Open Access
ARTICLE
Effects of Plant Extracts and Beauveria bassiana on the Activity of Defense-Related Enzymes in Solanum lycopersicum L. during Interaction with Fusarium oxysporum f. sp. lycopersici
Laboratorio de Biotecnología Vegetal, Tecnológico Nacional de Mexico, Campus Tuxtla Gutiérrez, Tuxtla Gutiérrez, 29050, Mexico
* Corresponding Authors: Carlos Alberto Lecona-Guzmán. Email: ,
; Joaquín Adolfo Montes-Molina. Email:
Phyton-International Journal of Experimental Botany 2023, 92(9), 2503-2518. https://doi.org/10.32604/phyton.2023.029784
Received 15 March 2023; Accepted 23 May 2023; Issue published 28 July 2023
Abstract
The objective to this work was to evaluate the enzymatic activity in the culture of Solanum lycopersicum L. infected with Fusarium oxysporum after the combined application of Beauveria bassiana and plant extracts. Solanum lycopersicum plantlets were transplanted 15 days after the emergency. Five days after transplanting, Beauveria bassiana spores were applied at a concentration of 1 × 107 spores mL−1 onto soil (along with A. indica (N) and P. auritum (H) leaf extracts) where S. lycopersicum plants were planted. Eight days after transplanting, spores of F. oxysporum strain were applied at a concentration of 1 × 106 spores mL−1 to soil where S. lycopersicum plants were growing. The development of S. lycopersicum plants was monitored for 114 days, whereby a randomized complete block treatment design was used, the roots of the plants were crushed with liquid nitrogen, after which UV/VIS spectrophotometry was used to determine protein concentration, as well as the activity of β-1,3-glucanases, peroxidases (POX, EC1.11.1.7), catalase and chitinases. The treatments combining B. bassiana and A. indica and P. auritum extracts, had a significant difference in enzymatic activity levels, thus contributing to better defense mechanisms and a greater protection to S. lycopersicum plants in the presence of F. oxysporum. The application of B. bassiana and plant extracts to the ground mitigated damage caused by F. oxysporum on S. lycopersicum plants.Keywords
The Solanaceae family is attacked by different pathogens, which cause losses in production. Tomatoes are considered a very important crop worldwide due to their economic and nutritional value, as this species is a source of vitamins A and C [1,2].
Because high humidity levels are required to keep tomatoes fresh, the growth of pathogenic fungi such as Alternaria alternata, Fusarium sp., Fusarium oxysporum is promoted, among others. Fusarium is a fungus found in agricultural products and is very common in soils, which is why it is classified as a soil-borne fungus [3]. These fungi cause rot, which is one of the main diseases occurring after harvest in S. lycopersicum, causing large losses [3]. Losses in S. lycopersicum L. production range between 30% and 40%, but it can sometimes reach up to 80% [4]. Fusarium oxysporum (Fo) soil-borne fungus is one of the most devastating diseases, ranking fifth among phytopathogenic fungi, which can significantly reduce the production of S. lycopersicum L. [4]. The Fusarium oxysporum f. sp. lycopersici exists in many pathogenic forms, and because it possesses many mechanisms that help it overcome the defenses of many plants, it is a parasite in more than 100 species of gymnosperm and angiosperm plants [5].
The control of this fungus is difficult as it establishes itself as an endophyte in cells and in vascular tissues. The most employed strategies for its control are the use of fungicides (such as N-methyl dithiocarbamate or ethidium bromide), seed disinfection with 1% hydrochloric acid, and the use of resistant cultivars. However, indiscriminate use of these agrochemicals has caused soil contamination and fungal resistance to these products, which is why new effective and environment-friendly strategies are being sought.
Biological control is considered as a reasonable alternative for controlling various diseases such as wilt, which is caused by Fusarium. The application of non-pathogenic antagonistic microorganisms, that are capable of minimizing or stopping the effects caused by this pathogen has been advised [6]. Recent studies have mentioned that the biological control of pathogens such as F. oxysporum may be considered a safer method for humans and the environment [7]. Beauveria bassiana (Bals.) Vuill. (Ascomycota: Hypocreales), has been used as an alternative for both the biological control of pests and as an attempt to replace chemical products. Currently, this non-pathogenic antagonistic microorganism is the active ingredient of different commercial products that are used for the sustainable management of pests [8].
It has been reported that B. bassiana grows naturally as an endophytic microorganism in vegetative tissues throughout the plant. It is generally found in the soil and colonizes the plant by horizontal transmission [9], and it can also be artificially introduced as an endophyte by different ways such as seed coating, soil irrigation, root immersion and foliar spraying [10]. Sinno et al. [11] reported that B. bassiana may protect tomatoes from pathogens attack, such as those by R. solani Kühn, B. cinerea Pers., and F. oxysporum Schltdl. Protection from attacks by pests, such as A. gossypii Glover, B. tabaci Gennadius, E. vitis Goethe, H. zea Boddie, H. armigera Hübner, O. sulcatus Fabricius, P. ficus Signoret, S. littoralis Boisduval, S. exigua Hübner, and T. absoluta Meyrick.
B. bassiana has recently been reported as a microorganism that can be used as a dual-purpose microbial control, meaning it may be effective against pathogens and insects. Culebro-Ricaldi et al. [12] reported that B. bassiana inhibits the mycelial growth of various pathogens under in vitro conditions. Inhibited pathogens include G. graminis var. Tritici, A. mellea, N. rosellina, F. oxysporum, B. cinerea and R. solani. These same authors report that B. bassiana induces cell lysis in pathogens such as P. ultimum, P. debaryanum and S. nodorum.
Extracts obtained from of A. indica A. Juss. tree, are a natural source against a wide variety of pathogenic microorganisms. A. indica A. Juss. belongs to the Meliaceae family, and is widely cultivated in Africa, Australia, the Caribbean, Central America, and South America [13]. It is known that several crude extracts of A. indica A. Juss. have microbicidal and insecticidal activity, including the blocking of the larval metamorphosis process. The main active metabolite is nortriterpenoid, also known as azadirachtin [14]. The ethanol extracts of A. indica A. Juss. seeds inhibited the growth of Bacillus thuringiensis, Bacillus subtilis and Nocardia sp. significantly. The antimicrobial components in A. indica A. Juss. seed extracts have been identified, and it has been found that nimbolide, nimbin, and nimbidin (triterpenoids), azadirachtin, and gedunin are jointly responsible for antimicrobial activity [15].
