Open Access
ARTICLE
Different Deficit Irrigation Lower Limits and Irrigation Quotas Affect the Yield and Water Use Efficiency of Winter Wheat by Regulating Photosynthetic Characteristics
Faculty of Engineering, Huanghe Science and Technology University, Zhengzhou, 450000, China
* Corresponding Author: Mingzhi Zhang. Email:
(This article belongs to the Special Issue: Development of New Sensing Technology in Sustainable Farming and Smart Environmental Monitoring)
Phyton-International Journal of Experimental Botany 2023, 92(12), 3211-3236. https://doi.org/10.32604/phyton.2023.031003
Received 09 May 2023; Accepted 05 September 2023; Issue published 28 December 2023
Abstract
To determine suitable thresholds for deficit irrigation of winter wheat in the well-irrigated area of the Huang-Huai-Hai Plain, we investigated the effects of different deficit irrigation lower limits and quotas on the photosynthetic characteristics and grain yield of winter wheat. Four irrigation lower limits were set for initiating irrigation (i.e., light drought (LD, 50%, 55%, 60% and 50% of field holding capacity (FC) at the seedling-regreening, jointing, heading and filling-ripening stages, respectively), medium drought (MD, 40%, 50%, 55% and 45% of FC at the same stages, respectively), adequate moisture (CK1, 60%, 65%, 70% and 60% of FC at the same stages, respectively), heavy drought (CK2, 35%, 40%, 45% and 40% of FC at the same stages, respectively)) and five irrigation quota per event (30, 60, 90, 120 and 180 mm) were set for each lower limit. We found that the increase of drought stress is conducive to normal photosynthesis of winter wheat leaves which is supported by the following findings. First, photosynthetic rate (Pn) of LD60 treatment was higher than that of LD30, LD90, LD120, LD180, MD30, MD60, MD90, MD120 and MD180. Then, Under the 90 mm irrigation quota treatment, the yield of winter wheat basically increased with the increase of irrigation’s lower limit. Moreover, With the increase in irrigation quota, the yield of winter wheat increased, and the water use efficiency (WUE) of winter wheat increased at first and then decreased. In addition, compared with the LD30, MD30, MD60, MD90, MD120, and MD180, the yield of winter wheat in LD60 treatment increased by about 3.23% (3-year average), 32.3%, 19.9%, 11.7%, 10.1%, and 14.6%. At the same time, the WUE with LD60 treatment of winter wheat was significantly higher than LD90, LD120, LD180, MD30, MD60, MD90, MD120, MD180 treatments. There was a positive correlation between soil volumetric water content and Pn and between yield and Pn. The key period for yield formation in winter wheat is 180 days after sowing. In conclusion, to achieve the dual goals of stable winter wheat yield and efficient utilization of water resources in this region, the suitable threshold for initiating deficit irrigation of winter wheat is the LD60 treatment. This conclusion provides data support for water-saving and stable yield of winter wheat in this area.Keywords
China is the world’s largest wheat producer, accounting for 11% of the total production and 17.7% of the total yield of the world. The Huang-Huai-Hai Plain is one of China’s predominant production regions for winter wheat, accounting for about 75% of national the production of winter wheat [1]. The water consumption of winter wheat during the growing season is approximately 300–450 mm. However, the rainfall in the growing season is only 110–180 mm, which is insufficient to meet the high and stable yield of winter wheat. As a result, wheat production in the Huang-Huai-Hai Plain heavily relies on supplementary irrigation by utilizing groundwater [2,3]. In order to achieve stable and high yield of winter wheat, local residents often use irrigation to make up for the necessary water during the growth period of winter wheat [4,5]. However, the main source of irrigation water is the exploitation of groundwater. Over the past 10 years, nearly 60–80 billion cubic meters of groundwater has been extracted in the plains of northern China, particularly in the Huang-Huai-Hai well irrigation area. The severe depletion of groundwater resources has led to a range of ecological and food production issues [6,7]. Therefore, it is urgent to improve the efficient utilization of water resources in this area.
The Huang-Huai-Hai Plain mainly undertakes the rotation of summer corn and winter wheat. The maximum root system of summer corn is about 1.00 m, which is mainly distributed above the 60 cm soil layer [8–10]. However, Winter wheat is a densely planted crop with deep and numerous roots, in which 0–100 cm roots are evenly distributed and the root depth can reach 2 m at flowering stage [11,12]. Compared to summer corn, the winter wheat can make use of water and nutrients stored in deep soil [13]. Regulated deficit irrigation is carried out based on the unique climatic and environmental conditions and planting patterns in this area, that is, the dual goals of increasing grain production and efficient utilization of water resources can be achieved through appropriate lower limit of irrigation and limited irrigation. Previous studies show that drought stress promotes the growth of winter wheat roots [14], which can improve the efficiency of water and fertilizer use in deep soil [15,16], and change the distribution of photosynthetic yield. Proper control of water will not reduce crop yield or even help to increase crop yield; at the same time, after the release of moderate water stress, it shows compensation effect or over-compensation effect [17,18]. However, the amount of limited irrigation (irrigation quota) is also very important for crop growth, due to the fact that too little irrigation water can not completely eliminate water stress, resulting in reduced yield and that too much irrigation water can easily cause crop lodging and soil water infiltration, resulting in a waste of water resources [19,20]. Therefore, suitable drought stress and reasonable irrigation quota is one of the effective ways to increase winter wheat yield, to realize efficient utilization of water resources, to reduce groundwater exploitation and to protect ecological environment [21,22]. Under short-term or mild drought stress, plant leaf water potential decreases, leaf stomata close to reduce transpiration, and CO2 uptake and photosynthesis also decrease, long-term and severe drought stress can inhibit plant growth and cause irreversible changes in apparent morphology, resulting in plant death [23,24]. Previous studies have demonstrated that implementing deficit irrigation with a regulation of 150 mm and simulating rainfall with 200 mm can result in significant improvements. Specifically, these practices can increase the average photosynthetic rate by 10.4%, stomatal conductance by 27.2%, transpiration rate by 9.3%, intercellular CO2 concentration by 4.0%, grain yield by 18.9%, and water use efficiency by 75.8% [25]. Regulated deficit irrigation can inhibit the decrease of yield of winter wheat [26,27]. The mild water deficit in the early stage of winter wheat (lower limit of irrigation is 55% of field water holding capacity.) has the potential to save water, maintain economic yield and improve water use efficiency [28]. The suitable irrigation quota of winter wheat is 45.0, 55.5 and 54.0 mm, respectively at the stages of turning green to jointing, jointing to heading and heading to filling [29]. With an increase in irrigation amount, the photosynthetic rate and yield of winter wheat also increase. The output value shows a parabolic increase, but the marginal output value starts to decrease. The output value per unit of irrigation decreases following a power function. Simultaneously, crop water consumption increases logarithmically, while soil water use efficiency decreases [30–32].
However, considering the unique water resources environment and winter wheat planting methods in the well irrigation area of the Huang-Huai-Hai Plain, there is still a lack of understanding regarding the optimization of the winter wheat regulated deficit irrigation system in this area and achieving the dual goals of water conservation and stable yield. Additionally, the mechanisms behind changes in soil moisture during winter wheat regulated deficit irrigation, as well as the responses of leaf photosynthesis and yield to these changes, remain unclear. Therefore, this study aims to investigate the effects of regulated deficit irrigation (lower irrigation limit and irrigation quota) on soil moisture, leaf photosynthetic characteristics, and winter wheat yield. The findings of this study will provide valuable data to support water-saving practices and ensure stable yields in the well irrigation area of the Huang-Huai-Hai Plain.
