Open Access
ARTICLE
Integrated Use of Organic and Bio-fertilizers to Improve Yield and Fruit Quality of Olives Grown in Low Fertility Sandy Soil in an Arid Environment
1
Biology Department, College of Science, Jouf University, Sakaka, 72341, Saudi Arabia
2
Horticulture Department, Faculty of Agriculture, Zagazig University, Zagazig, 44511, Egypt
3
Horticulture Department, (pomology), Faculty of Agriculture, Suez Canal University, Ismailia, 41522, Egypt
* Corresponding Author: Bassam F. Alowaiesh. Email:
Phyton-International Journal of Experimental Botany 2023, 92(6), 1813-1829. https://doi.org/10.32604/phyton.2023.026950
Received 05 October 2022; Accepted 05 January 2023; Issue published 11 April 2023
Abstract
Olive productivity should be improved through stimulating nutrition, particularly under poor fertility soils. Consequently, the objective of this study was to assess the efficacy of applying organic and bio-fertilizers on the physiological growth, yield and fruit quality of olive trees under newly reclaimed poor-fertility sandy soil in an arid environment. During a field experiment carried out at El-Qantara, North Sinai, Egypt over two consecutive seasons (2019–2020 and 2020–2021), olive Kalamata trees were evaluated under three organic fertilizer treatments alone or in combination with three bio-fertilizers treatments. Organic fertilizer was applied as goat manure (16.8 kg/tree/year), or olive pomace (8.5 kg/tree/year) in mid-December of each season vs. untreated trees. The bio-fertilizers were applied as N-fixing bacteria (150 g/tree) was inculated in early March of each season, or amino acid mixture (1.5%) was applied three times, at 70% of full bloom, 21 days after full bloom, and a month later in comparison to a non-fertilized trees (control). The cultivar used was Kalamata, a dual-purpose cultivar for oil and table olives whose value increases when processed as table olives. The results indicated that the goat manure followed by olive pomace significantly enhanced photosynthetic pigments (chlorophyll a, b, and carotenoids), leaf mineral contents (N, P, K, Ca, Mg and Fe), tree canopy volume, number of flowers per inflorescence, number of inflorescences per shoot, initial fruit set, fruit retention. For fruit quality, fruit length and width, fruit weight, and total fruit yield was increased compared to the non-fertilized control. Likewise, The bio-fertilizer N-fixing bacteria followed by the amino acid mixture significantly improved all of the aforementioned parameters. Accordingly, it is recommended, both environmentally and economically to utilize organic and bio-fertizers, particularly goat manure combined with N-fixing bacteria, in low-fertility soil to sustain olive production as well as reducing mineral fertilization.Keywords
Olives (Olea europaea L.) is an evergreen tree that belong to the family Oleaceae growing in a dry-subtropical climate [1]. It is a vital industrial crop in the Mediterranean region and has substantial economic and agricultural concerns [2]. The consumption of oil and table olives has gradually increased due to the fast-growing worldwide population. The total olives world cultivated area in 2022 was about 12.76 million hectares producing 23.64 million tonnes annually [3]. Egypt is involved in this statistic with 100,826 ha producing 932,927 tonnes with an average of 9,253 kg/ha [3]. Egypt is a major olive producer and a leader in olive cultivation in arid and semi-arid conditions, particularly in desert lands.
There are several olive cultivars for oil production or table olives, as well as several dual-purpose cultivars [4]. Kalamata is a dual-purpose cultivar of oil and table olives, but its value is higher when processed as table olives because it is considered as the best table olives in the world [5]. It is one of the most famous olive cultivars due to its attractive and deep reddish-purple fruits. Lately, the consumption of olive oil and table olives has gradually increased due to the fast-growing worldwide population. Hence, its productivity should be increased to reduce the gap between production and consumption.
Olive groves are commonly established in the newly reclaimed poor-fertility soils in the Mediterranean region [6]. The newly reclaimed soils have low biological and chemical properties as well as a lack of available mineral nutrients [7]. Consequently, agricultural production in newly reclaimed soils faces great challenges and yield low-crop productivity [8,9]. The deficiency of mineral nutrients is compensated by mineral fertilization. Olive tree growth depends on mineral components. According to the findings of a study by Yermiyahu et al. [10], the application of N and P may be advantageous because those nutrients’ availability is determined by how vigorously olive trees blossom. K is seen as being very crucial in the production of olives [11], and there is a strong association between K and yield. Additionally, significant Ca requirements are needed for the flowering process and reproductive tissues; foliar treatments of this element in combination with Mg might directly affect yield, and this requirement probably increases throughout the flowering stage [12]. Different N levels were applied to the “Barnea” olive orchard, which resulted in a rise in the content of free fatty acids, an increase in palmitoleic, linoleic, and linolenic fatty acids, and a decrease in the level of polyphenols in the olive oil. on the other hand, oleic acid, the monounsaturated-to-polyunsaturated fatty acid ratio, and the oleic-to-linoleic ratio decreased. N, P, or K levels had no impact on the peroxide value [13]. Calcium spraying treatment during the growing season increased the oil content and the concentration of phenol compounds in fruits of Manzanilla de Sevilla and Ascolanta tenera olive cultivars [14,15].
Continuous application of chemical fertilization leads to the decline of soil fertility and properties and might increase heavy metals in plant materials, impacting the edibility and nutritional value [16]. On the other hand, sustainable agriculture aims to reduce mineral fertilization, a major global challenge. Hence, it has become essential to find alternative eco-friendly and economical sources of fertilizers to enhance poor soils.
Organic fertilization depends on using natural inputs such as decaying remains of organic matter, animal manure, and excess crops [17]. Organic fertilizer contains a high concentration of elements that can maintain soil fertility and secure sustainable nutrients for plant growth and productivity [18,19]. It improves soil fertility, structure, physical characteristics, and moisture retention [20]. Besides, it adjusts soil pH, aggregates soil stability, and provides plants with required minerals that are not mostly found in chemical fertilization [21]. Furthermore, it has a great advantage in sandy soil by increasing soil organic matter, reducing wind and water erosion, water-holding capacity, providing a source of slow-release nutrients, and promoting the growth of earthworms and other beneficial soil organisms [22,23]. Organic fertilization improves soil microorganisms, which play an essential role as a buffering agent against undesirable fluctuations of soil pH [24,25]. Organic fertilizers and nutrients are important to plants and can be used safely on soil, crops, and the environment.
