Open Access
ARTICLE
Widely Untargeted Metabolome Profiling Provides Insight into Browning and Nutritional Quality Changes in Short-Term Stored Fresh-Cut Potato (Solanum tuberosum L.) Shreds
Fujian Key Laboratory of subtropical Plant Physiology and Biochemistry, Fujian Institute of Subtropical Botany, Xiamen, 361006, China
* Corresponding Author: Wenhua Wang. Email:
Phyton-International Journal of Experimental Botany 2023, 92(10), 2785-2805. https://doi.org/10.32604/phyton.2023.030928
Received 04 May 2023; Accepted 16 June 2023; Issue published 15 September 2023
Abstract
Potato (Solanum tuberosum L.) is susceptible to enzymatic browning after fresh processing, resulting in color change and potential alteration in the nutritional quality. In this study, a popular potato cultivar, Feiwuruita, was used to profile the metabolites involved in color and nutritional quality changes in fresh shreds stored at 0 and 4 h at 25°C (designated CK and CK4H, respectively). The shreds turned brown within 4 h of storage. In all, 723 metabolites consisting 12 classes of compounds were detected in the samples, largely lipids, phenolic acids, alkaloids, amino acids and derivatives, flavonoids, organic acids, nucleotides and derivatives. Of these, 163 metabolites accumulated differentially between CK and CK4H shreds. Polyphenolic compounds (phenolic acids and flavonoids) mostly increased in the shreds after 4 h storage. Conversely, the short-term storage drastically reduced lipid compounds (25 LysoPC and 19 LysoPE), while essential alkaloids and terpenoid compounds that are beneficial to human health increased in accumulation. The findings present global metabolome and nutritional composition changes in short-term stored shreds of Feiwuruita. This study provides important foundation for future studies on browning prevention/reduction and for better utilization of Feiwuruita.Keywords
Supplementary Material
Supplementary Material FilePotato (Solanum tuberosum L.) is a starchy root vegetable that is one of the most widely consumed foods in the world after wheat, rice and maize [1]. It is a good source of dietary fiber, carbohydrates, carotenes, anthocyanins and micronutrients (e.g., vitamin B6 and C, potassium, iron, folate, thiamin, riboflavin and niacin) [2–4]. It can be cooked in a variety of dishes such as boiling, baking, frying and roasting in different dishes, such as mashed potatoes, potato bread, French fries, potato chips and potato salad [5,6]. Others are processed into potato powder and starch for industrial use [7]. Robertson et al. [2] reported a number of epidemiological studies showing associations between potato consumption and obesity, type 2 diabetes and cardiovascular disease. Potato is an important staple crop in developing countries because of its relatively short production cycle (90–110 days) and high yield per acre [8].
Globally, China is the leading potato producer in terms of volume and area with almost half of its production concentrated in the northern part [1,9]. The potential of potatoes to improve food security and alleviate poverty, as well as their affordability and nutritional value, led the Chinese government to issue the “Guiding Opinions on Promoting the Development of Potato Industry” in 2016, and many provinces and cities have implemented related policies to benefit from potato production and demand [9]. As a result, China has more than 200 kinds of potato staple food products, several of which are currently being commercialized [7]. The major potato starch processing areas in China are located in the northwest, northeast (Heilongjiany, Jilin, and Liaoning), North China, and southwest and northwest (Gansu, Ningxia, Qinghai, Xinjing and Shaanxi) [7,10].
Food preservation consists of various processing steps, including growing, harvesting, processing and distribution, to maintain food quality at a desired level so that maximum benefit and nutritional value can be obtained [11]. Storage of potato tubers has been reported to cause large variations in dry matter content, carbohydrates, proteins, and amino acids [12,13]. Storage conditions and cultivar characteristics of potatoes also have a major influence on the content and structure of nitrogen compounds [14–16]. These changes affect both the consumers and processors through the formation of free amino acids, including glutamic and aspartic acid, serine, valine, proline and glutamine which ultimately affect the taste of the tubers [13]. These also affect the quality characteristics of food products derived from such tubers [17].
Potatoes are susceptible to enzymatic browning during and after fresh processing, which is an important quality issue [18–20]. To date, many anti-browning compounds such as ascorbic acid and its derivatives, xanthosine, thiol and others have been tested on fresh cut potatoes with inconsistent results [18,21–24]. Enzymatic browning is reported to be caused by polyphenol oxidases (PPOs), β-glucosidase (β-GLU), phenylalanine ammonia-lyase (PAL), and peroxidase (POD), which catalyze the conversion of phenolic substrates to quinones, resulting in the formation of dark-colored precipitates in fruits and vegetables. This leads to undesirable changes in the organoleptic properties and change nutritional quality of plant products [19,25]. It has been documented that sulfites are the most effective in preventing browning [24], but there are food safety concerns regarding their use [26,27]. Browning of the fruit/vegetable can easily occur during fresh-cut processing [28]. Once a physical stress or deteriorative process such as a wound response or senescence is initiated, browning symptoms begin to appear in fresh-cut potato tubers when polyphenol substrates react with oxidative enzymes (e.g., PPOs, PAL and POD) [29].
With respect to browning in plant products, Qiao et al. [30] identified metabolites associated with signal transduction (jasmonic acid, glutamate and gibberellins) enriched in a browning-resistant potato cultivar (Kexin (KX13)) and browning susceptible cultivar (Yunshu (YS505)), while L-aspartic acid and kynurenic acid were identified to slow browning. A number of metabolites, including chlorogenic acid, coumaroyl tartaric acid and dicaffeoyl quinic acid are reported to contribute to enzymatic browning in fresh-cut fruits and vegetables [31], and subsequent accumulation of phenolic compounds [25,28,32]. Most of the previous studies in potato were conducted among different cultivars [22,30,33], which have contributed to the selection of potato cultivars to be processed as fresh-cut products. However, findings from the previous studies are largely cultivar-dependent making them extremely difficult to apply to fresh-cut potato management. Therefore, to eliminate the cultivar-dependent factor, the present study used Feiwuruita cultivar which is one of the popular cultivars in Jilin Province and other places in China. This cultivar is popular because of its good agronomic characteristics and high commercial rate but it is highly susceptible to browning [34]. Understanding the metabolome changes in Feiwuruita cv could help major potato growing and processing provinces in China to benefit fully from this cultivar.
In addition to the above, an essential fatty acid, linolenic acid, and some essential vitamins such as vitamin E (α- and γ-tocopherol), vitamin B5 (pantothenic acid) and vitamin B6 (pyridoxal and pyridoxine) were detected in the study of Chaparro et al. [35]. Storage of potatoes decreased the concentration of vitamin C in potato tubers, as reported by Burgos et al. [36]. Inconsistent variations in chlorogenic acid in tubers of purple- or red-fleshed potato cultivars compared to yellow-fleshed tubers have been observed in fresh and cold stored tubers [37]. These studies highlight that nutritional quality changes occur during postharvest management and processing of potato tubers. To the best of our knowledge, no research has been reported on the change in nutritional quality of freshly cut and stored potato shreds.
Herein, we investigated the color and nutritional quality changes in the fresh-cut potato shreds stored shortly and profiled the metabolome changes in fresh-cut potato shreds (CK) stored for 4 h (CK4H) by ultra-performance liquid chromatography-tandem mass spectrometry (UPLC-MS/MS). The results of this study offer a theoretical basis and practical guidance for the inhibition of browning in fresh-cut and stored potatoes. This information would also serve as a reference point when evaluating the formation of various compounds during the processing of potato products [38].
2.1 Experimental Materials and Shred Preparation
In this study, well-developed, healthy and fresh potato (S. tuberosum L.) tubers of Feiwuruita cv were used which were obtained from the Jilin Academy of Agricultural Sciences [34]. The potato tubers were washed with clean running water and the washed tubers were further cleaned with tissue paper to dry them without delay. The washed and dried potato tubers were peeled and sliced to a thickness of 2 mm using an electric slicer MS-305 C (Foshan Komle Electric Appliance Co., Ltd., Foshan, China). The freshly cut potato shreds with uniform size and shape were stored for 4 h at room temperature (25°C). The samples were designated as CK before storage and CK4H after storage. Samples of CK and CK4H were immediately labeled and placed in a liquid nitrogen container and then stored in a −80°C ultra-low temperature refrigerator until further processing.
