Open Access
ARTICLE
A New Micropropagation Technology of Tilia amurensis: In Vitro Micropropagation of Mature Zygotic Embryos and the Establishment of a Plant Regeneration System
1 Jilin Provincial Academy of Forestry Sciences (Jilin Forestry Biological Control Central Station), Changchun, 130033, China
2 Institute of Forestry Inventory and Planning of Jilin Province, Changchun, 130022, China
3 Jilin Forest Tree and Seeding Management Station, Changchun, 130022, China
* Corresponding Author: Zhonghui Zhang. Email:
Phyton-International Journal of Experimental Botany 2024, 93(2), 277-289. https://doi.org/10.32604/phyton.2024.046989
Received 21 October 2023; Accepted 29 December 2023; Issue published 27 February 2024
Abstract
Tilia amurensis is an economically valuable broadleaf tree species in Northeast China. The production of high-quality T. amurensis varieties at commercial scales has been greatly limited by the low germination rates. There is thus a pressing need to develop an organogenesis protocol for in vitro propagation of T. amurensis to alleviate a shortage of high-quality T. amurensis seedlings. Here, we established a rapid in vitro propagation system for T. amurensis from mature zygotic embryos and analyzed the effects of plant growth regulators and culture media in different stages. We found that Woody plant medium (WPM) was the optimal primary culture medium for mature zygotic embryos. The highest callus induction percentage (68.76%) and number of axillary buds induced (3.2) were obtained in WPM + 0.89 µmol/L 6-benzyladenine (6-BA) + 0.46 µmol/L kinetin (KT) + 0.25 µmol/L indole-3-butryic acid (IBA) + 1.44 µmol/L gibberellin A3 (GA3). The multiple shoot bud development achieved the highest percentage (83.32%) in the Murashige and Skoog (MS) + 2.22 µmol/L 6-BA + 0.25 µmol/L IBA + 1.44 µmol/L GA3. The rooting percentage (96.70%) was highest in 1/2 MS medium + 1.48 µmol/L IBA. The survival percentage of transplanting plantlets was 82.22% in soil:vermiculite:perlite (5:3:1). Our study is the first to establish an effective organogenesis protocol for T. amurensis using mature zygotic embryos.Keywords
The rapid in vitro propagation of plants can be used to produce plants on commercial scales; the ability for maternal genetic characteristics to be maintained and plantlets to be produced at any site and in any season are some of the main advantages of rapid in vitro propagation systems [1–3]. Rapid in vitro propagation systems are critically important for the preservation of high-quality linden tree germplasm; generally, these systems are considered useful tools for enhancing the quality of the germplasm of forest trees and alleviating shortages of high-quality germplasm.
Tilia amurensis Rupr. is one of the most economically valuable broadleaf tree species in Northeast China, as well as an important wild nectar source [4,5]; it is classified as a second-grade national protected tree species in China. T. amurensis is also used for ornamental purposes in cities and gardens for its aesthetic features, including its shape and pleasing fragrance. Wild germplasm resources of T. amurensis are in decline because its seeds show deep dormancy, which affects the maintenance of its natural populations [4,6]. This, coupled with its low reproductive efficiency and uneven seedling emergence, poses further challenges to the maintenance of germplasm resources [7]. Given the current shortage of T. amurensis seedlings, there is an urgent need to develop a rapid in vitro propagation system for T. amurensis to both address this shortage in the short term as well as preserve existing high-quality resources [6,8].
Linden calli have been induced in different tissues of Tilia americana L. by Barker as early as 1969 [9]; Chalupa [10] obtained differentiated buds from the axillary buds of Tilia cordata Mill. for the first time in 1987. Adventitious buds were obtained from axillary buds in young branches of 15-year-old T. cordata Mill. trees, and these were used to obtain regenerated plants in 1988 [11]. Rapid in vitro propagation has been used to obtain regenerated plants in several species to date, including T. cordata Mill., Tilia platyphyllos Scop., Tilia japonica Simonk., and Tilia miqueliana [11–15]. Few studies have attempted to establish rapid in vitro propagation systems for T. amurensis. Differentiated buds have been obtained from the axillary buds of 2-year-old T. amurensis plantlets, but regenerated plants could not be obtained [8]. In addition, Preece et al. [16] and Ren et al. [17] proposed that the formation of callus at the base of the explant is beneficial for the development of axillary shoots. By contrast, Steephen et al. [18] and Hristova et al. [19] concluded that the large formation of callus at the base of the explant inhibits the formation of axillary shoots. The effect of callus formation at the base of the explants on the development of the axillary shoots on T. amurensis has not yet been clarified. The establishment of a rapid in vitro propagation system is one of the major challenges for alleviating the current shortage of T. amurensis seedlings. The development of an effective tissue culture system for T. amurensis is essential for preserving high-quality germplasm and for producing plantlets on a large scale.
