was evaluated in terms of viscosity and reducing sugars produced. In terms of viscosity, the addition of xylanase to pulps did not result in significant change (Fig. 4A). The control sample had an intrinsic viscosity of 826 mL/g, which decreased to 768 mL/g when treated with a high xylanase dose (500U/g pulp). Ngene et al. [6] explained this phenomenon as being attributed to the capability of xylanase treatments to solubilize low molar mass chains. Experiments with different doses of endoglucanase (80, 160, and 320EGU/g pulp) presented viscosity values between 584 and 449 mL/g (Fig. 4B). Similar results were reported by Sponla et al. [36], who observed a decrease in viscosity from 930 to 430 ml/g as endoglucanase dose increased. Willberg-Keyrilainen [24] also found that viscosity decreased to about half of the original pulp viscosity, using varying concentrations of the same enzyme preparation, Fibercare. The variations in results were likely due to differences in the origin of the pulps and the doses of endoglucanase used in the experiments.
Figure 4: Pulp viscosity (--) and reducing sugars (
RSSol,
RSIns) produced as a function of enzymatic hydrolysis of the treated bleached kraft pulp at different endoglucanase (EG) and xylanase (X) loadings
When xylanase was combined with endoglucanase, the pulp viscosity decreased further, reaching 484 ml/g. Specifically, using the 5X:160EG enzyme dose resulted in a significant decrease in viscosity compared to the respective controls, bringing the final viscosity of the pulps within an acceptable range for commercial dissolving pulp [14,21]. Based on these findings, it can be concluded that the two enzyme doses tested had different effects. The overall decrease in viscosity observed in all treatments can be attributed to the degradation of cellulose chains, which leads to a decrease in the degree of polymerization. The endoglucanase used in the treatments is responsible for the degradation of the amorphous regions in the pulp, generating shorter chains and new chain ends. The concentration of xylanase used in the treatment affects the extent of this degradation, as it is related to the removal of xylans in the pulp, polymers that form networks that protect the cellulose chains and hinder their degradation [43]. This demonstrates the combined effect of the two treatments on the residual cellulose.
High solubilization of reducing sugars and little changes in RSIns were observed in pulps treated with different doses of xylanase complemented with endoglucanase (80EG/g pulp) (Fig. 4A). The measured value of RSIns in the pulps treated by xylanase was similar to the values recorded by pulp control. Insoluble reducing sugars in the pulp primarily come from the hydroxyl group at the end of the cellulose chain, also known as the reducing terminal [25]. Therefore, differences in the amount of RSIns have been observed after enzymatic treatment of pulps with the combination of xylanase and endoglucanase. The expressive increase in RSins was observed with 5X:160EGU and 5X:320EGU cocktails (Fig. 4B). The pulps had different contents of extractable polysaccharides (hemicelluloses and low molecular mass cellulose) under the tested conditions, leading to high solubilization of reducing sugars, although there is no existing precedent linking reducing sugars, which are byproducts of the enzymatic process, to the viscosity of pulp. Previous research has shown a comparison of reducing sugars released from the short fiber sulfate pulp by different enzyme preparations and the pulp viscosity. It was found that the sugars released varied significantly, despite the identical activity dose of endoglucanase. Surprisingly, the enzyme that caused the highest release of soluble reducing sugars did not result in a faster decrease in viscosity [24]. By examining the RSIns content in the pulp and viscosity, our results suggest a correlation between them. Therefore, we can estimate the impact of endoglucanase on viscosity before analysis (Fig. 5). The results indicate that endoglucanase causes significant degradation of cellulose chains and the presence of residual reducing sugars in the pulps.
Figure 5: Correlation between intrinsic viscosity and insoluble reducing sugar of bleached kraft pulps enzymatically treated with combined xylanase and endoglucanase
In general, small-sized fibers increase the production of reduced sugars and lead to a decrease in intrinsic viscosity. Based on the foregoing, viscosity was mainly influenced by the action of endoglucanase on pulp. Literature results of using endoglucanase for controlling viscosity are shown in Table 2. Variations of the intrinsic viscosity after endoglucanase treatments in pulps are dependent on different variables due to its inherent supramolecular features. However, in all treatment conditions, endoglucanase decreased the viscosity of pulps to acceptable values for viscose production (400–600 ml/g) [21]. The results of these studies might be helpful to make an adequate selection of processing conditions for dissolving pulp production.