Piper auritum Kunth is a leafy plant that lives in warm, semi-warm and semi-dry climates. Its leaves are wide and large, greenish in color and give off a pleasant smell when squeezed. This evergreen plant is known as acuyo, cordoncillo, white cordon, aniseed, cancer leaf, holy leaf, momo or holy grass. It also received the name of holy leaf by the Spanish priests, who were impressed by its medicinal properties [16]. The physicochemical properties of P. autitum have been studied and 16 compounds have been isolated from the leaves and roots to this date. These compounds have been characterized as phenylpropanoids, triterpenoids and essential oils [17]. The essential oils have been characterized in previous studies, and their antifungal activity has been proven [18]. The objective to this research was to evaluate the enzymatic activity (glucanases, chitinases, peroxidases and catalases) in response to the combined application of Beauveria bassiana and plant extracts during the culture of Solanum lycopersicum L. infected with Fusarium oxysporum.
2.1 Plant Material and Preparation of Fungal Solution of B. bassiana and Solution of F. oxysporum f. sp. lycopersici
Commercial Rio Grande® S. lycopersicum Saladette seeds (Solanum lycopersicum L.) were used, which subsequently germinated in an agricultural seedbed of expanded polystyrene with 200 cavities. Compost was used as a substrate and one seed was placed in each cavity.
The F. oxysporum f. sp. lycopersici strain (Fol strain 17108, not registered) was provided by the Plant Breeding Department at the Universidad Autónoma Agraria Antonio Narro, located in Saltillo, Coahuila, México. The B. bassiana strain (Genbank number KX232465.1) was provided by the Tecnológico Nacional de México campus Tlajomulco, located in Tlajomulco de Zúñiga, Jalisco, México.
The conidial suspension was prepared in 250 mL Erlenmeyer flasks, for which 50 mL of medium were used. One liter of medium contained 0.5 gL−1 MgSO4 7H2O, 0.5 gL−1 KCl, 3 gL−1 NaNO3, 1 gL−1 K2HPO4, 0.01 gL−1 FeSO4, as well as 200 mL of V8 juice and distilled water. This was also supplemented with 1% colloidal chitin (w/v). The flasks were kept in incubation on a rotary shaker for eight days at 28°C at 180 rpm. The liquid medium was inoculated with mycelial discs with B. bassiana strain on PDA, where active growth has been reported [19]. Subsequently, under a hood with laminar flow with UV light, the supernatant was filtered through sterile gauze in a 250 mL Erlenmeyer flask to remove hyphae and to obtain the stock solution. The spore count was performed in a Neubauer chamber, and the solution was adjusted with sterile distilled water until a concentration of 1 × 107 conidia mL−1 was obtained [20].
A solution F. oxysporum f. sp. lycopersici conidia was grown in Erlenmeyer flasks with potato Dextrose liquid medium. A mycelial disc of the fungus was inoculated in an Erlenmeyer flask, which was allowed to grow for five weeks at a temperature of 28 ± 2°C. After that, the supernatant was filtered with sterile gauze in a 250 mL Erlenmeyer flask to remove hyphae and to obtain the stock solution, which was also done under a laminar flow hood with UV light. The spore count was performed in a Neubauer chamber, and the solution was adjusted with sterile distilled water until a concentration of 1 × 10−6 conidia mL−1 was obtained.
The study was carried out in the Biotechnology and Greenhouse Soil Laboratory of the Tecnológico Nacional de México Campus Tuxtla Gutiérrez (latitude 16.758311°, N, longitude −93.172347W). Leaves from A. indica A. Juss. (Azadirachta indica A. Juss.) and P. auritum, Kunth (Piper auritum Kunth) were collected from trees at the Instituto Tecnológico de Tuxtla Gutiérrez and within the limits of the City of Tuxtla Gutiérrez.
Subsequently, the leaves were removed from the stem and washed with water. After this came a disinfection step involving immersion in 1% commercial NaClO for 5 min, after which they were washed again with sterile distilled water to remove chlorine residues. Next step was the crushing of the fresh leaves with a blender, to obtain a particle size of 2 to 3 mm using 100 g of fresh leaf per liter of sterile water. Finally, the extraction of secondary metabolites via maceration of the leaves was carried out in an amber container for 72 h at room temperature and in the absence of light [13]. After 72 h, filtration was carried out under a hood with laminar flow and UV lights to avoid contamination. The extract was stored at a temperature of −4°C, in a freezer. Vegetable samples were prepared separately.
2.3 Inoculation of Beauveria Bassiana and Application of Plant Extracts in S. lycopersicum L. Plants
Inoculation was performed in the soil 10 days after transplantation (dat) with 10 mL of a spore suspension (1 × 107 conidia mL−1), which was inoculated every 8 days, as described previously [21]. At 5 dat, 5 mL of A. indica, A. Juss extract were applied to the soil, as well as 5 mL of P. auritum Kunth extract. This application was also performed at 8 day intervals, according to the programmed treatments.
2.4 Inoculation of Fusarium oxysporum f. sp. lycopersici in S. lycopersicum Plants
A single inoculation was performed in the soil at 26 dat with 10 mL of a spore suspension (conc. 1 × 106 conidia mL−1) containing the phytopathogenic fungus F. oxysporum f. sp. lycopersici, according to [22].
The roots of S. lycopersicum plants were crushed with liquid nitrogen. The plant material was washed 3 times with cold acetone for 1 min in a 1:2 ratio (w/v). After each wash, it was centrifuged at 1,000 rpm for 5 min at 4°C and was then resuspended in 100 mM phosphate buffer (pH 6.5), where the same 1:2 ratio (w/v) was kept and was stirred on ice for 1 h. Finally, the mixture was centrifuged at 4,500 rpm for 30 min at 4°C, after which the supernatant was deposited in Eppendorf tubes. Samples were then frozen at −20°C for subsequent determinations [23].
2.6 Evaluation of the Enzymatic Activity in Roots of S. lycopersicum Plants
2.6.1 Total Protein Extraction
The roots were collected at 50, 66, 82, 98 and 114 dat. However, the samples that were analyzed were those of 114 dat, as our research team observed that the plant presents a fungicidal effect at this time. The total protein concentration was determined with the Bio-Rad kit (Bio-Rad Laboratories) based on the Bradford method [24]. The reading was performed on a UV/VIS spectrophotometer at an absorbance of 595 nm. The standard curve was made from a stock solution of bovine serum albumin (BSA, 1 mg·mL−1). Three replicates were performed for each treatment.
