Open Access
ARTICLE
Ellagic Acid Enhances Antioxidant System Activity and Maintains the Quality of Strawberry Fruit during Storage
1 College of Chemistry and Material Science, Shandong Agricultural University, Taian, 271018, China
2 Agriculture and Rural Bureau of Penglai District, Yantai, 265699, China
3 Laiyang Inspection and Testing Center, Yantai, 265299, China
* Corresponding Author: Dandan Huang. Email:
Phyton-International Journal of Experimental Botany 2024, 93(1), 15-28. https://doi.org/10.32604/phyton.2023.045621
Received 02 September 2023; Accepted 14 November 2023; Issue published 26 January 2024
Abstract
Ellagic acid (EA) is a natural antioxidant, widely present in a lot of forms’ soft fruits, nuts, and other plant tissues, and helpful for promoting human health; however, its protective effect on postharvest fruit and improving the quality index of postharvest fruit have rarely been studied. In this experiment, the strawberries were soaked in 0, 100, 200, 300, 400, and 500 mg L−1 EA, respectively, and the influential EA on fruit quality and the antioxidant system of strawberries were studied. Compared with the control, EA treatment can reduce the browning degree and rotting rate of strawberry fruit during storage and augment the soluble solid content (SSC). EA treatment can also increase the content of related stuff and enzyme activity in antioxidant systems; the gene expression level of polyphenol oxidase (PPO) in strawberries treated with EA was always down-regulated, correspondingly, the expression of other antioxidant enzyme genes was enhanced. Among the strawberry fruits treated with EA of different concentrations, 300 mg L−1 EA had the best effect in the process of strawberry preservation. The results suggested that the proper concentration of exogenous EA at 300 mg L−1 could maintain strawberries’ quality and enhance the antioxidant system by improving the activities of antioxidative enzymes and the ascorbate-glutathione (AsA-GSH) cycle during storage.Keywords
Strawberry, a berry fruit with bright color and unique taste, rich in nutrients, is a good source of ellagic acid (EA), anthocyanins, and catechins, which have antioxidant functions and a good role in reducing cardiovascular disease. In addition, diets with adequate strawberries are suitable for the anti-aging brain [1]. So, strawberry is an essential origin of bioactive substances for human health [2]. However, strawberries are not easy to store after postharvesting, leading to rot and odor and seriously restricting their edible and commercial value [3,4]. Therefore, finding a simple and effective preservation technology is the key to expanding the market for strawberries.
At present, the preservation methods of strawberries include chemical, physical, and biological preservation. Studies have shown that a comestible coating based on inartificial Aloe vera (AV) gel combined with ascorbic acid reduces total aerobic mesophilic, yeasts, and mold number so that strawberries can be stored for a more extended time [5]. Alginate oligosaccharide enhances the strawberries’ shelf life by regulating the abscisic acid content [6]. Appropriately increasing the concentration of carbon dioxide delays strawberries’ softening and decay rate and prolongs their storage time [7]. Chitosan coatings as a carrier of nisin and natamycin are used to reduce the oxygen consumption of fresh strawberries to maintain their quality [8].
The excessive addition of reactive oxygen species (ROS) may cause oxidative damage and cell death. In plants, there is an active oxygen scavenging system composed of both an enzymatic defense system and a non-enzymatic oxidation resistance defense system [9,10]. The oxidation resistance system covering superoxide dismutase (SOD), peroxidase (POD), and catalase (CAT) could directly trim down the ROS levels by scavenging active oxygen. The ascorbate-glutathione (AsA-GSH) cycle is vital to the non-enzymatic antioxidant system. On the one hand, ROS produced in plants can be directly eliminated through the catalytic action of monodehydroascorbate reductase (MDHAR), dehydroascorbate reductase (DHAR), glutathione reductase (GR), and ascorbate peroxidase (APX). For another, the ASA-GSH cycle can promote the production of ascorbic acid (AsA) and glutathione (GSH), thus maintaining the balance of oxidation and reducing (REDOX) substances in plants [11,12]. Bioactive molecules have been reported to heighten the oxidation resistance system of fruits to mitigate the oxidic lesions correlated with the senescence of fruits and keep the high quality of fruits during storage [13,14]. Ellagic acid (EA), a polyphenol compound widely existing in plant tissues, has superb antioxidant activity and antimicrobial properties and is indispensable in the postharvest fruit production system [15]. Relevant studies have also begun applying EA to other fruit preservation to extend its storage time and improve its quality during storage, such as EA prevents ulceration caused by bacteria in kiwifruit [16], enhances oxidation resistance liveness, and maintains the postharvest character of kumquat fruit [17].
Although there are many methods to prolong the storage period of strawberries, there are few reports about the preservation outcome of EA on postharvest strawberries. In this experiment, strawberry was soaked with different concentrations of EA, the ramifications of EA on the oxidation resistance system of strawberries were studied, and the optimal EA concentration for postharvest strawberries was optimized to provide a potential method of utilizing EA in the conservation of postharvest fruit.
2.1 Fruit Material and Treatments
Strawberries (Fragaria ananassa cv. Tianbao) were picked from Taian, China. The strawberries, which were physiologically mature and had no pests and mechanical damage, were removed from the field heat at 4°C for 12 h. After soaked in double-distilled water (as the control) and 100, 200, 300, 400, and 500 mg L−1 ellagic acid (EA, Macklin., Ltd., Shanghai, China) solution for 5 min, the strawberries were dried with the natural wind and then stored at 4°C. Specimens were fetched at 0, 1, 2, 3, and 4 days throughout the period to evaluate browning degree, rotting rate, and soluble solid content (SSC). Meanwhile, strawberry fruit was cut into fragments and then stockpiled at −80°C for the subsequent analysis of enzymes, metabolites, and RNA extraction. One kilogram of strawberry fruit was specimen for each repetition, and biological tests were repeated three times.