The effect of xylanase on the solubilization of hemicellulose and the action of endoglucanase on amorphous cellulose can be evaluated using the DNS test to measure soluble and insoluble reducing sugars. Xylanase treatment predominantly enhanced the release of soluble reducing sugars of kraft pulp, while endoglucanase treatment resulted in changes in insoluble reducing sugars. The results showed that the RSIns proceeded at a much lower rate compared to RSSol, but both were equally reproducible. This suggests measuring both soluble and insoluble sugars is a practical process to evaluate an enzyme preparation under process conditions and this finding opens up the possibility for different enzymes to be exploited for the purification and activation of cellulosic pulps for dissolving grade pulp.
Synergistic effects between enzymes were a potential strategy to further enhance pulp properties. The maximum synergy between the two enzymes was observed at a high rate of endoglucanase to xylanase. This model of evaluation synergism based on RSIns is without precedent in the literature. Furthermore, the enzyme treatments led to modifications in pulp viscosity, a reduction in residual xylan, and changes in particle size. Overall, the DNS test proved to be a reliable and rapid parameter for assessing the efficiency of enzyme treatments on pulp, with significant correlations observed between RSins and the intrinsic viscosity of enzymatic-treated pulps.
Acknowledgement: B. Pereira is acknowledged for technical assistance in performing experiments, for the fiber length measurements.
Funding Statement: This work was supported by CNPq (303416/2018–1) and FAPESP (2019/25867-3). This study was also financed in part by the Coordenação de Aperfeiçoamento de Pessoal de Nível Superior—Finance Code 001.
Author Contributions: V.T.F.S. performed most of the experiments and drafted the manuscript and A.M.F.M. conceived the study, performed and supervised experiments, and prepared the final version.
Availability of Data and Materials: The data supporting the findings of this study are available from the corresponding author (Adriane Milagres, Email: adrianemilagres@usp.br), upon reasonable request.
Conflicts of Interest: The authors declare that they have no conflicts of interest to report regarding the present study.
References
1. Chapman, J., Ismail, A. E., Dinu, C. Z. (2018). Industrial applications of enzymes: Recent advances, techniques, and outlooks. Catalysts, 8(6), 238. [Google Scholar]
2. Yang, S., Yang, B., Duan, C., Fuller, D. A., Wang, X. et al. (2019). Applications of enzymatic technologies to the production of high-quality dissolving pulp: A review. Bioresource Technology, 281, 440–448. [Google Scholar] [PubMed]
3. Duan, C., Wang, X., Zhang, Y., Xu, Y., Ni, Y. (2017). Fractionation and cellulase treatment for enhancing the properties of kraft-based dissolving pulp. Bioresource Technology, 224, 439–444. [Google Scholar] [PubMed]
4. Zhao, L., Yuan, Z., Kapu, N. S., Chang, X. F., Beatson, R. et al. (2017). Increasing efficiency of enzymatic hemicellulose removal from bamboo for production of high-grade dissolving pulp. Bioresource Technology, 223, 40–46. [Google Scholar] [PubMed]
5. Balkissoon, S., Andrew, J., Sithole, B. (2023). Dissolving wood pulp production: A review. In: Biomass conversion and biorefinery, 13, 16607–16642. [Google Scholar]
6. Ngene, G. I., Roux, J. C., Lachenal, D. (2022). Xylan extraction strategies and the performance of the resulting high purity cellulose fiber to swelling and dissolution. Carbohydrate Polymer Technologies and Applications, 3, 100196. [Google Scholar]
7. Chen, C., Duan, C., Li, J., Liu, Y., Ma, X. et al. (2016). Cellulose (dissolving pulp) manufacturing processes and properties: A mini-review. BioResources, 11(2), 5553–5564. [Google Scholar]
8. Loureiro, P. G., Cadete, S. S., Tokin, R., Evtuguin, D. V., Lund, H. et al. (2021). Enzymatic fibre modification during production of dissolving wood pulp for regenerated cellulosic materials. Frontiers in Plant Science, 12, 717776. [Google Scholar] [PubMed]