2.6.2 Quantification of Chitinases (E.C.3.2.1.14)
Quantification was performed according to the methodology proposed previously [25]. A colloidal chitin substrate obtained from reactive grade chitin (Sigma-Aldrich) was used, with which a stock solution was prepared at a concentration of 10 mg·mL−1 chitin in distilled water. A mixture of colloidal chitin (300 µL) and enzymatic solution (600 µL) was added to each of the test tubes, after which they were placed under stirring in a vortex for sample homogenization. Subsequently, 150 µL of a 0.8 M sodium tetraborate buffer at pH 9.1 were added and incubated in a water bath for 60 min at 37°C, and then centrifuged at 1000 rpm for 5 min at 4°C. After the centrifugation time, 600 µL were separated from the supernatant and incubated for 3 min at boiling temperature. Finally, the tubes were cooled in an ice bath, after which 1,500 µL of p-dimethylaminobenzaldehyde (Sigma-Aldrich®) were added. The vortex tubes were homogenized, then incubated in a water bath for 20 min at 38°C and the absorbance of each tube was measured at 585 nm in a spectrophotometer. The variable used to quantify chitinase activity was the specific activity and was determined by the amount of released N-acetylglucosamine [26]. The calculation of the enzymatic activity was carried out according to the following equation [27]:
where:
OD = Optical density
Cot = Cotangent of the angle of the standard curve
Vassay = Volume of the test (cuvette)
Tincub = Incubation time
Vm = Volume of the sample (enzyme).
The specific activity was expressed in pkat/mg protein, as reported by [27].
The specific activity was determined according to the expression:
2.6.3 Quantification of β-1,3-Glucanases
The production of β-1,3-glucanases (EC 3.2.1.6) was determined according to the method described in previous reports [28,29]. The laminarin substrate (β-1,3 glucan polysaccharide) (Sigma-Aldrich®) was used as a stock solution with a concentration of 2 mg·mL−1, which was dissolved in 0.5 M sodium acetate buffer at pH 5.5.
For the reaction, 150 µL of laminarin were used together with enzyme extract and Somogyi reagent, which were subsequently incubated in a water bath for 40 min at 110°C. The reaction was stopped by using ice. After that, 150 µL of the Nelson reagent were added, and 1500 µL of distilled water were added while stirring gently. The reaction rate was calculated from a reading at 610 nm.
The standard curve was prepared from a D-glucose stock solution with a concentration of 0.008 mM mL−1, where the same procedure described above was performed. The calculation of the enzymatic activity and the specific activity was performed in the same manner as described for the chitinase enzymatic activity. The results of the specific activity of the β-1,3-glucanase enzyme were provided in pkat mg protein−1.
2.6.4 Quantification of Peroxidases
The POX (EC1.11.1.7) determination was carried out by a reaction mixture consisting of 0.25% (v/v) of guaiacol at 10 mM sodium phosphate buffer (pH 6 containing 10 mM hydrogen peroxide). A volume of 100 µL of the enzyme extract was added to initiate the reaction, which was measured by UV/VIS spectrophotometry. The activity was calculated by measuring the ratio at 470 nm/min = 0.01, which is defined as 1 unit of activity. The specific activity was expressed as U mg protein−1.
2.6.5 Quantification of Catalases
The activity of CAT (EC 1.11.1.6) was determined as reported elsewhere [30]. 100 µL of enzyme extract were added to 2,900 µl of a reaction mixture containing 20 mM hydrogen peroxide and 50 mM sodium phosphate buffer at pH 7. CAT activity was measured by controlling the absorbance reduction at 240 nm as result of the consumption of H2O2. Catalase activity was expressed as U mg protein−1, where one unit of enzymatic activity was defined as the decomposition of 1 µmol of H2O2 per minute.
To evaluate the morphological parameters, three plants were selected from each treatment. For the chlorophyll index, a Minolta SPAD 502 Plus chlorophyll meter equipment was used, with which the intensity of chlorophyll in the leaf was measured. The length of the aerial parts and roots was measured with a flexometer. Measurements were taken from the base of the plant to the axil of the youngest leaf. Stem diameter was measured at the base of the stem at a height of 1 cm above the substrate with the help of a vernier caliper. Fresh plant weight (foliage and root) was recorded in grams with the help of an Ohaus analytical balance.
2.8 Experimental Units and Treatments
The experimental units consisted of a black polyethylene bag (35 cm high × 20 cm wide), which contained 4 kg of compost as substrate. Transplanting was performed once the seedlings had grown for 25 days. The treatments were evaluated according to Table 1.
For the enzymatic activity experiment, a randomized complete block experimental design was used, with 10 treatments and 3 repetitions with 5 evaluations, adding up to a total of 300 experimental units. For the enzymatic activity of peroxidases and catalases, a completely randomized design with 10 treatments and 3 repetitions with 5 evaluations was used.
For data analysis, Statgraphics Centurion XVI software was used, whereby a simple variance analysis (ANOVA) was used. Tukey tests was applied to determine significant statistical differences between treatments, with an error of 0.05%.
3.1 Enzymatic Activity in S. lycopersicum Plant Roots
3.1.1 Activity of β-1,3-Glucanases
The activity of β-1,3-glucanases at 114 dat, the Azadirachta indica L. + Piper auritum Kunth (NH) treatment presented a significant difference (8.5 pkat mg protein−1) with respect to all treatments (Fig. 1), except for plants treated with Beauveria bassiana (Be). The treatment with B. bassiana + Fusarium oxysporum + A. indica A. Juss. + P. auritum Kunth (BeFuNH), showed a significant difference in the activity of β-1,3-glucanases with respect to all treatments, except for plants treated with B. bassiana. Also, the NH, Be and BeFuNH treatments showed significant statistical difference regarding the Q treatment.
Figure 1: Activity of β-1,3-glucanases in roots from S. lycopersicum plants at 114 dat. Different letters indicate statistically different averages according to Tukey’s test (p ≤ 0.05)
At 114 dat, it was observed that the BeFu treatment showed a significant difference in chitinase activity (0.85 pkat mg protein−1), with respect to the other treatments (Fig. 2). Also, the plants treated with the BeNH mixture presented a significant difference regards to all treatments, except for the plants treated with Fu and BeFu.