Additionally, it also boosts cation exchange capacity and provides growth hormones like auxins [26]. Accordingly, these characteristics enhance its suitability as amendments in low-fertility soils. Hence the use of organic fertilizers has a greater relative economic benefit than mineral fertilization in newly reclaimed low-fertility sandy soils.
Biofertilizer contains beneficial microorganisms that promote plant growth by maintaining soil productivity and providing nutrients [27,28]. It assists in accessing nutrients such as nitrogen, phosphorus, and potassium from organic fertilizers, enhancing plant health and increasing salt and drought tolerance [29]. Biofertilizer is a promising approach to decreasing the use of conventional mineral fertilizers, particularly under current climate change [30,31]. Moreover, biofertilizer provides biodegradable substances, boosts soil stability and fertility, recycles nutrients, stimulates mycorrhiza symbiosis, mitigates soil contamination, develops bioremediation processes, and enhances biological control and antagonism of phytopathogenic organisms. Accordingly, applying biofertilizers increases plant growth, productivity, and fruit parameters and reduces mineral fertilization [32–34].
Biofertilizers application in combination with antioxidants gave a promotive effect on yield, fruit quality, and flesh oils content of the Chemlali olive cultivar [35]. Higher concentrations of N, K, and Na were found in the leaves of Chemlali olive trees after the application of biofertilizers rich in NPK. While olive trees treated with biofertilizers rich in NPK and Ca had higher chlorophyll concentrations. However, all foliar biofertilizer treatments led to a decrease in phenolic compounds in flower buds [36].
For these reasons, the present study aimed to investigate the combined effect of different organic and bio-fertilization sources on physiological parameters, leaf mineral contents, growth parameters, flowering, and fruit yield of kalamata olive trees grownunder low-fertility sandy soil in an arid environment.
2.1 Experimental Site and Agricultural Practices
A field experiment was conducted at El-Qantara, North Sinai, Egypt (30° 53′ 09″ N, 32° 05′ 04″ E) over two consecutive seasons (2020 and 2021). Tables 1 and 2 show that the experimental site has an arid climate, low precipitation, and sandy soil (83.92% sand, 7.36% clay, and 8.71% silt). Also, chemical analysis of irrigated water of the used well at the experimental site is presented in Table 3. Ten-year-old bearing Kalamata olive trees cultivated at 6 m × 7 m apart was used. Healthy trees having nearly uniform growth, vitality, and physical properties, a drip irrigation system, and recommended horticultural practices for growing olives were chosen for this study. The experimental design was factorial randomized complete block design with three replications for each treatment, and three trees represented each replication. The applied nitrogen for the olive tree was 250 g N/tree/year alongside the organic and biofertilizer applications.
2.2 Organic and Biofertilizer Applications
Two different types of organic fertilizers (goat manure and olive pomace) were examined. Organic fertilizers were brought from private goat farms and olive pomace from olive pressing factory. The chemical analysis of tested organic fertilizer materials is presented in Table 4. Goat manure and olive pomace fertilizers were used at one level for each (goat manure at 16.8 kg/tree/year, olive pomace at 8.5 kg/tree/year, and untreated trees (control). In mid-December of each season, the organic fertilizer sources were performed in two trenches dinged on both sides of the Kalamata olive tree at 40 cm depth and at 75 cm apart from the trunk and covered with trench soil.
These organic fertilizer materials were tested only or in combination with two types of bio-fertilizers [Nitrogen-fixing (N-fixing, contained Azospirillum spp. and Azotobacter chroococcum) bacteria at 150 g/tree, and amino acid mixture]. Bio-fertilizers used in the study were obtained from National Research Center, Giza, Egypt. The N-fixing bacteria fertilizer was applied in early March of each season, in trenches (45 cm length × 25 cm width × 20 cm depth) beneath the tree, whether organic fertilizers were provided or not. The amino acid mixture was applied from a commercial compound named protamine contains 16% free amino acids (alanine, arginine, aspartic, glutamic, glycine, histidine, leucine, lysine, serine, threonine, tryptophan, tyrosine, valine, and proline), 9.8% organic nitrogen, and 3.4% potassium oxide. The applied amino acid mixture was dissolved at 1.5% (v/v) and made up to one liter. It was then added to the soil under ‘Kalamata’ olive trees, where organic fertilizers under study were either added or not. The bio-fertilizers amino acid mixture was applied three times throughout the growing season three times at 70% full bloom (late April), 21 days after full bloom (fruit set stage), and a month later. In addition, non-fertilized trees were used as controls. A multiple of 81 uniform, healthy, and vigorous trees were selected [9 treatments (combinations of three organic fertilizer treatments with three bio-fertilizer treatments) × 3 replicates × 3 trees/replicate].
2.3.1 Physiological Parameters
Ten mature leaves were collected from the middle of new shoot growth during late September of both seasons. Chlorophyll a and b and carotenoids were determined following the method of Fadeel [37]. Leaf samples were dried at 70°C until constant weight then ground to a powder for mineral content determination. A suitable sample (0.2 g) was taken from each dried ground leaf and wet digested using a mixture of Perchloric acid: Sulphuric acid (1:4 v/v) until a clear solution. Thereafter, in each leaf sample, the content of nitrogen (%), phosphorus (%), potassium (%), calcium (%), magnesium (%), and iron (ppm) was determined [38].