9. Hult, E. L., Larsson, P. T., Iversen, T. (2001). Cellulose fibril aggregation—An inherent property of kraft pulps. Polymer, 42, 3309–3314. [Google Scholar]
10. Li, H., Legere, S., He, Z., Zhang, H., Li, J. et al. (2018). Methods to increase the reactivity of dissolving pulp in the viscose rayon production process: A review. Cellulose, 25(7), 3733–3753. [Google Scholar]
11. Kaur, P., Bhardwaj, N. K., Sharma, J. (2016). Pretreatment with xylanase and its significance in hemicellulose removal from mixed hardwood kraft pulp as a process step for viscose. Carbohydrate Polymers, 145, 95–102. [Google Scholar] [PubMed]
12. Köpcke, V., Ibarra, D., Ek, M. (2008). Increasing accessibility and reactivity of paper grade pulp by enzymatic treatment for use as dissolving pulp. Nordic Pulp & Paper Research Journal, 23(4), 363–368. [Google Scholar]
13. Ceccherini, S., Rahikainen, J., Marjamaa, K., Sawada, D., Grönqvist, S. et al. (2021). Activation of softwood kraft pulp at high solids content by endoglucanase and lytic polysaccharide monooxygenase. Industrial Crops and Products, 166, 113463. [Google Scholar]
14. Hutterer, C., Kliba, G., Punz, M., Fackler, K., Potthast, A. (2017). Enzymatic pulp upgrade for producing high-value cellulose out of a kraft paper pulp. Enzyme and Microbial Technology, 102, 67–73. [Google Scholar] [PubMed]
15. Henriksson, G., Christiernin, M., Agnemo, R. (2005). Monocomponent endoglucanase treatment increases the reactivity of softwood sulphite dissolving pulp. Journal of Industrial Microbiology and Biotechnology, 32(5), 211–214. [Google Scholar] [PubMed]
16. Duan, C., Long, Y., Li, J., Ma, X., Ni, Y. (2015). Changes of cellulose accessibility to cellulase due to fiber hornification and its impact on enzymatic viscosity control of dissolving pulp. Cellulose, 22(4), 2729–2736. [Google Scholar]
17. Duan, C., Verma, S. K., Li, J., Ma, X., Ni, Y. (2016). Viscosity control and reactivity improvements of cellulose fibers by cellulase treatment. Cellulose, 23, 269–276. [Google Scholar]
18. Fock, W. (1959). A modified method for determining the reactivity of viscose-grade dissolving pulps. Papier, 13, 92–95. [Google Scholar]
19. Ceccherini, S., Maloney, T. (2019). Assessing wood pulp reactivity through its rheological behavior under dissolution. Cellulose, 26, 9877–9888. [Google Scholar]
20. Kumar, A. (2021). Dissolving pulp production: Cellulases and xylanases for the enhancement of cellulose accessibility and reactivity. Physical Science Reviews, 6, 111–129. [Google Scholar]
21. Carrillo-Varela, I., Vidal, C., Vidaurre, S., Parra, C., Machuca, A. et al. (2022). Alkalinization of kraft pulps from Pine and Eucalyptus and its effect on enzymatic saccharification and viscosity control of cellulose. Polymers, 14, 3127. [Google Scholar] [PubMed]
22. Liu, S., He, H., Fu, X., Wang, Y., Wang, Q. et al. (2019). Correlation between Fock reactivity and intrinsic viscosity of dissolving pulp during cellulase treatment. Journal of Wood Chemical and Technology, 39, 296–303. [Google Scholar]
23. Nagl, M., Haske-Cornelius, O., Bauer, W., Csarman, F., Ludwig, R. et al. (2022). Towards a better understanding of synergistic enzyme effects during refining of cellulose fibers. Carbohydrate Polymer Technologies and Applications, 4, 100223. [Google Scholar]
24. Willberg-Keyrilainen, P., Ropponen, J., Lahtinen, M., Pere, J. (2019). Improved reactivity and derivatization of cellulose after pre-hydrolysis with commercial enzymes. BioResources, 14, 561–574. [Google Scholar]
25. Silveira, M. H. L., Aguiar, R. S., Siika-Ajo, M., Ramos, L. P. (2014). Assessment of the enzymatic hydrolysis profile of cellulosic substrates based on reducing sugar release. Bioresource Technology, 151, 392–396. [Google Scholar] [PubMed]
26. Ghose, T. K. (1987). Measurement of cellulase activities. Pure and Applied Chemistry, 59, 257–268. [Google Scholar]
27. Bailey, M. J., Biely, P., Poutanen, K. (1992). Interlaboratory testing of methods for assay of xylanase activity. Journal of Biotechnology, 23(3), 257–270. [Google Scholar]
