Open Access
REVIEW
The Eph/Ephrin System in Hepatocellular Carcinoma: Functional Roles and Potential Therapeutic Targets
Institute of Infection, Immunology and Tumor Microenvironent, Hubei Province Key Laboratory of Occupational Hazard Identification and Control, Medical College, Wuhan University of Science and Technology, Wuhan, 430065, China
* Corresponding Authors: Qiang Wang. Email: ; Qingming Wu. Email:
Oncologie 2022, 24(3), 427-439. https://doi.org/10.32604/oncologie.2022.023248
Received 16 April 2022; Accepted 12 August 2022; Issue published 19 September 2022
Abstract
Liver cancer is ranked as the second leading cause of cancer-related death across the world, and hepatocellular carcinoma (HCC) comprises nearly 90% of all primary liver cancer cases. However, until now, the pathological mechanisms underlying HCC oncogenesis and development remains largely unclear. Eph receptors and their ephrin ligands (Eph/Ephrin system) are involved in various physiological process, such as embryogenesis, neuronal migration, immune response and vascular development. Recently, emerging evidence has showed that the Eph/Ephrin system also plays an essential role in initiation and development of multiple types of cancer, including liver cancer. The Eph/Ephrin system has been reported to contribute to cellular proliferation, apoptosis, migration, invasion, angiogenesis, stemness and drug/radiotherapy resistance in HCC. Thus, the aim of this review is to summarize the effects and underlying mechanisms of every member of this system in HCC, with special focus on assessing their therapeutic potential as drug targets.Keywords
Liver cancer is ranked as the second leading cause of cancer-related death across the world [1]. As the most common type of liver cancer, hepatocellular carcinoma (HCC) comprises nearly 90% of all primary liver cancer cases with a prevalence of 10.1 cases in 100000 worldwide [2,3]. The incidence of HCC is associated with geography and household income [4]. The majority of HCC occur in eastern Asia and Africa. At present, Hepatitis B virus (HBV) and hepatitis C virus (HCV) remain the most dominant etiologic factors for HCC, but effective prevention and treatment will decrease incidence of HBV/HCV-associated HCC in the coming years [5]. Recently, non-alcoholic fatty liver disease (NAFLD) is emerging as an important cause of HCC with the most rapidly increasing incidence [6]. In addition, excessive alcohol consumption and aflatoxin are also important risk factors of HCC [5]. Patients with HCC have usually developed advanced-staged disease at the time of diagnosis, for whom targeted therapy is an important strategy to control tumor progression. Although tyrosine kinase inhibitors (TKIs) have been recommended for advanced HCC patients, recent studies have shown that a considerable proportion of HCC patients are less responsive to TKIs therapy [7,8]. Therefore, a more profound and comprehensive understanding of molecular mechanism underlying biological process of tumor, such as proliferation, invasion, migration, angiogenesis and drug resistance, will serve to establish more effective diagnostic and treatment approaches of HCC.
Cancer stem cells (CSCs) are thought as drivers of establishment and growth of HCC, and associated with aggressiveness and therapy resistance of tumors [9]. CSCs arise from either de-differentiation of mature cells or transformation of adult tissue resident stem cells [10]. Chronic inflammation plays a prominent role in inducing mutation and dedifferentiation of hepatocytes during tumorigenesis and development of HCC [11]. Liver cirrhosis caused by chronic hepatitis was founded to be present in most HCC cases (80%∼90%) and exacerbated impairment of liver function [12]. Studies have demonstrated that some cytokine signaling and immune-related pathways including tumor necrosis factor-α (TNF-α), interleukin-6 (IL-6), signal transducer and activator of transcription 3 (STAT3), and nuclear factor kappa B (NF-κB) pathways are involved in chronic inflammation-related hepatocarcinogenesis [13–15]. However, the concrete mechanism has not yet been illuminated. In the past few years, dysregulation of erythropoietin-producing human hepatocellular receptors (Ephs) and their corresponding ligands ephrins was founded in multiple types of cancer including HCC and was associated with tumor initiation and/or progression [16–18]. In this review, we summarized the roles of Ephs and ephrins in HCC, and assessed therapeutic potential of targeting these molecules.
2 The Structure and Signaling of Eph/Ephrin
As the largest known subfamily of receptor tyrosine kinases (RTK), Eph receptors are classified into two categories according to their structure similarity and DNA sequence homology: EphA receptors (EphA1-8, EphA10) and EphB receptors (EphB1-4, EphB6). Generally, the EphA receptors promiscuously bind to ephrinA ligands (ephrinA1-5), while EphB receptors bind to ephrinB ligands (ephrinB1-3). However, there are some exceptions from the general principle (such as EphA4 binding to ephrinB2 and EphB2 binding to ephrinA5) [19,20].
The Eph receptors and ephrins are ubiquitously expressed on cell membrane in a variety of human cell types. The extracellular domain of Eph receptors consists of two fibronectin type III regions, a cysteine rich region and a globular domain which can bind to corresponding ephrins ligands. The intracellular domain is composed of a conserved tyrosine kinase domain, a sterile alpha motif (SAM) domain and a PDZ motif. The ephrinAs are anchored to the cell membrane via a glycosylphosphatidylinositol (GPI) linker, while the ephrinBs have a transmembrane domain and an intracellular PDZ motif. Typically, the ligand binding activation of Eph receptors is dependent on cell-cell interaction between adjacent plasma membranes. Subsequently, bi-directional signals are triggered between the two cells (Fig. 1). In forward signaling, after ephrin ligands bind to an Eph receptor, intracellular tyrosine residues of Eph receptor are auto-phosphorylated, and some adaptor proteins (such as including Ras-GTPase-activating protein, JAK2 and PI3K) are recruited to the receptor to initiate downstream signaling cascades into the cell [21,22]. Due to lack of intracellular domain, reverse signaling of ephrinAs is mediated by co-receptors including Ret receptor tyrosine kinase, tropomyosin receptor kinase B and p75 neurotrophin receptor [23]. In contrast, as ephrinBs also contain an intracellular PDZ domain, the downstream pathways of ephrinBs reverse signaling are similar to Eph forward signaling [22]. Intriguingly, recent studies indicated a soluble ephrins ligands-mediated non-classical mechanism via autocrine and/or paracrine pathways in some pathological conditions including cancer [24–26]. Ephs-ephrins signaling mediates a variety of cellular processes including cell migration, adhesion, proliferation, and differentiation [27]. Furthermore, it is also involved in immune response and angiogenesis [28,29], which are closely related to cancer development.