2.2 Determination of the Physiochemical Parameters of Strawberries
The browning degree was measured with an ultraviolet spectrophotometer (UV2450, Shimadzu, Japan) [18]. Strawberries tissue (2 g) was mixed evenly in 5 mL of 95% (v/v) ethanol and centrifuged at 10 000 × g for 10 min at 4°C. The supernatant was collected and shifted to the new centrifuge tubes, and then its absorbance at 410 nm was measured by ultraviolet spectrophotometer, and the browning degree was indicated to absorbance at 410 nm (OD410). The soluble solid content (SSC) was measured through the medium of a portable refractometer (WY015R, Syas Technology Ltd., China) and implied as °Brix. The rotting rate was classified according to the rotting area on the surface of strawberries: no rotting area was defined as grade 0, a rotting area less than 1/4 was as grade 1, and a rotting area between 1/4 and 1/2 was as grade 2. If the rotting area was more than 1/2, it was grade 3. Rotting rate = ∑ (grade × number of samples at this grade) / (highest grade × the total number of samples) × 100%.
2.3 Determination of the Content of Malondialdehyde and Reactive Oxygen Species
Malondialdehyde (MDA) content was gauged with a UV2450 spectrophotometer [13]. Strawberries tissue (5 g) was added to a test tube that contained 10 mL of 0.6 mol L−1 trichloroacetic acid (TCA) solution, then left for two minutes to mix thoroughly, and then centrifuged at 4°C at 5 000 × g for 10 min to obtain the upper liquid. Carefully transferred the upper liquid to the new clean centrifuge tubes and marked them. The extract (50 μL) was thoroughly blended with 1 mL of 10% TCA and boiled at 95°C for 20 min. Centrifuged at 12 000 × g and 4°C for 10 min, the upper layer liquid was absorbed and recorded at 600, 532, and 450 nm using the UV2450. MDA content was expressed as nmol g−1 FW (fresh weight, FW) and calculated with the formula:
2.4 Determination of the Activities of Polyphenol Oxidase, Peroxidase, Superoxide Dismutase, and Catalase
Ground strawberries (10 g) were thoroughly commixed with 30 mL 50 mmol L−1 sodium phosphate buffer solution (pH 7.8) and centrifuged at 4°C, 12 000 × g for 15 min. The catalase (CAT) activity was determined by the drop-off in absorbance caused by the decomposition of H2O2 [20] with the wavelength of the spectrophotometer at 240 nm. One unit of CAT activity was described as a variation in absorbance of 0.01 per min and stated as U g−1 FW. The polyphenol oxidase (PPO) activity was adopted in line with the scheme described by Lei et al. [18]. The mixture involved 1.0 mL 0.1% catechol, 1.0 mL of the extracting solution, and 2 mL 0.1 mol L−1 phosphate buffer (pH 6.5). The change of absorbance was measured at 420 nm. One unit of PPO activity description and unit representation is the same as CAT. The superoxide dismutase (SOD) activity was used by referring to Wei et al. [21]. One unit of SOD activity was described as the quantity of ferment inhibiting 50% photoreduction ability under assay conditions, and the unit description is the same as CAT. Peroxidase (POD) activity was measured in compliance with Ma et al. [14]. The absorbance of the fluids was measured at 580 nm. The unit of POD activity was represented as the same as CAT.
2.5 Determination of the Metabolite Content and Enzyme Activities in the Ascorbate–Glutathione Cycle
Determining the metabolite content and enzyme activities was referred to the method of Ma et al. [13]. Glutathione (GSH) and ascorbic acid (AsA) content were expressed as μmol g−1 FW. The activities of dehydroascorbate reductase (DHAR), monodehydroascorbate reductase (MDHAR), glutathione reductase (GR), and ascorbate peroxidase (APX) were expressed as the same as CAT.
2.6 Extraction of Total RNA and qRT-PCR
The total RNA was derived from strawberry tissue drawn on the technique of Meisel et al. [22]. The purity and content of RNA were determined by a Q5000 microspectrophotometer (Quawell, USA). Total RNA was reversely transcripted with cDNA PrimeScript™ II1st Strand cDNA Synthesis Kit (Takara, Dalian, China). qRT-PCR was carried out with the method of He et al. [23] with ACTIN as a housekeeping gene. The primers for qRT-PCR are listed in Table 1. The gene expression scale of strawberries untreated at day 0 was set as 1. The formula of PCR exponential amplification was Rn = R0 × (1 + Er)n. In this formula, Er was amplification efficiency, n was cycle number, Rn represented the number of PCR products after n cycles, and R0 was the number of original templates. The expression level of genes was calculated using formula 2(−ΔΔt). The internal reference gene of strawberries was FaActin.
Data was analyzed with a one-way analysis of square deviation (ANOVA), and the mean separations were used the minimum significant difference (LSD) test at a significance level of p < 0.05, highly significant level of p < 0.01, and not significant level of p ≥ 0.05. The presented data represented the mean ± standard deviation. Each test was repeated three times (n = 3).
3.1 Effect of EA on Browning Degree, Rotting Rate, and Soluble Solid Content of Strawberries
The browning degree of the EA-treated and controlled strawberries increased from beginning to end period (Fig. 1A). In contrast to the control, the treatments with EA observably retarded the increase in the browning degree of strawberries (p < 0.01). On the first day, the browning degree of strawberries treated with different EA concentrations was not significant (p ≥ 0.05), but the EA treatment significantly restrained the browning of strawberries compared to the control (p < 0.01). Significantly, the browning degree of strawberries treated with 300 mg L−1 EA remained inferior to the control and the treatments with other concentrations of EA at 2–4 days (p < 0.05). On day 4, the browning degree of strawberries with 300 mg L−1 EA was 0.542 OD410, only 65% of the control’s (p < 0.01).