2.2 Measurement of Color Changes in Fresh Cut Potato Shreds Stored for 0 and 4 h
The colors of shreds of CK and CK4H were determined according to the colorimetric values proposed by the Commission Internationale de l’Eclairage with colorimeter (SC-10; Shenzhen 3 nh technology Co., Ltd., Shenzhen, China). Color coordinates are L (lightness), a (redness/greenness) and b (yellowness/blueness). For each group (either CK or CK4H), the collection of color parameters was repeated three times (biological replicates). The color difference (ΔE) [39,40] between the CK and CK4H was computed by the formula below:
where
2.3 Metabolome Profiling in Shreds of Fresh Cut Potato Stored for 0 and 4 h by Ultra-Performance Liquid Chromatography-Tandem Mass Spectrometry
Six samples (CK and CK4H with three biological repeats each, weighing ≈ 3 g) were ground to powder after vacuum freeze-drying using a mixer mill MM400 and zirconia beads (15 mm) for 1.5 min at 30 Hz. Subsequently, 100 mg of sample powder from each of the six samples was extracted overnight at 4°C in 1.0 mL 70% aqueous methanol and centrifuged at 10,000 × g for 10 min. The extracts were further filtered and transferred to a new tube for UPLC-MS/MS analysis following Wen et al. [41]. In short, MetWare Biotechnology Co. Ltd. (Wuhan, China) was contracted to conduct and perform widely untargeted metabolites profiling with its self-built database.
To check the repeated analyses, a control analysis was performed by mixing sample extracts from six samples. Metabolites were qualitatively monitored using secondary spectral information by qualitative means. The metabolites were further quantified by analysis in a multi-reaction monitoring mode using a triple quadruple (QQQ) mass spectrometer, an AB4500 Q TRAP UPLC/MS/MS system, equipped with an ESI Turbo Ion Spray interface. The system was operated in both positive and negative ion modes. It was controlled by Analyst 1.6.3 software (AB Sciex). ESI source operating parameters were set: ion source, Turbo Spray; source temperature 550°C; ion spray voltage (IS) 5500 V (positive ion mode)/4500 V (negative ion mode); ion source gas I, gas II, curtain gas set to 50, 60, 25.0 psi, respectively. Collision-activated dissociation was set to high. QQQ and LIT modes were used for instrument tuning and mass calibration in 10 and 100 μmol/L polypropylene glycol solutions, respectively. QQQ scans were performed as multiple reaction monitoring (MRM) experiments. The collision gas (nitrogen) was set to medium. The declustering potential (DP) and collision energy (CE) for individual MRM transitions were determined. Further DP and CE optimization was performed. A specific set of MRM transitions was checked for each time period. These transitions corresponded to the metabolites eluted during that time period.
2.4 Statistical Analyses of Colorimetric Values, Polyphenol Activity and Metabolites in the Shreds of Fresh Cut Potato Stored for 0 and 4 h
A two-tailed t-test at 5% significance level was performed on colorimetric parameters (L, a, and b values) obtained in three biological replicates using Microsoft Excel (Microsoft Corporation, 2018; Redmond, Washington, United States). To evaluate the quality of metabolome data, we applied principal component analysis (PCA) with tidyverse [42] in R, while hierarchical clustering heatmap (HCH), and Pearson’s correlation analysis were done with the help of pheatmap [43] and corrplot [44] in R, respectively. These analyses were done based on relative concentration (ion intensities) of the detected metabolites. We adopted stringent filtering criteria with orthogonal partial least squares discriminant analysis (OPLS-DA) using a threshold of log2 fold change ≥1 i.e., |log2FC ≥ 1| and variable importance in projection (VIP) ≥1 [45] to identify the differentially accumulated metabolites (DAMs) between CK and CK4H shreds. The qualitative and quantitative mass spectrometry analysis of metabolites between shreds between CK and CK4H shreds were performed based on the Kyoto Encyclopedia of Genes and Genomes (KEGG) database [46], MetWare database (MWDB) and MRM [47]. The KEGG database was used to annotate DAMs with p-value ≤ 0.05, which were considered as significantly enriched pathways. Furthermore, heatmaps of selected DAMs were generated in R using the pheatmap package [43].
3.1 Browning Degree in Fresh Cut Potato and Activity of Polyphenol Oxidases
In the current study, freshly cut potato shreds stored for 0 and 4 h (CK and CK4H, respectively) were used to assess the color changes (Fig. 1). It was observed that the samples turned brown after 4 h (Fig. 1A). The L* (lightness) values of CK shreds (mean ± standard error; 38.74 ± 0.19) were significantly higher than those of CK4H (24.26 ± 0.27) (Fig. 1B), indicating that CK4H samples were darker after 4 h storage. Again, the a* (redness/greenness) was lower in the shreds of CK (1.45.26 ± 0.01) compared with CK4H (6.05 ± 0.10) (Fig. 1B), indicating that there was a large increase in redness/greenness of the shreds after 4 h storage. The shreds of CK recorded b* (yellowness/blueness) value of 11.00 ± 0.44 compared with the shreds of CK4H (10.81 ± 0.06), highlighting that the shreds of CK maintained relatively higher levels of yellowness/blueness, but showed a marginal decrease after 4 h storage (Fig. 1B). The ΔE value (6.18 ± 0.26 10) indicates that shreds of CK4H had much browner color compared to shreds of CK (Fig. 1B).
Figure 1: Color changes in the freshly cut potato tubers. (A) Trays containing shreds obtained from fresh cut potato stored at 0 and 4 h (CK and CK4H, respectively). (B) Colorimeter values obtained from shreds of the CK and CK4H. Color coordinates L* (lightness), a* (redness/greenness), b* (yellowness/blueness) and the color difference (ΔE*). The error bars were computed as standard error from three biological repeats. Indices with * between the two samples indicate significant difference by student-t test at p < 0.05
3.2 Overview of Metabolome Profiling in CK and CK4H Samples
Metabolomic analysis was performed to characterize the metabolite changes in the shreds of CK and CK4H (Figs. 1A and B). A total of 723 metabolites from 12 classes of compounds were detected by UPLC-MS/MS in the six samples (Fig. 2). The majority of compounds were lipids (16.74%), phenolic acids (14.66%), alkaloids (13.42%), amino acids and derivatives (12.45%), and flavonoids (9.13%) (Fig. 2A; Table S1). The remaining seven known classes include organic acids (8.44%), nucleotides and derivatives (7.05%), terpenoids (2.77%), lignans and coumarins (2.21%), tannins (0.69%), quinones (0.55%), and steroids (0.14%) (Fig. 2A; Table S1). Other compounds constituted 11.76% with saccharides and alcohols, vitamins, xanthone and several unknown classes of compounds (other) (Fig. 2A; Table S1).
Figure 2: Metabolome profiling in fresh cut potato shreds stored for 0 and 4 h at room temperature (CK and CK4H, respectively). (A) Proportion of the 723 metabolites belonging to 11 class of compounds. (B) Sum of relative ion intensities of compounds in each class in CK and CK4H
The sum of all compounds within each class varied between CK and CK4H (Fig. 2A). Specifically, the accumulation of alkaloids, lipids, flavonoids, terpenoids, tannins, and steroids decreased upon 4 h storage (CK4H), whereas the accumulation of phenolic acids, amino acids and derivatives, organic acids, nucleotides and derivatives, lignans and coumarins, quinones, and others increased upon storage (Fig. 2B). These suggest that storage of freshly cut potato shreds for 4 h at room temperature altered the metabolome.
PCA revealed the degree of metabolic variation between groups (CK and CK4H) and between samples within the same group. CK, CK4H and their combinations (mix samples) within the distribution of apparent dispersion showed that metabolite levels differed between groups (CK and CK4H) (Fig. 3). The mixed samples (CK + CK4H) were clearly separated and found in the middle of the plot. Taken together, the two principal component axes (PC1 and PC2) showed that the 723 metabolites accounted for 53.81% of the variability among the samples. HCH of the samples based on the ion intensities of the 723 metabolites detected showed that the three biological replicates from each sample were grouped together (Fig. 3). There was a high level of reproducibility between biological replicates with Pearson’s correlation coefficients ≥0.87 (Fig. S1). Therefore, the samples were sufficiently reproducible, suitable for use in the subsequent qualitative and quantitative analyses, and adequate to ensure the repeatability and reliability of the metabolomic data.
Figure 3: Variability of the 723 detected metabolites between fresh cut of potato shreds after 0 and 4 h storage (CK [deep green] and CK4H [brown], respectively) based on their relative ion intensities in triplicates. (A) Principal component analysis. (B) Hierarchical cluster heatmap. Metabolome profiling were done in triplicates for each sample (CK or CK4H). The Mix in the panel A was included as the control for quality check. Color gradients are shown on the right side of the figure, red, green and white colors mean increased, decreased and not detected, respectively
3.3 Detection of Metabolites with Differential Accumulation Between CK and CK4H Samples
To screen for DAMs between CK and CK4H (Table S1A), we applied stringent filtering criteria log2FC ≥ 1 and VIP ≥ 1. This resulted in 163 DAMs, which were grouped into two major clusters of metabolites with differential accumulation between CK and CK4H (Fig. 4A). Among them, only lipids had 91.84% compounds that accumulated more in CK than in CK4H (Fig. 4B), suggesting that storage led to a reduced content of lipid metabolites in potato shreds. On the contrary, alkaloids, amino acids and derivatives, flavonoids, lignans and coumarins, and terpenoids all accumulated more after 4 h storage of potato shreds (Fig. 4B). These results suggest that short-term storage (4 h) significantly alter the metabolomic composition and possibly nutritional quality of fresh cut potato shreds. Therefore, we focused on metabolites that may be involved in the color change of CK4H as well as those of nutritional importance.