Compared to other types of explants, the zygotic embryos are the most desirable source of explants because they are characterized by abundant availability and convenience of transportation and they are notably faster and more responsive to in vitro culture [20,21]. Our study aimed to characterize the effects of different plant growth regulators, including indole-3-butryic acid (IBA), 6-benzyladenine (6-BA), kinetin (KT), and gibberellin A3 (GA3), and culture media on the formation of callus at the basal cut ends of shoots, axillary bud formation of shoots, multiple shoot bud induction, and rooting when zygotic embryos excised from mature seeds were used as explants. Next, we characterized the effects of a nutrient soil:vermiculite: perlite (5:3:1) mixture on the survival of plantlets. The rapid in vitro propagation system established in this study could be used for the large-scale propagation of high-quality T. amurensis varieties.
Mature T. amurensis seeds were collected in Jiaohe City, Jilin Province, China in October 2019. After selecting several mother trees, they were dried in a cool place sheltered from the wind for one week and then stored at 4°C after the seed coat was removed.
2.2 Effects of Five Basic Media on the Primary Culture of Zygotic Embryos
The peeled T. amurensis seeds were washed with running water for 2 d, treated with 75% (v/v) alcohol for 1 min in a laminar flow hood (HDL-APPARATUS, Beijing, China), and then washed three to five times with sterile water. The washed seeds were disinfected by soaking in 10% (m/v) calcium hypochlorite solution for 20 min followed by rinsing three to five times with sterile water.
The disinfected seeds were placed on sterile filter paper to absorb water, the endosperm was removed, and the zygotic embryo was inoculated on five basic media: Woody plant medium (WPM) [22], Murashige and Skoog (MS) medium [23], 1/2 MS medium, Schenk and Hildebrandt (SH) medium [24], and Driver and Kuniyuki (DKW) medium [25]. The zygotic embryo was placed into a 25 ml flask filled with 15 mL of medium, with one embryo per flask. There were a total of 30 embryos per treatment, and three replications were performed for each treatment. Measurements of the survival percentage, plantlet height, root length, number of lateral roots, leaf color, and main stem roughness of plantlets were taken after 28 d of culture.
2.3 Effects of Different Plant Growth Regulators on the Callus Induction and Axillary Bud Formation of Shoots
Following 28 d of primary culture, the shoots from aseptic T. amurensis plantlets were cut into pieces of 1.5 to 2.0 cm in length and grown vertically on medium as explants (Fig. 2A). First, WPM was used as the base medium, followed by 6-BA (0.89, 2.22, or 4.44 μmol/L) and IBA (0.05, 0.25, or 0.49 μmol/L) to identify the optimal preliminary medium for axillary bud induction using a completely randomized block design with nine treatments. Next, using the identified preliminary optimal medium (WPM + 0.89 μmol/L 6-BA + 0.25 μmol/L IBA) as the basic medium, KT (0.23, 0.46, or 0.93 μmol/L) and GA3 (0.58, 1.44, or 2.89 μmol/L)(6-BA, IBA, KT, GA3)(PhytoTechnology, Lenexa, Kansas, United States) were further added to culture the shoots from the beginning and further identify the optimal axillary bud induction medium. The explants were cultured in a 240 ml flask filled with 50 mL of medium; each treatment had 50 explants, and three replications were performed. After 50 d, measurements of the basal callus formation percentage, axillary bud induction percentage, number of adventitious buds, adventitious bud length, and fresh callus weight were taken.