Figure 1: Changes in (A) browning degree, (B) soluble solid content (SSC), (C) rotting rate of strawberries after different treatments with ellagic acid (EA) during storage. Data are average ± standard error values. Different lowercase letters indicated significant differences between treatments for each acquisition time (p < 0.05). Each sample was measured three times (n = 3)
The soluble solid content (SSC) of strawberries diminished while storing, and EA could slow this decrease rate (Fig. 1B). The SSC of strawberries treated with 100, 200, 400, and 500 mg L−1 EA was 6.77, 6.93, 6.55, 6.36°Brix on day 4. Throughout the storage period, EA treatment significantly maintained higher SSC compared to control (p < 0.01). Compared with EA treatment of other concentrations and control, 300 mg L−1 EA treatment consistently maintained the highest SSC (p < 0.05) and better maintained the quality of strawberry fruit. On the fourth day, the SSC of strawberries with 300 mg L−1 EA was 7.45°Brix, which was 1.30 times as tall as that of the control (p < 0.01).
The rotting rate of control and EA-treated strawberries increased throughout the storage period, with the most significant rotting rate of control strawberries reaching 78% on the fourth day (Fig. 1C). The rotting rate of strawberries treated with 100, 200, 400, and 500 mg L−1 EA was 74%, 64%, 60%, and 64%, respectively, on day 4. Relative to the other treatments, treatment with 300 mg L−1 EA declined the rotting rate of strawberries in the entire period. On day 4, the rotting rate of strawberries handled with 300 mg L−1 EA was 41%, less than that of the contrast and other treatments (p < 0.01).
3.2 Effect of EA on the Content of Malondialdehyde and Reactive Oxygen Species of Strawberries
The malondialdehyde (MDA) content of strawberries increased in the first two days (Fig. 2A). The MDA content at 3–4 days had a slower upward trend compared with 1–2 days. During storage, the EA treatment could suppress the growth rate of MDA content in strawberries. The MDA content of strawberries with 300 mg L−1 EA on day 3 and day 4 was 0.883 and 0.901 mmol g−1 FW, significantly lower than the control (p < 0.01).
Figure 2: Changes in the content of (A) malondialdehyde (MDA) and (B) reactive oxygen species (ROS) of strawberries after different treatments with ellagic acid (EA) during storage. Data are average ± standard error values. Different lowercase letters indicated significant differences between treatments for each acquisition time (p < 0.05). Each sample was measured three times (n = 3)
The reactive oxygen species (ROS) content gradually increased during storage. The ROS content increased sharply on days 0–1 and tended to be stable on days 1–4 (Fig. 2B). Compared with the control, treatments with EA could inhibit ROS formation (p < 0.05) during storage, and 300 mg L−1 EA had the best effect. The data showed a significant disparity between the strawberries treated with 300 mg L−1 EA and the control (p < 0.01).
3.3 Effect of EA on the Activities and Relative Gene Expression of the Antioxidant Enzyme of Strawberries
Polyphenol oxidase (PPO) activity of all samples boosted to the peak value on day 2 (Fig. 3A). The PPO activity of strawberries with EA was diminished than those of the contrast (p < 0.05). On the second day, the PPO activity of strawberries treated with 300 mg L−1 EA was 5.21 U g−1 FW, which was 82% of that of the control (p < 0.01). It could be seen (Fig. 3B) that the peroxidase (POD) activity of all samples rose on the first two days and then fell. The POD activities of strawberries with EA were consistently elevated compared to the control’s. On the fourth day, the POD activity of strawberries with 300 mg L−1 EA was 5.89 U g−1 FW and 1.87 times as high as the control (p < 0.01). Therefore, EA treatment could enhance the POD activity of postharvest strawberries. The superoxide dismutase (SOD) activity of strawberries decreased from beginning to end period (Fig. 3C). EA alleviated the reduction in the SOD activities of strawberries. In contrast to the control and other treatments, treatment with 300 mg L−1 EA maintained higher activity of SOD the entire period (p < 0.05). On day 4, the SOD activity of strawberries with 300 mg L−1 EA was 4.74 U g−1 FW, which was 1.25 times as many as that of the contrast (p < 0.01). Catalase (CAT) activity of strawberries handled with EA was taller than those of the contrast (p < 0.05) while storing (Fig. 3D). On the fourth day, the CAT activity of strawberries handled with 300 mg L−1 EA was 24.3 U g−1 FW, which was 1.46 times as high as that of the contrast (p < 0.01).
Figure 3: Changes in the activities of (A) polyphenol oxidase (PPO), (B) peroxidase (POD), (C) superoxide dismutase (SOD), (D) catalase (CAT), and the relative gene expression of (E) polyphenol oxidase (PPO), (F) peroxidase (POD), (G) manganese superoxide dismutase (MnSOD), (H) copper/zinc superoxide dismutase (Cu/ZnSOD), (I) iron superoxide dismutase (FeSOD) and (J) catalase (CAT) of strawberries after different treatments with ellagic acid (EA) during storage. Data are average ± standard error values. Different lowercase letters indicated significant differences between treatments for each acquisition time (p < 0.05). Each sample was measured three times (n = 3)
EA down-regulated PPO expression level (p < 0.01), and the expression degree of POD in strawberries treated with EA was upward than that of the comparison (p < 0.05) on days 1–4 during storage (Figs. 3E, 3F). EA only up-regulated the gene expression of manganese superoxide dismutase (MnSOD) on days 2 and 3 (p < 0.01), copper/zinc superoxide dismutase (Cu/ZnSOD) on day 4, and iron superoxide dismutase (FeSOD) on day 2 (p < 0.01), and down-regulated the gene expression of Cu/ZnSOD on days 1, 2 and 3 (p < 0.01), FeSOD on days 1, 3 and 4 (p < 0.01) (Figs. 3G–3I). The gene repression level of CAT in fruit treated with EA was higher from days 2–4 (p < 0.01) but lower on day 1 (p < 0.05) than that of the control (Fig. 3J).