Figure 1: The structure and signaling of Eph/Ephrin system
After binding of ephrin ligands (membrane-anchored or unanchored), Eph receptors are auto-phosphorylated to activate downstream signaling including Ras-GTPase-activating protein (Ras-GAP), JAK2 and PI3K. On the other hand, the interaction between Eph receptors and ephrin ligands can also trigger reverse signaling via ephrinAs-coreceptors or ephrinBs.
Up to date, 9 of Ephs (EphA1-5, EphA7, EphB1-2 and EphB6) and 6 of ephrins (ephrinA1-5 and ephrinB1) have been investigated in HCC. In general, Eph/Ephrin system plays a pro-tumorigenic role in HCC, expect for EphA4 and ephrinA5 which exhibit an anti-tumor effect. The system has been confirmed to be involved in regulation of cellular proliferation, migration, invasion, apoptosis, angiogenesis, maintenance of stemness and drug/radiotherapy resistance in HCC (Fig. 2).
Figure 2: Eph/Ephrin system is involved in regulation of cellular proliferation, apoptosis, angiogenesis, migration, invasion and drug/radiotherapy resistance
During establishment and development of HCC, each subtype of Eph/Ephrin system has a distinct role. Subtypes marked with red font promote tumorigenesis, while subtypes marked with green font suppress tumorigenesis.
EphA1
As the first discovered subtype in Eph/ephrin system, EphA1 was identified in an erythropoietin-producing human HCC cell line (ETL-1) and was deemed to be probably associated with some neoplastic process in 1987 [30]. Later, Iida et al. showed that EphA1 was overexpressed in human HCC tissues producing AFP and HCC cell lines, and that the activation of EphA1 stimulated by ephrinA1-fc promoted proliferation of HLE cells by suppressing p21 expression [31]. Furthermore, stable knockdown of EphA1 in Huh-7 cells decreased proliferation, motility and invasion capability in vitro and reduced tumor growth as well as microvascular density in vivo [32]. The loss of EphA1 also reduced the expression and MMP2, MMP9 and VEGF, which contributed to angiogenesis and development of HCC [32,33]. A recent study verified that EphA1 in HCC cells could activate the SDF-1/CXCR4 axis by PI3K and mTOR pathways and enhanced the chemotaxis of endothelial progenitor cells (EPCs) to HCC, which in turn promoted tumor neovascularization [34].
EphA2
Multiple clinical researches have demonstrated that EphA2 was overexpressed in HCC and was associated with dedifferentiation, TNM stage, portal vein invasion, microvascular density and rate of recurrence [35–37]. Mechanistically, EphA2 drove HCC tumor initiation and progression via AKT and JAK1/STAT3 signaling [38]. In HBV-associated HCC, EphA2 also promoted HBV replication and tumor cell proliferation via p38MAPK and ERK1/2 pathways [39]. Besides, there is emerging evidence that EphA2 can enhance tumor stemness of HCC in a hypoxic microenvironment which is formed due to fast-growing nature of tumor and insufficient vasculatures [40].
Radiotherapy is an effective strategy for cancer and can elevate local control rates in patients with unresectable HCC [41]. However, resistance to radiotherapy is common [42]. The resistance to radiotherapy in HCC seems to correlate with overexpression of EphA2. In HCC cells, up-regulation of EphA2 attenuated radio-sensitivity via p38MAPK pathway, with a significant increase in cell viability and healing capacity as well as a decline in apoptosis rate after low-dose ionizing radiation, while knockdown of EphA2 reversed the above effects [43,44]. In addition, EphA2 was also involved in paclitaxel resistance in HCC cells [45].
EphA3
Cheng et al. reported that expression of EphA3 was positively correlated with tumor grade, tumor size and TNM stage in HCC [46]. In line with this finding, the downregulation of EphA3 markedly dampened the invasive capacity of HCC cells by decreasing expression of VEGF in vitro [46]. Recently, some novel mutations of EhpA3, including one D219V missense mutation in the extracellular domain and two alterations in SAM domain, were detected in HCC tissues. These mutations may participate in progression of HCC [47].
EphA4
Differing from other Eph receptors, EphA4 appears to play a tumor suppressive role in HCC. Decreased EphA4 expression was observed in HCC compared adjacent non-tumor liver tissues [48]. Overexpression of EphA4 inhibited invasion and migration by way blocking the epithelial-mesenchymal transition (EMT) process in QGY-7703 cells [48,49]. This, in fact, is not surprising because activation of EphA4 can inhibit integrin signaling pathways which is essential in invasion and metastasis of tumor [50,51].
EphA5
Wang et al. observed that p-EphA5 (activated form of EphA5) was abundant in HCC tissues and HCC cell lines [52]. The activation of EphA5 is required for tumor growth and is highly associated with worse prognosis in patients with HCC. Furthermore, EphA5 was recognized as one of client proteins of Hsp90, which can help oncoproteins to avoid degradation and misfolding in HCC cells [52,53].
EphA7
The mRNA level of EphA7 was founded to be up-regulated 26-fold in HCC compared with healthy liver tissue [54]. Besides, the staining for EphA7 was clearly strong and intense in blood vessels. Similarly, expression of EPHA7 was positively related to microvascular density of tumor [55]. This suggested EphA7 may exert a pro-angiogenic signal in HCC, although further validation is still needed.
EphB1
Currently, very few studies explored the roles of EphB1 in HCC. Kim et al. identified seven polymorphisms of the EphB1 gene (rs1502174, rs9877457rs, 11929692, rs6766459, rs7644369, rs6776570 and rs3821502) which may be associated with susceptibility to HCC in the South Korean population [56]. However, the effects of EphB1 on initiation and development of HCC remain to be elucidated.
EphB2
As mentioned above, a stepwise increase of EphB2 expression was observed from normal liver to fibrotic liver to HCC tissue, and the overexpression was associated with worse prognosis in HCC patients [54,57,58]. In vivo, knockout of EphB2 attenuated tumor growth of HCC. Furthermore, RNA-sequencing analysis revealed that EphB2 is the most significantly upregulated kinases in sorafenib-resistant patient-derived tumor xenografts (PDX) compared with their corresponding mock controls, which suggested that EphB2 was related to drug resistance. High expression of EphB2 was also founded to enhance the stemness of HCC cells. Mechanistically, EphB2 activated AKT/GSK3β/β-catenin signaling pathway by physically interacting with and phosphorylating SRC. In turn, β-catenin/TCF1 pathway initiated transcription of EphB2 gene, which forms a TCF1/EphB2/β-catenin positive feedback loop finally [58].