2.1 Experimental Site and Management
The experiment was conducted at the Xuchang irrigation experimental station (E112°42′, N34°16′, Fig. 1) using a lysimeter facility equipped with a power-driven rain shelter. The experiment took place during the winter wheat growing seasons from 2019 to 2022. The dimensions of the lysimeter were 2.4 m in length, 3.6 m in width, and 3.0 m in height. The experimental site is located in the continental monsoon climate of the north temperate zone, with an altitude of 85.0 m. The average annual temperature is 14.3°C, and the average annual rainfall is 640.9 mm. The period from June to September accounts for more than 70% of the annual precipitation, and the frost-free period lasts for 220 days. The annual sunshine duration is approximately 2400 hours. The average bulk density of 1m soil layer was 1.53 g/cm3, and the field capacity was 25.40% (mass moisture content, Determination by ring knife method). The soil of the experimental site was medium loam and the mass fractions of sand, silt, and clay are 52.46%, 31.25%, and 16.29%, respectively. The content of organic matter, total phosphorus (P), total potassium (K), total nitrogen, available nitrogen, available phosphorus, and available potassium, in the plough layer before sowing was 17.85, 14.25, 2.34, 1.89, 0.97, 0.24 and 0.11 g/kg, respectively.
Figure 1: Xuchang irrigation experiment station
Winter wheat in 2019 was sown on October 21, 2019 and harvested on June 02, 2020. The whole growth period of winter wheat in 2019 was 225 days. Winter wheat in 2020 was sown on October 17, 2020 and harvested on May 24, 2021. The whole growth period of winter wheat in 2020 was 219 days. Winter wheat in 2021 was sown on October 20, 2021 and harvested on May 29, 2022. The whole growth period of winter wheat in 2021 was 221 days. The sowing rate was 149 kg/ha, and the row spacing of winter wheat was 20 cm each year. The agricultural management (fertilization, weeding, pest control, etc.) was consistent. The meteorological data of winter wheat growth period are shown in Fig. 2.
Figure 2: Meteorological data during winter wheat growth period
Two factors were set up: irrigation lower limit (D) and irrigation quota (One time with irrigation amount of winter wheat per unit irrigated area, I).
The irrigation lower limit was set at four levels, light drought (LD) and moderate drought (MD), adequate moisture (CK1) and heavy drought (CK2). Among them, the CK1 was 60%, 65%, 70% and 60% of field capacity (FC) at seedling to regreening stage, jointing stage, heading stage and filling to ripening stage, respectively. The LD was 50%, 55%, 60% and 50% of FC at the seedling to regreening stage, jointing stage, heading stage and filling to ripening stage, respectively. The MD was 40%, 50%, 55% and 45% of FC at seedling to regreening stage, jointing stage, heading stage and filling to ripening stage, respectively. The heavy drought (CK2) was 35%, 40%, 45% and 40% of FC at seedling to regreening stage, jointing stage, heading stage and filling to ripening stage, respectively. And supplementary irrigation was carried out to 80% of FC after sowing. The irrigation quota was set at five levels, which were 30, 60, 90, 120 and 180 mm, respectively, and only 90 mm of I was set for the control CK1 and CK2.
12 treatments were designed, and each treatment was repeated 3 times (Table 1). The soil moisture was monitored 30 days after winter wheat emergence. The soil moisture of winter wheat was monitored every 5 days. When the soil moisture of winter wheat was lower than the D of designed by the experiment, the irrigation quota designed by the experiment was carried out for irrigation. The monitoring and irrigation of soil moisture of winter wheat were ceased 210 days after sowing.
2.3 Measurements and Computational Methods
(1) Soil volumetric water content
The soil volumetric water content (in cm3/cm3) was measured by TRIME-IPH (TRIME-PICO-IPH, IMKO, Inc., Ettlingen, Germany) soil moisture monitoring system at 160, 170, 180 and 200 days after winter wheat sowing. The soil volumetric water content of 0-20, 20–40, 40–60, 60–80, 80–100, 100–120, 120–140, 140–160 and 160–180 cm soil layers was measured, respectively. Two monitoring points were selected for each plot, and the average value of these two monitoring points was used to represent the soil volumetric water content of the respective test plot.
(2) Photosynthetic characteristics
The LI-6400 (Li-Cor, Inc., Lincoln, Nebraska, USA) photosynthetic measurement system of LI-COR company was used to randomly select the conjoined healthy leaves with full light and consistent leaf position to measure the gas exchange parameters such as photosynthetic rate (Pn), stomatal conductance (Gs), intercellular CO2 concentration (Ci) and transpiration rate (Tr). The open-air path was used in the measurement. The CO2 gas was collected from a relatively stable 2–3 m air; the light intensity was a natural light source, and the flow rate was set at 500 μmol/s. Three to four plants were selected in each plot, and one leaf was selected for each plant. The date of determination was determined at about 9:00–11:00 AM on 160,170,180 and 200 days after winter wheat planting, and the error was within 3 days.
(3) Yield and water use efficiency
The 3 pieces of 1 m2 wheat were randomly selected from each plot to be harvested and threshed manually. The weight of 1 m2 wheat was weighed by electronic scale (0.00001 kg), and finally converted into yield per hectare (0.01 kg/ha).
The TRIME-IPH was used to measure the soil volume moisture content at different layers of soil (0–20, 20–40, 40–60, 60–80, 80–100, 100–120, 120–140, 140–160, and 160–180 cm, respectively). The soil volume moisture content of winter wheat was measured once during sowing and harvesting. Water consumption (
In the equation: WUE is crop water use efficiency, kg/m3.
3.1 Effects of Different Treatments on Soil Volumetric Water Content at Different Depths
The soil volumetric water content (VWC) for each treatment demonstrated an upward trend as soil depths increased (Figs. 3 and 4). The soil volumetric water content of the CK1 treatment was significantly higher than that of the drought stress treatment (p ≤ 0.05). As soil depth increased, the range of change in soil VWC diminished over time post-sowing, with the most substantial range observed in the 0–20 cm layer, approximately 44.1% (2019), 54.6% (2020), and 56.7% (2021). The soil VWC initially increased with the escalation of the drought stress degree (D), and then subsequently decreased. The maximum amplitude of shallow layer was 9.18% (3-year average), and the deep layer was 5.13%. The change of deep soil VWC further indicated that LD was helpful for winter wheat roots to tie down and to absorb deep soil moisture. The shallow soil (≤80 cm) VWC of 90 mm I varied greatly with the passage of time after sowing. With the increase of I, the VWC of deep soil decreased first and then increased, indicating that large I (≥120 mm) is helpful to recharge deep soil VWC. Until the utilization of shallow soil moisture is completed, the root system is down, and the deep soil VWC can be absorbed and utilized. In this study, the average change range of soil VWC of LD60 treatment with the time after sowing was higher (p ≤ 0.05), about 41.3% (2019), 21.6% (2020), 31.8% (2021).
Figure 3: Changes of soil volumetric water content at different depths during the years of 2019–2021
In this study, 12 treatments were repeated three times, a total of 36 experimental plots, each plot measured three replicates. The figures in the figure are average value ± standard error. 160d represents 160 days after sowing of winter wheat, 170d represents 170 days after sowing of winter wheat, 180d represents 180 days after sowing of winter wheat, 200d represents 200 days after sowing of winter wheat. 2019 represents winter wheat in 2019, 2020 represents winter wheat in 2020, 2021 represents winter wheat in 2021.