Figure 4: Differentially accumulated metabolites (DAM) between fresh cut of potato stored for 0 h (CK) and 4 h (CK4H). (A) Hierarchical cluster heatmap. The row clustering was based on the relative ion intensities of the detected DAMs. Color gradients are shown on the right side of the figure, red, green and white colors mean increased, decreased and not detected, respectively. (B) Extent of accumulation of DAMs. Up-and down-accumulated mean accumulated higher in CK4H than in CK, and vice versa, respectively
3.3.1 Candidate Metabolites Involved in Browning of Shreds of CK4H
Polyphenols are known to be synthesized mainly through the phenylpropanoid pathway, which involves many different enzymes/metabolites involved in browning of fresh cut potatoes and other crops [48–52]. Therefore, the DAMs for polyphenols and other classes of compounds involved in browning were investigated in this section.
Changes in Polyphenols in the Fresh Cut Samples of Potato
Polyphenols (i.e., phenolic acids, flavonoids and their derivatives, tannins, stilbenes, and lignans) are reported to contribute to bitterness, astringency, color, flavor, odor, and oxidative stability in various crops and their related products [8,53]. Twenty-five polyphenolic compounds accumulated differentially between CK and CK4H, with higher accumulation in the shreds of CK4H than in CK (Fig. 5A). Of these, one flavonoid compound, isobavachalcone D, was completely absent in CK, while the remaining two flavonoids (gallocatechin and 8-methoxykaempferol-7-O-rhamnoside) accumulated 2.70 and 9.30 times higher in CK4H than in CK, respectively. Gallocatechin and its derivatives are reported to be brown in color under ambient conditions [54]. Similarly, three lignan and coumarin compounds (isofraxidin, esculetin and ayapin) accumulated 2.47–16.52 times more in CK4H than in CK.
Figure 5: Log 2 transformed ion intensities of differentially accumulated metabolites (DAMs) in fresh cut potato shred stored for 0 h (CK) and 4 h (CK4H). (A) Polyphenol compounds. (B) Amino acid and derivative compounds. (C) Nucleotide and derivative compounds. The color legend is shown on the right hand side in the Figure
In addition to flavonoids, lignans and coumarins, 19 phenolic acids differed significantly between CK and CK4H (Fig. 5A). Among them, syringic acid, 3-O-p-coumaroylshikimic acid, p-coumaroyl alcohol, protocatechualdehyde and 2,5-dihydroxybenzaldehyde were completely absent in CK compared to CK4H (Fig. 5A). The remaining 14 phenolic compounds, including cinnamic acid, vanillic acid, vanillin, 5-O-caffeoylshikimic acid, 5-O-p-coumaroylquinic acid, 3-O-p-coumaroylquinic acid, isoferulic acid, ferulic acid, 2-(formylamino)benzoic acid, and caffeic acid, were more abundant in the shreds of CK4H than in CK.
The above results suggest that the enzymatic browning observed in this study may be largely modulated by polyphenols.
Variations in Amino Acids and Nucleotides, and Their Derivatives in the Shreds of CK and CK4H
Some amino acids and their derivatives have been documented to affect both enzymatic and nonenzymatic browning in several plant products depending on their concentrations [13,55–57]. Eighteen compounds belonging to amino acids and derivatives accumulated differentially in CK_vs_CK4H (Fig. 5B). From these, reduced form of glutathione accumulated 2.62 times more in CK relative to CK4H (Fig. 5B). Glutathione according to Kuijpers et al. [58] controls browning by forming colorless addition products with o-quinones of 5-dicaffeoylquinic acids, the decreased accumulation of reduced form of glutathione in CK4H may be involved in browning process (Fig. 5B). Other two compounds (L-Prolyl-L-phenylalanine and L-prolyl-L-leucine) were absent in CK4H, suggesting that these compounds may make CK4H more prone to browning compared to CK.
Among the remaining 15 amino acids and derivatives, only L-valyl-L-phenylalanine was completely absent in CK, while the 14 including L-isoleucyl-L-aspartate, L-glycyl-L-phenylalanine, L-valyl-L-leucine, L-glycyl-L-isoleucine, N-glycyl-L-leucine, N-acetyl-L-tryptophan, and S-adenosyl-L-methionine accumulated more in CK4H than in CK (Fig. 5B).
Twenty-five differentially accumulated nucleotide and derivative compounds were identified between CK and CK4H (Fig. 5C). With the exception of cytidine 5’-monophosphate, cytidylic acid, methotrexate and 2’-deoxycytidine, which were not detected in CK, the remaining 22 compounds accumulated more in CK4H than in CK (Fig. 5C).
The higher accumulation of several amino acids and derivatives as well as nucleotides and derivatives suggest their involvement in modulating the browning of shreds from CK4H (Fig. 1B).
Comparative Analysis of Organic Acids and Others (Vitamins, Saccharides and Alcohols) in the Potato Shreds of CK and CK4H
Most organic acids are shown to exhibit anti-browning properties due to their metal-chelating activities or pH lowering effects and are able to deactivate the PPO and POD enzymes [59,60]. Twelve organic acids were more accumulated in the shreds of CK4H compared with CK (Table 1). These include (-)-jasmonoyl-L-isoleucine, 2-hydroxyisocaproic acid, fumaric acid, citraconic acid and 2-hydroxycinnamic acid. For example, two jasmonates (jasmonic acid and N-((-)-jasmonoyl)-S-isoleucine) are reported to contribute to pericarp browning of litchi fruit [61].
Two saccharides and alcohols (N-acetyl-D-glucosamine-1-phosphate and D-glucurono-6,3-lactone) accumulated more in CK than in CK4H (Table 1). Two other saccharides and alcohols (xylitol and 2,6-dimethyl-7-octene-2,3,6-triol) accumulated more in CK4H than in CK. In addition, five vitamins: L-ascorbic acid (vitamin C), 4-pyridoxic acid, riboflavin (vitamin B2), dehydroascorbic acid and pyridoxine differed significantly between CK and CK4H. Of these, only L-ascorbic acid (vitamin C) accumulated more than 2-fold higher in CK than in CK4H, the remaining four accumulated higher in CK4H than in CK (Table 1). Three other unknown compounds (N-feruloyloctopamine, P-hydroxycinnamic acid, p-hydroxyphenethylamine, and eucommiol) accumulated highly in CK4H, while another, propyl 2-(trimethylammonio)ethyl phosphate, accumulated highly in CK.
The variations in organic acids and others (vitamins, saccharides, and alcohols) highlight their involvement in regulating browning in CK4H from CK (Figs. 1A and 1B).
3.3.2 Alteration in Nutritional Composition by Short term Storage (4 h) of Fresh Cut Potato Shreds
Variations in Lipid Compounds Content between CK and CK4H Samples
Lipid compounds dominated among metabolites in the study of Chaparro et al. [35]. In this study, 49 lipid compounds consisting of four lipid classes: 25 lysophosphatidylcholine (LysoPC), 19 lysophosphatidylethanolamine (LysoPE), four free fatty acids, and one glycerol ester accumulated differentially between CK and CK4H (Table S2). The 19 LysoPE and their isomers all accumulated higher in the CK than in the CK4H samples. On the other hand, only LysoPC 19:2 increased in accumulation upon the 4 h storage, the remaining 24 LysoPC isomers decreased in accumulation upon 4 h storage (Table S2).
In addition, one saturated fatty acid, stearic acid, and one monounsaturated fatty acid, eicosenoic acid, were found to be more abundant in CK than in CK4H (Table S2). Strikingly, two free fatty acid compounds, 1-linolenoyl-rac-glycerol diglucoside and eicosadienoic acid, as well as a glycerol ester, 2-linolenoylglycerol-1,3-di-O-glucoside, increased in abundance in CK4H compared to CK (Table S2). Taken together, a drastic reduction of lipid compounds in CK4H compared to CK would be beneficial for human health.