2.3.2 Growth, Yield, and Fruit Parameters
Tree canopy volume was measured using the following equation:
where D1 and D2 are the diameters of the tree crown in two distinct directions, Ht is tree height, and Hs is the distance of the lowest point of the tree crown from the soil. Ten shoots were chosen randomly on each tree during full bloom (late April) and labeled to determine the number of flowers/inflorescences and number of inflorescences/shoots. The number of fruitlets and fruits was counted at monthly intervals up to harvest. The percentage of fruits set was determined using the following equation:
Fruit retention was recorded based on the initial number of fruit set and the total number of fruitlet drop on the other during each season. Fruits harvesting was performed in November at the ripening stage, and 75% of olive fruits reached the violet skin color (the suitable stage for olive extraction). The fruits of each tree were weighed (kg). Fruit length (cm) and fruit width (cm) were recorded using a vernier caliper. Fresh weight (g) and stone weight (g) of olive fruits was determined for all fruit samples.
R software (version 4.1.1) was used for performing all statistical analyses. The combined analysis of variance was applied to explore the differences among the organic fertilizer, biofertilizer, and their interactions in the two seasons. The differences among the assessed treatments were separated using the protected Tukey’s HSD test at a significance level of p ≤ 0.05. The heatmap was implemented to explore the relationship among evaluated traits in R software using RColorBrewer.
The evaluated organic and bio-fertilizer applications and their interaction displayed significant impacts on most evaluated physiological parameters during the two seasons 2020 and 2021 (Tables 5 and 6). Both assessed organic fertilizers; olive pomace and goat manure substantially promoted photosynthetic pigments chlorophyll a, chlorophyll b, and carotenoids (Table 5) and leaf mineral contents N, P, K, Ca, Mg, and Fe (Table 6) compared with the non-fertilized control, with superiority of goat manure in both seasons. Likewise, both evaluated bio-fertilizer, N-fixing bacteria, and amino acid considerably elevated photosynthetic pigments and leaf mineral contents compared with untreated control with superiority of N-fixing bacteria in both seasons (Tables 5 and 6). There was a significant interaction effect between organic and bio-fertilizers on all evaluated photosynthetic pigments and leaf mineral contents in both seasons. The lowest values in all evaluated photosynthetic pigments and leaf mineral contents were recorded by untreated control in both studied factors during both seasons (Tables 5 and 6). The interaction of goat manure treatment (16.8 kg/tree) with amino acids mixture (1.5%) displayed the highest enhancement in the content of chlorophyll a, chlorophyll b, and carotenoids in olive ‘Kalamata’ leaves by 24.86%, 52.18% and 85.59% respectively in the first season and 31.54%, 57.53% and 218.03% in the second season compared to the untreated control. Similarly, the treatment of goat manure combined with N-fixing bacteria improved the contents of N, P, K, Ca, Mg and Fe by 29.79%, 9.64%, 13.23%, 25.0%, 11.76%, and 26.85% in the first season and 16.77%, 6.74%, 8.99%, 28.57%, 26.67%, and 26.72% in the second season in comparison with untreated control trees.
3.2 Growth, Yield, and Fruit Parameters
Tree canopy volume, number of flowers per inflorescence, number of inflorescences per shoot, initial fruit set, fruit retention, fruit length, fruit width, fruit fresh weight, and total fruit yield significantly differed between the tested treatments and their interaction in both seasons (Tables 7 and 8). The evaluated organic fertilizers, olive pomace, and goat manure substantially ameliorated all the parameters mentioned above compared with that of the non-fertilized trees with the superiority of goat manure (16.8 kg/tree/year) in most traits in the two seasons of 2020 and 2021. Similarly, the assessed bio-fertilizers; N-fixing bacteria, and amino acid mixture considerably enhanced all growth, yield, and fruit parameters compared to the untreated control, with the superiority of N-fixing bacteria in most traits (Tables 7 and 8). The lowest values in all studied growth, yield, and fruit parameters were produced by the untreated trees in both studied factors in both seasons (Tables 7 and 8). On the contrary, goat manure combined with N-fixing bacteria presented the uppermost interaction impact compared to the other treatments in both seasons. The interaction between goat manure with N-fixing bacteria improved tree canopy volume, number of flowers per inflorescence, number of inflorescences per shoot, initial fruit set, and fruit retention, by 48.08%, 68.10%, 179.1%, 91.13%, and 111.2% respectively in the first season and 26.06%, 92.06%, 117.5%, 19.68%, and 104.0% compared untreated control. Similarly, the interaction between goat manure with N-fixing bacteria enhanced fruit length, fruit width, fruit fresh weight, and total fruit yield by 31.60%, 22.84%, 53.35%, and 86.56% respectively in the first season and 18.60%, 24.68%, 59.53%, and 70.73% compared to untreated trees.
3.3 Interrelationship among the Assessed Applications and Evaluated Parameters
Understanding the interrelationship between the evaluated applications and studied parameters of ‘Kalamata’ olive trees is a pivotal aspect that can provide helpful information. The heatmap and hierarchical clustering is an applicable statistical analysis to explore the relationship between the evaluated applications and studied parameters for ‘Kalamata’ olive cv. The present study divided the evaluated organic and bio-fertilizers into clusters based on photosynthetic pigments, leaf mineral contents, growth, yield, and fruit parameters (Fig. 1). The combination treatment of goat manure with N-fixing bacteria and amino acid mixture exhibited the highest values, followed by olive pomace with both biofertilizers for all evaluated parameters (depicted in blue color). On the contrary, the non-fertilized control olive trees possessed the lowest values of all evaluated parameters (represented in red color).