2.4 Effects of Different Plant Growth Regulators on the Proliferation of Adventitious Buds with Calli
Adventitious buds with calli obtained from the above were used for proliferation culture. The basal culture media were WPM and MS supplemented with 6-BA (0.89, 2.22, or 4.44 μmol/L), IBA (0.25 μmol/L), and GA3 (0.29, 1.44, or 2.89 μmol/L). The explants were cultured in a 240 ml flask filled with 60 mL of medium; each treatment had 35 explants, with three replications. Measurements of the multiple shoot bud development percentage [26], number of adventitious shoots, and length of adventitious shoots were taken for 40 d.
2.5 Effects of Culture Media and Plant Growth Regulator Concentration on Shoot Rooting
Shoots approximately 3 to 4 cm in length from the proliferation culture were inoculated on WPM and 1/2 MS medium supplemented with IBA (0, 0.49, 1.48, 2.46, or 3.94 μmol/L). The shoots were cultured in a 240 ml flask filled with 50 mL of medium; each treatment had 30 shoots per treatment, with three replications. Measurements of the rooting percentage, number of roots, and root length were taken following 45 d of culture.
The materials obtained above were placed on a medium supplemented with 30 g/L sucrose and 6 g/L agar (Jiafeng Gardening Supplies Co., Ltd., Shanghai, China). Before autoclaving (105 Pa at 120°C, 20 min), the pH of the medium was adjusted to 5.8. The temperature of the culture room was 23°C ± 2°C; the light intensity was approximately 35 μmol·m−2·s−1; and all plant materials were subjected to a 16 h/8 h light/dark photoperiod.
2.7 Acclimation and Transplantation of Regenerated Plants
Following 45 d of rooting, tissue-cultured plantlets were acclimatized to the external environment. First, multiple small holes were made in a plastic film along the tissue culture flask, and the flask was subjected to a relative humidity of 65%–75% and temperature of 25°C–28°C for 3 d. The plastic film was removed, and the rooted plantlets were washed with tap water to remove the remaining medium and transplanted to a soil:vermiculite: perlite (5:3:1) mixture. The relative humidity of the microenvironment at the beginning of the transplantation experiment was maintained between 75% and 85%. There were a total of 30 plants transplanted, and three replicates were performed. Following 30 d of growth, the survival percentage was calculated.
One-way analysis of variance was conducted using SPSS 19.0 software (IBM, New York, NY), and the statistical significance of differences between groups was determined using Duncan’s multiple range test (p < 0.05).
3.1 Effects of Five Basic Media on the Primary Culture of Zygotic Embryos
The leaves of plantlets cultured on WPM were wide and dark green or green, and the main stem of these plantlets was strong (Fig. 1A). Plantlets that had been grown on the other four media had green or light-green leaves, relatively thin stems, and few lateral roots (Figs. 1B–1E). The planting percentage was significantly higher on WPM (70.36%) than on the other four media (p < 0.05) (Table 1). The height and root length of plantlets (5.31 and 10.37 cm, respectively) were significantly higher on WPM than on the other four media (p < 0.05). No significant differences in plantlet height were observed among plantlets grown on MS, 1/2 MS, DKW, and SH medium (p > 0.05). The number of lateral roots of plantlets was significantly higher when they were grown on WPM (average of 7.03) than on the other four media (p < 0.05). Thus, WPM was the optimal medium for the primary culture of T. amurensis zygotic embryos.
Figure 1: Growth status of aseptic T. amurensis plantlets in five media following 28 d. (A) WPM, bar = 2 cm; (B) MS, bar = 2 cm; (C) SH, bar = 2 cm; (D) ½ MS, bar = 2 cm; (E) DKW, bar = 2 cm
3.2 Effects of Different Plant Growth Regulators on the Basal Callus Induction and Axillary Bud Formation of Shoots
The formation of callus at the base of explants has a significant impact on the growth of axillary shoots. Comparing all nine treatments, the axillary bud induction percentage, the basal callus formation percentage, and basal callus fresh weight of the shoots of plants were significantly higher (24%, 66%, and 437.69 mg, respectively) when they were cultured on WPM supplemented with 0.89 μmol/L 6-BA and 0.25 μmol/L IBA than in the other eight treatments (Table 2). The basal calli formed included semi-firm and dense calli (Fig. 2B), loose calli (Fig. 2C), and firm and dense calli (Fig. 2D). The highest number of sprouted adventitious buds was 1.25, and the average bud length was 0.78 cm (Fig. 2D). No calli at basal cut ends of explants and axillary bud were formed when the concentration of IBA added was low (0.05 μmol/L). However, when the concentration of IBA added was high (0.25 μmol/L), the basal callus formation percentage and the fresh weight of calli increased as the concentration of 6-BA applied decreased, and this resulted in a small number of axillary buds.