Changes in Alkaloid and Terpenoid Compounds after Short Term Storage (4 h) of Fresh Cut Potato Shred
Alkaloids have been reported as having anti-inflammatory, anticancer, analgesic, local anesthesia and analgesia, neuropharmacological, antimicrobial, antifungal, and other activities [62–64]. Therefore, alkaloids are routinely used as dietary ingredients, dietary supplements, and pharmaceuticals, in medicine and other uses in human life. Eighteen alkaloid compounds accumulated differentially in the shreds of CK and CK4H (Table S2). Fifteen alkaloid compounds, including 9α-hydroxysophoramine, tataramide A, phenylethanolamine, 4-hydroxy-2-benzoxazolone (HBOA), and N-feruloyltyramine (Table S2), increased in accumulation upon short-term (4 h) storage of potato shreds. These compounds have been highlighted to have human health benefits [63,65–68], making short-term storage (4 h) of shreds useful for human health.
Terpenoids have a wide range of documented biological properties, including cancer chemoprevention, antimicrobial, antifungal, antiviral, antihyperglycemic, anti-inflammatory, antiparasitic, and memory enhancing [65–67]. Among the 20 terpenoid compounds detected (Table S2), hispanolone, pimaric acid and eucommioside are reported to have anti-inflammatory and anti-tumor activities [66]. These compounds increased highly in CK4H shreds compared to CK shreds (Table S2). Therefore, changes in alkaloids and terpenoids may make short-term storage of potato shreds beneficial for human health, however further validation experiment is needed to ascertain this claim.
3.4 KEGG Pathway Enrichment Analysis of Differentially Accumulated Metabolites between the Two Tubers of Potato
To identify significantly enriched metabolic pathways among the 163 DAMs, we further used the Kyoto Encyclopedia of Genes and Genomes (KEGG) database [46]. Among the top 20 KEGG pathways, phenylpropanoid biosynthesis, pyrimidine metabolism, purine metabolism, and zeatin biosynthesis were significantly (p ≤ 0.05) enriched (Fig. 6), with seven, eight, eight, and five compounds, respectively. The significantly enriched phenylpropanoid biosynthetic pathway compounds consisted of one organic acid (2-hydroxycinnamic acid) and six phenols (cinnamic acid, p-coumaryl alcohol, 5-O-caffeoylshikimic acid, 5-O-p-coumaroylquinic acid, ferulic acid, and caffeic acid), all of which were up-accumulated in CK4H relative to CK.
Figure 6: Top twenty KEGG pathways enriched in DAMs between the CK and CK4H of fresh tubers of potato. The X-axis represents the enrichment score. The Y-axis represents the top 20 KEGG pathways enriched in DAMs with those underlined being significant at p-value ≤ 0.05. The X-axis represents enrichment factor (rich factor), thus the ratio of the number of DEGs to the number of total annotated genes in a certain pathway. The size of the bubble indicates the number of DAMs enriched in the pathway, and the color of the bubble indicates the magnitude of the p-value
Compounds of the pyrimidine pathway included cytidine 5’-monophosphate (cytidylic acid), thymidine, 2’-deoxycytidine, uridine 5’-monophosphate, cytosine, uridine, cytidine, and 2-deoxyribose-1-phosphate. Whereas in the case of purine pathway was enriched in hypoxanthine, guanosine 5’-monophosphate, adenosine 5’-diphosphate, 2’-deoxyguanosine, xanthosine, guanine, adenine and guanosine. All enriched compounds in the pyrimidine and purine pathways accumulated more in CK4H than in CK.
The zeatin biosynthetic pathway had two amino acids and derivatives (O-acetylserine and S-adenosyl-L-methionine) and three nucleotides and derivatives (adenosine 5’-diphosphate, adenine, and 5’-deoxy-5’-(methylthio)adenosine) accumulated more in CK4H than in CK. The results of KEGG analysis suggest a complex nature of browning in potato tuber shreds.
Based on the above results, we propose a model for browning and nutritional quality changes in shreds obtained from fresh cut potatoes (Fig. 7). Shreds obtained from clean and healthy fresh potato tubers contain high lipid content. Wounds and short-term storage (4 h) at room temperature (25°C) resulted in an increase in PPO activity as well as polyphenols, organic acids and nucleotides and their derivatives, leading to browning of the stored shreds. However, the short-stored shreds had a drastic reduction in lipid content and an increase in beneficial alkaloids (9α-hydroxysophoramine, tataramide A, phenylethanolamine, HBOA, and N-feruloyltyramine) and terpenoid (hispanolone) (Fig. 7).
Figure 7: A proposed schematic model to elucidate the link between browning and changes in nutritional quality in short term stored (4 h) of freshly cut potato shred by polyphenol oxidases (PPO) and changes in metabolites. The black arrows point to change in the short-term stored shreds. The blue and red arrows indicate increased and decreased, respectively
4.1 Browning of CK4H Shreds Largely Controlled by Polyphenols, Amino Acids and Organic Acids with Increased PPO Activity
Several studies have been conducted on metabolites involved in browning or anti-browning of fresh potato and its related products with at least two different cultivars [22,30,33,37]. These previous studies allowed for the identification of metabolites involved in browning of fresh potato and its products. However, the confounding genetic background of many different cultivars makes it extremely difficult to apply in production technology to prevent/reduce browning in the potato industry [69]. Therefore, to overcome the confounding effect of different genetic background of potato cultivars used in previous studies, the present study used fresh cut potato shreds of Feiwuruita cv (highly susceptible to browning) [34] stored for 0 and 4 h (25°C) to quantify color changes and global metabolome changes involved in browning of shreds during short-term storage. The freshly cut potato shreds turned brown within 4 h of storage (Figs. 1A and 1B). This highlights the susceptibility of Feiwuruita cv to browning. Also, the wounds created during processing (peeling and shredding) according to Cabezas-Serrano et al. [29] predispose the shreds to browning due to increase in PPO and other enzymes activity (Figs. 1B and 7). PPOs are known as copper-containing enzymes that catalyze the oxidation of a wide range of phenolic compounds to their respective quinones [19,29].
It was observed that polyphenolic compounds (i.e., phenolic acids, and flavonoids) largely showed higher accumulation in the shreds during short-term storage (4 h) (Fig. 5A). Polyphenolic compounds are known to be substrates for PPO activity, among other factors that have been elucidated to influence browning in potato and other crops [28,33,55,56,58,70,71]. For example, caffeic acid accumulated almost 11 times more in the shreds of CK4H than in CK (Fig. 5A), and this metabolite has been shown to be one of the suitable substrates for PPO activity in potato as reported by Feng et al. [55]. This and other identified polyphenolic compounds (e.g., cinnamic acid, vanillic acid, vanillin, 5-O-caffeoylshikimic acid, 5-O-p-coumaroylquinic acid, 3-O-p-coumaroylquinic acid, isoferulic acid, ferulic acid, 2-(formylamino)benzoic acid) are likely to initiate symptoms of browning in fresh-cut potato shreds by serving as substrates and reacting with oxidative enzymes [29].
In this study, several metabolites such as L-ascorbic acid and glutathione in reduced form were highly accumulated prior to short-term storage of the fresh-cut potato shreds, but their accumulation in CK4H were reduced (Fig. 5B; Table 1). These two compounds have been shown to inhibit browning by enhancing the formation of colorless products from o-quinones using reductive compounds that either reduce o-quinones back to their o-diphenolic precursors or form colorless addition products with o-quinones [58,72]. An attempt could be made to intentionally increase the abundance of reduced form compounds of L-ascorbic acid and glutathione in freshly cut potato shreds stored for a short period of time to mitigate browning caused by polyphenolic compounds. Conversely, several other amino acids accumulated more in the CK4H shreds than in the CK shreds (Fig. 5B; Table 1). Amino acids have been shown to react with quninones to facilitate the browning process [57]. For example, the phenylalanine compound caused 165% increase in browning of potato tubers in the study by Ali et al. [57]. Specifically, Mayer [73] showed that highly reactive quinones can polymerize with proteins, amino acids, or other macromolecules to form black, brown, or red pigments that accumulate on wounded tissues, including potato tubers. Five derivatives of phenylalanine (L-alanyl-L-phenylalanine, L-leucyl-L-phenylalanine, L-valyl-L-phenylalanine, 3,4-dihydroxy-L-phenylalanine (L-dopa), and L-glycyl-L-phenylalanine) were identified to accumulate highly during short-term storage of shreds, and these compounds with high abundance may contribute to induce browning by forming colored catechol-amino acid adducts [57,74]. To gain the actual role of some the key metabolites in promoting browning of shreds from Feiwuruita cv, future studies will be designed to include some of them to unearth the relationship between each of the metabolites and browning.
Phenylalanine metabolism is a precursor for phenylpropanoid biosynthesis, which largely produces polyphenolic compounds that can serve as substrates for PPO activity [48–50]. In this study, several compounds accumulated differentially in the phenylpropanoid biosynthetic pathway (Fig. 6). Phenylalanine, pyrimidine, and purine metabolism as well as zeatin biosynthetic pathway may account for high proportion of amino acids and nucleotides and derivatives, which have been elucidated above to moderate browning of fresh-cut potato shreds in this study and several others [12–14,25,57,70,75]. Therefore, the identified metabolites could be validated and used as biomarkers for selection of potato shreds during processing.