Figure 1: Heatmap and hierarchical clustering divide the evaluated organic and bio-fertilizers and amino acid mixture into clusters based on photosynthetic pigments, leaf mineral contents, growth, yield, and fruit parameters. Red and blue colors reveal low and high values for the corresponding parameters, respectively
Newly reclaimed soils suffer from a deficiency of mineral nutrients and numerous environmental stresses [39,40]. Mineral fertilization contributes to environmental pollution with nitrate leading to negative impacts on human health [41]. Consequently, it is necessary to find economic and environment-friendly applications to ameliorate plant growth and productivity of ‘Kalamata’ olive trees under these poor conditions [42,43]. Organic and biofertilizers have become more critical to sustain agricultural production and diminish mineral fertilization. Furthermore, in a circular economy framework, using waste by-products as fertilizer is essential to support sustainable agriculture [44,45]. Goat manure is conventional manure collected from local large goat herds. Besides, olive pomace is obtained from olive oil production and could be used as a beneficial alternative organic fertilizer. This is consistent with the findings of Erel et al. [46] and Tekaya et al. [47]. Similarly, Bellitürk et al. [48] showed that olive ‘Kalamata’ trees mineral elements (N, P, K, Ca, Mg, and Fe) were increased by utilizing organic fertilizers. Hence, the current study was performed to explore the influence of integrated use of organic (olive pomace and goat manure) and bio-fertilizer N-fixing bacteria as well as amino acid on physiological growth, yield, and fruit parameters of ‘Kalamata’ olive trees grown on poor-fertility sandy soil containing low nutrient contents. Organic material provides a source of phosphorus and carbon to the microflora population, as well as sites for the microflora to colonize. Bacteria secrete enzymes that catalyze the liberation of calcium and phosphorus from insoluble calcium phosphate and iron and phosphorus from insoluble iron phosphate [49]. As a result, it supplies the amount of calcium required to maintain the stability and integrity of the cytoplasmic membranes in the table olive fruit, by enhancing firmness fruit quality and boosting the cell wall’s resistance by forming bonds with the median lamella’s pectin. Calcium concentrations have been linked to physiological processes such as fruit softening, browning reactions, and ripening-related alterations [50].
Organic fertilizer greatly benefits poor-fertility sandy soils by elevating soil organic matter, water-holding capacity, and providing a source of slow-release macro and micronutrients [51]. It improves cation exchange capacity and elevates soil fertility, soil structure, and moisture retention [52]. Earlier published reports disclosed the importance of organic fertilization in elevating nutrient contents (nitrogen, phosphorus, potassium, calcium, and magnesium) in the soil [53–55].
Moreover, it stimulates beneficial soil micro-organisms that have a crucial role in improving soil properties, adjusting undesirable fluctuations of soil pH and processing ability, and mobilizing unavailable forms of nutrient components [56,57]. Accordingly, these advantages ameliorate plant growth and productivity under poor-fertility sandy soils. The obtained results displayed that both evaluated organic fertilizers; goat manure (16.8 kg/tree/year) and olive pomace (8.5 kg/tree/year), significantly promoted photosynthetic pigments (chlorophyll a, chlorophyll b, and carotenoids) of ‘Kalamata’ olive treated trees. Also, these organic fertilizers under study significantly increased leaf mineral contents (N, P, K, Ca, Mg, and Fe), tree canopy volume, number of flowers per inflorescence, number of inflorescences per shoot, initial fruit set, fruit retention, fruit length, fruit width, fruit fresh weight, and total fruit yield compared with the non-fertilized kalamata olive trees. The enhancement of evaluated parameters was attributed to the improved availability of nutrients released in the soil by olive pomace and goat manure compared to the non-fertilized olive trees. Besides, olive pomace and goat manure contain an adequate amount of organic matter which holds water and enhances the mineralization process. This rise in physical characteristics could be brought about by the increased leaf area of kalamata olive trees after using organic and bio-fertilizers. Under the application of organic and bio-fertilizers, there was a large increase in leaf area and stomatal density, which may have been caused by the growth in cell size and number. This may also be a result of the synergistic and antagonistic interactions between cytokines and auxins, which are both facilitated by all treatments with biofertilizers under investigation and implicated in the control of cell expansion [58].
In this context, El-Alakmy et al. [59] depicted that applying organic fertilizer particularly fish scrap provided a high olive yield and quality and protected trees from environmental stress. Similarly, Nwaigwe et al. [60] elucidated that organic fertilizer, particularly goat manure stimulated sweet potato growth, yield component, and root yield compared to non-fertilized control. Moreover, Hassan et al. [61] proved that organic fertilizer, especially poultry manure, improved flowering density, fruit set, fruit yield, and quality of olive compared to the non-fertilized control.
Biofertilizer is a natural stimulant that contains effective microbial strains [62]. It enhances soil fertility and plant nutrient uptake by increasing the availability of nutrients to plant roots [27]. Hence, it stimulates plant growth and productivity. The application of biofertilizer reduces synthetic fertilizer’s utilization and mitigates its hazardous impacts [63]. Therefore, its application enhances sustainable agriculture, remediation of polluted soils, and improves the quality of agricultural products [64]. It is an effective, promising, renewable source, inexpensive, and eco-friendly alternative to mineral fertilization. The present study investigated the impact of bio-fertilizer: N-fixing bacteria, and amino acid mixture on Kalamata olive photosynthetic pigments, leaf mineral contents, growth, yield, and fruit parameters. Both evaluated N-fixing bacteria and amino acid mixture significantly enhanced studied parameters compared with the untreated trees.
Similarly, Senjobi et al. [51,65] disclosed that applied organic fertilizer and supported with biofertilizer remarkably enhanced photosynthetic pigments and mineral contents of N, P, K, Ca, Mg, Fe, Mn, and Zn. Furthermore, Mazeh et al. [66] deduced that applying organic fertilizer combined with biofertilizer is highly efficient in supplying the nutritional requirements of olive trees with essential elements. These nutrients are vital compounds for the biosynthesis of nucleic acids, enzymes, proteins, and photosynthetic pigments [67,68]. Additionally, these nutrients are valuable compounds for transferring and storing energy in plants and maintaining cell turgor, cell expansion, and stomatal function [69,70]. Accordingly, all these positive impacts of organic fertilizer and biofertilizer are reflected in photosynthetic pigments, leaf mineral contents, growth, yield, and quality parameters of olive.
The heatmap and hierarchical clustering is a proper statistical approach to exploring the association between assessed treatments and studied parameters [71,72]. It was employed in the current study to explore the interrelationship among the evaluated organic and biofertilizer N-fixing bacteria and amino acid mixture based on photosynthetic pigments, leaf mineral contents, growth, yield, and fruit parameters. The evaluated applications were divided into different clusters based on their performance. The application of goat manure combined with N-fixing bacteria or amino acid mixture exhibited the highest values, followed by olive pomace combined with both N-fixing bacteria and amino acid mixture for all evaluated parameters compared with the non-fertilized control trees. Accordingly, the heatmap and hierarchical clustering results reinforced the aforementioned presented results. Similarly, El-Alakmy et al. [59,73] and Nwaigwe et al. [60,74] effectively applied the heatmap and hierarchical clustering to explore the relationship between studied treatments and evaluated traits.