Figure 2: Chitinase activity in roots from S. lycopersicum plants at 114 dat. Different letters indicate statistically different averages according to Tukey’s test (p ≤ 0.05)
At 114 dat, it is observed that the NH treatment showed a significant difference in the activity of peroxidases (7.06 U mg protein−1), as seen in Fig. 3. Plants treated with NH showed a significant difference as compared to the plants receiving the other treatments. NH treatment plants showed the highest peroxidase activity (7.06 U/mg protein), followed by BeNH treatment plants (1.25 U/mg protein), the Fu treatment presented a significant statistical difference as compared to the C treatment.
Figure 3: Peroxidase activity in roots from S. lycopersicum plants at 114 dat. Different letters indicate statistically different averages according to Tukey’s test (p ≤ 0.05)
It was observed that the NH treatment had the highest catalase activity at 114 dat (203 U mg protein−1), showing significant differences with respect to all treatments (Fig. 4). BeFu treatment plants showed an increase in catalase enzymatic activity at the root of the plants, which is in contrasts with the observed effect in the plants of the Be and Fu treatments.
Figure 4: Catalase activity in roots from S. lycopersicum plants at 114 dat. Different letters indicate statistically different averages according to Tukey’s test (p ≤ 0.05)
The application of the combination of B. bassiana with the plant extracts did not have a detrimental effect on the morphometric parameters of the Solanum lycopersicum plants. However, the plants that were infused with Fusarium (Fu treatment) did exhibit effects on the mentioned parameters, as seen in Table 2. Fig. 5 shows that the plants treated with Fu present senescence in the leaves and have a smaller size, compared to the plants from the other treatments.
Figure 5: S. lycopersicum plants at 114 dat. (A) Treatment Q: chemical (Captan fungicide), (B) Treatment C (water), (C) Treatment NH (Azadirachta indica A. Juss. + Piper auritum Kunth), (D) Treatment Be (Beauveria bassiana), (E) Treatment BeNH (B. bassiana + A. indica A. Juss. + P. auritum Kunth), (F) Treatment BeFuNH (B. bassiana + F. oxysporum f. sp. lycopersici + A. indica A. Juss. + P. auritum Kunth), (G) Treatment FU (Fusarium oxysporum f. sp. lycopersici), (H) Treatment FuQ (F. oxysporum f. sp. lycopersici + Chemical), (I) Treatment BeFu (B. bassiana + F. oxysporum f. sp. lycopersici) and (J) Treatment FuNH (F. oxysporum f. sp. lycopersici + A. indica A. Juss. + P. auritum Kunth). Colored flags on a stake corresponds to a height of 25 cm
In this study, it was observed that treatments with A. indica A. Juss. and Piper auritum Kunth plant extracts presented a significant difference in the activity of the enzyme β-1,3-glucanases during experimentation. This behavior was observed as well when applying the entomopathogenic fungus Beauveria bassiana.
In this regard, previous studies have revealed that enzymatic activity increases as a defense mechanism against environmental stress, such as a fungal infection [31,32]. The mixture of extracts from A. indica and P. auritum potentiate the activity of β-1,3-glucanases in S. lycopersicum plants, when they are inoculated with F. oxysporum or B. bassiana. This is possibly explained by the presence of azaridachtin and safrol metabolites that are contained in these extracts, which increase the enzymatic activity of β-1,3-glucanases in the plants. Randomidachtin is the main secondary metabolite found in Azadirachta indica A. Juss. Limonoids are tetratriterpenes that have triterpene as a precursor. Plants that have limonoids, in addition to insecticidal activity, have other applications, such as antitumor, antifungal, bactericidal and antiviral, which suggests a role in the defense of the plant against certain microorganisms [33]. Azadirachtin is a tetraterpenoid that is characteristic of the Meliaceae family, especially in the A. indica A. Juss. tree. This compound is found in the bark, leaves, fruits and, mainly, in the seed of the tree [34].
Barra-Bucarei et al. [35] suggested that the action of B. bassiana as an endophyte increases the plant’s ability to resist the attack of pathogens such as B. cinerea and Fusarium ssp [36]. In tomatoes, chili peppers and maize plants, B. bassiana acts as an opportunistic parasite on various phytopathogens, as it has pathogenic and saprophytic habits. This behavior increases the plant’s activity through the production of chitinase enzymes, glucanases, proteases and lipases, which are important for the invasion and degradation of the phytopathogenic target [37,38].
In this study, it was observed that treatments with plant extracts from A. indica A. Juss. and P. auritum Kunth, as well as the entomopathogenic fungus B. bassiana, showed an increase in chitinase activity in the S. lycopersicum plant when B. bassiana + F. oxysporum (BeFu) were applied to the plants. It is possible that B. bassiana potentiates the chitinase activity in the plant when competing with F. oxysporum. When measuring chitinase activity in the other treatments (C and Be), the corresponding values are lower, and the same is observed with the enzymatic activity from the BeNH treatment (B. bassiana + A. indica + P. auritum).
B. bassiana infects the pathogenic fungus by direct penetration of the cuticle or cell wall by using different enzymes. Chitinases are important to facilitate each stage of the fungal infection, such as conidia germination, growth of the hypha and even the penetration of these structures into the affected organism [39]. B. bassiana produces numerous secondary metabolites including beauvericin, bassianine, beauverolides, bassianolides, oosporein, and bassianolone, among others [39]. Out of these compounds, beauvericin is particularly interesting due to its antimicrobial properties, and is secreted by the genera Beauveria and Isaria [40]. The induction of chitinases and glucanases was also evaluated in S. lycopersicum seedlings after inoculation with Fusarium solani, Trichoderma harzianum, or both. It was established that both microorganisms induced chitinases in the plant, albeit at different times [41].
It was observed that the treatment consisting of plant extracts from A. indica + P. auritum had the highest enzymatic peroxidases activity, compared with the other evaluated treatments. Similarly, the treatment with F. oxysporum f. sp. lycopersici presented greater activity as compared to the control treatment. In this experiment, it was observed that the A. indica + P. auritum plant extracts caused the S. lycopersicum plant to increase its peroxidase activity significantly, which enabled a protective feature for the plants.
The production and accumulation of reactive oxygen species (ROS) in plants causes damage to different cell organelles, as well as a malfunction in the cell membrane due to its peroxidation, which causes degradation of biological macromolecules, ultimately leading to cell death [42,43].