4.2 Short Term Storage of Fresh Cut Potato Shreds Altered Nutritional Quality
Our current study showed that shreds of fresh Feiwuruita cv [34] contain high quantities of lipid compounds (Table S1, Figs. 2A and 7) which is consistent with the observation made by Chaparro et al. [35]. However, it was generally observed that the short-term storage of the shreds of fresh Feiwuruita significantly reduced the abundance of lipid compounds that will be beneficial to human health. Yamamoto et al. [76] showed that lysoPE, e.g., LysoPC 18: 2, is involved in lipid droplet formation by suppressing lipolysis and fatty acid biosynthesis, indicating its possible pathological role in the induction of fatty liver disease. Other compelling evidence from Gonzalez et al. [77] suggest the involvement of some members of LysoPE and LysoPC in chronic pain in female adolescents by signaling molecules known to moderate pain perception and mediate inflammation. According to Tan et al. [78], Schober et al. [79], Engel et al. [80], LysoPE and LysoPC levels increase significantly in human inflammatory liver tissue. Interestingly, eicosenoic acid, which is an unsaturated fatty acid, for example cis-11-eicosenoic acid, is found in a variety of plant oils [81]. Increased levels of eicosenoic acid were observed in red blood cells have been reported to be associated with autism in children [82]. All this suggests that short-term storage of freshly cut potato shavings reduces lipids (LysoPC and LysoPE) (Table S2), which may be a solution to reduce the abundance of undesirable lipid compounds in freshly harvested potato tubers. Strikingly, 2-linoleoylglycerol-1,3-di-O-glucoside accumulated slightly more than 2-fold higher in CK4H than in CK (Table S2), and this metabolite has been identified as a major contributor to the flavor of radish (Raphanus sativus L.) taproot [53]. This suggests that CK4H shred may have a better flavor than the CKs, but further studies are needed to validate this speculation.
In contrast to the reduced lipids in CK4H shreds, short-term storage (4 h) increased 9α-hydroxysophoramine, tataramide A, phenylethanolamine, 4-hydroxy-2-benzoxazolone (HBOA), and N-feruloyltyramine (Table S2). 9α-Hydroxysophoramine is reported to have phytopharmacological properties used to expel worms from the intestine, treat fatigue, jaundice and fever [83]. Plants with tataramide A compound are being promoted to accelerate adult neurogenesis as it possesses ability to help aged people to recover from neurodegeneration and cognitive impairments [84]. Phenylethanolamine is one of the so-called trace amines (TA), which are reported to be involved in the regulation of a number of behaviors and play a central role in the proper functioning of the nervous system in genera [85]. They also showed that TAs are present in minute amounts in the brain, but a reduction in TA compounds such as phenylethanolamine is associated with a variety of neurological disorders, including Parkinson’s disease, schizophrenia, depression, and many others [85]. Another study showed that HBOA has beneficial effects against liver fibrosis by inhibiting the inflammatory response in rats [86]. Another alkaloid compound, N-feruloyltyramine, has been shown to prevent scopolamine-induced memory impairment and significantly reduce scopolamine-induced changes in brain areas [87]. These elaborated compounds and a terpenoid compound (hispanolone) require their extraction from short-term stored (4 h) freshly cut potato shreds to determine their functional roles in vitro.
This study shows that a popular Chinese potato Feiwuruita cv shreds turned brown within 4 h of storage, which was largely controlled by PPO activity. The metabolomic profile of CK and CK4H shreds differed significantly in alkaloids, amino acids and derivatives, flavonoids, phenolic acids, lignans and coumarins, nucleotides and derivatives, terpenoids, lipids, and others (vitamins, saccharides, and alcohols). It was generally observed that polyphenolic compounds including phenolic acids, flavonoid and its derivatives and, amino acids and nucleotides as well as their derivatives largely increased in abundance upon short term storage of potato shreds. On the other hand, short-term storage of freshly cut potato shreds resulted in a drastic reduction of lipid compounds and an increased abundance of trace alkaloids and terpenoids that are beneficial to human health. The detected compounds were largely enriched in phenylpropanoid and zeatin biosynthesis, as well as pyrimidine and purine metabolism. The information provided herein would be useful for potato shreds processing.
Acknowledgement: Not applicable.
Funding Statement: This research was funded by Major Science and Technology Project of Xiamen, China (3502Z20211004), Xiamen Science and Technology Assistance Project (3502Z20194509, 3502Z20204504-2, 3502Z20204501-3).
Author Contributions: The authors confirm contribution to the paper as follows: study conceptualization, L. H., J. W., W. W.; methodology, L. H.; software, L. H.; validation, E. H., W. Z., M. Z.; formal analysis, L. H.; investigation, L. H., E. H.,W. Z., M. Z.; resources, E. H.,W. Z., M. Z.; writing—original draft preparation, L. H.; writing—review and editing, J. W., W. W.; visualization, M. Z., J. W.; supervision, W. W.; project administration, W. W.; funding acquisition, W. W. All authors reviewed the results and approved the final version of the manuscript.
Availability of Data and Materials: Data supporting reported results can be found in the supplementary files.
Conflicts of Interest: The authors declare that they have no conflicts of interest to report regarding the present study.
Supplementary Materials: The supplementary material is available online at https://doi.org/10.32604/phyton.2023.030928.
References
1. Zhang, H., Xu, F., Wu, Y., Hu, H. H., Dai, X. F. (2017). Progress of potato staple food research and industry development in China. Journal of Integrative Agriculture, 16(12), 2924–2932. https://doi.org/10.1016/S2095-3119(17)61736-2 [Google Scholar] [CrossRef]
2. Robertson, T. M., Alzaabi, A. Z., Robertson, M. D., Fielding, B. A. (2018). Starchy carbohydrates in a healthy diet: The role of the humble potato. Nutrients, 10(11), 1764. https://doi.org/10.3390/nu10111764 [Google Scholar] [PubMed] [CrossRef]
3. Beals, K. A. (2019). Potatoes, nutrition and health. American Journal of Potato Research, 96(2), 102–110. https://doi.org/10.1007/s12230-018-09705-4 [Google Scholar] [CrossRef]
4. Górska-Warsewicz, H., Rejman, K., Kaczorowska, J., Laskowski, W. (2021). Vegetables, potatoes and their products as sources of energy and nutrients to the average diet in Poland. International Journal of Environmental Research and Public Health, 18(6), 3217. https://doi.org/10.3390/ijerph18063217 [Google Scholar] [PubMed] [CrossRef]
5. Decker, E. A., Ferruzzi, M. G. (2013). Innovations in food chemistry and processing to enhance the nutrient profile of the white potato in all forms. Advances in Nutrition, 4(3), 345S–350S. https://doi.org/10.3945/an.112.003574 [Google Scholar] [PubMed] [CrossRef]
6. Tian, J., Chen, J., Ye, X., Chen, S. (2016). Health benefits of the potato affected by domestic cooking: A review. Food Chemistry, 202(5), 165–175. https://doi.org/10.1016/j.foodchem.2016.01.120 [Google Scholar] [PubMed] [CrossRef]
7. Wang, Z. J., Liu, H., Zeng, F. K., Yang, Y. C., Xu, D. et al. (2022). Potato processing industry in China: Current scenario, future trends and global impact. Potato Research, 66(2), 543–562. https://doi.org/10.1007/s11540-022-09588-3 [Google Scholar] [PubMed] [CrossRef]