3.4 Effect of EA on the Content of Ascorbic Acid and Glutathione of Strawberries
The ascorbic acid (AsA) content of all samples decreased during storage (Fig. 4A). EA delayed the rate of decrease in the AsA content of strawberries during storage. On day 3 and day 4, the AsA content of strawberries with 300 mg L−1 EA was 12.03 and 11.80 μmol g−1 FW, which was 1.21 (p < 0.01) and 1.24 (p < 0.01) times, severally, as high as that of the control. Similar to the changes of AsA, the decrease in the glutathione (GSH) content (Fig. 4B) of strawberries was relieved by the treatment with 300 mg L−1 EA during storage. On day 2 and day 4, the GSH content of strawberries with 300 mg L−1 EA was 0.0384 and 0.0146 μmol g−1 FW, which was 3.79 (p < 0.01) and 5.35 (p < 0.01) times, as high as that of the control.
Figure 4: Changes in the content of (A) ascorbic acid (AsA) and (B) glutathione (GSH) of strawberries after different treatments with ellagic acid (EA) during storage. Data are average ± standard error values. Different lowercase letters indicated significant differences between treatments for each acquisition time (p < 0.05). Each sample was measured three times (n = 3)
3.5 Effect of EA on the Activities and Relative Gene Expression of Enzymes in the Ascorbate–Glutathione Cycle of Strawberries
Dehydroascorbate reductase (DHAR) activity of the control strawberries decreased before day 2 and then started to pick up on the third and fourth day (Fig. 5A). The second day of the strawberry’s storage process, the DHAR activity of strawberries with 300 mg L−1 EA was 19.8 U g−1 FW and was 1.65 times that of the control (p < 0.01). On day 4, the DHAR activity of strawberries with 300 mg L−1 EA was 1.87 times as high as those of the control (p < 0.01). Compared with other concentrations of EA, EA at 300 mg L−1 more effectively improved the DHAR activity of strawberries during storage (p < 0.05). The gene expression level of DHAR in strawberries with EA was lower on day 1 (p < 0.01) and higher after day 4 (p < 0.01) than that of the control (Fig. 5E).
Figure 5: Changes in the activities of (A) dehydroascorbate reductase (DHAR), (B) monodehydroascorbate reductase (MDHAR), (C) glutathione reductase (GR), (D) ascorbate peroxidase (APX), and the relative gene expression of (E) dehydroascorbate reductase (DHAR), (F) monodehydroascorbate reductase (MDHAR), (G) glutathione reductase (GR) and (H) ascorbate peroxidase (APX) of strawberries after different treatments with ellagic acid (EA) during storage. Data are average ± standard error values. Different lowercase letters indicated significant differences between treatments for each acquisition time (p < 0.05). Each sample was measured three times (n = 3)
Monodehydroascorbate reductase (MDHAR) activity gradually rose to numerical vertex on day 3 and then declined (Fig. 5B). The MDHAR activity of strawberries with EA was superior to that of the control (P < 0.05). On the third day, the MDHAR activity of strawberries conducted with 300 mg L−1 EA was 77.14 U g−1 FW, which was 1.1 times as high as the control’s (p < 0.01). In different treatment concentrations, EA at 300 mg L−1 preserved the highest MDHAR activity of strawberries throughout the storage process. Exogenous EA up-regulated the gene expression of MDHAR on days 2–4 than those of the control (p < 0.05) (Fig. 5F).
Glutathione reductase (GR) activity of strawberries boosted to reach the numerical peak on day 2 and then declined (Fig. 5C). EA could improve the activity of GR from beginning to end period. The GR activity of strawberries with 300 mg L−1 was higher in contrast to the control and the treatments with other concentrations of EA during storage (p < 0.01). The manifestation of GR in strawberries processed with EA was lower on days 1–3 (p < 0.05) and taller on day 4 (p < 0.01) than that of the control (Fig. 5G).
Ascorbate peroxidase (APX) activity of strawberries rose to the zenith on day 2 and then diminished during storage (Fig. 5D). Treatments with EA significantly increased APX activity on days 1–3 (P < 0.01). Compared with other treatments, EA at 300 mg L−1 maintained the highest APX activity of strawberries while storing. On day 4, the APX activity of strawberries with 300 mg L−1 was 1.76 times (p < 0.01), 1.23 times (p < 0.01), and 1.71 times (p < 0.01) as high as that of the control, treatment with 200 mg L−1 EA, and treatment with 400 mg L−1 EA, respectively (Fig. 5D). The gene expression of APX in strawberries treated with EA was up-regulated from days 2–4 (Fig. 5H) (p < 0.01).