Figure 2: Induced basal calli and axillary buds in T. amurensis shoots. (A) Shoot explants obtained from aseptic T. amurensis plantlets, bar = 2 cm; (B) Semi-firm calli at basal cut ends of explants (WPM + 0.89 μmol/L 6-BA + 0.25 μmol/L IBA), bar = 1 cm; (C) Loose calli at basal cut ends of explants (WPM + 0.89 μmol/L 6-BA + 0.25 μmol/L IBA), bar = 1 cm; (D) Firm and dense calli at basal cut ends of explants (WPM + 0.89 μmol/L 6-BA + 0.25 μmol/L IBA), bar = 2 cm; (E, F) Axillary bud induced (WPM + 0.89 μmol/L 6-BA + 0.25 μmol/L IBA + 0.46 μmol/L KT + 1.44 μmol/L GA3), bar = 2 cm; (G) Firm and dense calli at basal cut ends of explants (WPM + 0.89 μmol/L 6-BA + 0.25 μmol/L IBA + 0.46 μmol/L KT + 1.44 μmol/L GA3), bar = 2 cm; (H) Adventitious bud differentiation (WPM + 0.89 μmol/L 6-BA + 0.25 μmol/L IBA + 0.46 μmol/L KT + 1.44 μmol/L GA3), bar = 3 cm. Red arrows indicate adventitious shoots
When both GA3 and KT were added, the optimal medium was WPM + 0.89 μmol/L 6-BA + 0.25 μmol/L IBA + 0.46 μmol/L KT + 1.44 μmol/L GA3. The basal callus formation percentage was highest on this medium (68.76%); the basal calli were mostly firm and dense (Fig. 2G); and the fresh weight of the callus tissue was 1,170.78 mg/plant, suggesting that the combination of 6-BA, IBA, KT, and GA3 can promote basal callus formation. The highest axillary bud induction percentage, maximum number of differentiated adventitious buds, and maximum plant height were 41.87%, 3.2, and 5.50 cm, respectively (Figs. 2E–2H). The plantlets grown on the optimal medium were elongated and robust, and their leaves were bright green. Thus, the optimal medium for callus induction and axillary bud formation of T. amurensis shoots was WPM + 0.89 μmol/L 6-BA + 0.25 μmol/L IBA + 0.46 μmol/L KT + 1.44 μmol/L GA3.
3.3 Effects of Different Media and Plant Growth Regulator Combinations on the Proliferation of Adventitious Buds
Adventitious bud proliferation was highest on MS + 2.22 μmol/L 6-BA + 0.25 μmol/L IBA + 1.44 μmol/L GA3. The multiple shoot bud development percentage, number of adventitious shoots, and length of adventitious shoots were highest in this treatment (83.32%, 14.32, and 7.91 cm, respectively), and differences in these three characteristics between this treatment and all other treatments were significant (p < 0.05) (Table 3). The buds observed were robust and had dark-green leaves (Figs. 3A, 3B). The second optimal treatment was MS + 2.22 μmol/L 6-BA + 0.25 μmol/L IBA + 0.29 μmol/L GA3, and the multiple shoot bud development percentage, number of adventitious shoots, and length of adventitious shoots were 74.06%, 11.73, and 5.56 cm, respectively. The third optimal treatment was MS + 0.89 μmol/L 6-BA + 0.25 μmol/L IBA + 1.44 μmol/L GA3, and the multiple shoot bud development percentage, number of adventitious shoots, and length of adventitious shoots were 73.69%, 10.78, and 5.43 cm, respectively.