EphB6
Pharmacogenomic data analysis uncovered that EphB6 mutations (mainly nonsense mutations and a missense mutation, Q926R) were involved in paclitaxel resistance in cancer [45]. Q926R mutation reduced the flexibility of the SAM domain, thereby inhibiting degradation of EphA2 by suppressing recruitment of c-Cbl ubiquitin E3 ligase. Subsequently, EphA2 promoted JNK-mediated expression of cadherin 11 (CDH11) and activated RhoA/FAK signaling pathway, which in turn induced cell adhesion-mediated paclitaxel resistance in HCC cells.
EphrinA1
As the ligand of EphA1 and EphA2, ephrinA1 expression was elevated in cirrhotic tissues and HCC tissues, and was significantly associated with AFP expression and prognosis of patients [31,35,59]. In HLE cells, up-regulation of ephrinA1 promoted the cellular proliferation. Furthermore, ephrinA1 regulating tumor development by inducing expression of genes related to the cell cycle, cell-cell interactions and angiogenesis. Notably, ephrinA1 is detectable in the serum of patients with HCC, suggesting it may be a useful marker for the diagnosis of HCC and a predictor for outcome of patients [35].
EphrinA2
Feng et al. described a significant increase of ephrinA2 expression in both cell lines and clinical samples of HCC, especially in the tumors with portal veins invasion [60]. Overexpression of EphrinA2 in HCC cells significantly enhanced oncogenic effect in vivo tumorigenicity, while down-regulation of this gene inhibited tumor growth. Further exploration revealed that the reduced Rac1/Akt/NF-κB pathway-related apoptosis, rather than enhanced proliferation, was responsible for ephrinA2-induced tumorigenesis.
EphrinA3
Hypoxia regions within tumors have been demonstrated to be associated with poor prognosis of patient and are now recognized to be involved in maintenance of stemness, metabolism, drug resistance and metastasis in various tumors [61–64]. Hypoxia inducible factor 1 alpha (HIF-1α) is one of key transcription factors that help tumors to make adaptive changes for cellular survival under hypoxia. Hypoxic-induced HIF-1α can directly promote expression of ephrinA3 to active EphA2 in HCC cells. And then, the activated ephrinA3/EphA2 axis enhanced the abilities of self-renewal, proliferation and migration in HCC by regulating cellular metabolic plasticity via promoting the maturation of SREBP1 and expression of ACLY [40].
EphrinA4
EphrinA4 was founded to be highly expressed in HCC samples and associated with poor overall survival and progression-free survival [65]. High expression of ephrinA4 promoted the cellular migration and proliferation by activating EphA2 receptor phosphorylation at Ser897 and downstream PIK3R2/GSK3b/β-catenin positive feedback loop in HCC cells.
EphrinA5
EphrinA5, as the ligand of EphA4, also exhibited a tumor-suppressive effect as expect. Wang et al. identified two alternative isoforms of ephrinA5 in clinical samples, a large isoform (ephrinA5L) and a small isoform (ephrinA5S) [66]. Both ephrinA5S and ephrinA5L were down-regulated in HCC samples, but only expression of ephrinA5S was significantly correlated with prognosis of patients after resection. Moreover, ephrinA5S showed a more pronounced inhibitory effect compared with ephrinA5L on cellular proliferation and migration in vitro. The anti-tumor effect of ephrinA5 was attributable to c-Cbl-mediated EGFR degradation in HCC [66].
EphrinBs
The mRNA level of the ephrinB1 was significantly higher in HCC samples than in normal liver, while the differences in expression of ephrinB2 and ephrinB3 were not obvious [67]. In vivo, the up-regulation of ephrinB1 accelerated tumor growth and increased tumor vessel number. In addition, in vitro experiments confirmed that ephrinB1 promoted neovascularization by inducing proliferation and migration of human umbilical vein endothelial cells (HUVECs) [67].
4 Therapeutic Potential of Eph/Ephrin-Targeted Drugs in HCC
Over the last decade, advances in systemic therapy have contributed to tremendous improvements for patients diagnosed with advanced-stage HCC or patients who experienced failures in other therapies. As our knowledge of the molecular profiling changes regulating tumorigenesis evolves, some targeted drugs have been developed and been implemented in the clinic, which ushered a new era in treatment in HCC. A few drugs have been confirmed to significantly improve prognosis of patients with HCC compared with placebo, such as sorafenib and regorafenib [68–70]. However, drug resistance is frequently observed especially in patients who receiving long-term corresponding drugs treatment [71,72]. Therefore, the identification of novel targets and prognostic predictors in HCC remains crucial and will facilitate the shift towards individualized precision medicine.
As described above, multiple members of Eph/Ephrin system are involved in oncogenesis and associated with clinical outcome of patients in HCC. Blocking tumor-promoting Eph/Ephrin-related signal pathways could offer an effective strategy for treatment in HCC. Many Eph/Ephrin system-targeted drugs have been developed and investigated in cancers, such as antibodies, recombinant proteins and small molecules [18,73]. Some of these drugs have entered to clinical trials (Table 1). However, among them only sEphB4-HSA was evaluated in HCC in a phase I study, which reported that 1 of 8 HCC patients had a partial response [74].
On the other hand, results from preclinical studies provided novel promising strategies for design and selection of Eph/Ephrin system-targeted drugs in HCC treatment (Table 2). Yamaguchi et al. reported that EphA2-derived peptide vaccine with amphiphilic poly nanoparticles (Eph-NPs) exhibited an anti-tumor effect against liver cancer in mice [82]. Immunization with Eph-NPs promoted generation of EphA2-specific CD8+ T cells without any toxic damage to liver [82]. As a selective EphA2 small molecular inhibitor, ALW-II-41–27 was recently confirmed to have anti-tumor effects in many malignant tumors [83–85]. In HCC, ALW-II-41–27 significantly impaired the capacity of cellular proliferation in vitro [38]. Due to the specific tropism of rAAV-8 for liver in vivo, rAAV-8-shEphB2 was established by et al. for treatment of HCC [58,86]. In immunocompetent mice, rAAV-8-shEphB2 increased sensitivity of HCC to sorafenib [58].