On the other hand, Tian et al. [44] performed an analysis of enzymatic activities, including peroxidase, in roots of 2 genotypes of Pisum sativum with different susceptibilities to Fusarium oxysporum and Fusarium solani, where they found an increase in activity for this enzyme in infected roots from day 2 until day 28 post-inoculation. In apples, an increase in peroxidase activity was reported, as well as in the lignin content. In turn, a decrease in the incidence of the disease caused by Penicillium expansum [45].
In our study, it was observed that applying the A. indica + P. auritum plant extracts together resulted in S. lycopersicum plants producing significant catalase activity. This is something that did not occur when these were combined with B. bassiana + F. oxysporum (BeFuNH) or with B. bassiana (BeNH) and F. oxysporum (FuNH) separately. It was found that catalase activity in S. lycopersicum plants that were treated only with distilled water (C) was low, which indicated that plant extracts can lead to a better defense in S. lycopersicum against attacks by fungal pathogens.
When aqueous extracts of neem (A. indica) and willow (Salix babylonica) were used on S. lycopersicum plants, a reduced incidence of F. oxysporum wilt disease was observed by almost 30% in S. lycopersicum seedlings. This was likely achieved by increasing the activities of the antioxidant defensive enzymes secreted by the S. lycopersicum plant, such as superoxide dismutase (SOD), catalase (CAT) and peroxidase (POX) [1]. Also, the use of A. indica + P. auritum plant extracts made it possible for the S. lycopersicum plants to significantly increase peroxidase activity, which enabled plant protection. Normally, catalase protects the plant against the oxidative stress that is exerted by reactive oxygen species [46]. CAT is one of the antioxidant enzymes whose function is to regulate the production of free radicals and their metabolites. Its role is essential in protecting to the cell from ROS, and its inhibition can cause ROS accumulation and cell damage [47].
Plants produce secondary metabolites such as tannins, flavonoids, quinones, phenols, terpenoids, and alkaloids, which protect them from attacks by herbivores and microorganisms. In this sense, extracts, or essential oils from aromatic and/or medicinal plants have been used for pest control, as they have shown efficacy in the different development stages of diverse insect pests [48]. Extracts and/or essential oils also shown antimicrobial activity against various microorganisms [44]. Bedini et al. [49] reported that plant extracts have become relevant for the control of some pests, as they are a low-cost option that is also friendly to the environment and have no residual effects. In addition, the use of organic extracts reduces pest or microorganism resistance to the active compound [49].
Organic insecticides have shown more efficiency when combined with synthetic or microbial insecticides [50]. The combination of entomopathogenic fungi and pesticides (EPF) have contributed towards investigation of new products for integrated pest management (IPM) [51]. This has resulted in improving the effect of the substances that are used to control pests because the application doses are minimized, thus decreasing the resistance of pests to the active compound, while also reducing the risks of contamination [52].
The compatibility of the plant extracts and EPF depends on the qualitative and quantitative factors that are related to the composition of the secondary metabolites that are present, which can cause a negative effect on the EPF [53]. Islam et al. [54] reported that the combination of B. bassiana and neem extracts caused a high mortality rate in B. tabaci nymphs compared to individual treatments. Halder et al. [55] evaluated the efficiency of four EPF, including B. bassiana, which was evaluated in an attempt to control different pests, where it was used by itself and in combination with neem extract at a 1:1 ratio. The combination of the extract with B. bassiana was the treatment where a higher mortality rate was noted, as compared to the other treatments. This indicates that the relationship between the plant extract and the entomopathogenic microorganism is an important factor that should be considered in IPM. Jaber et al. [56] reported that the use of B. bassiana or M. brunneum, in combination with C. procera or I. viscosa plant extracts, showed an increase in the mortality of B. tabaci Gennadius. It has been reported that eucalyptus extracts with B. bassiana had a mortality rate of 87% in B. tabaci after 5 days of treatment [57].
López-López et al. [58] reported an increase in variables such as chlorophyll content, stem diameter, as well as other morphometric variables when tomato plants were co-inoculated with B. bassiana. These parameters were similar in our study, as plants treated with BeFu and BeNH had a greater height, stem diameter and root length, as well as a higher chlorophyll content. This is likely because B. bassiana solubilizes nitrogen and phosphorus, which helps to increase biomass in shoots and roots, as well as increasing photosynthetic activity.
The treatments with the A. indica and P. auritum extracts showed a positive stimulus in the enzymatic activity of β-1,3-glucanases, peroxidases and catalases, while the combination of B. bassiana and plant extracts increased the enzymatic activity of chitinases and peroxidases. This serves as proof that plant extracts alone or in combination with B. bassiana induce defense mechanisms which confer greater protection to S. lycopersicum plants in the presence of F. oxysporum f. sp. lycopersici, which is likely related to the content of azadirachtin and safrol in the plant extracts.
Application of B. bassiana and plant extracts mitigate the damage caused by F. oxysporum f. sp. lycopersici in S. lycopersicum plants. This occurs without affecting the morphometric parameters of the plants. Thus, they could be considered for the development of tools for the biocontrol of this pathogen in tomato plants.
Acknowledgement: We would like to thank the Universidad Autónoma Agraria Antonio Narro and the Tecnológico Nacional de México Campus Tlajomulco for donating the strains used in this research. We also show gratitude towards CINVESTAV Zacatenco, the Soil Ecology Laboratory and Dr. Luc Dendooven, and kindly thank the Consejo Nacional de Ciencia y Tecnología (CONACyT)-México for the scholarship awarded to José Adrian Perez Robles and the Electrón S.A. de C.V. Drinking Water Company.
Funding Statement: The project was funded by the Tecnológico Nacional de México (TECNM): Project No. 6602.18-P.
Author Contributions: The authors confirm contribution to the paper as follows: Study conception and design: M-M JA.; Analysis and interpretation of results: P-R JA., R-V VM., L-G CA., M-M JA.; Supervision: M-M JA., L-G CA.; Translation and editing of the manuscript: L-G CA. All authors reviewed the results and approved the final version of the manuscript.
Conflicts of Interest: The authors declare that they have no conflicts of interest to report regarding the present study.