Figure 3: Proliferation of adventitious buds of T. amurensis. (A–B) The proliferation of adventitious buds, bar = 3 cm
3.4 Effects of Different Basic Media and IBA Concentrations on the Rooting of T. amurensis Plantlets
The rooting percentage (96.70%) and maximum number of roots (2.80) were significantly higher when plantlets were grown on 1/2 MS + 1.48 µmol/L IBA than in the other treatments (p < 0.05) (Figs. 4A, 4B) (Table 4); the average root length of plantlets in this treatment was 8.32 cm. The second most optimal treatment was 1/2 MS + 2.46 µmol/L IBA, and the rooting percentage, maximum number of roots, and average root length of plantlets in this treatment were 87.12%, 2.32, and 8.30 cm, respectively. The root length of plantlets grown on WPM + 1.48 µmol/L IBA was 8.91 cm, but no significant difference was observed in the root length of plantlets grown on this medium and plantlets grown on 1/2 MS + 1.48 µmol/L IBA and 1/2 MS + 2.46 µmol/L IBA (p > 0.05); however, the rooting percentage and maximum number of roots were significantly lower when plantlets were grown on WPM + 1.48 µmol/L IBA than on 1/2 MS + 1.48 µmol/L IBA and 1/2 MS + 2.46 µmol/L IBA (p < 0.05). Thus, the optimal medium for the rooting of T. amurensis plantlets was 1/2 MS + 1.48 µmol/L IBA because the rooting percentage and number of roots of plantlets were highest in this treatment and the root system of the plantlets in this treatment was healthy.
Figure 4: Rooting status of T. amurensis plantlets. (A, B) Rooting of tissue culture plantlet at 45 d, bar = 2 cm
3.5 Cultivation and Transplantation of Regenerated Plantlets
The survival percentage of transplanted plantlets was 82.22% in the soil:vermiculite: perlite (5:3:1) mixture (Figs. 5A–5E).
Figure 5: Transplantation of T. amurensis tissue culture-resulted plantlets. (A, B) Rooting of tissue culture-resulted plantlets, bar = 3 cm; (C) Tissue culture-resulted plantlets following transplantation for 0 d, bar = 5 cm; (D) Tissue culture-resulted plantlets following transplantation for 7 d, bar = 5 cm; (E) Tissue culture-resulted plantlets following transplantation for 3 months, bar = 3 cm
Different plants have different nutritional demands for growth, which might be the reflection of differences in nutrient absorption. The growth of different plants must confirm a rationally basic medium. The compositions of the most commonly used basal media, especially MS, 1/2 MS, DKW (B5), and WPM (1/4 MS) are used to elicit and support various developmental responses [27]. The study by Li et al. [28] suggested that 1/2 MS medium has a better effect than MS, 1/4 MS, and WPM medium in culturing Vernicia fordii. In the cultivation of Rosa canina, researchers suggested Van der Salm (VS) has a better effect than MS medium [29]. In this study, the survival percentage of aseptic plantlets and the growth status of plantlets varied among the basic media. The WPM medium has a better culture effect than MS, 1/2 MS, DKW, and SH medium in culturing aseptic plantlets of T. amurensis. The aseptic plantlets on WPM had thick stems with thick, dark-green leaves. The planting percentage, shoot height, root length, and number of lateral roots of plantlets were highest on WPM. Our findings are similar to the results of a tissue culture study of Paeonia suffruticosa showing that the most suitable medium for the primary culture of scale buds of ‘Fengdan NL10’ is WPM [30].
Several factors can contribute to morphological changes in plant cells, tissues, and organs, and plant growth regulators have particularly important effects on plant morphology [31,32]. In this study, fewer basal compact calli formed, fewer axillary buds were differentiated, and the growth of shoots was weak when only 6-BA and IBA were added to the medium. However, the basal callus formation percentage, axillary bud induction percentage, number of adventitious buds differentiated, and shoot height were highest when 0.89 μmol/L 6-BA, 0.25 μmol/L IBA, 0.46 μmol/L KT, and 1.44 μmol/L GA3 were added to the medium, and the calli were also hard and dense. This indicates that the combined application of 6-BA, IBA, KT, and GA3 plays a key role in basal callus formation and axillary bud formation. In addition, the formation of callus at the base of the shoots significantly increased the axillary bud induction percentage, and several adventitious buds differentiated. Research on tea plants and silver maple has shown that the formation of basal callus is beneficial for the growth and development of axillary buds [16,17], which is consistent with the results of our study. By contrast, the formation of basal callus inhibited the growth and development of axillary buds, and the results of studies of Artemisia chamaemelifolia and Vitex negundo differed [18,19]. This indicates that the effects of the formation of callus at the base of explants on the growth of the axillary buds vary among plants.