Postharvest strawberries have a high metabolic rate and vigorous respiration. Therefore, the storability of strawberries is poor, mainly reflected in the loss of water, browning, and decaying. Browning and decay can destroy the appearance and quality of strawberries, affect their flavor, reduce their commercial and nutritional value, and then affect the desire of buyers [24]. Some relevant studies show ellagic acid (EA) could reduce the decay rate of cranberry pomace during storage by inhibiting the growth and reproduction of microorganisms [25]. This study also showed that EA treatment could efficiently curb the browning and rotting rates of strawberries and thus maintain the surface color of strawberries, among which 300 mg L−1 EA treatment had the best effect. Soluble solid content (SSC) is some intracellular protective substance, mainly composed of nutrients such as soluble sugar and organic acids. Fruit flavor is closely related to SSC. Increasing the content of SSC in strawberry fruit can significantly improve the standard of strawberries. This experimental study showed that SSC expressed a downward trend during storage, but in comparison to the control group, strawberries treated with EA could effectively assuage this process, and treatment with 300 mg L−1 EA had the second to none effect on restraining the decline of SSC of strawberries.
Plants produce plenty of reactive oxygen species (ROS) under adverse environments, causing oxidative damage to plant cells and affecting the normal physiological activities of cells. Reducing the ROS production rate can effectively extend postharvest fruits’ shelf life [26]. ROS, as a product of plants under oxidative stress, can devastate cell membranes, causing membrane lipid peroxidation and accelerating cell senescence. The antioxidant system in plants regulates the immune system and maintains the normal function of cells [27]. According to the results of this experiment, as shown in Fig. 2B, by contrast with the control, EA treatment can lessen the generation of ROS and ameliorate the preservation effect of strawberry fruit, in which both 100 and 300 mg L−1 EA have better effects than others. Malondialdehyde (MDA) is a crucial index for evaluating lipid peroxidation and the structural integrity of membranes, as well as the end product of lipid peroxidation, and its content can directly mirror the injury to cell membranes [28]. As shown in Fig. 2A, MDA content showed an increasing trend while storing, but the accumulation of MDA could be refrained by EA treatment.
In the enzymatic defense system, peroxidase (POD) is an important ROS scavenging agent in plants, can regulate oxygen concentration by scavenging hydrogen peroxide in tissues, and maintains the equilibrium of reactive oxygen species metabolism in cells [29]. The activity and gene expression level of POD of strawberries handled with EA was notably higher than that of the control, indicating that EA could improve POD activity to reduce the ROS content in plant tissues. Analogical consequences are also discovered in strawberries treated with salicylic acid and methyl jasmonate [30]. During storage, EA significantly increased the activities and gene expression levels of superoxide dismutase (SOD) and catalase (CAT), accelerating the transition of superoxide anion to hydrogen peroxide and then further into water [31], thereby improving the antioxidant capacity of strawberries. Similar influences of EA on the activities of antioxidative enzymes are also obtained in kumquat fruits during storage [17]. It seems that the gene expression of manganese superoxide dismutase (MnSOD), copper/zinc superoxide dismutase (Cu/ZnSOD), and iron superoxide dismutase (FeSOD) contributed to the SOD activity on day 2–3, day 4, and day 2, respectively. The time differences in changes in the gene expression of MnSOD, Cu/ZnSOD, and FeSOD in strawberries treated with EA suggested that the three isoenzymes of SOD contributed to the enzyme activity in different periods.
In the non-enzymatic antioxidant defense system, ascorbic acid (AsA)and glutathione (GSH) are momentous reducing substances in plant cells and synergistically scavenge ROS, eliminate peroxide chain reactions, and alleviate the damage of free radicals to plant cells, indicating that they are effective antioxidants in plants [32]. As shown in Fig. 4, treatment with 300 mg L−1 EA could significantly increase the assay of AsA and GSH in postharvest strawberries, maintain the relatively steady state of AsA and GSH in cells, and enhance the eliminate ability of plant cells to ROS. Similar results are obtained in strawberries treated with allyl isothiocyanate [33].
Monodehydroascorbate reductase (MDHAR), dehydroascorbate reductase (DHAR), glutathione reductase (GR), and ascorbate peroxidase (APX), which work together to complete the regeneration of AsA and GSH and to remove free radicals, are vital enzymes in the AsA-GSH cycle [34]. AsA is altered to reduce oxidized ascorbate (DHA) and then renovates H2O2 to H2O by means of the oxidation of APX, and this reaction is accommodated by DHAR and MDHAR. APX is an essential antioxidant enzyme that attenuates cellular lipid peroxidation, which can eliminate excess H2O2 in plants and augment the rate of ROS metabolism. The decrease in APX enzyme activity can be used as a basis for plant senescence [35]. DHAR and MDHAR promote the regeneration of AsA by DHA and monodehydroascorbic acid (MDHA). MDHAR and DHAR are the key enzymes to maintain the content of AsA [36]. As seen in Fig. 5, EA enhanced the APX activity of strawberries during storage and delayed the senescence of the fruits. It is similar to the results of strawberries treated with chitosan [37]. Treatment with 300 mg L−1 EA positively increased the activity of MDHAR and DHAR and maintained the AsA content in strawberries, which helped improve the resistance to oxidative damage. Meanwhile, the activity of APX in strawberries was always more advanced than that of DHAR and MDHAR, indicating that APX had a more vital ability to oxidize AsA than MDHAR and DHAR, similar to the results of Jin et al. [38]. GR also plays a vital role in the AsA-GSH defense system, regulating redox balance with other antioxidants in plants and maintaining the regular physiological activity of fruits [39]. Treatment with EA increased the activity of GR during storage, which improved the ability of GR to scavenge ROS under oxidative stress, indicating that EA could add to the antioxidant activity of strawberries and prolong their guarantee period. Semblable outcomes are also discovered in strawberries disposed with salicylic acid [40]. The gene expression levels of DHAR and GR were inconsistent with the variations in enzyme activities in strawberries with EA treatment. It has been reported that preservatives can form complexes with antioxidant enzymes to alter their liveness by affecting the constitution of these enzymes [14]. We speculated that EA might similarly perform combined with DHAR and GR to directly regulate the enzyme activities instead of influencing the transcription levels of their genes, but the formation and the formation mechanism of the compound are meriting further research.