Accumulative evidence demonstrated that Eph receptors and their corresponding ephrin ligands had an important role in the initiation and development of HCC. In general, Ephs and ephrins exhibited a pro-tumorigenic effect in HCC by influencing proliferation, apoptosis, migration, invasion, angiogenesis, stemness and drug/radiotherapy resistance, although there are exceptions (EphA4 and ephrinA5 as tumor suppressors). However, the precise mechanisms and roles of Eph/Ephrin in HCC remain unclear. Specifically, the reverse signaling of ephrins is almost never mentioned in HCC. Besides, given the key function of the system in immunity, the association between Eph/Ephrin and tumor immune microenvironment in HCC deserves further exploration in order to offer possible strategies for immunotherapy. Furthermore, although their therapeutic potential is promising, the high promiscuity between Ephs and ephrins can easily surmount the selective blockage of a single target. Thus, designing multitargeted anti-Eph/Ephrin drugs may be a better strategy. For example, UniPR1331 has been identified as a broad-spectrum antagonist of the Eph/Ephrin system, and its effect on HCC should be further investigated in the future. On the other hand, due to the ubiquitous expression of the system in various tissues, non-specific targeting should be avoided.
Authorship: QMW and QW conceived the project; XS carried out the database and publication searching and summary; XS wrote the original manuscript; all authors revised the manuscript and approved the final manuscript.
Ethics Approval and Informed Consent Statement: This study was approved by local ethical authorities of Medical School of Wuhan University of Science and Technology (202179).
Availability of Data and Materials: All available data have been included in the final manuscript.
Funding Statement: This study was funded by China Undergraduate Innovation and Entrepreneurship Training Project (Xin Shu, Grant No. 202110488025).
Conflicts of Interest: The authors declare that they have no conflicts of interest to report regarding the present study.
Reference
1. Sia, D., Villanueva, A., Friedman, S. L., Llovet, J. M. (2017). Liver cancer cell of origin, molecular class, and effects on patient prognosis. Gastroenterology, 152(4), 745–761. DOI 10.1053/j.gastro.2016.11.048. [Google Scholar] [CrossRef]
2. Llovet, J. M., Zucman-Rossi, J., Pikarsky, E., Sangro, B., Schwartz, M. et al. (2016). Hepatocellular carcinoma. Nature Reviews Disease Primers, 2, 16018. DOI 10.1038/nrdp.2016.18. [Google Scholar] [CrossRef]
3. Forner, A., Reig, M., Bruix, J. (2018). Hepatocellular carcinoma. Lancet, 391, 1301–1314. DOI 10.1016/S0140-6736(18)30010-2. [Google Scholar] [CrossRef]
4. Wong, R. J., Saab, S., Konyn, P., Sundaram, V., Khalili, M. (2021). Rural-urban geographical disparities in hepatocellular carcinoma incidence among US adults, 2004–2017. American Journal of Gastroenterology, 116(2), 401–406. DOI 10.14309/ajg.0000000000000948. [Google Scholar] [CrossRef]
5. McGlynn, K. A., Petrick, J. L., El-Serag, H. B. (2021). Epidemiology of hepatocellular carcinoma. Hepatology, 73, 4–13. DOI 10.1002/hep.31288. [Google Scholar] [CrossRef]
6. Ioannou, G. N. (2021). Epidemiology and risk-stratification of NAFLD-associated HCC. Journal of Hepatology, 75(6), 1476–1484. DOI 10.1016/j.jhep.2021.08.012. [Google Scholar] [CrossRef]
7. Mou, L., Tian, X., Zhou, B., Zhan, Y., Chen, J. et al. (2021). Improving outcomes of tyrosine kinase inhibitors in hepatocellular carcinoma: New data and ongoing trials. Frontiers in Oncology, 11, 752725. DOI 10.3389/fonc.2021.752725. [Google Scholar] [CrossRef]
8. Marin, J. J. G., Macias, R. I. R., Monte, M. J., Romero, M. R., Asensio, M. et al. (2020). Molecular bases of drug resistance in hepatocellular carcinoma. Cancers, 12(6), 1663. DOI 10.3390/cancers12061663. [Google Scholar] [CrossRef]
9. Liu, Y. C., Yeh, C. T., Lin, K. H. (2020). Cancer stem cell functions in hepatocellular carcinoma and comprehensive therapeutic strategies. Cells, 9(6). DOI 10.3390/cells9061331. [Google Scholar] [CrossRef]
10. Walcher, L., Kistenmacher, A. K., Suo, H., Kitte, R., Dluczek, S. et al. (2020). Cancer stem cells-origins and biomarkers: Perspectives for targeted personalized therapies. Frontiers in Immunology, 11, 1280. DOI 10.3389/fimmu.2020.01280. [Google Scholar] [CrossRef]
11. Yang, Y. M., Kim, S. Y., Seki, E. (2019). Inflammation and liver cancer: Molecular mechanisms and therapeutic targets. Seminars in Liver Disease, 39(1), 26–42. DOI 10.1055/s-0038-1676806. [Google Scholar] [CrossRef]
12. Ringelhan, M., Pfister, D., O’Connor, T., Pikarsky, E., Heikenwalder, M. (2018). The immunology of hepatocellular carcinoma. Nature Immunology, 19(3), 222–232. DOI 10.1038/s41590-018-0044-z. [Google Scholar] [CrossRef]
13. Nakagawa, H., Umemura, A., Taniguchi, K., Font-Burgada, J., Dhar, D. et al. (2014). ER stress cooperates with hypernutrition to trigger TNF-dependent spontaneous HCC development. Cancer Cell, 26(3), 331–343. DOI 10.1016/j.ccr.2014.07.001. [Google Scholar] [CrossRef]