References
1. Olatunji, T. L., Afolayan, A. J. (2018). The suitability of chili pepper (Capsicum annuum L.) for alleviating human micronutrient dietary deficiencies: A review. Food Science & Nutrition, 6(8), 2239–2251. https://doi.org/10.1002/fsn3.790 [Google Scholar] [PubMed] [CrossRef]
2. Pramanik, K., Mohapatra, P. P. (2017). Role of auxin on growth, yield and quality of tomato–A review. International Journal of Current Microbiology and Applied Science, 6(11), 1624–1636. https://doi.org/10.20546/ijcmas.2017.611.195 [Google Scholar] [CrossRef]
3. Attia, M. S., Abdelaziz, A. M., Al-Askar, A. A., Arishi, A. A., Abdelhakim, A. M. et al. (2022). Plant growth-promoting fungi as biocontrol tool against Fusarium wilt disease of tomato plant. Journal of Fungi, 8(8), 775. https://doi.org/10.3390/jof8080775 [Google Scholar] [PubMed] [CrossRef]
4. Dayarathne, M. C., Mridha, A. U., Wang, Y. (2020). Diagnosis of fungal plant pathogens using conventional and molecular approaches. In: Diagnostics of plant diseases, pp. 19–42. London: IntechOpen. https://doi.org/10.5772/intechopen.94980 [Google Scholar] [CrossRef]
5. Dean, R., Van-Kan, J. A., Pretorius, Z. A., Hammond-Kosack, K. E., di Pietro, A. et al. (2012). The Top 10 fungal pathogens in molecular plant pathology. Molecular Plant Pathology, 13(14), 414–430. https://doi.org/10.1111/j.1364-3703.2011.00783.x [Google Scholar] [PubMed] [CrossRef]
6. Glick, B. R. (2012). Plant growth-promoting bacteria: Mechanisms and applications. Scientifica, 2012(5), 1–15. https://doi.org/10.6064/2012/963401 [Google Scholar] [PubMed] [CrossRef]
7. Abdelaziz, A. M. (2022). Inhibition of aspergillus flavus growth and aflatoxin production in Zea mays L. using endophytic Aspergillus fumigatus. Journal of Fungi, 8(5), 582. https://www.mdpi.com/2309-608X/8/5/482# [Google Scholar]
8. Saranraj, P., Jayaparakash, A. (2017). Agrobeneficial entomopathogenic fungi—Beauveria bassiana: A review. Indo-Asian Journal of Multidisciplinary Research, 3(2), 1051–1087. [Google Scholar]
9. Vidal, S., Jaber, L. R. (2015). Entomopathogenic fungi as endophytes: Plant-endophyte-herbivore interactions and prospects for use in biological control. Current Science, 109, 46–54. http://www.jstor.org/stable/24905690 [Google Scholar]
10. Allegrucci, N., Velazquez, M. S., Russo, M. L., Perez, E., Scorsetti, A. C. (2017). Endophytic colonization of tomato by the entomopathogenic fungus Beauveria bassiana: The use of different inoculation techniques and their effects on the tomato leafminer Tuta absoluta (Lepidoptera: Gelechiidae). Journal of Plant Protection Research, 57(4), 206–211. https://doi.org/10.1515/jppr-2017-0045 [Google Scholar] [CrossRef]
11. Sinno, M., Ranesi, M., Gioia, L., D’errico, G., Woo, S. L. (2020). Endophytic fungi of tomato and their potential applications for crop improvement. Agriculture, 10(12), 587. https://doi.org/10.3390/agriculture10120587 [Google Scholar] [CrossRef]
12. Culebro-Ricaldi, J. M., Ruíz-Valdiviezo, V. M., Rodríguez-Mendiola, M. A., Ávila-Miranda, M. E., Gutiérrez-Miceli, F. A. et al. (2017). Propiedades antifúngicas de cepas de Beauveria bassiana contra Fusarium oxysporum f. sp. Lycopersici raza 3 en cultivo de tomate. Journal of Environmental Biology, 38, 821–827. https://doi.org/10.22438/jeb/38/5/MRN-412 [Google Scholar] [CrossRef]
13. Ownley, B. H., Pereira, R. M., Klingeman, W. E., Quigley, N. B., Leckie, B. M. (2004). Beauveria bassiana, a dual-purpose biocontrol organism, with activity against insect pest and plant pathogens. In: Lartey, R. T., Caesar, A. J. (Eds.Emerging concepts in plant health management, pp. 256–269. Kerala, India: Research Signpost. [Google Scholar]
14. Montes-Molina, J. A., Nuricumbo-Zarate, I. H., Hernández-Díaz, J., Gutiérrez-Miceli, F. A., Dendooven, L. et al. (2014). Characteristics of tomato plants treated with leaf extracts of neem (Azadirachta indica A. Juss. (L.)) and mata-raton (Gliricidia sepium (Jacquin)A greenhouse experiment. Journal of Environmental Biology, 35(4), 935–942. [Google Scholar] [PubMed]
15. Wylie, M. R., Merrell, D. S. (2022). The antimicrobial potential of the neem tree azadirachta indica. Frontiers in Pharmacology, 13, 891535. https://doi.org/10.3389/fphar.2022.891535 [Google Scholar] [PubMed] [CrossRef]
16. Garcia, A., Leyva, M., Martinez, J., Stashenko, E. (2007). Determinación de la composición química y actividad antioxidante in vitro del aceite esencial de Piper auritum Kunth (Piperaceae) difundida en la Costa colombiana. Scientia et Technica, 1(33), 439–442. [Google Scholar]
17. Salleh, W. (2021). A systematic review of botany, phytochemicals and pharmacological properties of “Hoja santa” (Piper auritum Kunth). Zeitschrift für Naturforschung C, 76(3–4), 93–102. https://doi.org/10.1515/znc-2020-0116 [Google Scholar] [PubMed] [CrossRef]
18. Sánchez, Y., Pino, O., Correa, T. M., Naranjo, E., Iglesia, A. (2009). Estudio químico y microbiológico del aceite esencial de Piper auritum Kunth (Caisimón de anís). Revista de Protección Vegetal, 24(1), 39–46. [Google Scholar]
19. Arora, A., Sairam, R. K., Srivastava, G. C. (2002). Oxidative stress and antioxidative system in plants. Current Science, 82(10), 1227–1238. http://www.jstor.org/stable/24107045 [Google Scholar]
20. Ahmed, I. H., Labuschagne, N., Korsten, L. (2007). Screening rhizobacteria for biological control of Fusarium root and crown rot of sorghum in Ethiopia. Biological Control, 40(1), 97–106. https://doi.org/10.1016/j.biocontrol.2006.07.017 [Google Scholar] [CrossRef]