The present study clarified the potential of organic fertilizers (goat manure or olive pomace) combined with biofertilizers (N-fixing bacteria and amino acid mixture) in enhancing photosynthetic pigments (chlorophyll a, b, and carotenoids), leaf mineral contents (N, P, K, Ca, Mg, and Fe), tree canopy volume, number of flowers per inflorescence, number of inflorescences per shoot, initial fruit set, fruit retention, fruit length, fruit width, fruit fresh weight, and total fruit yield of ‘Kalamata’ olive cultivar compared to the non-fertilized control trees. Conclusively, the co-application of organic and biofertilizer, particularly goat manure (16.8 kg/tree/year) combined with N-fixing bacteria (150 g/tree of Azospirillum spp. and Azotobacter chroococcum) could be recommended in low-fertility soil for sustaining olive production and reducing mineral fertilization. Agricultural byproducts can be used efficiently in the production of good and affordable organic fertilizers, which can then be used to solve the problems of safe disposal of agricultural wastes in Egypt, in order to increase the productivity of one of the most significant dual-purpose crops in Egypt and the rest of the world, olive trees ‘Kalamata’ cv.
Funding Statement: The authors extend their appreciation to the Deanship of Scientific Research at Jouf University for funding this work through Research Grant No. (DSR2020-01-2553).
Author Contributions: The authors confirm their contribution to the paper as follows: study conception and design: B.F.A., M.S.M.A.; data collection: B.F.A., M. M.G.; analysis and interpretation of results: B.F.A., M. M.G.; draft manuscript preparation: B.F.A., M.S.M.A. All authors reviewed the results and approved the final version of the manuscript.
Conflicts of Interest: The authors declare they have no conflicts of interest to report regarding the present study.
References
1. Vossen, P. (2007). Olive oil: History, production, and characteristics of the world’s classic oils. HortScience, 42(5), 1093–1100. [Google Scholar]
2. Moghazy, N. H., Kaluarachchi, J. J. (2020). Sustainable agriculture development in the Western Desert of Egypt: A case study on crop production, profit, and uncertainty in the Siwa region. Sustainability, 12(16), 6568. [Google Scholar]
3. FAOSTAT (2022). Food and agriculture organization of the United Nations. Statistical Database. http://www.fao.org/faostat/en/#data [Google Scholar]
4. Romeo, F. V., Timpanaro, N., Intelisano, S., Rapisarda, P. (2018). Quality evaluation of Aitana, Caiazzana and Nocellara del Belice table olives fermented with a commercial starter culture. Emirates Journal of Food and Agriculture, 30(7), 604–610. [Google Scholar]
5. Patumi, M., d’Andria, R., Marsilio, V., Fontanazza, G., Morelli, G. et al. (2002). Olive and olive oil quality after intensive monocone olive growing (Olea europaea L., cv. Kalamata) in different irrigation regimes. Food Chemistry, 77(1), 27–34. [Google Scholar]
6. Karydas, C. G., Sekuloska, T., Silleos, G. N. (2009). Quantification and site-specification of the support practice factor when mapping soil erosion risk associated with olive plantations in the Mediterranean Island of Crete. Environmental Monitoring and Assessment, 149, 19–28. [Google Scholar] [PubMed]
7. Mansour, E., Merwad, A. M. A., Yasin, M. A. T., Abdul-Hamid, M. I. E., El-Sobky, E. E. A. et al. (2017). Nitrogen use efficiency in spring wheat: Genotypic variation and grain yield response under sandy soil conditions. Journal of Agricultural Science Cambridge Core, 155(9), 1407–1423. https://doi.org/10.1017/S0021859617000600 [Google Scholar] [CrossRef]
8. Omar, M., Rabie, H. A., Mowafi, S. A., Othman, H. T., Abd El-Moneim, D. et al. (2022). Multivariate analysis of agronomic traits in newly developed maize hybrids grown under different agro-environments. Plants, 11(9), 1187. https://doi.org/10.3390/plants11091187 [Google Scholar] [PubMed] [CrossRef]
9. El-Hady, A., Mohamed, A., Abd-Elkrem, Y. M., Rady, M. O., Mansour, E. et al. (2022). Impact on plant productivity under low fertility sandy soil in arid environment by revitalization of lentil roots. Frontiers in Plant Science, 13, 937073. https://doi.org/10.3389/fpls.2022.937073 [Google Scholar] [PubMed] [CrossRef]
10. Yermiyahu, U., Erel, R., Ben-Gal, A., Schwartz, A., Dag, A. (2009). The role of macro-nutrients in olive tree flowering and fruit set. The Proceedings of the International Plant Nutrition Colloquium XVI, California. Permalink. https://escholarship.org/uc/item/7bg4m169 [Google Scholar]
11. Fernandez-Escobar, R. (2010). Fertilization. In: Barranco, D., Fernandez-Escobar, R., Rallo, L. (Eds.Olive growing, pp. 267–297. Australia: Rural Industries Research and Development Corporation. [Google Scholar]
12. Cakmak, I., Kirkby, E. A. (2008). Role of magnesium in carbon partitioning and alleviating photooxidative damage. Physiologia Plantarum, 133(4), 692–704. https://doi.org/10.1111/j.1399-3054.2007.01042.x [Google Scholar] [PubMed] [CrossRef]
13. Zipori, I., Yermiyahu, U., Dag, A., Erel, R., Ben-Gal, A. et al. (2022). Effect of macronutrient fertilization on olive oil composition and quality under irrigated, intensive cultivation management. Journal of the Science of Food and Agriculture, 103(1), 48–56. https://doi.org/10.1002/jsfa.12110 [Google Scholar] [PubMed] [CrossRef]