14. Gu, F. M., Li, Q. L., Gao, Q., Jiang, J. H., Zhu, K. et al. (2011). IL-17 induces AKT-dependent IL-6/JAK2/STAT3 activation and tumor progression in hepatocellular carcinoma. Molecular Cancer, 10, 150. DOI 10.1186/1476-4598-10-150. [Google Scholar] [CrossRef]
15. Taniguchi, K., Karin, M. (2018). NF-kappaB, inflammation, immunity and cancer: Coming of age. Nature Reviews Immunology, 18(5), 309–324. DOI 10.1038/nri.2017.142. [Google Scholar] [CrossRef]
16. Pergaris, A., Danas, E., Goutas, D., Sykaras, A. G., Soranidis, A. et al. (2021). The clinical impact of the EPH/Ephrin system in cancer: Unwinding the thread. Journal of Molecular Sciences, 22(16). DOI 10.3390/ijms22168412. [Google Scholar] [CrossRef]
17. Lodola, A., Giorgio, C., Incerti, M., Zanotti, I., Tognolini, M. (2017). Targeting Eph/ephrin system in cancer therapy. European Journal of Medicinal Chemistry, 142, 152–162. DOI 10.1016/j.ejmech.2017.07.029. [Google Scholar] [CrossRef]
18. Buckens, O. J., El Hassouni, B., Giovannetti, E., Peters, G. J. (2020). The role of Eph receptors in cancer and how to target them: Novel approaches in cancer treatment. Expert Opinion on Investigational Drugs, 29(6), 567–582. DOI 10.1080/13543784.2020.1762566. [Google Scholar] [CrossRef]
19. Kania, A., Klein, R. (2016). Mechanisms of ephrin-Eph signalling in development, physiology and disease. Nature Reviews Molecular Cell Biology, 17(4), 240–256. DOI 10.1038/nrm.2015.16. [Google Scholar] [CrossRef]
20. Wykosky, J., Palma, E., Gibo, D. M., Ringler, S., Turner, C. P. et al. (2008). Soluble monomeric EphrinA1 is released from tumor cells and is a functional ligand for the EphA2 receptor. Oncogene, 27(58), 7260–7273. DOI 10.1038/onc.2008.328. [Google Scholar] [CrossRef]
21. Kullander, K., Klein, R. (2002). Mechanisms and functions of Eph and ephrin signalling. Nature Reviews Molecular Cell Biology, 3(7), 475–486. DOI 10.1038/nrm856. [Google Scholar] [CrossRef]
22. Darling, T. K., Lamb, T. J. (2019). Emerging roles for Eph receptors and ephrin ligands in immunity. Frontiers in Immunology, 10, 1473. DOI 10.3389/fimmu.2019.01473. [Google Scholar] [CrossRef]
23. Lisabeth, E. M., Falivelli, G., Pasquale, E. B. (2013). Eph receptor signaling and ephrins. Cold Spring Harbor Perspectives in Biology, 5(9), 1288–1302. DOI 10.1101/cshperspect.a009159. [Google Scholar] [CrossRef]
24. Ieguchi, K., Tomita, T., Omori, T., Komatsu, A., Deguchi, A. et al. (2014). ADAM12-cleaved Ephrin-A1 contributes to lung metastasis. Oncogene, 33(17), 2179–2190. DOI 10.1038/onc.2013.180. [Google Scholar] [CrossRef]
25. Oricchio, E., Nanjangud, G., Wolfe, A. L., Schatz, J. H., Mavrakis, K. J. et al. (2011). The Eph-receptor A7 is a soluble tumor suppressor for follicular lymphoma. Cell, 147(3), 554–564. DOI 10.1016/j.cell.2011.09.035. [Google Scholar] [CrossRef]
26. Lagares, D., Ghassemi-Kakroodi, P., Tremblay, C., Santos, A., Probst, C. K. et al. (2017). ADAM10-mediated Ephrin-B2 shedding promotes myofibroblast activation and organ fibrosis. Nature Medicine, 23(12), 1405–1415. DOI 10.1038/nm.4419. [Google Scholar] [CrossRef]
27. Kandouz, M. (2012). The Eph/Ephrin family in cancer metastasis: Communication at the service of invasion. Cancer and Metastasis Review, 31(1–2), 353–373. DOI 10.1007/s10555-012-9352-1. [Google Scholar] [CrossRef]
28. Munoz, J. J., Cejalvo, T., Alonso-Colmenar, L. M., Alfaro, D., Garcia-Ceca, J. et al. (2011). Eph/Ephrin-mediated interactions in the thymus. Neuroimmunomodulation, 18(5), 271–280. DOI 10.1159/000329490. [Google Scholar] [CrossRef]
29. Hiratsuka, S. (2011). Vasculogenensis, angiogenesis and special features of tumor blood vessels. Frontiers in Molecular Biosciences, 16(4), 1413–1427. DOI 10.2741/3796. [Google Scholar] [CrossRef]
30. Hirai, H., Maru, Y., Hagiwara, K., Nishida, J., Takaku, F. (1987). A novel putative tyrosine kinase receptor encoded by the eph gene. Science, 238(4834), 1717–1720. DOI 10.1126/science.2825356. [Google Scholar] [CrossRef]
31. Iida, H., Honda, M., Kawai, H. F., Yamashita, T., Shirota, Y. et al. (2005). Ephrin-A1 expression contributes to the malignant characteristics of {α}-fetoprotein producing hepatocellular carcinoma. Gut, 54(6), 843–851. DOI 10.1136/gut.2004.049486. [Google Scholar] [CrossRef]
32. Chen, G., Wang, Y., Zhou, M., Shi, H., Yu, Z. et al. (2010). EphA1 receptor silencing by small interfering RNA has antiangiogenic and antitumor efficacy in hepatocellular carcinoma. Oncology Report, 23(2), 563–570. [Google Scholar]
33. Yoshiji, H., Kuriyama, S., Noguchi, R., Yoshii, J., Ikenaka, Y. et al. (2005). Angiopoietin 2 displays a vascular endothelial growth factor dependent synergistic effect in hepatocellular carcinoma development in mice. Gut, 54(12), 1768–1775. DOI 10.1136/gut.2005.067900. [Google Scholar] [CrossRef]
34. Wang, Y., Yu, H., Shan, Y., Tao, C., Wu, F. et al. (2016). EphA1 activation promotes the homing of endothelial progenitor cells to hepatocellular carcinoma for tumor neovascularization through the SDF-1/CXCR4 signaling pathway. Journal of Experimental and Clinical Cancer Research, 35, 65. DOI 10.1186/s13046-016-0339-6. [Google Scholar] [CrossRef]