The success of proliferation culture is essential for the establishment of large-scale in vitro propagation systems. However, the different basic culture media have different effects on the same variety at the same culture stage [21]. Thus, The selection of an appropriate base medium and concentrations of plant growth regulators is essential for ensuring the high proliferation of adventitious buds [33,34]. We found that MS was superior to WPM for proliferation culture. The application of 6-BA, IBA, and GA3 promotes the proliferation process in plants [35,36]. In our study, the most effective medium for the proliferation culture of T. amurensis plantlets was MS + 2.22 µmol/L 6-BA + 0.25 µmol/L IBA + 1.44 µmol/L GA3, as multiple shoot bud development was highest on this medium. In addition, the buds were strong and bright green, the number of buds was highest, and the adventitious shoots were longest on this medium. The application of an excessively high or low 6-BA concentration was not effective for the proliferation culture of T. amurensis plantlets; the application of an excessively high or low concentration of GA3 was not effective for the proliferation of adventitious buds. These findings indicate that cytokinin is essential for multiple shoot bud development and that GA3 is necessary for large-scale shoot bud regeneration and elongation. The combined application of cytokinin and GA3 promotes shoot bud elongation and increases the multiple shoot bud development percentage. Similarly, the combined application of GA3 and cytokinin promotes shoot bud elongation and multiple shoot bud development in Gymnema sylvestre and Isodon wightii [26,37].
Several factors affect the formation of the adventitious roots of plants, including plant growth regulators, culture medium, and the concentrations of inorganic salts [33,34,38]. In our study, Table 4 shows that different medium and IBA combinations affected the rooting of T. amurensis plantlets. The optimal medium for rooting was 1/2 MS + 1.48 µmol/L IBA. In this treatment, the rooting percentage, root length, and number of main roots were highest. The concentration of IBA and 1/2 MS medium played an important role in the rooting of T. amurensis. Lower concentrations of IBA promoted root formation and growth, and higher concentrations of IBA inhibited root development. Similarly, the optimal media for the rooting of T. japonica and T. cordata were 1/2 MS + 2.46 µmol/L IBA + 0.27 µmol/L NAA and 1/2 MS + 4.92 µmol/L IBA + 1.61 µmol/L NAA, respectively [12,14]. In the rooting research of other plants, the best medium for rooting of Polygonatum cyrtonema Hua explants was: 1/2 MS + 1.00 mg/L NAA [39]. These results were similar to the results of this study. In addition, the best medium that affects the rooting of Dracocephalum rupestre Hance explants was: MS + 0.10 mg/L NAA [40]. In previous studies, we also found that MS medium has a better rooting effect on plants. In further experiments, the effect of 1/2 MS and MS medium on the rooting of T. amurensis explants will be discussed in order to identify a more suitable tissue culture system.
In conclusion, we conducted various experiments using zygotic embryos of mature T. amurensis seeds as explants to establish an efficient in vitro micropropagation system for T. amurensis. Our findings revealed that WPM was the optimal medium for the growth of T. amurensis zygotic embryos. The optimal medium for the formation of basal calli and axillary buds was WPM + 0.89 µmol/L 6-BA + 0.25 µmol/L IBA + 0.46 µmol/L KT + 1.44 µmol/L GA3. The optimal medium for adventitious bud proliferation was MS + 2.22 µmol/L 6-BA + 0.25 µmol/L IBA + 1.44 µmol/L GA3. The optimal rooting medium was 1/2 MS + 1.48 µmol/L IBA. The survival percentage of transplanting explants in soil:vermiculite:perlite (5:3:1) was 82.22%. This study laid a solid foundation for the industrialization of asexual multiplication of plantlets for T. amurensis.
Acknowledgement: The authors thank the reviewers for their thoughtful comments to improve our manuscript.
Funding Statement: This work was supported by the Science and Technology Development Plan Project of Jilin Province, China (20200402115NC).
Author Contributions: S. Lin and Z. Zhang designed the experiment and provided guidance for the study. H. Zhu and T. Gou prepared samples for all analyses. S. Lin, Z. Wang, C. Wang, Y. Luo, D. Zhang and H. He conducted the experiments and statistical analyses. G. Bao and H. He provided advice and interpreted the experiment and analyses. S. Lin and Z. Wang wrote the manuscript. All authors read and approved the manuscript for publication.