8. Drapal, M., de Boeck, B., Kreuze, H. L., Bonierbale, M., Fraser, P. D. (2023). Identification of metabolites associated with boiled potato sensory attributes in freshly harvested and stored potatoes. Journal of Food Composition and Analysis, 115, 104934. https://doi.org/10.1016/j.jfca.2022.104934 [Google Scholar] [CrossRef]
9. Su, W., Wang, J. (2019). Potato and food security in China. American Journal of Potato Research, 96(2), 100–101. https://doi.org/10.1007/s12230-018-09709-0 [Google Scholar] [CrossRef]
10. Zhuang, H., Liu, S., Wang, K., Zhong, R., Aheto, J. H. et al. (2022). Characterisation of pasting, structural and volatile properties of potato flour. Agriculture, 12(12), 1974. https://doi.org/10.3390/agriculture12121974 [Google Scholar] [CrossRef]
11. Amit, S. K., Uddin, M. M., Rahman, R., Islam, S. M. R., Khan, M. S. (2017). A review on mechanisms and commercial aspects of food preservation and processing. Agriculture and Food Security, 6(1), 51. https://doi.org/10.1186/s40066-017-0130-8 [Google Scholar] [CrossRef]
12. Matsuura-Endo, C., Ohara-Takada, A., Chuda, Y., Ono, H., Yada, H. et al. (2006). Effects of storage temperature on the contents of sugars and free amino acids in tubers from different potato cultivars and acrylamide in chips. Bioscience, Biotechnology, and Biochemistry, 70(5), 1173–1180. https://doi.org/10.1271/bbb.70.1173 [Google Scholar] [PubMed] [CrossRef]
13. Pęksa, A., Miedzianka, J., Nemś, A., Rytel, E. (2021). The free-amino-acid content in six potatoes cultivars through storage. Molecules, 26(5), 1322. https://doi.org/10.3390/molecules26051322 [Google Scholar] [PubMed] [CrossRef]
14. Brierley, E. R., Bonner, P. L. R., Cobb, A. H. (1996). Factors influencing the free amino acid content of potato (Solanum tuberosum L.) tubers during prolonged storage. Journal of the Science of Food and Agriculture, 70, 515–525. https://doi.org/10.1002/(SICI)1097-0010(199604)70:4<515::AID-JSFA529>3.0.CO;2-P [Google Scholar] [CrossRef]
15. Galdón, B. R., Mesa, D. R., Rodríguez, E. M. R., Romero, C. D. (2010). Amino acid content in traditional potato cultivars from the Canary Islands. Journal of Food Composition and Analysis, 23(2), 148–153. https://doi.org/10.1016/j.jfca.2009.08.009 [Google Scholar] [CrossRef]
16. Pęksa, A., Kita, A., Kułakowska, K., Aniołowska, M., Hamouz, K. et al. (2013). The quality of protein of coloured fleshed potatoes. Food Chemistry, 141(3), 2960–2966. https://doi.org/10.1016/j.foodchem.2013.05.125 [Google Scholar] [PubMed] [CrossRef]
17. Blenkinsop, R. W., Copp, L. J., Yada, R. Y., Marangoni, A. G. (2002). Changes in compositional parameters of tubers of potato (Solanum tuberosum) during Low-temperature storage and their relationship to chip processing quality. Journal of Agricultural and Food Chemistry, 50(3), 4545–4553. https://doi.org/10.1016/j.foodchem.2013.05.125 [Google Scholar] [CrossRef]
18. Mosneaguta, R., Alvarez, V., Barringer, S. A. (2012). The effect of antibrowning agents on inhibition of potato browning, volatile organic compound profile, and microbial inhibition. Journal of Food Science, 77(11), C1234–C1240. https://doi.org/10.1111/j.1750-3841.2012.02957.x [Google Scholar] [PubMed] [CrossRef]
19. González, M. N., Massa, G. A., Andersson, M., Turesson, H., Olsson, N. et al. (2020). Reduced enzymatic browning in potato tubers by specific editing of a polyphenol oxidase gene via ribonucleoprotein complexes delivery of the CRISPR/Cas9 system. Frontiers in Plant Science, 10, 2019. https://doi.org/10.3389/fpls.2019.01649 [Google Scholar] [PubMed] [CrossRef]
20. Xiao, Q., Bai, X., He, Y. (2020). Rapid screen of the color and water content of fresh-cut potato tuber slices using hyperspectral imaging coupled with multivariate analysis. Foods, 9(1), 94. https://doi.org/10.3390/foods9010094 [Google Scholar] [PubMed] [CrossRef]
21. Mi Moon, K., Young Kim, C., Yeul Ma, J., Lee, B. (2019). Xanthone-related compounds as an anti-browning and antioxidant food additive. Food Chemistry, 274, 345–350. https://doi.org/10.1016/j.foodchem.2018.08.144 [Google Scholar] [PubMed] [CrossRef]
22. Tao, N., Wang, R., Xu, X., Dong, T., Zhang, S. et al. (2021). Xanthosine is a novel anti-browning compound in potato identified by widely targeted metabolomic analysis and in vitro test. Postharvest Biology and Technology, 171, 111367. https://doi.org/10.1016/j.postharvbio.2020.111367 [Google Scholar] [CrossRef]
23. Tsikrika, K., Tzima, K., Rai, D. K. (2022). Recent advances in anti-browning methods in minimally processed potatoes–A review. Journal of Food Processing and Preservation, 46, e16298. https://doi.org/10.1111/jfpp.16298 [Google Scholar] [CrossRef]
24. Ma, Y., Wang, Q., Hong, G., Cantwell, M. (2010). Reassessment of treatments to retard browning of fresh-cut Russet potato with emphasis on controlled atmospheres and low concentrations of bisulphite. International Journal of Food Science and Technology, 45(7), 1486–1494. https://doi.org/10.1111/j.1365-2621.2010.02294.x [Google Scholar] [CrossRef]
25. Meng, Z., Wang, T., Malik, A. U., Wang, Q. (2022). Exogenous isoleucine can confer browning resistance on fresh-cut potato by suppressing polyphenol oxidase activity and improving the antioxidant capacity. Postharvest Biology and Technology, 184, 111772. https://doi.org/10.1016/j.postharvbio.2021.111772 [Google Scholar] [CrossRef]
26. Vally, H., Misso, N. L. A. (2012). Adverse reactions to the sulphite additives. Gastroenterology and Hepatology from Bed to Bench, 5(1), 16–23. [Google Scholar] [PubMed]
27. D’Amore, T., di Taranto, A., Berardi, G., Vita, V., Marchesani, G. et al. (2020). Sulfites in meat: Occurrence, activity, toxicity, regulation, and detection. A comprehensive review. Comprehensive Reviews in Food Science and Food Safety, 19(5), 2701–2720. https://doi.org/10.1111/1541-4337.12607 [Google Scholar] [PubMed] [CrossRef]
28. Hou, X., Liu, W., Zuo, W., Zhang, R., Zou, Q. et al. (2022). Analysis of enzymes and phenolic metabolites which affecting the anti-browning property of ‘Shannongsu’ pear. LWT, 168(15), 113919. https://doi.org/10.1016/j.lwt.2022.113919 [Google Scholar] [CrossRef]
29. Cabezas-Serrano, A. B., Amodio, M. L., Cornacchia, R., Rinaldi, R., Colelli, G. (2009). Suitability of five different potato cultivars (Solanum tuberosum L.) to be processed as fresh-cut products. Postharvest Biology and Technology, 53(3), 138–144. https://doi.org/10.1016/j.postharvbio.2009.03.009 [Google Scholar] [CrossRef]
30. Qiao, L., Gao, M., Wang, Y., Tian, X., Lu, L. et al. (2022). Integrated transcriptomic and metabolomic analysis of cultivar differences provides insights into the browning mechanism of fresh-cut potato tubers. Postharvest Biology and Technology, 188(3), 111905. https://doi.org/10.1016/j.postharvbio.2022.111905 [Google Scholar] [CrossRef]
31. Garcia, C. J., García-Villalba, R., Garrido, Y., Gil, M. I., Tomás-Barberán, F. A. (2016). Untargeted metabolomics approach using UPLC-ESI-QTOF-MS to explore the metabolome of fresh-cut iceberg lettuce. Metabolomics, 12(8), 138. https://doi.org/10.1007/s11306-016-1082-x [Google Scholar] [CrossRef]
32. Hu W., Guan, Y., Feng K. (2022). Biosynthesis of phenolic compounds and antioxidant activity in fresh-cut fruits and vegetables. Frontiers in Microbiology, 13, 906069. https://doi.org/10.3389/fmicb.2022.906069 [Google Scholar] [PubMed] [CrossRef]
33. Cantos, E., Tudela, J. A., Gil, M. I., Espín, J. C. (2002). Phenolic compounds and related enzymes are not rate-limiting in browning development of fresh-cut potatoes. Journal of Agricultural and Food Chemistry, 50(10), 3015–3023. https://doi.org/10.1021/jf0116350 [Google Scholar] [PubMed] [CrossRef]