21. Akanmu, A. O., Odebode, A. C., Abiala, M. A., Aiyelaagbe, O. O., Olaoluwa, O. O. (2014). Inhibition of Fusarium pathogens in millet by extracts of Jatropha curcas and Mangifera indica. International Journal of Plant Biology & Research, 2(1), 1–8. [Google Scholar]
22. Ardila, H. D., Higuera, B. L. (2005). Polyphenoloxidase and β-1,3-glucanase differential induction in carnation (Dianthus caryophyllus) infectedby Fusarium oxysporum f. sp. dianthi race 2. Acta Biológica Colombiana, 10(2), 61–64. [Google Scholar]
23. Bradford, M. M. (1976). A rapid and sensitive method for the quantitation of microgram quantities of protein utilizing the principle of protein-dye binding. Analytical Biochemistry, 72(1–2), 248–254. https://doi.org/10.1016/0003-2697(76)90527-3 [Google Scholar] [CrossRef]
24. Boller, T., Gehri, A., Mauch, F., Vogeli, U. (1983). Chitinase in bean leaves: Induction by ethylene, purification, properties and possible function. Planta, 157(1), 22–31. https://doi.org/10.1007/BF00394536 [Google Scholar] [PubMed] [CrossRef]
25. Reissing, J. L., Strominger, J. L., Letair, L. L. (1955). A modified colorimetric method for the stimulation of N-acetyl amino sugar. Journal of Biological Chemistry, 217, 959–966. [Google Scholar] [PubMed]
26. Solórzano, E., Meneses, A. R., Rodríguez, Y., Pérez, E., Fernández, A. et al. (2001). Inducción de cinco sistemas enzimáticos en la simbiosis tomate-micorriza arbuscular (MA). Revista Protección Vegetal, 16(1), 30–39. [Google Scholar]
27. Nelson, N. (1944). A photometric adaptation of the Somogyi method for determination of glucose. Journal of Biological Chemistry, 153, 375–380. [Google Scholar]
28. Somogyi, M. (1952). Notes on sugar determination. Journal of Biological Chemistry, 195(1), 19–23. https://doi.org/10.1016/S0021-9258(19)50870-5 [Google Scholar] [CrossRef]
29. Aebi, H. (1974). Catalase. In: Bergmeyer, H. U. (Ed.Methods of enzymatic analysis, pp. 673–677. Weinheim/NewYork, USA: Verlag Chemie/Academic Press Inc. [Google Scholar]
30. Miranda Júnior, R. N., Dolabela, M. F., da Silva, M. N., Póvoa, M. M., Maia, J. G. (2012). Antiplasmodial activity of the andiroba (Carapa guianensis Aubl., Meliaceae) oil and its limonoid-rich fraction. Journal of Ethnopharmacology, 142(3), 679–683. https://doi.org/10.1016/j.jep.2012.05.037 [Google Scholar] [PubMed] [CrossRef]
31. Shanmugan, V., Kanoujia, N. (2011). Biological management of vascular wilt of tomato caused by Fusarium oxysporum f. sp. lycopersici by plant growth promoting rhizobacterial mixture. Biological Control, 57(2), 85–93. https://doi.org/10.1016/j.biocontrol.2011.02.001 [Google Scholar] [CrossRef]
32. Reyes-Zambrano, S. J., Lecona-Guzmán, C. A., Gutiérrez-Miceli, F. A., Santana-Buzzy, N., Islas-Flores, I. et al. (2020). Scanning electron microscopy and enzymatic analysis in Agave americana during Fusarium oxysporum infection. Revista Mexicana de Fitopatología, 38(3), 408–419. [Google Scholar]
33. Valladares, G., Defago, M., Palacios, T., Carpinella, S., M., C. (1997). Laboratory evaluation of Melia azedarach (Meliaceae) extracts against the elm leaf beetle (Coleoptera: Chrysomelidae). Journal of Economic Entomology, 90(3), 747–750. https://doi.org/10.1093/jee/90.3.747 [Google Scholar] [CrossRef]
34. Jetiyanon, K. (2007). Defensive-related enzyme response in plants treated with a mixture of Bacillus strains (IN937a and IN937b) against different pathogens. Biological Control, 42(2), 178–185. https://doi.org/10.1016/j.biocontrol.2007.05.008 [Google Scholar] [CrossRef]
35. Barra-Bucarei, L., France-Iglesias, A., Gerding-González, M., Silva-Aguayo, G., Carrasco-Fernández, J. et al. (2020). Antifungal Activity of Beauveria bassiana Endophyte against Botrytis cinerea in two Solanaceae crops. Microorganisms, 8(1), 65. https://doi.org/10.3390/microorganisms8010065 [Google Scholar] [PubMed] [CrossRef]
36. Orole, O. O., Adejumo, T. O. (2009). Activity of fungal endophytes against four maize wilt pathogens. African Journal of Microbiology Research, 3(12), 969–973. [Google Scholar]
37. Clark, M. M. (2006). Biological control methods for damping-off of tomato seedlings caused by Pythium myriotylum (Master’s Thesis). Universidad de Tennessee, Knoxville, USA. [Google Scholar]
38. Peteira, B., Gonzáles, I., Arias, Y., Fernández, A., Miranda, I. et al. (2011). Caracterización bioquímica de seis aislamientos de Beauveria bassiana (BALSAMO) Vuillemin. Revista de Protección Vegetal, 26(1), 16–22. [Google Scholar]
39. Ortiz-Urquiza, A., Luo, Z., Keyhani, N. O. (2015). Improving mycoinsecticides for insect biological control. Applied Microbiology and Biotechnology, 99(3), 1057–1068. https://doi.org/10.1007/s00253-014-6270-x [Google Scholar] [PubMed] [CrossRef]
40. Wang, Q., Xu, L. (2012). Beauvericin, a bioactive compound produced by fungi: A short review. Molecules, 17(3), 2367–2377. https://doi.org/10.3390/molecules17032367 [Google Scholar] [PubMed] [CrossRef]
41. Chatterton, S., Punja, Z. K. (2009). Chitinase and β-1, 3-glucanase enzyme production by the mycoparasite Clonostachys rosea f. catenulata against fungal plant pathogens. Canadian Journal of Microbiology, 55(4), 356–367. https://doi.org/10.1139/w08-156 [Google Scholar] [PubMed] [CrossRef]