Figure 4: Variation of average soil volumetric water content during winter wheat growth period
Note: In this study, 12 treatments were repeated three times, a total of 36 experimental plots, each plot measured three replicates. The figures in the figure are average value ± standard error. 2019 represents winter wheat in 2019, 2020 represents winter wheat in 2020, 2021 represents winter wheat in 2021
3.2 Effects of Different Treatments on Photosynthetic Characteristics of Winter Wheat
Different treatments exerted varied effects on the soil volumetric water content (VWC) in the root zone of winter wheat. Leaf gas exchange could rapidly respond to the degree of soil water deficit in crop roots. Tables 2–5 and Figs. 5–8 illustrate the effects of different treatments on photosynthetic characteristics. The irrigation lower limit (D) had a significant impact on the photosynthetic rate (Pn), stomatal conductance (Gs), intercellular CO2 concentration (Ci), and transpiration rate (Tr) of winter wheat leaves (p ≤ 0.05). The irrigation quota (I) also significantly influenced the Pn, Gs, Ci, and Tr of winter wheat leaves (p ≤ 0.05). As the winter wheat growth period progressed, the Pn, Gs, Ci, and Tr of the leaves initially increased and then subsequently decreased.
Figure 5: Effects of different combinations of D and I on the average Pn of winter wheat leaf growth period
Note: In this study, 12 treatments were repeated three times, a total of 36 experimental plots, each plot measured three replicates. The figures in the figure are average value ± standard error. 2019 represents winter wheat in 2019, 2020 represents winter wheat in 2020, 2021 represents winter wheat in 2021. The different letters on the histogram of winter wheat in the same year indicated the significant difference between different treatments at the 0.05 level, the same below.
Figure 6: Effects of different combinations of D and I on the average Gs of winter wheat leaf growth period
Note: In this study, 12 treatments were repeated three times, a total of 36 experimental plots, each plot measured three replicates. The figures in the figure are average value ± standard error. 2019 represents winter wheat in 2019, 2020 represents winter wheat in 2020, 2021 represents winter wheat in 2021. The different letters on the histogram of winter wheat in the same year indicated the significant difference between different treatments at the 0.05 level, the same as below.
Figure 7: Effects of different combinations of D and I on the average Ci of winter wheat leaf growth period
Note: In this study, 12 treatments were repeated three times, a total of 36 experimental plots, each plot measured three replicates. The figures in the figure are average value ± standard error. 2019 represents winter wheat in 2019, 2020 represents winter wheat in 2020, 2021 represents winter wheat in 2021. The different letters on the histogram of winter wheat in the same year indicated the significant difference between different treatments at the 0.05 level, the same as below.
Figure 8: Effects of different combinations of D and I on the average Tr of winter wheat leaf growth period
Note: In this study, 12 treatments were repeated three times, a total of 36 experimental plots, each plot measured three replicates. The figures in the figure are average value ± standard error. 2019 represents winter wheat in 2019, 2020 represents winter wheat in 2020, 2021 represents winter wheat in 2021. The different letters on the histogram of winter wheat in the same year indicated the significant difference between different treatments at the 0.05 level, the same as below.
3.2.1 Leaf Photosynthetic Rate (Pn) of Winter Wheat
It can be seen from Table 2 and Fig. 5 that the LD60 treatment was higher than LD30, LD90, LD120, LD180, MD30, MD60, MD90, MD120, MD180, and CK2 by about 13.13%, 1.21%, 2.59%, 4.02%, 12.52%, 17.18%, 18.12%, 8.86%, 2.99%, and 33.04% (3-year average, p ≤ 0.05). With the increase of D, the Pn of winter wheat leaves decreased (p ≤ 0.05). Among them, when the lower limit of irrigation decreased from CK1 to CK2, the Pn of winter wheat leaves decreased by about 31.62%, 56.48%, and 57.26% (3-year average, p ≤ 0.05). Under the LD, with the increase of I, the Pn of winter wheat leaves increased first and then decreased. Compared with 30, 90, 120, and 180 mm, the Pn of winter wheat leaves with I of 60 mm increased by about 13.23%, 1.35%, 2.00%, and 4.41% (3-year average, p ≤ 0.05). Under the MD, with the increase of I, the Pn of winter wheat leaves showed an increasing trend. The greater the degree of D, the more the Pn of winter wheat leaves increased with the increase of I. This indicates that with the increase of D, large I (≥120 mm) helps to store water in the deep layer (>80 cm). When shallow soil volumetric water content (VWC) is low, the use of soil water potential difference can compensate for the shallow layer, ensuring the normal photosynthesis of winter wheat leaves.
3.2.2 Leaf Stomatal Conductance (Gs) of Winter Wheat
It can be seen from Table 3 and Fig. 6 that the Gs of winter wheat leaves treated with LD60 were higher than those of LD30, LD90, LD180, MD30, MD60, MD90, MD120, and MD180 (p ≤ 0.05). With the increase in D, the Gs of winter wheat leaves showed a decreasing trend, and the Gs of winter wheat leaves were the lowest under CK2 (p ≤ 0.05). The Gs of winter wheat leaves with an I of 60 mm under LD treatment were higher than those of 30, 90, and 180 mm (p ≤ 0.05). As the I increased under MD conditions, the Gs of winter wheat leaves with an I of 180 mm were 1.39, 1.30, 1.52, and 1.31 times higher (3-year average, p ≤ 0.05) than that of 60, 90, and 120 mm.
3.2.3 Leaf Intercellular CO2 Concentration (Ci) of Winter Wheat
It can be seen from Table 4 and Fig. 7 that the Ci value of winter wheat leaves under LD60 treatment varied greatly (46.62%, 3-year average, p ≤ 0.05) from 160 to 200 days after sowing. With the increase of D, the Ci of winter wheat leaves decreased (p ≤ 0.05). With the increase of I, the Ci of winter wheat leaves under LD treatment increased first and then decreased (p ≤ 0.05). With the increase of I, the Ci of winter wheat leaves under MD increased first, then decreased, and then increased (p ≤ 0.05).
3.2.4 Leaf Transpiration Rate (Tr) of Winter Wheat
It can be seen from Table 5 and Fig. 8 that the Tr of winter wheat leaves treated with LD60 was higher than that of LD30, LD90, LD120, LD180, MD30, MD60, MD90, MD120 and MD180 treatments (p ≤ 0.05). With the increase of D, the Tr of winter wheat leaves showed a decreasing trend (p ≤ 0.05). With the increase of I, the Tr of winter wheat leaves increased first and then decreased (p ≤ 0.05).
3.3 Effects of Different Treatments on Yield and Water Use Efficiency of Winter Wheat
This study indicates that factors D, I, and their interaction had significant effects on winter wheat yield, crop water consumption, and water use efficiency (WUE), as shown in Figs. 9 and 10. When compared with LD30, MD30, MD60, MD90, MD120, MD180 and CK2, the yield of winter wheat in the LD60 treatment was higher, increasing by about 3.23% (3-year average, p ≤ 0.05), 32.26%, 19.86%, 11.6%, 10.1%, 14.5%, and 72.4%. There was no significant decrease in yield when compared with CK1, LD90, LD120, and LD180. At the same time, the WUE of winter wheat treated with LD60 was significantly higher than that of CK1, LD90, LD120, LD180, MD30, MD60, MD90, MD120, MD180, and CK2, increasing by about 18.63% (3-year average, p ≤ 0.05), 15.3%, 8.59%, 26.2%, 4.86%, 2.99%, 15.5%, 19.9%, 50.6%, and 24.7%.