14. Morales-Sillero, A., Lodolini, E. M., Suárez, M. P., Navarrete, V., Jiménez, M. R. et al. (2020). Calcium applications throughout fruit development enhance olive quality, oil yield, and antioxidant compounds’ content. Journal of the Science of Food and Agriculture, 101(5), 1944–1952. https://doi.org/10.1002/jsfa.10810 [Google Scholar] [PubMed] [CrossRef]
15. Lodolini, E. M., Fernandez, A., Morales-Sillero, A., Mendiano, A., Vertedor, D. M. (2023). Influence of pre-harvest calcium applications on table olive characteristics during Spanish-system elaporation process. Scientia Horticulturae, 308, 1–9. https://doi.org/10.1016/j.scienta.2022.111577 [Google Scholar] [CrossRef]
16. Marzouk, H., Kassem, H. (2011). Improving fruit quality, nutritional value and yield of Zaghloul dates by the application of organic and/or mineral fertilizers. Scientia Horticulturae, 127(3), 249–254. [Google Scholar]
17. Magdoff, F., Weil, R. R. (2004). Soil organic matter management strategies. Soil Organic Matter in Sustainable Agriculture, 45–65. [Google Scholar]
18. Johnston, A. E., Poulton, P. R., Coleman, K. (2009). Soil organic matter: Its importance in sustainable agriculture and carbon dioxide fluxes. Advances in Agronomy, 101, 1–57. [Google Scholar]
19. Uwah, D. F., Eyo, V. (2014). Effects of number and rate of goat manure application on soil properties, growth and yield of sweet maize (Zea mays L. saccharata Strut). Sustainable Agriculture Research, 3(4), 75–83. [Google Scholar]
20. Thomas, C. L., Acquah, G. E., Whitmore, A. P., McGrath, S. P., Haefele, S. M. (2019). The effect of different organic fertilizers on yield and soil and crop nutrient concentrations. Agronomy, 9(12), 776. [Google Scholar]
21. Gutser, R., Ebertseder, T., Weber, A., Schraml, M., Schmidhalter, U. (2005). Short-term and residual availability of nitrogen after long-term application of organic fertilizers on arable land. Journal of Plant Nutrition and Soil Science, 168, 439–446. [Google Scholar]
22. Lim, S. L., Wu, T. Y., Lim, P. N., Shak, K. P. Y. (2015). The use of vermicompost in organic farming: Overview, effects on soil and economics. Journal of the Science of Food and Agriculture, 95(6), 1143–1156. [Google Scholar] [PubMed]
23. Sinha, R. K., Herat, S., Chauhan, K., Valani, D. (2009). Earthworms vermicompost: A powerful crop nutrient over the conventional compost & protective soil conditioner against the destructive chemical fertilizers for food safety and security. Journal of Agriculture and Environmental Sciences, 5, 1–55. [Google Scholar]
24. Lazcano, C., Zhu-Barker, X., Decock, C. (2021). Effects of organic fertilizers on the soil microorganisms responsible for N2O emissions: A review. Microorganisms, 9(5), 983. [Google Scholar] [PubMed]
25. Yuan, Y., Li, M., Hu, W., Zhang, J., Zhao, L. et al. (2011). Effects of biological organic fertilizer on tomato bacterial wilt and soil microorganisms. Journal of Agro-Environment Science, 30, 1344–1350. [Google Scholar]
26. Barzegar, A., Yousefi, A., Daryashenas, A. (2002). The effect of addition of different amounts and types of organic materials on soil physical properties and yield of wheat. Plant and Soil, 247(2), 295–301. https://doi.org/10.1023/A:1021561628045 [Google Scholar] [CrossRef]
27. Yadav, K. K., Sarkar, S. (2019). Biofertilizers, impact on soil fertility and crop productivity under sustainable agriculture. Environment and Ecology, 37(1), 89–93. [Google Scholar]
28. Das, P. P., Singh, K. R., Nagpure, G., Mansoori, A., Singh, R. P. et al. (2022). Plant-soil-microbes: A tripartite interaction for nutrient acquisition and better plant growth for sustainable agricultural practices. Environmental Research, 214(6), 113821. https://doi.org/10.1016/j.envres.2022.113821 [Google Scholar] [PubMed] [CrossRef]
29. Vessey, J. K. (2003). Plant growth promoting rhizobacteria as biofertilizers. Plant and Soil, 255(2), 571–586. https://doi.org/10.1023/A:1026037216893 [Google Scholar] [CrossRef]
30. Schütz, L., Gattinger, A., Meier, M., Müller, A., Boller, T. et al. (2018). Improving crop yield and nutrient use efficiency via biofertilization—A global meta-analysis. Frontiers in Plant Science, 8, 2204. [Google Scholar]
31. Mahapatra, S., Yadav, R., Ramakrishna, W. (2022). Bacillus subtilis impact on plant growth, soil health and environment. Journal of Applied Microbiology, 132(5), 3543–3562. [Google Scholar] [PubMed]
32. Elsayed, S. I., Glala, A., Abdalla, A. M., El-Sayed, A. E. G. A., Darwish, M. A. (2020). Effect of biofertilizer and organic fertilization on growth, nutrient contents and fresh yield of dill (Anethum graveolens). Bulletin of the National Research Centre, 44(122), 1–10. [Google Scholar]
33. El-Shamy, M. A., Alshaal, T., Mohamed, H. H., Rady, A. M. S., Hafez, E. M. et al. (2022). Quinoa response to application of phosphogypsum and plant growth-promoting rhizobacteria under water stress associated with salt-affected soil. Plants, 11(7), 872. https://doi.org/10.3390/plants11070872 [Google Scholar] [PubMed] [CrossRef]
34. Dukare, A., Paul, S., Kumar, R., Sharma, V. (2021). Microbial-based inoculants in sustainable agriculture: Current perspectives and future prospects. Biofertilizers, 1, 167–181. [Google Scholar]