34. Wang, C., Du, C., Yang, Z., Wang, H., Shang, L. et al. (2022). Study on the cultivation of seedlings using buds of potato (Solanum tuberosum L.). PeerJ, 10(3), e13804. https://doi.org/10.7717/peerj.13804 [Google Scholar] [PubMed] [CrossRef]
35. Chaparro, J. M., Holm, D. G., Broeckling, C. D., Prenni, J. E., Heuberger, A. L. (2018). Metabolomics and ionomics of potato tuber reveals an influence of cultivar and market class on human nutrients and bioactive compounds. Frontiers in Nutrition, 5, 36. https://doi.org/10.3389/fnut.2018.00036 [Google Scholar] [PubMed] [CrossRef]
36. Burgos, G., Zum Felde, T., Andre, C., Kubow, S. (2020). The potato and its contribution to the human diet and health. In: Campos, H., Ortiz, O. (Eds.The potato crop: Its agricultural, nutritional and social contribution to humankind, pp. 37–74. Cham, Switzerland: Springer International Publishing. [Google Scholar]
37. Orsák, M., Hamouz, K., Lachman, J., Kasal, P. (2019). Chlorogenic acid content in potato tubers with colored flesh as affected by a genotype, location and long-term storage. Plant, Soil and Environment, 65(7), 355–360. https://doi.org/10.17221/195/2019-PSE [Google Scholar] [CrossRef]
38. Dresow, J. F., Böhm, H. (2009). The influence of volatile compounds of the flavour of raw, boiled and baked potatoes: Impact of agricultural measures on the volatile components. Landbauforschung-vTI Agriculture and Forestry Research, 59, 309–338. [Google Scholar]
39. Sarpong, F., Oteng-Darko, P., Golly, M. K., Amenorfe, L. P., Rashid, M. T. et al. (2018). Comparative study of enzymes inactivation and browning pigmentation of apple (Malus domestica) slices by selected gums during low temperature storage. Journal of Food Biochemistry, 42, e12681. https://doi.org/10.1111/jfbc.12681 [Google Scholar] [CrossRef]
40. Bai, J. W., Dai, Y., Wang, Y. C., Cai, J. R., Zhang, L. et al. (2022). Potato slices drying: Pretreatment affects the three-dimensional appearance and quality attributes. Agriculture, 12(11), 1841. https://doi.org/10.3390/agriculture12111841 [Google Scholar] [CrossRef]
41. Wen, Y. J., Zhang, H., Ni, Y. L., Huang, B., Zhang, J. et al. (2018). Methodological implementation of mixed linear models in multi-locus genome-wide association studies. Brief in Bioinformatics, 18(5), 700–712. https://doi.org/10.1093/bib/bbw145 [Google Scholar] [PubMed] [CrossRef]
42. Wickham, H., Averick, M., Bryan, J., Chang, W., McGowan, L. D. A. et al. (2019). Welcome to the Tidyverse. Journal of Open Source Software, 4(43), 1686. https://doi.org/10.21105/joss.01686 [Google Scholar] [CrossRef]
43. Kolde, R. (2012). Pheatmap: Pretty heatmaps. R Package Version, 1, 747. [Google Scholar]
44. Vanhaeren, H., Nam, Y. J., De Milde, L., Chae, E., Storme, V. et al. (2017). Forever young: The role of ubiquitin receptor DA1 and E3 ligase BIG BROTHER in controlling leaf growth and development. Plant Physiology, 173(2), 1269–1282. https://doi.org/10.1104/pp.16.01410 [Google Scholar] [PubMed] [CrossRef]
45. Shan, M., Liu, H., Hao, Y., Song, K., Meng, T. et al. (2022). Metabolomic profiling reveals that 5-Hydroxylysine and 1-Methylnicotinamide are metabolic indicators of keloid severity. Frontiers in Genetics, 12, 804248. https://doi.org/10.3389/fgene.2021.804248 [Google Scholar] [PubMed] [CrossRef]
46. Arakawa, K., Kono, N., Yamada, Y., Mori, H., Tomita, M. (2005). KEGG-based pathway visualization tool for complex omics data. In Silico Biology, 5, 419–423. [Google Scholar] [PubMed]
47. Li, Q., Song, J. (2019). Analysis of widely targeted metabolites of the euhalophyte Suaeda salsa under saline conditions provides new insights into salt tolerance and nutritional value in halophytic species. BMC Plant Biology, 19(1), 388. https://doi.org/10.1186/s12870-019-2006-5 [Google Scholar] [PubMed] [CrossRef]
48. Navarre, D. A., Payyavula, R. S., Shakya, R., Knowles, N. R., Pillai, S. S. (2013). Changes in potato phenylpropanoid metabolism during tuber development. Plant Physiology and Biochemistry, 65, 89–101. https://doi.org/10.1016/j.plaphy.2013.01.007 [Google Scholar] [PubMed] [CrossRef]
49. Fan, J., Du, W., Chen, Q. L., Zhang, J. G., Yang, X. P. et al. (2021). Comparative transcriptomic analyses provide insights into the enzymatic browning mechanism of fresh-cut sand pear fruit. Horticulturae, 7(11), 502. https://doi.org/10.3390/horticulturae7110502 [Google Scholar] [CrossRef]
50. Li, X., Zhang, S., Wang, Q., Dong, T. (2023). Diacetyl inhibits the browning of fresh-cut stem lettuce by regulating the metabolism of phenylpropane and antioxidant ability. Foods, 12(4), 740. https://doi.org/10.3390/foods12040740 [Google Scholar] [PubMed] [CrossRef]
51. Payyavula, R. S., Navarre, D. A., Kuhl, J. C., Pantoja, A., Pillai, S. S. (2012). Differential effects of environment on potato phenylpropanoid and carotenoid expression. BMC Plant Biology, 12(1), 39. https://doi.org/10.1186/1471-2229-12-39 [Google Scholar] [PubMed] [CrossRef]
52. Feduraev, P., Skrypnik, L., Riabova, A., Pungin, A., Tokupova, E. et al. (2020). Phenylalanine and Tyrosine as exogenous precursors of wheat (Triticum aestivum L.) secondary metabolism through PAL-associated pathways. Plants, 9(4), 476. https://doi.org/10.3390/plants9040476 [Google Scholar] [PubMed] [CrossRef]
53. Mei, S., He, Z., Zhang, J. (2022). Identification and analysis of major flavor compounds in radish taproots by widely targeted metabolomics. Frontiers in Nutrition, 9, 889407. https://doi.org/10.3389/fnut.2022.889407 [Google Scholar] [PubMed] [CrossRef]
54. Sheng, Y. Y., Xiang, J., Lu, J. L., Ye, J. H., Chen, Z. J. et al. (2022). Protective effects of gallocatechin gallate against ultraviolet B induced skin damages in hairless mice. Scientific Reports, 12(1), 1310. https://doi.org/10.1038/s41598-022-05305-9 [Google Scholar] [PubMed] [CrossRef]
55. Feng, Y., Liu, Q., Liu, P., Shi, J., Wang, Q. (2020). Aspartic acid can effectively prevent the enzymatic browning of potato by regulating the generation and transformation of brown product. Postharvest Biology and Technology, 166, 111209. https://doi.org/10.1016/j.postharvbio.2020.111209 [Google Scholar] [CrossRef]
56. Baek, S. H., Kwon, S. Y., Lee, H. G., Baek, H. H. (2008). Maillard browning reaction of D-psicose as affected by reaction factors. Food Science and Biotechnology, 17(6), 1349–1351. [Google Scholar]
57. Ali, H. M., El-Gizawy, A. M., El-Bassiouny, R. E. I., Saleh, M. A. (2016). The role of various amino acids in enzymatic browning process in potato tubers, and identifying the browning products. Food Chemistry, 192, 879–885. https://doi.org/10.1016/j.foodchem.2015.07.100 [Google Scholar] [PubMed] [CrossRef]
58. Kuijpers, T. F. M., Narváez-Cuenca, C. E., Vincken, J. P., Verloop, A. J. W., van Berkel, W. J. H. et al. (2012). Inhibition of enzymatic browning of chlorogenic acid by sulfur-containing compounds. Journal of Agricultural and Food Chemistry, 60(13), 3507–3514. https://doi.org/10.1021/jf205290w [Google Scholar] [PubMed] [CrossRef]
59. Hamdan, N., Lee, C. H., Wong, S. L., Fauzi, C. E., Zamri, N. M. et al. (2022). Prevention of enzymatic browning by natural extracts and genome-editing. A Review on Recent Progress. Molecules, 27(3), 1101. https://doi.org/10.3390/molecules27031101 [Google Scholar] [CrossRef]
60. Dias, C., Fonseca, A. M. A., Amaro, A. L., Vilas-Boas, A. A., Oliveira, A. et al. (2020). Natural-based antioxidant extracts as potential mitigators of fruit browning. Antioxidants, 9(8), 715. https://doi.org/10.3390/antiox9080715 [Google Scholar] [PubMed] [CrossRef]