Figure 9: Effects of different treatments on yield and water use efficiency of winter wheat
Note: In this study, 12 treatments were repeated three times, a total of 36 experimental plots, each plot measured three replicates. The figures in the figure are average value ± standard error. 2019 represents winter wheat in 2019, 2020 represents winter wheat in 2020, 2021 represents winter wheat in 2021. The different letters on the histogram of winter wheat in the same year indicated the significant difference between different treatments at the 0.05 level, the same as below.
Figure 10: Effects of different treatments on crop water consumption of winter wheat
Note: In this study, 12 treatments were repeated three times, a total of 36 experimental plots, each plot measured three replicates. The figures in the figure are average value ± standard error. 2019 represents winter wheat in 2019, 2020 represents winter wheat in 2020, 2021 represents winter wheat in 2021. The different letters on the histogram of winter wheat in the same year indicated the significant difference between different treatments at the 0.05 level, the same as below.
With the increase of the D, the yield of winter wheat exhibited an increasing trend. The yield under the LD treatment was significantly higher than that under MD and CK2 by about 25.0% and 85.4%, respectively (3-year average, p ≤ 0.05). The Water Use Efficiency (WUE) of winter wheat under LD was significantly higher than that of CK2 by about 9.21% (3-year average, p ≤ 0.05). Therefore, the LD is a suitable choice. With the increase of the I, the yield of winter wheat increased (p ≤ 0.05), and the WUE initially increased and then decreased (p ≤ 0.05). In 2019, the yield of winter wheat with 60 mm I was 14.8% higher than that with 90 mm I (p ≤ 0.05). In 2021, the yield of winter wheat with 60 mm I was 10.3% higher than that with 90 and 180 mm treatments (p ≤ 0.05). In 2019, the WUE of winter wheat under the 60 mm treatment was significantly higher than that under the 180 and 90 mm treatments by about 7.45% and 16.41%, respectively (p ≤ 0.05). In 2020, the WUE of winter wheat under the 60 mm treatment was significantly higher than that under the 90, 120, and 180 mm treatments by about 28.1%, 14.2%, and 9.5%, respectively (p ≤ 0.05). The WUE of winter wheat in the 60 mm treatment was significantly higher than that of the 180, 120, 90 mm treatments by about 70.9%, 21.9%, and 16.8%, respectively (p ≤ 0.05), indicating that the appropriate I was 60 mm. After comprehensive consideration, the LD60 treatment can significantly improve WUE without significantly reducing the yield.
3.4 Relationship between Soil VWC, Pn, and Yield of Winter Wheat
The Pearson two-tailed test showed that there was a positive correlation between soil VWC and leaf Pn of winter wheat on different days after sowing. With the advancement of the winter wheat growth period, the correlation coefficient between soil VWC and Pn increased first and then decreased, and the correlation between soil VWC and Pn was higher at 180 days after winter wheat sowing. With the increase in soil depth, the correlation coefficient between soil VWC and Pn increased first and then decreased, and the peak value was 0.729. The correlation coefficient was higher in 20–40 cm soil layer, indicating that the soil VWC in 20–40 cm soil layer was closely related to the photosynthetic characteristics of winter wheat leaves. In order to further describe the relationship between Pn of winter wheat leaves and soil VWC at 20–40 cm soil depth 180 days after winter wheat sowing, regression analysis was used to analyze this relationship, as shown in Figs. 11a–11c. There was a positive correlation between leaf Pn and the yield of winter wheat. With the advance of the winter wheat growth period, the correlation between Pn and yield of winter wheat leaves at 180 days after sowing was the best [0.655 (2019, average value), 0.858 (2020), 0.581 (2021)], indicating that the photosynthetic product distribution of winter wheat leaves reached the best period about 180 days after winter wheat sowing. To further illustrate the relationship between leaf Pn and yield of winter wheat 180 days after winter wheat sowing, see Figs. 11d–11f.
Figure 11: Regression analysis between Pn and soil volumetric water content, and between grain yield and Pn
Note: 2019 represents winter wheat in 2019, 2020 represents winter wheat in 2020, and 2021 represents winter wheat in 2021. Pn represents the photosynthetic rate of winter wheat leaves.
The relationship between soil VWC and Pn of leaves in the 20–40 cm soil layer was a quadratic parabolic curve at 180 days after winter wheat sowing, and the determination coefficient R2 was over 0.5654. The relationship between the Pn of leaves and the yield of winter wheat at 180 days after sowing showed a quadratic parabolic curve, in which the determination coefficient R2 was over 0.5981. The yield was estimated by using the Pn of winter wheat leaves, and the production potential of winter wheat in this area could also be indirectly evaluated by the soil VWC of 20–40 cm soil layer at 180 days after winter wheat sowing.
4.1 Effect of Regulated Deficit Irrigation on Photosynthetic Characteristics of Winter Wheat
In this study, we observed that the photosynthetic parameter Pn of winter wheat leaves decreases proportional to an increase in D, revealing the impact of D on the photosynthetic characteristics of winter wheat. Specifically, we found that D triggers a reduction in the activity of the hydrogen peroxide enzyme and an increase in the activity of malondialdehyde. This, in turn, restricts the opening of stomata in the leaves [34,35], resulting in a decrease in PSII efficiency and photosynthetic quantum conversion efficiency [36,37]. This finding is consistent with the results of Shang et al.’s [38] study on winter wheat study on winter wheat, Liu et al.’s [39] study on cucumber, and Zhang et al.’s [40] study on cotton, indicating that the effect of drought stress on plant photosynthetic characteristics is consistent. Under LD conditions, the Pn of winter wheat leaves first increases and then decreases with an increase in I. This trend is caused by the difficulty of gas exchange between the deep soil and the atmosphere, which affects the growth and development of crop roots and decreases the photosynthetic characteristics of the leaves [41,42]. This difficulty in gas exchange is due to the lower degree of water deficiency, longer duration of mild drought stress, larger single irrigation amount, and higher water content in shallow soil. Under MD conditions, the Pn of winter wheat leaves increases with an increase in I. This further indicates that large-scale irrigation can store some water in the soil when the lower limit of irrigation is below 55% of the field water-holding capacity. When there is a shortage of water in the shallow soil in the later stage, deep soil water can be transported to the shallow soil through capillary action or absorbed by the roots of winter wheat, which helps meet the photosynthetic water demand of the leaves [43,44]. These results reveal the mechanism of the influence of water factors on the photosynthetic characteristics of winter wheat leaves. They also provide a scientific foundation for the management and optimization of irrigation and photosynthetic efficiency in winter wheat.