35. Maksoud, M. A., Saleh, M. A., El-Shamma, M. S., Fouad, A. A. (2009). The beneficial effect of biofertilizers and antioxidants on olive trees under calcareous soil conditions. World Journal of Agricultural Sciences, 5(3), 350–352. [Google Scholar]
36. Dabbaghi, O., Tekaya, M., M’barki, N., Ouled ali, S., Öden, S. et al. (2018). Effect of foliar bio-fertilization on growth and biochemical parameters of olive trees at flowering. Journal of Plant Nutrition, 41(18), 2281–2297. https://doi.org/10.1080/01904167.2018.1500592 [Google Scholar] [CrossRef]
37. Fadeel, A. (1962). Location and properties of chloroplasts and pigment determination in roots. Physiologia Plantarum, 15(1), 130–146. [Google Scholar]
38. Reuter, D., Robinson, J. B. (1997). Plant analysis: An interpretation manual. Australia: CSIRO Publishing. [Google Scholar]
39. Mansour, E., Mahgoub, H. A. M., Mahgoub, S. A., El-Sobky, E. S. E. A., Abdul-Hamid, M. I. et al. (2021). Enhancement of drought tolerance in diverse Vicia faba cultivars by inoculation with plant growth-promoting rhizobacteria under newly reclaimed soil conditions. Scientific Reports, 11(1), 24142. https://doi.org/10.1038/s41598-021-02847-2 [Google Scholar] [PubMed] [CrossRef]
40. Desoky, E. S. M., Mansour, E., Ali, M. M. A., Yasin, M. A. T., Abdul-Hamid, M. I. E. et al. (2021). Exogenously used 24-epibrassinolide promotes drought tolerance in maize hybrids by improving plant and water productivity in an arid environment. Plants, 10(2), 354. https://doi.org/10.3390/plants10020354 [Google Scholar] [PubMed] [CrossRef]
41. Abdelrasheed, K. G., Mazrou, Y., Omara, A. E. D., Osman, H. S., Nehela, Y. et al. (2021). Soil amendment using biochar and application of K-humate enhance the growth, productivity, and nutritional value of onion (Allium cepa L.) under deficit irrigation conditions. Plants, 10(12), 2598. https://doi.org/10.3390/plants10122598 [Google Scholar] [PubMed] [CrossRef]
42. Desoky, E. S. M., Mansour, E., Yasin, M. A. T., El-Sobky, E. S. E. A., Rady, M. M. (2020). Improvement of drought tolerance in five different cultivars of Vicia faba with foliar application of ascorbic acid or silicon. Spanish Journal of Agricultural Research, 18(2), e0802. https://doi.org/10.5424/sjar/2020182-16122 [Google Scholar] [CrossRef]
43. Abd El-Moneim, D., ELsarag, E. I. S., Aloufi, S., El-Azraq, A. M., ALshamrani, S. M. et al. (2021). Quinoa (Chenopodium quinoa Willd.Genetic diversity according to ISSR and SCoT markers, relative gene expression, and morpho-physiological variation under salinity stress. Plants, 10(12), 2802. https://doi.org/10.3390/plants10122802 [Google Scholar] [PubMed] [CrossRef]
44. Lacolla, G., Rinaldi, M., Savino, M., Russo, M., Caranfa, D. et al. (2021). Effects of organic fertilization from wet olive pomace on emmer wheat (Triticum dicoccum Shrank) grain yield and composition. Journal of Cereal Science, 102, 103369. [Google Scholar]
45. Esmail, S. M., Aboulila, A. A., Abd El-Moneim, D. (2020). Variation in several pathogenesis-related (PR) protein genes in wheat (Triticum aestivum) involved in defense against Puccinia striiformis f. sp. tritici. Physiological and Molecular Plant Pathology, 112, 101545. [Google Scholar]
46. Erel, R., Dag, A., Ben-Gal, A., Schwartz, A., Yermiyahu, U. (2008). Flowering and fruit set of olive trees in response to nitrogen, phosphorus, and potassium. Journal of the American Society for Horticultural Science, 133(5), 639–647. [Google Scholar]
47. Tekaya, M., El-Gharbi, S., Mechri, B., Chehab, H., Bchir, A. et al. (2016). Improving performance of olive trees by the enhancement of key physiological parameters of olive leaves in response to foliar fertilization. Acta Physiologiae Plantarum, 38(4), 1–12. [Google Scholar]
48. Bellitürk, K., Çelik, A., Baran, M. F. (2022). The effect of vermicompost application on soil properties in olive (Olea europaea L. cv. Memecik) plant. Erwerbs-Obstbau, 64(1), 107–113. [Google Scholar]
49. Tian, J., Fei, G., Zhang, D., Deng, S., Liu, X. (2021). Roles of phosphate solubilizing microorganisms from managing soil phosphorus deficiency to mediating biogeochemical P cycle. Biology, 10(2), 158. [Google Scholar] [PubMed]
50. Hocking, B., Tyerman, S. D., Burton, R. A., Gilliham, M. (2016). Fruit calcium: Transport and physiology. Frontiers in Plant Science, 7, 569. [Google Scholar] [PubMed]
51. Senjobi, B., Akinsete, S., Ande, O., Ademoye, O. (2013). Sandy soil improvement using organic materials and mineral fertilizer on the yield and quality of jute plant (Corchorus olitorius). Journal of Biology and Life Science, 4(1), 219–233. [Google Scholar]
52. Ramos, F. T., Dores, E. F. D. C., Weber, O. L. D. S., Beber, D. C., CampeloJr, J. H. et al. (2018). Soil organic matter doubles the cation exchange capacity of tropical soil under no-till farming in Brazil. Journal of the Science of Food and Agriculture, 98(9), 3595–3602. [Google Scholar] [PubMed]
53. Omara, A. E. D., Hafez, E. M., Osman, H. S., Rashwan, E., El-Said, M. A. A. et al. (2022). Collaborative impact of compost and beneficial rhizobacteria on soil properties, physiological attributes, and productivity of wheat subjected to deficit irrigation in salt affected soil. Plants, 11(7), 877. https://doi.org/10.3390/plants11070877 [Google Scholar] [PubMed] [CrossRef]