28. Bradford, M. M. (1976). A rapid and sensitive method for the quantitation of microgram quantities of protein utilizing the principle of protein-dye binding. Analytical Biochemistry, 72, 248–254. [Google Scholar] [PubMed]
29. Miller, G. L. (1959). Use of dinitrosalicylic acid reagent for determination of reducing sugar. Analytical Chemistry, 31(3), 426–428. [Google Scholar]
30. Jeoh, T., Wilson, D. B., Walker, L. P. (2006). Effect of cellulase mole fraction and cellulose recalcitrance on synergism in cellulose hydrolysis and binding. Biotechnology Progress, 22, 270–277. [Google Scholar] [PubMed]
31. Sluiter, A., Hames, B., Ruiz, R., Scarlata, C., Sluiter, J. et al. (2008). Determination of sugars, byproducts, and degradation products in liquid fraction process samples. Laboratory Analytical Procedure (LAP) Technical Report. NREL/TP-510-42623. https://www.nrel.gov/docs/gen/fy08/42623.pdf (accessed on 09/06/2023). [Google Scholar]
32. Ferraz, A. A., Rodriguez, J., Freer, J., Baeza, J. (2000). Biodegradation of Pinus radiata softwood by white—and brown-rot fungi. Bioresource Technology, 74, 201–212. [Google Scholar]
33. Dias, I. K. R., Siqueira, G. A., Arantes, V. (2022). Xylanase increases the selectivity of the enzymatic hydrolysis with endoglucanase to produce cellulose nanocrystals with improved properties. International Journal of Biological Macromolecules, 220, 589–600. [Google Scholar] [PubMed]
34. Kangas, H., Felissia, F. E., Filgueira, D., Ehman, N. V., Vallejos, M. E. et al. (2019). 3D Printing high-consistency enzymatic nanocellulose obtained from a soda-ethanol-O2 pine sawdust pulp. Bioengineering, 6(3), 60. [Google Scholar] [PubMed]
35. Sporck, D., Reinoso, F. A. M., Rencoret, J., Gutiérrez, A., Rio, J. C. et al. (2017). Xylan extraction from pretreated sugarcane bagasse using alkaline and enzymatic approaches. Biotechnology for Biofuels, 10, 296. [Google Scholar] [PubMed]
36. Spönla, E., Rahikainen, J., Potthast, A., Grönqvist, S. (2023). High consistency enzymatic pretreatment of eucalyptus and softwood kraft fibres for regenerated fibre products. Cellulose, 30, 4609–4622. [Google Scholar]
37. Liu, X., Jiang, Y., Wang, L., Song, X., Qin, C. et al. (2020). Tuning of size and properties of cellulose nanofibers isolated from sugarcane bagasse by endoglucanase-assisted mechanical grinding. Industrial Crops and Products, 146, 112201. [Google Scholar]
38. Ko, C. H., Chen, F. J., Lee, J. J., Tzou, D. L. M. (2011). Effects of fiber physical and chemical characteristics on the interaction between endoglucanase and eucalypt fibers. Cellulose, 18, 1043–1054. [Google Scholar]
39. Ceccherini, S., Stahl, M., Sawada, D., Hummel, M., Maloney, T. C. (2021). Effect of enzymatic depolymerization of cellulose and hemicelluloses on the direct dissolution of prehydrolysis kraft dissolving pulp. Biomacromolecules, 22, 4805–4813. [Google Scholar] [PubMed]
40. Vlasenko, E., Schülein, M., Cherry, J., Xu, F. (2010). Substrate specificity of family 5, 6, 7, 9, 12, and 45 endoglucanases. Bioresource Technology, 101, 2405–2411. [Google Scholar] [PubMed]
41. Hu, J., Tian, D., Renneckar, S., Saddler, J. N. (2018). Enzyme mediated nanofibrillation of cellulose by the synergistic actions of an endoglucanase, lytic polysaccharide monooxygenase (LPMO) and xylanase. Scientific Reports, 8, 4–11. [Google Scholar]
42. Song, H. T., Gao, Y., Yang, Y. M., Xiao, W. J., Liu, S. H. et al. (2016). Synergistic effect of cellulase and xylanase during hydrolysis of natural lignocellulosic substrates. Bioresource Technology, 219, 710–715. [Google Scholar] [PubMed]
43. Nagl, M., Haske-Cornelius, O., Skopek, L., Pellis, A., Bauer, W. et al. (2021). Biorefining: The role of endoglucanases in refining of cellulose fibers. Cellulose, 28, 7633–7650. [Google Scholar]
44. Las-Casas, B., Arantes, V. (2023). Endoglucanase pretreatment aids in isolating tailored-cellulose nanofibrils combining energy saving and high-performance packaging. International Journal of Biological Macromolecules, 242, 125057. [Google Scholar] [PubMed]
Cite This Article
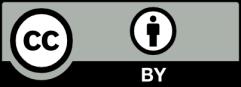