35. Cui, X. D., Lee, M. J., Yu, G. R., Kim, I. H., Yu, H. C. et al. (2010). EFNA1 ligand and its receptor EphA2: Potential biomarkers for hepatocellular carcinoma. International Journal of Cancer, 126(4), 940–949. DOI 10.1002/ijc.24798. [Google Scholar] [CrossRef]
36. Yang, P., Yuan, W., He, J., Wang, J., Yu, L. et al. (2009). Overexpression of EphA2, MMP-9, and MVD-CD34 in hepatocellular carcinoma: Implications for tumor progression and prognosis. Hepatology Research, 39(12), 1169–1177. DOI 10.1111/j.1872-034X.2009.00563.x. [Google Scholar] [CrossRef]
37. Fan, M., Liu, Y., Xia, F., Wang, Z., Huang, Y. et al. (2013). Increased expression of EphA2 and E-N cadherin switch in primary hepatocellular carcinoma. Tumori Journal, 99(6), 689–696. DOI 10.1700/1390.15457. [Google Scholar] [CrossRef]
38. Wang, H., Hou, W., Perera, A., Bettler, C., Beach, J. R. et al. (2021). Targeting EphA2 suppresses hepatocellular carcinoma initiation and progression by dual inhibition of JAK1/STAT3 and AKT signaling. Cell Reports, 34(8), 108765. DOI 10.1016/j.celrep.2021.108765. [Google Scholar] [CrossRef]
39. Tian, J. H., Liu, W. D., Zhang, Z. Y., Tang, L. H., Li, D. et al. (2019). Influence of miR-520e-mediated MAPK signalling pathway on HBV replication and regulation of hepatocellular carcinoma cells via targeting EphA2. Journal of Viral Hepatitis, 26(4), 496–505. DOI 10.1111/jvh.13048. [Google Scholar] [CrossRef]
40. Husain, A., Chiu, Y. T., Sze, K. M., Ho, D. W., Tsui, Y. M. et al. (2022). Ephrin-A3/EphA2 axis regulates cellular metabolic plasticity to enhance cancer stemness in hypoxic hepatocellular carcinoma. Journal of Hepatology, 77(2), 383–396. DOI 10.1016/j.jhep.2022.02.018. [Google Scholar] [CrossRef]
41. Chen, C. P. (2019). Role of radiotherapy in the treatment of hepatocellular carcinoma. Journal of Clinical and Translational Hepatology, 7(2), 183–190. DOI 10.14218/JCTH.2018.00060. [Google Scholar] [CrossRef]
42. Yoo, S. Y., Badrinath, N., Woo, H. Y., Heo, J. (2017). Oncolytic virus-based immunotherapies for hepatocellular carcinoma. Mediators of Inflammation, 2017, 5198798. DOI 10.1155/2017/5198798. [Google Scholar] [CrossRef]
43. Jin, Q., Li, X., Cao, P. (2015). EphA2 modulates radiosensitive of hepatocellular carcinoma cells via p38/mitogen-activated protein kinase-mediated signal pathways. Kaohsiung Journal of Medical Sciences, 31(10), 510–517. DOI 10.1016/j.kjms.2015.09.001. [Google Scholar] [CrossRef]
44. Jin, Q., Li, X. J., Cao, P. G. (2016). MicroRNA-26b enhances the radiosensitivity of hepatocellular carcinoma cells by targeting EphA2. Tohoku Journal of Experimental Medicine, 238(2), 143–151. DOI 10.1620/tjem.238.143. [Google Scholar] [CrossRef]
45. Yoon, S., Choi, J. H., Kim, S. J., Lee, E. J., Shah, M. et al. (2019). EPHB6 mutation induces cell adhesion-mediated paclitaxel resistance via EPHA2 and CDH11 expression.Experimental and Molecular Medicine, 51(6), 1–12. DOI 10.1038/s12276-019-0261-z. [Google Scholar] [CrossRef]
46. Lu, C. Y., Yang, Z. X., Zhou, L., Huang, Z. Z., Zhang, H. T. et al. (2013). High levels of EphA3 expression are associated with high invasive capacity and poor overall survival in hepatocellular carcinoma. Oncology Report, 30(5), 2179–2186. DOI 10.3892/or.2013.2679. [Google Scholar] [CrossRef]
47. Bae, H. J., Song, J. H., Noh, J. H., Kim, J. K., Jung, K. H. et al. (2009). Low frequency mutation of the ephrin receptor A3 gene in hepatocellular carcinoma. Neoplasma, 56(4), 331–334. DOI 10.4149/neo_2009_04_331. [Google Scholar] [CrossRef]
48. Yan, Y., Luo, Y. C., Wan, H. Y., Wang, J., Zhang, P. P. et al. (2013). MicroRNA-10a is involved in the metastatic process by regulating Eph tyrosine kinase receptor A4-mediated epithelial-mesenchymal transition and adhesion in hepatoma cells. Hepatology, 57(2), 667–677. DOI 10.1002/hep.26071. [Google Scholar] [CrossRef]
49. Wang, Y., Liu, Z., Yao, B., Dou, C., Xu, M. et al. (2016). Long non-coding RNA TUSC7 acts a molecular sponge for miR-10a and suppresses EMT in hepatocellular carcinoma. Tumor Biology, 37(8), 11429–11441. DOI 10.1007/s13277-016-4892-6. [Google Scholar] [CrossRef]
50. Bourgin, C., Murai, K. K., Richter, M., Pasquale, E. B. (2007). The EphA4 receptor regulates dendritic spine remodeling by affecting beta1-integrin signaling pathways. Journal of Cell Biology, 178(8), 1295–1307. DOI 10.1083/jcb.200610139. [Google Scholar] [CrossRef]
51. Barkan, D., Chambers, A. F. (2011). Beta1-integrin: A potential therapeutic target in the battle against cancer recurrence. Clinical Cancer Research, 17(23), 7219–7223. DOI 10.1158/1078-0432.CCR-11-0642. [Google Scholar] [CrossRef]
52. Wang, X., Zhang, M., Ping, F., Liu, H., Sun, J. et al. (2019). Identification and therapeutic intervention of coactivated anaplastic lymphoma kinase, fibroblast growth factor receptor 2, and ephrin type-A receptor 5 kinases in hepatocellular carcinoma. Hepatology, 69(2), 573–586. DOI 10.1002/hep.29792. [Google Scholar] [CrossRef]