4.2 Effect of Regulated Deficit Irrigation on Yield and Water Use Efficiency of Winter Wheat
This study found that with the increase of D, the yield of winter wheat showed a decreasing trend. This may be due to the fact that drought stress can lead to a decrease in soil moisture. Under drought stress, crops produce more abscisic acid (ABA) in their root systems. The inability of the crop’s root system to absorb sufficient water for photosynthesis in the leaves, coupled with the abscisic acid produced by the root system, leads to a reduction in stomatal aperture, limiting gas exchange between the leaves and the external environment, thereby reducing the photosynthetic rate of the crop. The photosynthetic rate is one of the key factors in crop growth and yield formation, so drought stress directly affects the yield of winter wheat [45,46]. It may also be due to the fact that drought stress affects the physiological and metabolic processes of crops. For example, drought stress can induce water loss in crop leaves, leading to an increase in leaf temperature, which consequently affects photosynthesis [47]. Simultaneously, drought stress can trigger a series of stress responses in crops, such as the accumulation of reactive oxygen species and alterations in the activity of antioxidant systems, further affecting crop growth and yield. These findings are supported by other studies [48]. For example, Du et al. [28] found in their study on winter wheat that under drought stress, both the net photosynthetic rate (Pn) and yield of crops decreased. In addition, Yang et al.’s [49] studied drip-irrigated chili peppers and Eyup’s [50] studied furrow-irrigated melons, both showing that as drought stress increases, crop yield also decreases. Furthermore, Wang et al.’s [51] research also indicated that reducing irrigation volume has a negative impact on melon yield. These consistent results indicate that drought stress has a negative impact on grain crop yield. Therefore, optimizing irrigation volume at different growth stages can achieve water-saving and high-yielding winter wheat [52]. Further research and the development of efficient irrigation management strategies are crucial for ensuring sustainable crop production under drought conditions.
This study found that with an increase in irrigation amount, the yield of winter wheat initially increases and then decreases. This could be due to the fact that when the irrigation volume is below 60 mm, the soil’s volumetric water content is low, which exposes the crops to water stress. Water stress affects the growth and development of crops, resulting in a decrease in yield. When the irrigation volume is 60 mm, the soil’s volumetric water content is moderate, which can meet the growth needs of the crops and avoid water stress. This appropriate water utilization can increase crop yield. However, when the irrigation volume exceeds 60 mm, the soil’s filled porosity increases, which results in a decrease in soil aeration. This can lead to an oxygen deficiency in the soil, adversely affecting crop growth and photosynthesis, and consequently reducing yield [53–55]. The study also found that the Pn (net photosynthetic rate) of winter wheat leaves under the 60 mm treatment was higher than the 30-mm, 90-millimeter, 120-millimeter, and 180-millimeter treatments, with increases of 13.23%, 1.35%, 2.00%, and 4.41%, respectively (three-year average values). The lower photosynthetic rate of winter wheat leaves limits the increase in their yield [56,57]. This conclusion is consistent with Golzardi et al.’s [58] findings on corn and Agbna et al.’s [59] findings on tomatoes, suggesting that increasing irrigation volume within a certain range can increase crop yield. Previous studies have also indicated that moderately dry soil can inhibit stem elongation, promote root development, significantly increase the root-to-shoot ratio, without significantly reducing yield, and improve water use efficiency [60,61]. As irrigation volume increases, although crop yield can effectively increase, the increase in crop water consumption is greater than the increase in yield, resulting in a decrease in crop water use efficiency [62,63]. The yield of the independent treatment with extensive irrigation slightly decreases, which is due to the longer duration of extensive irrigation, causing temporary oxygen stress in the plant’s root system, and resulting in a decrease in cumulative crop yield [64].
This study found that LD60 treatment of winter wheat could significantly improve soil WUE while not significantly reducing yield. It may be due to the suitable soil hydrothermal environment of LD60 treatment, which positively promotes the interaction between crop roots and soil environment [65]. The suitable soil hydro-thermal environment is also beneficial to the increase of Pn of winter wheat leaves. The Pn of winter wheat leaves treated with LD60 was higher than that of LD30, LD90, LD120, LD180, MD30, MD60, MD90, MD120, MD180 and CK2 by 13.13%, 1.21%, 2.59%, 4.02%, 12.52%, 17.18%, 18.12%, 8.86%. 2.99%, and 33.04% (3-year average, p ≤ 0.05), respectively. The higher Pn provided a guarantee for the stable yield of winter wheat [66]. In this study, although the yield of LD60 treatment was lower than that of CK1, LD90, LD120, and LD180, the water consumption of LD60 treatment was smaller. Simultaneously, compared with LD30, MD30, MD60, MD90, MD120, MD180, and CK2 treatments, the increase of yield in LD60 treatment was less than that of water consumption, which led to the dual goal of stable yield and efficient water use of winter wheat in LD60 treatment.
4.3 Relationship between Soil VWC, Leaf Photosynthetic Characteristics, and Yield of Winter Wheat Under Regulated Deficit Irrigation
This study found that as the winter wheat growth period progresses, the soil moisture in each treatment exhibits a decreasing trend. This is because the water demand of winter wheat gradually increases during its growth, resulting in the rate of water absorption and evaporation by the crops surpassing the replenishment rate of soil moisture. As winter wheat grows, its root system gradually extends into deeper soil layers, thereby increasing the extraction of deep soil moisture. Furthermore, as the leaf area of winter wheat increases, transpiration also increases, leading to a rise in the evaporation rate of soil moisture [67]. With the increase in vegetation coverage, plant transpiration intensifies, resulting in reduced soil evaporation and a triangular distribution of the winter wheat root system. This makes the absorption of water by the crop’s root system the primary factor causing fluctuations in soil moisture, prompting changes in deep soil moisture [68,69]. Previous studies have indicated that during the filling and maturity stage of winter wheat, the external temperature is high, surface evaporation is significant, the shallow soil water potential is low, and the deep soil water potential is high [35]. Therefore, deep soil moisture can supply shallow soil moisture through micro-capillary action, which can conserve soil moisture and enhance the drought resistance of winter wheat.
This study found that the correlation between soil VWC and Pn of winter wheat leaves was strongest 180 days after winter wheat sowing. This could be attributed to the robust growth phase of winter wheat during this period. With well-developed roots and heightened transpiration, the crop becomes more sensitive to water deficits. Consequently, excessively high or low soil moisture can hinder the opening of the winter wheat’s Gs, thus reducing the Pn of the leaves [70,71]. The Pn of winter wheat leaves was positively correlated with yield, which was consistent with the conclusions of Yang et al. [72] and Wan et al.’s [71] studies on the relationship between photosynthetic parameters and yield of winter wheat. Due to the high soil VWC, the plant grows vigorously, the leaf area index is high, the leaf pigment content is high, and it is easy to accumulate photosynthetic dry matter and increase the yield [73]. The study further revealed a synergistic change between the volumetric water content of deep soil and the photosynthetic characteristics and yield of leaves. With the increase of D, the utilization rate of deep soil moisture by winter wheat increased gradually. Possibly due to the increase in deep soil volume containing water, more water supply can be provided to the root system of winter wheat. As a result, the root system of winter wheat can better absorb and utilize the water in the soil, thereby promoting leaf photosynthesis. Photosynthesis is the process by which plants convert carbon dioxide and water into organic matter using light energy [74]. This further contributes to an increase in winter wheat yield. Moreover, the root system of winter wheat can more efficiently absorb water and nutrients. When the utilization rate of deep soil moisture by winter wheat gradually increases, it can also enhance crop yield. This conclusion is consistent with Xu’s study, which found that a large amount of rainfall in summer can be stored in the deep soil and can be utilized by winter wheat with less rainfall during its growth period.