Availability of Data and Materials: The data used in the study are available upon request from the corresponding author.
Ethics Approval: Not applicable.
Conflicts of Interest: The authors declare that they have no conflicts of interest to report regarding the present study.
References
1. Thambiraj J, Paulsamy S. Rapid in vitro multiplication of the ethnomedicinal shrub, Acacia caesia (L.) Willd. (Mimosaceae) from leaf explants. Asian Pac J Trop Bio. 2012;2(2):S618–22. [Google Scholar]
2. Chávez-Ortiz LI, Morales-Domínguez JF, Rodríguez-Sahagún A, Pérez-Molphe-Balch E. In vitro propagation of Agave guiengola gentry using semisolid medium and temporary immersion bioreactors. Phyton-Int J Exp Bot. 2021;90(3):1003–13. doi:10.32604/phyton.2021.012862. [Google Scholar] [CrossRef]
3. Arias-Pérez E, Lecona-Guzmán CA, Gutiérrez-Miceli FA, Joaquín Adolfo Montes-Molina JA, Ruiz-Lau N. Encapsulation of immature somatic embryos of Coffea arabica L. for in vitro preservation. Phyton-Int J Exp Bot. 2021;90(6):1741–8. doi:10.32604/phyton.2021.016004. [Google Scholar] [CrossRef]
4. Na S, Wang H, Shen H, Yang L. Techniques of quickly breaking seed dormancy of Tilia amurensis. Adv Mat Res. 2011;183–185:678–82. [Google Scholar]
5. Kim KH, Moon E, Cha JM, Lee S, Yu JS, Kim CS, et al. Antineuroinflammatory and antiproliferative activities of constituents from Tilia amurensis. Chem Pharm Bull. 2015;63(10):837–42. [Google Scholar]
6. Chun SW, Lee JW, Ahn JY. Development and characterization of novel microsatellite markers in Tilia amurensis Rupr. using next‐generation sequencing. Mol Biol Rep. 2022;49(2):1637–41. [Google Scholar] [PubMed]
7. Kang HI, Lee CB, Kwon SH, Park JM, Kang KS, Shim DH. Comparative transcriptome analysis during developmental stages of direct somatic embryogenesis in Tilia amurensis Rupr. Sci Rep. 2021;11(1):6359. [Google Scholar] [PubMed]
8. Zhang J, Y. LV, Li J, Zhang H. Analysis of factors affecting rapid propagation of Tilia amurensis through axillary bud proliferation pathway. Acta Agric Jiangxi. 2018;30(11):23–6. [Google Scholar]
9. Barker WG. Behavior in vitro of plant cells from various sources within the same organism. Can J Bot. 1969;47(8):1334–6. [Google Scholar]
10. Chalupa V. Effect of benzylamlnopurine and thidiazuron on in vitro shoot proliferation of Tilia cordata MILL., Sorbus aucuparia L. and Robinia pseudoacacia L. Biol Plantarum. 1987;29(6):425–9. [Google Scholar]
11. Youn Y, Ishii K, Saito A, Ohba K. In vitro plantlet regeneration from axillary buds of mature trees of Tilia cordata. J Jpn For Soc. 1988;70(7):315–7. [Google Scholar]
12. Bai H, Xing Y. Embryo culture and rapid propagation of Tilia cordata Mill. Plant Physiol Commun. 2008;44(6):1165–6. [Google Scholar]
13. Chalupa V. In vitro propagation of Tilia platyphyllos by axillary shoot proliferation and somatic embryogenesis. J For Sci. 2003;49(12):537–43. [Google Scholar]
14. Chen G, Ma D, Ye J, Pan W, Fan J. Tissue culture and rapid propagation of Tilia japonica Simonk. Plant Physiol Commun. 2007;43(2):327. [Google Scholar]
15. Tang S, Li N, Tang F. Establishment of tissue culture and rapid propagation system of Tilia miqueliana. Jiangsu Agric Sci. 2008;6:87–9. [Google Scholar]
16. Preece JE, Huetteman CA, Ashby WC, Roth PL. Micro and cutting propagation of silver maple. I. results with adult and juvenile propagules. J Am Soc Hortic Sci. 1991;116(1):142–8. [Google Scholar]