53. Nouri-Vaskeh, M., Alizadeh, L., Hajiasgharzadeh, K., Mokhtarzadeh, A., Halimi, M. et al. (2020). The role of HSP90 molecular chaperones in hepatocellular carcinoma. Journal of Cellular Physiology, 235(12), 9110–9120. DOI 10.1002/jcp.29776. [Google Scholar] [CrossRef]
54. Hafner, C., Schmitz, G., Meyer, S., Bataille, F., Hau, P. et al. (2004). Differential gene expression of Eph receptors and ephrins in benign human tissues and cancers. Clinical Chemistry, 50(3), 490–499. DOI 10.1373/clinchem.2003.026849. [Google Scholar] [CrossRef]
55. Wang, L. F., Fokas, E., Juricko, J., You, A., Rose, F. et al. (2008). Increased expression of EphA7 correlates with adverse outcome in primary and recurrent glioblastoma multiforme patients. BMC Cancer, 8, 79. DOI 10.1186/1471-2407-8-79. [Google Scholar] [CrossRef]
56. Kim, S. K., Jeon, J. W., Park, J. J., Cha, J. M., Joo, K. R. et al. (2011). Associations of EPHB1 polymorphisms with hepatocellular carcinoma in the Korean population. Human Immunology, 72(10), 916–920. DOI 10.1016/j.humimm.2011.06.014. [Google Scholar] [CrossRef]
57. Butler, N. S., Schmidt, N. W. (2015). Erythropoietin-producing hepatocellular receptor B2 receptor tyrosine kinase: A novel regulator of infection- and inflammation-induced liver fibrosis. Hepatology, 62(3), 680–683. DOI 10.1002/hep.27868. [Google Scholar] [CrossRef]
58. Leung, H. W., Leung, C. O. N., Lau, E. Y., Chung, K. P. S., Mok, E. H. et al. (2021). EPHB2 activates beta-catenin to enhance cancer stem cell properties and drive sorafenib resistance in hepatocellular carcinoma. Cancer Research, 81(12), 3229–3240. DOI 10.1158/0008-5472.CAN-21-0184. [Google Scholar] [CrossRef]
59. Wada, H., Yamamoto, H., Kim, C., Uemura, M., Akita, H. et al. (2014). Association between ephrin-A1 mRNA expression and poor prognosis after hepatectomy to treat hepatocellular carcinoma. International Journal of Clinical Oncology, 45(3), 1051–1058. DOI 10.3892/ijo.2014.2519. [Google Scholar] [CrossRef]
60. Feng, Y. X., Zhao, J. S., Li, J. J., Wang, T., Cheng, S. Q. et al. (2010). Liver cancer: EphrinA2 promotes tumorigenicity through Rac1/Akt/NF-kappaB signaling pathway. Hepatology, 51(2), 535–544. DOI 10.1002/hep.23313. [Google Scholar] [CrossRef]
61. Wilson, G. K., Tennant, D. A., McKeating, J. A. (2014). Hypoxia inducible factors hypoxia inducible factors in liver disease and hepatocellular carcinoma: Current understanding and future directions. Journal of Hepatology, 61(6), 1397–1406. DOI 10.1016/j.jhep.2014.08.025. [Google Scholar] [CrossRef]
62. Yu, M., Chen, S., Hong, W., Gu, Y., Huang, B. et al. (2019). Prognostic role of glycolysis for cancer outcome: Evidence from 86 studies. Journal of Cancer Research and Clinical Oncology, 145(4), 967–999. DOI 10.1007/s00432-019-02847-w. [Google Scholar] [CrossRef]
63. Zheng, S. S., Chen, X. H., Yin, X., Zhang, B. H. (2013). Prognostic significance of HIF-1α expression in hepatocellular carcinoma: A meta-analysis. PLoS One, 8(6), e65753. DOI 10.1371/journal.pone.0065753. [Google Scholar] [CrossRef]
64. Bao, M. H., Wong, C. C. (2021). Hypoxia, metabolic reprogramming, and drug resistance in liver cancer. Cells, 10(7), 1715. DOI 10.3390/cells10071715. [Google Scholar] [CrossRef]
65. Lin, J., Zeng, C., Zhang, J., Song, Z., Qi, N. et al. (2021). EFNA4 promotes cell proliferation and tumor metastasis in hepatocellular carcinoma through a PIK3R2/GSK3beta/beta-catenin positive feedback loop. Molecular Therapy-Nucleic Acids, 25, 328–341. DOI 10.1016/j.omtn.2021.06.002. [Google Scholar] [CrossRef]
66. Wang, T. H., Ng, K. F., Yeh, T. S., Wang, Y. L., Liang, K. H. et al. (2012). Peritumoral small ephrinA5 isoform level predicts the postoperative survival in hepatocellular carcinoma. PLoS One, 7(7), e41749. DOI 10.1371/journal.pone.0041749. [Google Scholar] [CrossRef]
67. Sawai, Y., Tamura, S., Fukui, K., Ito, N., Imanaka, K. et al. (2003). Expression of ephrin-B1 in hepatocellular carcinoma: Possible involvement in neovascularization. Journal of Hepatology, 39(6), 991–996. DOI 10.1016/s0168-8278(03)00498-7. [Google Scholar] [CrossRef]
68. Llovet, J. M., Ricci, S., Mazzaferro, V., Hilgard, P., Gane, E. et al. (2008). Sorafenib in advanced hepatocellular carcinoma. New England Journal of Medicine, 359(4), 378–390. DOI 10.1056/NEJMoa0708857. [Google Scholar] [CrossRef]
69. Cheng, A. L., Kang, Y. K., Chen, Z., Tsao, C. J., Qin, S. et al. (2009). Efficacy and safety of sorafenib in patients in the Asia-Pacific region with advanced hepatocellular carcinoma: A phase III randomised, double-blind, placebo-controlled trial. Lancet Oncology, 10(1), 25–34. DOI 10.1016/S1470-2045(08)70285-7. [Google Scholar] [CrossRef]
70. Bruix, J., Qin, S., Merle, P., Granito, A., Huang, Y. H. et al. (2017). Regorafenib for patients with hepatocellular carcinoma who progressed on sorafenib treatment (RESORCEA randomised, double-blind, placebo-controlled, phase 3 trial. Lancet, 389(10064), 56–66. DOI 10.1016/S0140-6736(16)32453-9. [Google Scholar] [CrossRef]