The postharvest strawberries soaked in EA solution of 300 mg L−1 exhibited more effective regulation of fruit quality and antioxidant system than the treatments with EA of 100, 200, 400, and 500 mg L−1. EA at 300 mg L−1 could significantly reduce strawberries’ browning degree, rotting rate, MDA, and ROS content compared to the control. Additionally, treatment with EA can maintain high activities of POD, SOD, CAT, APX, GR, DHAR, and MDHAR, maintain high content of ASA and GSH, downgrade the content of MDA and ROS, and up-regulate the gene expression level of POD, SOD, CAT, MDHAR, and APX. Based on the data obtained in this study, it was suggested that exogenous EA can excellently trim down oxidative stress by adjusting the enzymatic and non-enzymatic antioxidant defense systems in strawberries. In conclusion, EA treatment is an efficient technology to preserve postharvest character by improving the antioxidant system of fruits.
Acknowledgement: We sincerely acknowledge Wenxin Yuan and Junwen Wang for their help in the experiments.
Funding Statement: This research was funded by the National Natural Science Foundation of China, Grant Number 31800581.
Author Contributions: Conceptualization, D. Huang and S. Zhu; methodology, investigation, data curation, writing—original draft preparation, J. Chen; resources, visualization, J. Zhang; investigation, data curation, G. Pan; writing—review and editing, S. Zhu; supervision, project administration, funding acquisition, D. Huang. All authors have read and agreed to the published version of the manuscript.
Availability of Data and Materials: The raw data supporting the findings of this study are available from the authors upon reasonable request.
Ethics Approval: Not applicable.
Conflicts of Interest: The authors declare that they have no conflicts of interest to report regarding the present study.
References
1. Hannum, S. M. (2004). Potential impact of strawberries on human health: A review of the science. Critical Reviews in Food Science and Nutrition, 44, 1–17. https://doi.org/10.1080/10408690490263756 [Google Scholar] [PubMed] [CrossRef]
2. Huang, M., Han, Y., Li, L., Rakariyatham, K., Wu, X. et al. (2022). Protective effects of non-extractable phenolics from strawberry against inflammation and colon cancer in vitro. Food Chemistry, 374, 131759. https://doi.org/10.1016/j.foodchem.2021.131759 [Google Scholar] [PubMed] [CrossRef]
3. Huang, D., Wang, Y., Zhang, D., Dong, Y., Meng, Q. et al. (2021). Strigolactone maintains strawberry quality by regulating phenylpropanoid, NO, and H2S metabolism during storage. Postharvest Biology and Technology, 178, 111546. https://doi.org/10.1016/j.postharvbio.2021.111546 [Google Scholar] [CrossRef]
4. Quarshi, H. Q., Ahmed, W., Azmant, R., Chendouh-Brahmi, N., Quyyum, A. et al. (2023). Postharvest problems of strawberry and their solutions. In: Kafkas, N. E. (Ed.Recent studies on strawberries. Rijeka: IntechOpen. https://doi.org/10.5772/intechopen.102963 [Google Scholar] [CrossRef]
5. Sogvar, O. B., Koushesh Saba, M., Emamifar, A. (2016). Aloe vera and ascorbic acid coatings maintain postharvest quality and reduce microbial load of strawberry fruit. Postharvest Biology and Technology, 114, 29–35. https://doi.org/10.1016/j.postharvbio.2015.11.019 [Google Scholar] [CrossRef]
6. Bose, S. K., Howlader, P., Jia, X., Wang, W., Yin, H. (2019). Alginate oligosaccharide postharvest treatment preserve fruit quality and increase storage life via abscisic acid signaling in strawberry. Food Chemistry, 283, 665–674. https://doi.org/10.1016/j.foodchem.2019.01.060 [Google Scholar] [PubMed] [CrossRef]
7. van de Velde, F., Méndez-Galarraga, M. P., Grace, M. H., Fenoglio, C., Lila, M. A. (2019). Changes due to high oxygen and high carbon dioxide atmospheres on the general quality and the polyphenolic profile of strawberries. Postharvest Biology and Technology, 148, 49–57. https://doi.org/10.1016/j.postharvbio.2018.10.015 [Google Scholar] [CrossRef]
8. Duran, M., Aday, M. S., Zorba, N. N. D., Temizkan, R., Büyükcan, M. B. et al. (2016). Potential of antimicrobial active packaging ‘containing natamycin, nisin, pomegranate and grape seed extract in chitosan coating’ to extend shelf life of fresh strawberry. Food and Bioproducts Processing, 98, 354–363. https://doi.org/10.1016/j.fbp.2016.01.007 [Google Scholar] [CrossRef]
9. Ron, M. (2002). Oxidative stress, antioxidants and stress tolerance. Trends in Plant Science, 7, 405–410. https://doi.org/10.1016/S1360-1385(02)02312-9 [Google Scholar] [PubMed] [CrossRef]
10. You, J., Chen, Z. L. (2015). ROS regulation during abiotic stress responses in crop plants. Frontiers in Plant Science, 6, 1092. https://doi.org/10.3389/fpls.2015.01092 [Google Scholar] [PubMed] [CrossRef]