We conducted a systematic study on the impact of deficit irrigation on the photosynthetic characteristics and yield of winter wheat under different combinations of D (irrigation lower limits) and I (irrigation quota). Through our experiments, we found that under large quota irrigation (≥120 mm), as the degree of drought increased, the degree of drought stress actually decreased, while the use of deep soil water increased. Throughout the growth period, the photosynthetic rate (Pn) of winter wheat leaves exhibited an initial increase followed by a decrease. Notably, the Pn of winter wheat leaves under the LD60 treatment is better than other treatments, further indicating that moderate water deficits can enhance the photosynthetic efficiency of winter wheat. The WUE of winter wheat treated with LD60 was significantly higher than that of CK1, LD90, LD120, LD180, MD30, MD60, MD90, MD120, MD180, and CK2, increasing by about 18.63% (3-year average, p ≤ 0.05), 15.3%, 8.59%, 26.2%, 4.86%, 2.99%, 15.5%, 19.9%, 50.6%, and 24.7%. As D and I increased, the yield of winter wheat also increased, but the water use efficiency (WUE) first increased and then decreased. In addition, we found that soil VWC and Pn were positively correlated with winter wheat yield, further confirming the important impact of water and photosynthesis on winter wheat yield. Especially 180 days after winter wheat sowing, which is a critical period for yield formation. Considering all factors, to achieve the dual goals of stable yield of winter wheat in this area and efficient use of water resources, we recommend adopting the LD60 treatment as the appropriate deficit irrigation threshold for winter wheat. The findings of this study provide crucial references for the irrigation management of winter wheat and contribute new insights for the efficient use of water resources.
Acknowledgement: The authors sincerely thank the anonymous reviewers who made valuable comments on this paper.
Funding Statement: This work is supported jointly by the Natural Science Foundation of China (No. 41807041), the Ninth Batch of Key Disciplines in Henan Province—Mechanical Design, Manufacturing and Automation (JG [2018] No. 119).
Author Contributions: Formal analysis, H.Y.; Data curation, N.X.; Methodology, H.L.; Writing—review and editing, M.Z. All authors have read and agreed to the published version of the manuscript.
Availability of Data and Materials: The data that support the findings of this study are available from the corresponding authors upon reasonable request.
Ethics Approval: Not applicable.
Conflicts of Interest: The authors declared that they have no confilcts of interest to report regarding the present study.
References
1. Ren, P., Huang, F., Li, B. (2022). Spatiotemporal patterns of water consumption and irrigation require-ments of wheat-maize in the Huang-Huai-Hai Plain, China and options of their reduction. Agricultural Water Management, 263, 107468. [Google Scholar]
2. Li, J., Zhang, Z., Liu, Y., Yao, C., Song, W. et al. (2019). Effects of micro-sprinkling with different irrigation amount on grain yield and water use efficiency of winter wheat in the North China Plain. Agricultural Water Management, 224, 105736. [Google Scholar]
3. Zhang, X., Pei, D., Chen, S., Sun, H., Yang, Y. (2006). Performance of double-cropped winter wheat-summer maize under minimum irrigation in the North China Plain. Agronomy Journal, 98, 1620–1626. [Google Scholar]
4. Xing, W., Wang, W., Shao, Q., Ding, A. Y. (2018). Estimating net irrigation requirements of winter wheat across central-eastern China under present and future climate scenarios. Journal of Irrigation and Drainage Engineering, 144, 5018005–5018018. [Google Scholar]
5. Shirazi, S. Z., Mei, X., Liu, B., Liu, Y. (2022). Estimating potential yield and change in water budget for wheat and maize across Huang-Huai-Hai Plain in the future. Agricultural Water Management, 260, 107282. [Google Scholar]
6. Liu, J., Sun, B., Shen, H., Ding, P., Ning, D. et al. (2022). Crop water requirement and utili-zation efficiency-based planting structure optimization in the Southern Huang-Huai-Hai Plain. Agronomy, 12, 2219. [Google Scholar]
7. Sun, H., Zhang, X., Liu, X., Liu, X., Shao, L. et al. (2019). Impact of different crop-ping systems and irrigation schedules on evapotranspiration, grain yield and groundwater level in the North China Plain. Agricultural Water Management, 211, 202–209. [Google Scholar]
8. Guan, D., Al-Kaisi, M. M., Zhang, Y., Duan, L., Tan, W. et al. (2014). Tillage practices affect biomass and grain yield through regulating root growth, root-bleeding sap and nutrients uptake in summer maize. Field Crop Research, 157, 89–97. [Google Scholar]
9. Chilundo, M., Joel, A., Wesström, I., Brito, R., Messing, I. (2017). Response of maize root growth to irrigation and nitrogen management strategies in semi-arid loamy sandy soil. Field Crop Research, 200, 143–162. [Google Scholar]
10. Wang, Y., Chen, S., Zhang, D., Yang, L., Cui, T. et al. (2020). Effects of subsoiling depth, period interval and combined tillage practice on soil properties and yield in the Huang-Huai-Hai Plain, China. Journal of Integrative Agriculture, 19, 1596–1608. [Google Scholar]
11. Feng, S., Gu, S., Zhang, H., Wang, D. (2017). Root vertical distribution is important to improve water use efficiency and grain yield of wheat. Field Crop Research, 214, 131–141. [Google Scholar]
12. Wang, J., Gong, S., Xu, D., Yu, Y. (2011). Effects of irrigation models on the space distribution of root system and yield of winter wheat. Journal of Hydraulic Engineering, 42, 1239–1246. [Google Scholar]
13. Xu, X., Zhang, M., Li, J., Liu, Z., Zhao, Z. et al. (2018). Improving water use efficiency and grain yield of winter wheat by optimizing irrigations in the North China Plain. Field Crop Research, 221, 219–227. [Google Scholar]
14. Jiang, Y., Wang, B., Xie, B., Zhang, H., Duan, W. et al. (2016). Analysis of antioxidant characteristics in seedling roots of sweetpotato (Ipomoea batatas (L.) Lam.) with different drought tolerance under simulated drought stress. Agricultural Science & Technology, 17(2), 245–250. [Google Scholar]
15. Lu, H., Xue, J., Guo, D. (2017). Efficacy of planting date adjustment as a cultivation strategy to cope with drought stress and increase rainfed maize yield and water-use efficiency. Agricultural Water Management, 179(1), 227–235. [Google Scholar]
16. Ren, S., Guo, B., Wu, X., Zhang, L., Ji, M. et al. (2021). Winter wheat planted area monitoring and yield modeling using MODIS data in the Huang-Huai-Hai Plain, China. Computers and Electronics in Agriculture, 182, 106049. [Google Scholar]
17. Eissa, M. A., Roshdy, N. M. K. (2018). Effect of nitrogen rates on drip irrigated maize grown under deficit irrigation. Journal of Plant Nutrition, 42(2), 127–136. [Google Scholar]
18. Kamran, M., Yan, Z., Ahmad, I., Jia, Q., Ghani, M. U. et al. (2023). Assessment of greenhouse gases emissions, global warming potential and net ecosystem eco-nomic benefits from wheat field with reduced irrigation and nitrogen management in an arid region of China. Agriculture, Ecosystems & Environment, 341, 108197. [Google Scholar]