61. He, M., Zhou, Y., Zhu, H., Jiang, Y., Qu, H. (2022). Metabolome, transcriptome and physiological analyses provide insight into the color transition of litchi pericarp. Postharvest Biology and Technology, 192, 112031. https://doi.org/10.1016/j.postharvbio.2022.112031 [Google Scholar] [CrossRef]
62. Joanna, K. (2019). Alkaloids–their importance in nature and for human life. Rijeka: IntechOpen. https://doi.org/10.5772/intechopen.85400 [Google Scholar] [CrossRef]
63. Debnath, B., Singh, W. S., Das, M., Goswami, S., Singh, M. K. et al. (2018). Role of plant alkaloids on human health: A review of biological activities. Materials Today Chemistry, 9(2), 56–72. https://doi.org/10.1016/j.mtchem.2018.05.001 [Google Scholar] [CrossRef]
64. Senchina, D. S., Hallam, J. E., Kohut, M. L., Nguyen, N. A., Perera, M. A. (2014). Alkaloids and athlete immune function: Caffeine, theophylline, gingerol, ephedrine, and their congeners. Exercise Immunology Review, 20, 68–93. [Google Scholar] [PubMed]
65. Deepak Kumar, D., Chandra Kishore, T., Anil Kumar, S., Vaibhav, T. (2022). Revisiting the medicinal value of terpenes and terpenoids. In: Vijay Singh, M., Hanuman Prasad, P., Sunita Kumari, M. (Eds.Revisiting plant biostimulants. Rijeka: IntechOpen. https://doi.org/10.5772/intechopen.102612 [Google Scholar] [CrossRef]
66. Jiménez-García, L., Higueras, M.Á., Herranz, S., Hernández-López, M., Luque, A. et al. (2018). A hispanolone-derived diterpenoid inhibits M2-Macrophage polarization in vitro via JAK/STAT and attenuates chitin induced inflammation in vivo. Biochemical Pharmacology, 154, 373–383. https://doi.org/10.1016/j.bcp.2018.06.002 [Google Scholar] [PubMed] [CrossRef]
67. Siraj, M. A., Islam, M. A., Al Fahad, M. A., Kheya, H. R., Xiao, J. et al. (2021). Cancer chemopreventive role of dietary terpenoids by modulating Keap1-Nrf2-ARE signaling system—A comprehensive update. Applied Sciences, 11(22), 10806. https://doi.org/10.3390/app112210806 [Google Scholar] [CrossRef]
68. Dey, P., Kundu, A., Chakraborty, H. J., Kar, B., Choi, W. S. et al. (2019). Therapeutic value of steroidal alkaloids in cancer: Current trends and future perspectives. International Journal of Cancer, 145(7), 1731–1744. https://doi.org/10.1002/ijc.31965 [Google Scholar] [PubMed] [CrossRef]
69. Liu, Y., Li, Y. M., Liu, Z., Wang, L., Wang, L. K. et al. (2023). Integrative analysis of metabolome and transcriptome reveals a dynamic regulatory network of potato tuber pigmentation. iScience, 26(2), 105903. https://doi.org/10.1016/j.isci.2022.105903 [Google Scholar] [PubMed] [CrossRef]
70. Mishra, B. B., Gautam, S., Sharma, A. (2013). Free phenolics and polyphenol oxidase (PPOThe factors affecting post-cut browning in eggplant (Solanum melongena). Food Chemistry, 139(1–4), 105–114. https://doi.org/10.1016/j.foodchem.2013.01.074 [Google Scholar] [PubMed] [CrossRef]
71. Toro-Uribe, S., Godoy-Chivatá, J., Villamizar-Jaimes, A. R., Perea-Flores, M. D. J., López-Giraldo, L. J. (2020). Insight of polyphenol oxidase enzyme inhibition and total polyphenol recovery from cocoa beans. Antioxidants, 9(6), 458. https://doi.org/10.3390/antiox9060458 [Google Scholar] [PubMed] [CrossRef]
72. Nappi, A. J., Vass, E. (1994). Chromatographic analyses of the effects of glutathione, cysteine and ascorbic acid on the monophenol and diphenol oxidase activities of tyrosinase. Journal of Liquid Chromatography, 17(4), 793–815. https://doi.org/10.1080/10826079408013369 [Google Scholar] [CrossRef]
73. Mayer, A. M. (2006). Polyphenol oxidases in plants and fungi: Going places? A review. Phytochemistry, 67(21), 2318–2331. https://doi.org/10.1016/j.phytochem.2006.08.006 [Google Scholar] [PubMed] [CrossRef]
74. Girelli, A. M., Mattei, E., Messina, A., Tarola, A. M. (2004). Inhibition of polyphenol oxidases activity by various dipeptides. Journal of Agricultural and Food Chemistry, 52(10), 2741–2745. https://doi.org/10.1021/jf0305276 [Google Scholar] [PubMed] [CrossRef]
75. Xu, X., Liu, P., Dong, T., Zhang, S., Wang, Q. (2022). Short-term warming inhibits polyphenol oxidase activity and influences free amino acid accumulation and de novo synthesis of tyrosine in fresh-cut potato. Postharvest Biology and Technology, 186(7), 111830. https://doi.org/10.1016/j.postharvbio.2021.111830 [Google Scholar] [CrossRef]
76. Yamamoto, Y., Sakurai, T., Chen, Z., Inoue, N., Chiba, H. et al. (2022). Lysophosphatidylethanolamine affects lipid accumulation and metabolism in a human liver-derived cell line. Nutrients, 14(3), 579. https://doi.org/10.3390/nu14030579 [Google Scholar] [PubMed] [CrossRef]
77. Gonzalez, P. A., Simcox, J., Raff, H., Wade, G., von Bank, H. et al. (2022). Lipid signatures of chronic pain in female adolescents with and without obesity. Lipids in Health and Disease, 21(1), 80. https://doi.org/10.1186/s12944-022-01690-2 [Google Scholar] [PubMed] [CrossRef]
78. Tan, S. T., Ramesh, T., Toh, X. R., Nguyen, L. N. (2020). Emerging roles of lysophospholipids in health and disease. Progress in Lipid Research, 80(5), 101068. https://doi.org/10.1016/j.plipres.2020.101068 [Google Scholar] [PubMed] [CrossRef]
79. Schober, C., Schiller, J., Pinker, F., Hengstler, J. G., Fuchs, B. (2009). Lysophosphatidylethanolamine is-in contrast to-choline–generated under in vivo conditions exclusively by phospholipase A2 but not by hypochlorous acid. Bioorganic Chemistry, 37(6), 202–210. https://doi.org/10.1016/j.bioorg.2009.09.002 [Google Scholar] [PubMed] [CrossRef]
80. Engel, K. M., Schiller, J., Galuska, C. E., Fuchs, B. (2021). Phospholipases and reactive oxygen species derived lipid biomarkers in healthy and diseased humans and animals—A focus on lysophosphatidylcholine. Frontiers in Physiology, 12, 732319. https://doi.org/10.3389/fphys.2021.732319 [Google Scholar] [PubMed] [CrossRef]
81. Wishart, D. S., Knox, C., Guo, A. C., Eisner, R., Young, N. et al. (2009). HMDB: A knowledgebase for the human metabolome. Nucleic Acids Research, 37(S1), D603–D610. [Google Scholar] [PubMed]
82. Bu, B., Ashwood, P., Harvey, D., King, I. B., Water, J. V. D. et al. (2006). Fatty acid compositions of red blood cell phospholipids in children with autism. Prostaglandins, Leukotrienes and Essential Fatty Acids, 74(4), 215–221. https://doi.org/10.1016/j.plefa.2006.02.001 [Google Scholar] [PubMed] [CrossRef]
83. Wiart, C. (2014). Alkaloids. In: Wiart, C. (Ed.Lead compounds from medicinal plants for the treatment of neurodegenerative diseases, pp. 1–188. San Diego, USA: Academic Press. [Google Scholar]
84. Krishnapriya, Sasikumar, P., Aswathy, M., Prem, P. T., Radhakrishnan, K. V. et al. (2022). Plant derived bioactive compounds and their potential to enhance adult neurogenesis. Phytomedicine Plus, 2(1), 100191. https://doi.org/10.1016/j.phyplu.2021.100191 [Google Scholar] [CrossRef]
85. Roeder, T. (2016). Trace amines: An overview. In: Farooqui, T., Farooqui, A. A. (Eds.Trace amines and neurological disorders, pp. 3–9. San Diego, USA: Academic Press. [Google Scholar]
86. Wai, K. K., Liang, Y., Zhou, L., Cai, L., Liang, C. et al. (2015). The protective effects of Acanthus ilicifolius alkaloid A and its derivatives on pro- and anti-inflammatory cytokines in rats with hepatic fibrosis. Biotechnology and Applied Biochemistry, 62(4), 537–546. https://doi.org/10.1002/bab.1292 [Google Scholar] [PubMed] [CrossRef]
87. Sirin, S., Nigdelioglu Dolanbay, S., Aslim, B. (2023). Role of plant derived alkaloids as antioxidant agents for neurodegenerative diseases. Health Sciences Review, 6, 100071. https://doi.org/10.1016/j.hsr.2022.100071 [Google Scholar] [CrossRef]
Cite This Article
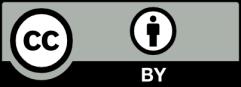