11. Li, Y. L., Liu, Y. F., Zhang, J. G. (2010). Advances in the research on the AsA-GSH cycle in horticultural crops. Frontiers of Agriculture in China, 4, 84–90. https://doi.org/10.1007/s11703-009-0089-8 [Google Scholar] [CrossRef]
12. Wang, F., Zhang, X. P., Yang, Q. Z., Zhao, Q. F. (2019). Exogenous melatonin delays postharvest fruit senescence and maintains the quality of sweet cherries. Food Chemistry, 301, 125311. https://doi.org/10.1016/j.foodchem.2019.125311 [Google Scholar] [PubMed] [CrossRef]
13. Ma, Y., Huang, D., Chen, C., Zhu, S., Gao, J. (2019). Regulation of ascorbate-glutathione cycle in peaches via nitric oxide treatment during cold storage. Scientia Horticulturae, 247, 400–406. https://doi.org/10.1016/j.scienta.2018.12.039 [Google Scholar] [CrossRef]
14. Ma, Y., Fu, L., Hussain, Z., Huang, D., Zhu, S. (2019). Enhancement of storability and antioxidant systems of sweet cherry fruit by nitric oxide-releasing chitosan nanoparticles (GSNO-CS NPs). Food Chemistry, 285, 10–21. https://doi.org/10.1016/j.foodchem.2019.01.156 [Google Scholar] [PubMed] [CrossRef]
15. Sharifi-Rad, J., Quispe, C., Castillo, C. M. S., Caroca, R., Lazo-Vélez, M. A. et al. (2022). Ellagic acid: A review on its natural sources, chemical stability, and therapeutic potential. Oxidative Medicine and Cellular Longevity, 2022, 3848084. https://doi.org/10.1155/2022/3848084 [Google Scholar] [PubMed] [CrossRef]
16. Rossetti, A., Mazzaglia, A., Muganu, M., Paolocci, M., Sguizzato, M. et al. (2017). Microparticles containing gallic and ellagic acids for the biological control of bacterial diseases of kiwifruit plants. Journal of Plant Diseases and Protection, 124, 563–575. https://doi.org/10.1007/s41348-017-0096-6 [Google Scholar] [CrossRef]
17. Liu, Y., Liu, Y., Liu, Y., Liu, H., Shang, Y. (2018). Evaluating effects of ellagic acid on the quality of kumquat fruits during storage. Scientia Horticulturae, 227, 244–254. https://doi.org/10.1016/j.scienta.2017.08.055 [Google Scholar] [CrossRef]
18. Lei, J., Li, B., Zhang, N., Yan, R., Guan, W. et al. (2018). Effects of UV-C treatment on browning and the expression of polyphenol oxidase (PPO) genes in different tissues of Agaricus bisporus during cold storage. Postharvest Biology and Technology, 139, 99–105. https://doi.org/10.1016/j.postharvbio.2017.11.022 [Google Scholar] [CrossRef]
19. Jambunathan, N. (2010). Determination and detection of reactive oxygen species (ROSlipid peroxidation, and electrolyte leakage in plants. Methods in Molecular Biology, 639, 291–297. https://doi.org/10.1007/978-1-60761-702-0_18 [Google Scholar] [PubMed] [CrossRef]
20. Gong, B., Miao, L., Kong, W., Bai, J. G., Wang, X. et al. (2014). Nitric oxide, as a downstream signal, plays vital role in auxin induced cucumber tolerance to sodic alkaline stress. Plant Physiology and Biochemistry, 83, 258–266. https://doi.org/10.1016/j.plaphy.2014.08.004 [Google Scholar] [PubMed] [CrossRef]
21. Wei, G. Q., Zhang, W. W., Cao, H., Yue, S. S., Li, P. et al. (2019). Effects hydrogen sulfide on the antioxidant system and membrane stability in mitochondria of Malus hupehensis under NaCl stress. Biologia Plantarum, 63, 228–236. https://doi.org/10.32615/bp.2019.026 [Google Scholar] [CrossRef]
22. Meisel, L., Fonseca, B., González, S., Baeza-Yates, R., Cambiazo, V. et al. (2005). A rapid and efficient method for purifying high quality total RNA from peaches (Prunus persica) for functional genomics analyses. Biological Research, 38, 83–88. https://doi.org/10.4067/s0716-97602005000100010 [Google Scholar] [PubMed] [CrossRef]
23. He, D., Xia, B., Zhou, Q., Wang, L., Huang, X. (2019). Rare earth elements regulate the endocytosis and DNA methylation in root cells of Arabidopsis thaliana. Chemosphere, 227, 522–532. https://doi.org/10.1016/j.chemosphere.2019.04.076 [Google Scholar] [PubMed] [CrossRef]
24. Contigiani, E. V., Jaramillo-Sánchez, G., Castro, M. A., Gómez, P. L., Alzamora, S. M. (2018). Postharvest quality of strawberry fruit (Fragaria x Ananassa Duch cv. Albion) as affected by ozone washing: Fungal spoilage, mechanical properties, and structure. Food and Bioprocess Technology, 11, 1639–1650. https://doi.org/10.1007/s11947-018-2127-0 [Google Scholar] [CrossRef]
25. Vattem, D. A., Shetty, K. (2003). Ellagic acid production and phenolic antioxidant activity in cranberry pomace (Vaccinium macrocarpon) mediated by Lentinus edodes using a solid-state system. Process Biochemistry, 39, 367–379. https://doi.org/10.1016/S0032-9592(03)00089-X [Google Scholar] [CrossRef]