19. Wang, P., Song, X., Han, D., Zhang, Y., Zhang, B. (2012). Determination of evaporation, transpiration and deep percolation of summer corn and winter wheat after irrigation. Agricultural Water Management, 105, 32–37. [Google Scholar]
20. Zheng, L. J., Ma, J. J., Sun, X. H., Guo, X. H., Jiang, J. et al. (2018). Responses of photosynthesis, dry mass and carbon isotope discrimination in winter wheat to different irrigation depths. Photosynthetica, 56, 1437–1446. [Google Scholar]
21. Greaves, G. E., Wang, Y. (2017). Effect of regulated deficit irrigation scheduling on water use of corn in southern Taiwan tropical environment. Agricultural Water Management, 188, 115–125. [Google Scholar]
22. Wang, C., Qi, Z., Zhao, J., Gao, Z., Zhao, J. et al. (2023). Sustainable water and nitrogen optimization to adapt to different temperature variations and rainfall patterns for a trade-off between winter wheat yield and N2O emissions. Science of the Total Environment, 854, 158822. [Google Scholar] [PubMed]
23. Yu, R., Wang, L., Yang, X., Chen, L., Jiang, Q. et al. (2019). Soil moisture dynamics and physiological characteristics of moving of Caragana intermedia. Acta Ecologica Sinica, 39(19), 7249–7257. [Google Scholar]
24. Lang, Y., Wang, M., Zhang, G. C., Zhao, Q. K. (2013). Experimental and simulated light responses of photosynthesis in leaves of three tree species under different soil water conditions. Photosynthetica, 51, 370–378. [Google Scholar]
25. Ali, S., Xu, Y., Jia, Q., Ahmad, I., Wei, T. et al. (2018). Cultivation techniques combined with deficit irrigation improves winter wheat photosynthetic characteristics, dry matter translocation and water use efficiency under simulated rainfall conditions. Agricultural Water Management, 201, 207–218. [Google Scholar]
26. Qin, W., Zhang, X., Chen, S., Sun, H., Shao, L. (2018). Crop rotation and N application rate affecting the performance of winter wheat under deficit irrigation. Agricultural Water Management, 210, 330–339. [Google Scholar]
27. Geng, S., Tan, J., Li, L., Miao, Y., Wang, Y. (2023). Legumes can increase the yield of subsequent wheat with or without grain harvesting compared to Gramineae crops: A meta-analysis. European Journal of Agronomy, 142, 126643. [Google Scholar]
28. Du, T., Kang, S., Sun, J., Zhang, X., Zhang, J. (2010). An improved water use efficiency of cereals under temporal and spatial deficit irrigation in North China. Agricultural Water Management, 97, 66–74. [Google Scholar]
29. Zhou, L., Xue, B., Meng, F., Li, J., Zang, W. et al. (2018). Effects of irrigation quota and irrigation frequency on yield and quality of winter wheat under sprinkler irrigation system. Chinese Society for Agricultural Machinery, 49, 235–243. [Google Scholar]
30. Wang, D., Yu, Z., White, P. J. (2013). The effect of supplemental irrigation after jointing on leaf senescence and grain filling in wheat. Field Crop Research, 151, 35–44. [Google Scholar]
31. Liu, X., Shao, L., Sun, H., Chen, S., Zhang, X. (2013). Responses of yield and water use efficiency to irrigation amount decided by pan evaporation for winter wheat. Agricultural Water Management, 129, 173–180. [Google Scholar]
32. Zhang, C., Xie, Z., Wang, Q., Tang, M., Feng, S. et al. (2022). AquaCrop modeling to explore optimal irrigation of winter wheat for improving grain yield and water productivity. Agricultural Water Management, 266, 107580. [Google Scholar]
33. Zhang, M., Xiao, N., Yang, H., Li, Y., Gao, F. et al. (2023). The layout measures of micro-sprinkler irrigation under plastic film regulate tomato soil bacterial community and root system. Frontiers in Plant Science, 14, 1–17. [Google Scholar]
34. Franks, P. J., Drake, P. L., Beerling, D. J. (2009). Plasticity in maximum stomatal conductance constrained by negative correlation between stomatal size and density: An analysis using Eucalyptus globulus. Plant, Cell & Environment, 32, 1737–1748. [Google Scholar]
35. Luo, H. H., Zhang, Y. L., Zhang, W. F. (2016). Effects of water stress and rewatering on photosynthesis, root activity, and yield of cotton with drip irrigation under mulch. Photosynthetica, 54, 65–73. [Google Scholar]
36. Subrahmanyam, D., Subash, N., Haris, A., Sikka, A. K. (2006). Influence of water stress on leaf photosynthetic characteristics in wheat cultivars differing in their susceptibility to drought. Photosynthetica, 44, 125–129. [Google Scholar]
37. Zhang, Y., Wang, J., Gong, S., Xu, D., Mo, Y. et al. (2021). Straw mulching improves soil water content, increases flag leaf photosynthetic parameters and maintaines the yield of winter wheat with different irriga-tion amounts. Agricultural Water Management, 249, 106809. [Google Scholar]
38. Shangguan, Z., Shao, M., Dyckmans, J. (2000). Effects of nitrogen nutrition and water deficit on net photosynthetic rate and chlorophyll fluorescence in winter wheat. Journal of Plant Physiology, 156, 46–51. [Google Scholar]
39. Liu, B. B., Li, M., Li, Q. M., Cui, Q. Q., Zhang, W. D. et al. (2018). Combined effects of elevated CO2 concentration and drought stress on photosynthetic performance and leaf structure of cucumber (Cucumis sativus L.) seedlings. Photosynthetica, 56, 942–952. [Google Scholar]
40. Zhang, C., Zhan, D. X., Luo, H. H., Zhang, Y. L., Zhang, W. F. (2016). Photorespiration and photoinhibition in the bracts of cotton under water stress. Photosynthetica, 54, 12–18. [Google Scholar]
41. Mustroph, A., BardingJr, G. A., Kaiser, K. A., Larive, C. K., Bailey-serres, J. (2014). Characterization of distinct root and shoot responses to low-oxygen stress in Arabidopsis with a focus on primary C- and N-metabolism. Plant, Cell & Environment, 37(10), 2366–2380. [Google Scholar]
42. Olckers, S., Osthoff, G., Guzmán, C., Wentzel, B., van Biljon, A., et al. (2022). Drought and heat stress effects on gluten protein composition and its relation to bread-making quality in wheat. Journal of Cereal Science, 108, 103562. [Google Scholar]
43. Mair, A., Dupuy, L., Ptashnyk, M. (2023). Can root systems redistribute soil water to mitigate the effects of drought? Field Crop Research, 300, 109006. [Google Scholar]
44. Wang, H., Fan, J., Fu, W., Du, M., Zhou, G. et al. (2022). Good harvests of winter wheat from stored soil water and improved temperature during fallow period by plastic film mulching. Agricultural Water Management, 274, 107910. [Google Scholar]
45. Yin, X., Feng, Q., Liu, W., Zhu, M., Zhang, J. et al. (2023). Assessment and mechanism analysis of plant salt tolerance regulates soil moisture dynamics and controls root zone salinity and sodicity in seasonally irrigated agroecosystems. Journal of Hydrology, 129138(2), 129138. [Google Scholar]
46. Li, Y., Liu, N., Fan, H., Su, J., Fei, C. et al. (2019). Effects of deficit irrigation on photosynthesis, photosynthate allocation, and water use efficiency of sugar beet. Agricultural Water Management, 223, 105701. [Google Scholar]
47. Ru, C., Hu, X., Chen, D., Wang, W., Zhen, J. (2023). Photosynthetic, antioxidant activities, and osmoregulatory responses in winter wheat differ during the stress and recovery periods under heat, drought, and combined stress. Plant Science, 327, 111557. [Google Scholar] [PubMed]