71. Berasain, C. (2013). Hepatocellular carcinoma and sorafenib: Too many resistance mechanisms? Gut, 62(12), 1674–1675. DOI 10.1136/gutjnl-2013-304564. [Google Scholar] [CrossRef]
72. Chen, R., Li, Q., Xu, S., Ye, C., Tian, T. et al. (2022). Modulation of the tumour microenvironment in hepatocellular carcinoma by tyrosine kinase inhibitors: From modulation to combination therapy targeting the microenvironment. Cancer Cell International, 22(1), 73. DOI 10.1186/s12935-021-02435-4. [Google Scholar] [CrossRef]
73. Saha, N., Robev, D., Mason, E. O., Himanen, J. P., Nikolov, D. B. (2018). Therapeutic potential of targeting the Eph/ephrin signaling complex. The International Journal of Biochemistry and Cell Biology, 105, 123–133. DOI 10.1016/j.biocel.2018.10.006. [Google Scholar] [CrossRef]
74. El-Khoueiry, A., Gitlitz, B., Cole, S., Tsao-Wei, D., Goldkorn, A. et al. (2016). A first-in-human phase I study of sEphB4-HSA in patients with advanced solid tumors with expansion at the maximum tolerated dose (MTD) or recommended phase II dose (RP2D). European Journal of Cancer, 69, S11. DOI 10.1016/S0959-8049(16)32623-5. [Google Scholar] [CrossRef]
75. Shitara, K., Satoh, T., Iwasa, S., Yamaguchi, K., Muro, K. et al. (2019). Safety, tolerability, pharmacokinetics, and pharmacodynamics of the afucosylated, humanized anti-EPHA2 antibody DS-8895a: A first-in-human phase I dose escalation and dose expansion study in patients with advanced solid tumors. Journal for Immuno Therapy of Cancer, 7(1), 219. DOI 10.1186/s40425-019-0679-9. [Google Scholar] [CrossRef]
76. Gan, H. K., Parakh, S., Lee, F. T., Tebbutt, N. C., Ameratunga, M. et al. (2022). A phase 1 safety and bioimaging trial of antibody DS-8895a against EphA2 in patients with advanced or metastatic EphA2 positive cancers. Investigational New Drugs, 40(4), 747–755. DOI 10.1007/s10637-022-01237-3. [Google Scholar] [CrossRef]
77. Annunziata, C. M., Kohn, E. C., LoRusso, P., Houston, N. D., Coleman, R. L. et al. (2013). Phase 1, open-label study of MEDI-547 in patients with relapsed or refractory solid tumors. Investigational New Drugs, 31(1), 77–84. DOI 10.1007/s10637-012-9801-2. [Google Scholar] [CrossRef]
78. Fraguas-Sanchez, A. I., Lozza, I., Torres-Suarez, A. I. (2022). Actively targeted nanomedicines in breast cancer: From pre-clinal investigation to clinic. Cancers, 14(5), 1198. DOI 10.3390/cancers14051198. [Google Scholar] [CrossRef]
79. Bennett, G., Brown, A., Mudd, G., Huxley, P., van Rietschoten, K. et al. (2020). MMAE delivery using the bicycle toxin conjugate BT5528. Molecular Cancer Therapeutics, 19(7), 1385–1394. DOI 10.1158/1535-7163.MCT-19-1092. [Google Scholar] [CrossRef]
80. Swords, R. T., Greenberg, P. L., Wei, A. H., Durrant, S., Advani, A. S. et al. (2016). KB004, a first in class monoclonal antibody targeting the receptor tyrosine kinase EphA3, in patients with advanced hematologic malignancies: Results from a phase 1 study. Leukemia Research, 50, 123–131. DOI 10.1016/j.leukres.2016.09.012. [Google Scholar] [CrossRef]
81. Garrido-Laguna, I., Krop, I., Burris 3rd, H., Hamilton, E., Braiteh, F. et al. (2019). First-in-human, phase I study of PF-06647263, an anti-EFNA4 calicheamicin antibody-drug conjugate, in patients with advanced solid tumors. International Journal of Cancer, 145(7), 1798–1808. DOI 10.1002/ijc.32154. [Google Scholar] [CrossRef]
82. Yamaguchi, S., Tatsumi, T., Takehara, T., Sasakawa, A., Yamamoto, M. et al. (2010). EphA2-derived peptide vaccine with amphiphilic poly (gamma-glutamic acid) nanoparticles elicits an anti-tumor effect against mouse liver tumor. Cancer Immunology Immunotherapy, 59(5), 759–767. DOI 10.1007/s00262-009-0796-2. [Google Scholar] [CrossRef]
83. Amato, K. R., Wang, S., Hastings, A. K., Youngblood, V. M., Santapuram, P. R. et al. (2014). Genetic and pharmacologic inhibition of EPHA2 promotes apoptosis in NSCLC. The Journal of Clinical Investigation, 124(5), 2037–2049. DOI 10.1172/JCI72522. [Google Scholar] [CrossRef]
84. Amato, K. R., Wang, S., Tan, L., Hastings, A. K., Song, W. et al. (2016). EPHA2 blockade overcomes acquired resistance to EGFR kinase inhibitors in lung cancer. Cancer Research, 76(2), 305–318. DOI 10.1158/0008-5472.CAN-15-0717. [Google Scholar] [CrossRef]
85. Martini, G., Cardone, C., Vitiello, P. P., Belli, V., Napolitano, S. et al. (2019). EPHA2 is a predictive biomarker of resistance and a potential therapeutic target for improving antiepidermal growth factor receptor therapy in colorectal cancer. Molecular Cancer Therapeutics, 18(4), 845–855. DOI 10.1158/1535-7163.MCT-18-0539. [Google Scholar] [CrossRef]
86. Pan, X. Y., You, H. M., Wang, L., Bi, Y. H., Yang, Y. et al. (2019). Methylation of RCAN1.4 mediated by DNMT1 and DNMT3b enhances hepatic stellate cell activation and liver fibrogenesis through Calcineurin/NFAT3 signaling. Theranostics, 9(15), 4308–4323. DOI 10.7150/thno.32710. [Google Scholar] [CrossRef]
Cite This Article
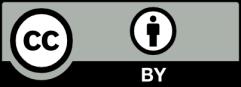