26. Peláez-Vico, M. Á., Fichman, Y., Zandalinas, S. I., van Breusegem, F., Karpiński, S. M. et al. (2022). ROS and redox regulation of cell-to-cell and systemic signaling in plants during stress. Free Radical Biology and Medicine, 193, 354–362. https://doi.org/10.1016/j.freeradbiomed.2022.10.305 [Google Scholar] [PubMed] [CrossRef]
27. Willian, B. S., Gláucia, M. C. S., Diederson, B. S., Acácio, R. S., David, N. M. et al. (2018). Chitosan delays ripening and ROS production in guava (Psidium guajava L.) fruit. Food Chemistry, 242, 232–238. https://doi.org/10.1016/j.foodchem.2017.09.052 [Google Scholar] [PubMed] [CrossRef]
28. Giuseppina, A., Marisa, D. M., Milena, P. (2021). Multifunctional role of chitosan edible coatings on antioxidant systems in fruit crops: A review. International Journal of Molecular Sciences, 22, 2633. https://doi.org/10.3390/IJMS22052633 [Google Scholar] [PubMed] [CrossRef]
29. Fan, X. J., Zhang, B., Yan, H., Feng, J. T., Ma, Z. Q. et al. (2019). Effect of lotus leaf extract incorporated composite coating on the postharvest quality of fresh goji (Lycium barbarum L.) fruit. Postharvest Biology and Technology, 148, 132–140. https://doi.org/10.1016/j.postharvbio.2018.10.020 [Google Scholar] [CrossRef]
30. Asghari, M., Hasanlooe, A. R. (2015). Interaction effects of salicylic acid and methyl jasmonate on total antioxidant content, catalase and peroxidase enzymes activity in “Sabrosa” strawberry fruit during storage. Scientia Horticulturae, 197, 490–495. https://doi.org/10.1016/j.scienta.2015.10.009 [Google Scholar] [CrossRef]
31. Morteza, S. A., Roohangiz, N., Abbasali, J., Mohammad, A. A. S., Mesbah, B. (2016). Enhancement of postharvest chilling tolerance of anthurium cut flowers by γ-aminobutyric acid (GABA) treatments. Scientia Horticulturae, 198, 52–60. https://doi.org/10.1016/j.scienta.2015.11.019 [Google Scholar] [CrossRef]
32. Dong, Y., Liu, L., Zhao, Z., Zhi, H., Guan, J. (2015). Effects of 1-MCP on reactive oxygen species, polyphenol oxidase activity, and cellular ultra-structure of core tissue in ‘Yali’ pear (Pyrus bretschneideri Rehd.) during storage. Horticulture, Environment, and Biotechnology, 56, 207–215. https://doi.org/10.1007/s13580-015-0094-1 [Google Scholar] [CrossRef]
33. Ugolini, L., Pagnotta, E., Matteo, R., Malaguti, L., Francesco, A. D. et al. (2019). Brassica meal-derived allyl-isothiocyanate postharvest application: Influence on strawberry nutraceutical and biochemical parameters. Science of Food Agriculture, 99, 4235–4241. https://doi.org/10.1002/jsfa.9654 [Google Scholar] [PubMed] [CrossRef]
34. Wang, Y., Ye, X. Y., Qiu, X. M., Li, Z. G. (2019). Methylglyoxal triggers the heat tolerance in maize seedlings by driving AsA-GSH cycle and reactive oxygen species-/methylglyoxal-scavenging system. Plant Physiology and Biochemistry, 138, 91–99. https://doi.org/10.1016/j.plaphy.2019.02.027 [Google Scholar] [PubMed] [CrossRef]
35. Soheila, S., Maryam, N. E., Roya, K., Lam-Son, P. C. (2018). Physiological and biochemical modifications by postharvest treatment with sodium nitroprusside extend vase life of cut flowers of two gerbera cultivars. Postharvest Biology and Technology, 137, 1–8. https://doi.org/10.1016/j.postharvbio.2017.11.009 [Google Scholar] [CrossRef]
36. Cruz-Rus, E., Amaya, I., Sánchez-Sevilla, J. F., Botella, M. A., Valpuesta, V. (2011). Regulation of L-ascorbic acid content in strawberry fruits. Journal of Experimental Botany, 62, 4191–4201. https://doi.org/10.1093/jxb/err122 [Google Scholar] [PubMed] [CrossRef]
37. Petriccione, M., Mastrobuoni, F., Pasquariello, M. S., Zampella, L., Nobis, E. et al. (2015). Effect of chitosan coating on the postharvest quality and antioxidant enzyme system response of strawberry fruit during cold storage. Foods, 4, 501–523. https://doi.org/10.3390/foods4040501 [Google Scholar] [PubMed] [CrossRef]
38. Jin, Y. H., Tao, D. L., Hao, Z. Q., Ye, J., Du, Y. J. (2003). Environmental stresses and redox status of ascorbate. Journal of Integrative Plant Biology, 45, 795–801. https://www.jipb.net/EN/Y2003/V45/I7/795 [Google Scholar]
39. Ge, Y., Deng, H., Bi, Y., Li, C., Liu, Y. (2015). Postharvest ASM dipping and DPI pre-treatment regulated reactive oxygen species metabolism in muskmelon (Cucumis melo L.) fruit. Postharvest Biology and Technology, 99, 160–167. https://doi.org/10.1016/j.postharvbio.2014.09.001 [Google Scholar] [CrossRef]
40. Jamali, B., Eshghi, S., Kholdebarin, B. (2016). Antioxidant responses of ‘Selva’ strawberry as affected by salicylic acid under salt stress. Journal of Berry Research, 6, 291–301. https://doi.org/10.3233/jbr-160130 [Google Scholar] [CrossRef]
Cite This Article
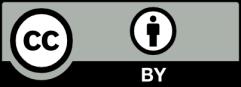