48. Hlaváčová, M., Klem, K., Rapantová, B., Novotná, K., Urban, O. et al. (2018). Interactive effects of high temperature and drought stress during stem elongation, anthesis and early grain filling on the yield formation and photosynthesis of winter wheat. Field Crop Research, 221(9), 182–195. [Google Scholar]
49. Yang, H., Liu, H., Zheng, J., Huang, Q. (2018). Effects of regulated deficit irrigation on yield and water productivity of chili pepper (Capsicum annuum L.) in the arid environment of Northwest China. Irrigation Science, 36, 61–74. [Google Scholar]
50. Koksal, E. S., Tasan, M., Artik, C., Gowda, P. (2017). Evaluation of financial efficiency of drip-irrigation of red pepper based on evapotranspiration calculated using an iterative soil water-budget approach. Scientia Horticulturae, 226, 398–405. [Google Scholar]
51. Wang, J., Huang, G., Li, J., Zheng, J., Huang, Q. et al. (2017). Effect of soil moisture-based furrow irrigation scheduling on melon (Cucumis melo L.) yield and quality in an arid region of Northwest China. Agricultural Water Management, 179, 167–176. [Google Scholar]
52. Wang, Y., Zhang, Y., Zhang, R., Li, J., Zhang, M. et al. (2018). Reduced irrigation increases the water use efficiency and productivity of winter wheat-summer maize rotation on the North China Plain. Science of Total Environment, 618, 112–120. [Google Scholar]
53. Li, Z., Wan, S., Chen, G., Han, Y., Lei, Y. et al. (2023). Effects of irrigation regime on soil hydrothermal microenvironment, cotton biomass, and yield under non-film drip irrigation system in cotton fields in Southern Xinjiang, China. Industrial Crops and Products, 198, 116738. [Google Scholar]
54. Zhu, Y., Dyck, M., Cai, H., Song, L., Chen, H. (2019). The effects of aerated irrigation on soil respiration, oxygen, and porosity. Journal of Integrative Agriculture, 18(12), 2854–2868. [Google Scholar]
55. Fan, Y., Liu, J., Zhao, J., Ma, Y., Li, Q. (2019). Effects of delayed irrigation during the jointing stage on the photosynthetic characteristics and yield of winter wheat under different planting patterns. Agricultural Water Management, 221, 371–376. [Google Scholar]
56. Chen, X., Qi, Z., Gui, D., Sima, M. W., Zeng, F. et al. (2022). Responses of cotton photo-synthesis and growth to a new irrigation control method under deficit irrigation. Field Crop Research, 275(11), 108373. [Google Scholar]
57. Zhang, Z., Yu, Z. W., Shi, Y., Zhang, Y. L. (2023). Effects of micro-sprinkling with different irrigation levels on winter wheat grain yield and greenhouse gas emissions in the North China Plain. European Journal of Agronomy, 143(9), 126725. [Google Scholar]
58. Golzardi, F., Baghdadi, A., Afshar, R. K. (2017). Alternate furrow irrigation affects yield and water-use efficiency of maize under deficit irrigation. Crop and Pasture Science, 68(8), 726–734. [Google Scholar]
59. Agbna, G. H. D., Dongli, S., Zhipeng, L., Elshaikh, N. A., Guangcheng, S. et al. (2017). Effects of deficit irrigation and biochar addition on the growth, yield, and quality of tomato. Scientia Horticulturae, 222(11), 90–101. [Google Scholar]
60. Kang, S., Shi, W., Zhang, J. (2000). An improved water-use efficiency for maize grown under regulated deficit irrigation. Field Crop Research, 67(3), 207–214. [Google Scholar]
61. Javed, A., Ahmad, N., Ahmed, J., Hameed, A., Ashraf, M. A. et al. (2022). Grain yield, chlorophyll and protein contents of elite wheat genotypes under drought stress. Journal of King Saud University-Science, 34(7), 102279. [Google Scholar]
62. Kheir, A. M. S., Alrajhi, A. A., Ghoneim, A. M., Ali, E. F., Magrashi, A. et al. (2021). Modeling deficit irrigation-based evapotranspiration optimizes wheat yield and water productivity in arid regions. Agricultural Water Management, 256(32), 107122. [Google Scholar]
63. You, Y., Song, P., Yang, X., Zheng, Y., Dong, L. et al. (2022). Optimizing irrigation for winter wheat to maximize yield and maintain high-efficient water use in a semi-arid environment. Agricultural Water Management, 273(1), 107901. [Google Scholar]
64. Devkota, K. P., Yadav, S., Humphreys, E., Kumar, A., Kumar, P. et al. (2021). Land gradient and configuration effects on yield, irrigation amount and irrigation water productivity in rice-wheat and maize-wheat cropping systems in Eastern India. Agricultural Water Management, 255, 107036. [Google Scholar] [PubMed]
65. Wang, J., Li, Y., Niu, W. (2021). Effect of alternating drip irrigation on soil gas emissions, microbial community composition, and root-soil interactions. Agricultural Water Management, 256(5), 107123. [Google Scholar]
66. Xu, J., Zhao, H., Wang, S., Zheng, Y., Mai, B. et al. (2023). Assessment of photosynthesis and yield loss of winter wheat under ground-level ozone exposure. Environmental Technology & Innovation, 29(4), 103013. [Google Scholar]
67. Yang, D., Li, S., Wu, M., Yang, H., Zhang, W. et al. (2023). Drip irrigation improves spring wheat water productivity by reducing leaf area while increasing yield. European Journal of Agronomy, 143(5), 126710. [Google Scholar]
68. Liu, C., Yan, H., Wang, W., Han, R., Li, Z. et al. (2023). Layered application of phosphate fertilizer increased winter wheat yield by promoting root proliferation and phosphorus accumulation. Soil and Tillage Research, 225, 105546. [Google Scholar]
69. Cui, M., Chen, X., Yin, F., Xia, G., Yi, Y. et al. (2022). Hybridization affects the structure and function of root microbiome by altering gene expression in roots of wheat introgression line under saline-alkali stress. Science of Total Environment, 835, 155467. [Google Scholar]
70. Maseka, P., Sarcheshmehpour, M., Solaiman, Z. M. (2022). Influence of aged wood biochar on mycor-rhizal colonisation, growth and leaf gas exchange of wheat and clover with different water regimes. Agronomy, 12(10), 2420. [Google Scholar]
71. Wan, W., Zhao, Y., Xu, J., Liu, K., Guan, S. et al. (2022). Reducing and delaying nitrogen recommended by leaf critical SPAD value was more suitable for nitrogen utilization of spring wheat under a new type of drip-irrigated system. Agronomy, 12(10), 2331. [Google Scholar]
72. Yang, D. Q., Dong, W. H., Luo, Y. L., Song, W. T., Cai, T. et al. (2018). Effects of nitrogen application and supplemental irrigation on canopy temperature and photosynthetic characteristics in winter wheat. The Journal of Agricultural Science, 156(1), 13–23. [Google Scholar]
73. Tang, Y., Zhang, J., Wang, L., Wang, H., Long, H. et al. (2023). Water deficit aggravated the inhibition of photosynthetic performance of maize under mercury stress but is alleviated by brassinosteroids. Journal of Hazardous Materials, 443(1), 130365. [Google Scholar] [PubMed]
74. Wang, H., Zheng, C., Ning, S., Cao, C., Li, K. et al. (2023). Impacts of long-term saline water irrigation on soil properties and crop yields under maize-wheat crop rotation. Agricultural Water Management, 286, 108383. [Google Scholar]
Cite This Article
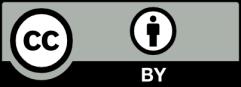