54. Han, S. H., An, J. Y., Hwang, J., Kim, S. B., Park, B. B. (2016). The effects of organic manure and chemical fertilizer on the growth and nutrient concentrations of yellow poplar (Liriodendron tulipifera Lin.) in a nursery system. Forest Science and Technology, 12(3), 137–143. [Google Scholar]
55. Souto, A. G. D. L., Cavalcante, L. F., Silva, M. R. M. D., Lima Neto, A. J. D., Diniz, B. L. M. T. (2018). Nutritional status and production of noni plants fertilized with manure and potassium. Journal of Soil Science and Plant Nutrition, 18(2), 403–417. [Google Scholar]
56. Angelova, V., Akova, V., Artinova, N., Ivanov, K. (2013). The effect of organic amendments on soil chemical characteristics. Bulgarian Journal of Agricultural Science, 19(5), 958–971. [Google Scholar]
57. de Boer, W., Kowalchuk, G. A. (2001). Nitrification in acid soils: Micro-organisms and mechanisms. Soil Biology and Biochemistry, 33(7), 853–866. https://doi.org/10.1016/S0038-0717(00)00247-9 [Google Scholar] [CrossRef]
58. Coenen, C., Lomax, T. L. (1997). Auxin-cytokinin interactions in higher plants: Old problems and new tools. Trends in Plant Science, 2(9), 351–356. https://doi.org/10.1016/S1360-1385(97)84623-7 [Google Scholar] [PubMed] [CrossRef]
59. El-Alakmy, D. A., Nagaty, M., Sourour, M., El-Deeb, M. (2018). Effect of bio and organic fertilizers on flowering, yield, and fruit quality of kalamata olive trees under North Sinai condition. Journal of Plant Production, 9(11), 939–945. https://doi.org/10.21608/jpp.2018.36607 [Google Scholar] [CrossRef]
60. Nwaigwe, G., Nwankwo, I. (2015). Effect of goat manure on soil amendment and correlation studies for selecting plant characters for root yield in sweet potato. International Journal of Engineering and Technical Research, 3(6), 219–229. [Google Scholar]
61. Hassan, A. M., Abd-Alhamid, N., Aly, R. A., Hassan, H. S. A., Abdelhafez, A. A. et al. (2015). Effect of organic and bio-fertilization on yield and quality of “Manzanillo” olives. Manzanillo, 4(3), 485–493. [Google Scholar]
62. Al Abboud, M., Ghany, T. A., Alawlaqi, M. (2014). Role of biofertilizers in agriculture: A brief review. Mycopath, 11(2), 95–101. [Google Scholar]
63. Nosheen, S., Ajmal, I., Song, Y. (2021). Microbes as biofertilizers, a potential approach for sustainable crop production. Sustainability, 13(4), 1868. https://doi.org/10.3390/su13041868 [Google Scholar] [CrossRef]
64. Asoegwu, C. R., Awuchi, C. G., Nelson, K., Orji, C. G., Nwosu, O. U. et al. (2020). A review on the role of biofertilizers in reducing soil pollution and increasing soil nutrients. Himalayan Journal of Agriculture, 1(1), 34–38. [Google Scholar]
65. El-Kharafen, A. S., El-Alakmy, H. A., Ahmed, R. B., Sourour, M. M., ElDeep, M. D. (2018). The use of some organic and bio-fertilizers for earligrande peach trees fertilization under North Sinai conditions: Vegetative growth and leaf chemical content. Sinai Journal of Applied Sciences, 7(2), 99–114. https://doi.org/10.21608/sinjas.2018.78908 [Google Scholar] [CrossRef]
66. Mazeh, M., Almadi, L., Paoletti, A., Cinosi, N., Daher, E. et al. (2021). Use of an organic fertilizer also having a biostimulant action to promote the growth of young olive trees. Agriculture, 11(7), 593. [Google Scholar]
67. Maathuis, F. J. (2009). Physiological functions of mineral macronutrients. Current Opinion in Plant Biology, 12(3), 250–258. [Google Scholar] [PubMed]
68. Adekiya, A. O., Ejue, W. S., Olayanju, A., Dunsin, O., Aboyeji, C. M. et al. (2020). Different organic manure sources and NPK fertilizer on soil chemical properties, growth, yield and quality of okra. Scientific Reports, 10, 16083. [Google Scholar] [PubMed]
69. Latef Abdel, A. A. H. (2011). Ameliorative effect of calcium chloride on growth, antioxidant enzymes, protein patterns and some metabolic activities of canola (Brassica napus L.) under seawater stress. Journal of Plant Nutrition, 34, 1303–1320. [Google Scholar]
70. Waraich, E. A., Ahmad, R., Ashraf, M. (2011). Role of mineral nutrition in alleviation of drought stress in plants. Australian Journal of Crop Science, 5(6), 764–777. [Google Scholar]
71. Abd El-Moneim, D. A., Mohamed, I. N., Belal, A. H., Atta, M. E. (2008). Screening bread wheat genotypes for drought tolerance: 1-Germination, radical growth and mean performance of yield and its components. Annals of Agricultural Sciences, 53(1), 171–181. [Google Scholar]
72. Gowayed, S., Abd El-Moneim, D., Metwali, E., El-Malky, M. (2020). Combining ability and heterosis studies for some economic traits in rice (Oryza sativa L.). Research Journal of Biotechnology, 15(1), 101–111. [Google Scholar]
73. Rehman, F., Saeed, A., Yaseen, M., Shakeel, A., Ziaf, K. et al. (2019). Genetic evaluation and characterization using cluster heat map to assess NaCl tolerance in tomato germplasm at the seedling stage. Chilean Journal of Agricultural Research, 79(1), 56–65. [Google Scholar]
74. Cloete, K. J., Šmit, Ž., Minnis-Ndimba, R., Vavpetič, P., Du Plessis, A. et al. (2019). Physico-elemental analysis of roasted organic coffee beans from Ethiopia, Colombia, Honduras, and Mexico using X-ray micro-computed tomography and external beam particle induced X-ray emission. Food Chemistry, 2, 100032. [Google Scholar]
Cite This Article
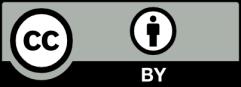