42. Rajput, V. D., Harish-Singh, R. K., Verma, K. K., Sharma, L., Quiroz-Figueroa, F. R. et al. (2021). Recent developments in enzymatic antioxidant defence mechanism in plants with special reference to abiotic stress. Biology, 10(4), 267. https://doi.org/10.3390/biology10040267 [Google Scholar] [PubMed] [CrossRef]
43. Levine, A., Tenhhaaken, R., Dixon, R., Lamb, C. (1994). H2O2 from the oxidative burst orchestrates the plant hypersensitive disease resistance response. Cell, 79(4), 583–593. https://doi.org/10.1016/0092-8674(94)90544-4 [Google Scholar] [PubMed] [CrossRef]
44. Tian, S., Wan, Y., Qin, G., Xu, Y. (2006). Induction of defense responses against Alternaria rot by different elicitors in harvested pear fruit. Applied Microbial and Cell Physiology, 70(6), 729–734. https://doi.org/10.1007/s00253-005-0125-4 [Google Scholar] [PubMed] [CrossRef]
45. Valentines, M., Vilaplana, R., Torres, R., Usall, J., Larrigaudiere, C. (2005). Specific roles of enzymatic browning and lignification in apple disease resistance. Postharvest Biology and Technology, 36(3), 227–234. https://doi.org/10.1016/j.postharvbio.2005.01.002 [Google Scholar] [CrossRef]
46. Valenzuela-Cota, D. F., Buitimea-Cantúa, G. V., Plascencia-Jatomea, M., Cinco-Moroyoqui, F. J., Martínez-Higuera, A. A. et al. (2019). Inhibition of the antioxidant activity of catalase and superoxide dismutase from fusarium verticillioides exposed to a jacquinia macrocarpa antifungal fraction. The Journal of Environmental Science and Health, Part B, 54(8), 647–654. https://doi.org/10.1080/03601234.2019.1622978 [Google Scholar] [PubMed] [CrossRef]
47. Ahmed, M., Peiwen, Q., Gu, Z., Liu, Y., Sikandar, A. et al. (2020). Insecticidal activity and biochemical composition of Citrullus colocynthis, Cannabis indica and Artemisia argyi extracts against cabbage aphid (Brevicoryne brassicae L.). Scientific Reports, 10(1), 522. https://doi.org/10.1038/s41598-019-57092-5 [Google Scholar] [PubMed] [CrossRef]
48. González-Lamothe, R., Mitchell, G., Gattuso, M., Diarra, M. S., Malouin, F. et al. (2009). Plant antimicrobial agents and their effects on plant and human pathogens. International Journal of Molecular Sciences, 10(8), 3400–3419. https://doi.org/10.3390/ijms10083400 [Google Scholar] [PubMed] [CrossRef]
49. Bedini, S., Guarino, S., Echeverria, M. C., Flamini, G., Ascrizzi, R. et al. (2020). Allium sativum, Rosmarinus officinalis, and Salvia officinalis essential oils: A spiced shield against blowflies. Insects, 11(3), 143. https://doi.org/10.3390/insects11030143 [Google Scholar] [PubMed] [CrossRef]
50. Isman, M. B. (2006). Botanical insecticides, deterrents, and repellents in modern agriculture and an increasingly regulated world. Annual Review of Entomology, 51(1), 45–66. https://doi.org/10.1146/annurev.ento.51.110104.151146 [Google Scholar] [PubMed] [CrossRef]
51. Neves, P. M. O. J., Hirose, E., Tchujo, P. T. (2001). Compatibility of entomopathogenic fungi with neonicotinoid insecticides. Neotropical Entomology, 30(2), 263–268. https://doi.org/10.1590/S1519-566X2001000200009 [Google Scholar] [CrossRef]
52. Usha, J., Babu, M. N., Padmaja, V. (2014). Detection of compatibility of entomopathogenic fungus Beauveria bassiana (Bals.) Vuill. with pesticides, fungicides and botanicals. International Journal of Plant, Animal and Environmental Sciences, 4(2), 613–624. [Google Scholar]
53. Ribeiro, L. P., Blume, E., Bogorni, P. C., Dequech, S. T. B., Brand, S. C. et al. (2012). Compatibility of Beauveria bassiana commercial isolate with botanical insecticides utilized in organic crops in Southern Brazil. Biological Agriculture and Horticulture, 28(4), 223–240. https://doi.org/10.1080/01448765.2012.735088 [Google Scholar] [CrossRef]
54. Islam, M. T., Castle, S. J., Ren, S. X. (2010). Compatibility of the insect pathogenic fungus Beauveria bassiana with neem against sweetpotato whitefly, Bemisia tabaci, on eggplant. Entomologia Experimentalis et Applicata, 134(1), 28–34. https://doi.org/10.1111/j.1570-7458.2009.00933.x [Google Scholar] [CrossRef]
55. Halder, J., Rai, A. B., Kodandaram, M. H. (2013). Compatibility of neem oil and different entomopathogens for the management of major vegetable sucking pests. National Academy Science Letters, 36(1), 19–25. https://doi.org/10.1007/s40009-012-0091-1 [Google Scholar] [CrossRef]
56. Jaber, L. R., Araj, S. E., Qasem, J. R. (2018). Compatibility of endophytic fungal entomopathogens with plant extracts for the management of sweetpotato whitefly Bemesia tabaci Gennadius (Homoptera: Aleyrodidae). Biological Control, 117(10), 164–171. https://doi.org/10.1016/j.biocontrol.2017.11.009 [Google Scholar] [CrossRef]
57. Ali, S., Farooqi, M. A., Ullah, M. I., Qureshi, A. K., Siddique, B. et al. (2018). Compatibility of entomopathogenic fungi and botanical extracts against the wheat aphid, Sitobion avenae (Fab.) (Hemiptera: Aphididae). Egyptian Journal of Biological Pest Control, 28(1), 97. https://doi.org/10.1186/s41938-018-0101-9 [Google Scholar] [CrossRef]
58. López-López, H., Ruiz-Lau, N., Meza-Gordillo, R., Ruiz-Valdiviezo, V. M., Robledo-Luchetti, J. G. et al. (2023). Antifungal potential of Beauveria bassiana on solanum lycopersicum L. Infected with Fusarium oxysporum f. sp. lycopersici. Phyton-International Journal of Experimental Botany, 92(4), 1235–1255. https://doi.org/10.32604/phyton.2023.025716 [Google Scholar] [CrossRef]
Cite This Article
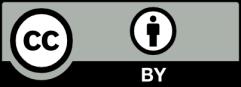