17. Ren L, Zhang Y, Huang K, Wan X, Zhang Z, Zhu M, et al. An efficient system for regenerating adventitious buds in stem segments of tea plants. Chin Bull Bot. 2023;58(2):308–15. [Google Scholar]
18. Steephen M, Nagarajan S, Ganesh D. Phloroglucinol and silver nitrate enhances axillary shoot proliferation in nodal explants of Vitex negundo L.–an aromatic medicinal plant. Iran J Biotechnol. 2010;8(2):82–9. [Google Scholar]
19. Hristova L, Damyanova E, Doichinova Z, Kapchina-Toteva V. Effect of 6-benzylaminopurine on micropropagation of Artemisia chamaemelifolia Vill.(Asteraceae). Bulg J Agric Sci. 2013;19(2):57–60. [Google Scholar]
20. Abdullah R, Zainal A, Heng WY, Li LC, Beng YC, Phing LM, et al. Immature embryo: a useful tool for oil palm (Elaeis guineensis Jacq.) genetic transformation studies. Electron J Biotechnol. 2005;8(1):24–34. [Google Scholar]
21. Thuzar M, Vanavichit A, Tragoonrung S, Jantasuriyarat C. Efficient and rapid plant regeneration of oil palm zygotic embryos cv. ‘Tenera’ through somatic embryogenesis. Acta Physiol Plant. 2011;33(1):123–8. [Google Scholar]
22. Nas MN, Read PE. A hypothesis for the development of a defined tissue culture medium of higher plants and micropropagation of hazelnuts. Sci Hortic. 2004;101(1–2):189–200. [Google Scholar]
23. Murashige T, Skoog F. A revised medium for rapid growth and bioassay with tobacco tissue culture. Physiol Plant. 1962;15(3):473–97. [Google Scholar]
24. Schenk RU, Hildebrandt AC. Medium and techniques for induction and growth of monocotyledonous and dicotyledonous plant cell cultures. Can J Bot. 1972;50(1):199–204. [Google Scholar]
25. Driver JA, Kuniyuki AH. In vitro propagation of paradox walnut rootstock. HortScience. 1984;19(4):507–9. [Google Scholar]
26. Thiyagarajan M, Venkatachalam P. A reproducible and high frequency plant regeneration from mature axillary node explants of Gymnema sylvestre (Gurmur)–An important antidiabetic endangered medicinal plant. Ind Crop Prod. 2013;50:517–24. [Google Scholar]
27. Phillips GC, Garda M. Plant tissue culture media and practices: an overview. Vitro Cell & Dev Biol−Plant. 2019;55(3):242–57. [Google Scholar]
28. Li Z, Tan X, Zhang L, Long H, Yuan J, Fan X, et al. Establishment of regeneration system for mature embryo in Vernicia fordii. Nonwood For Res. 2012;30(4):119–22. [Google Scholar]
29. Samiei L, Davoudi Pahnehkolayi M, Tehranifar A, Karimian Z. Organic and inorganic elicitors enhance in vitro regeneration of Rosa canina. J Genet Eng Biotechnol. 2021;19:60. [Google Scholar] [PubMed]
30. Liu J, Wang S, Li S, Yin T, Wen S, Li X. Primary study on tissue culture and propagation of a variety ‘Fengdan NL10’ rich in edible oil. Mol Plant Breed. 2021;19(16):5447–57. [Google Scholar]
31. Avdiu V, Thomaj F, Sylanaj S, Kullaj E, Lepaja K. Effect of growth regulators on the sap flow in the apple tree. Acta Hortic. 2016;1139:323–8. [Google Scholar]
32. Hajam MA, Hassan GI, Bhat TA, Bhat IA, Rather AM, Parray EA, et al. Understanding plant growth regulators, their interplay: for nursery establishment in fruits. Int J Chem Stud. 2017;5(5):905–10. [Google Scholar]
33. Malik K, Birla D, Yadav H, Sainger H, Chaudhary D, Jaiwal PK, et al. Evaluation of carbon sources, gelling agents, growth hormones and additives for efficient callus induction and plant regeneration in Indian wheat (Triticum aestivum L.) genotypes using mature embryos. J Crop Sci Biotechnol. 2017;20(3):185–92. [Google Scholar]
34. Ameh SJ, Aguoru CU, Iheukwumere CC, Olasan OJ, Alfred UJ. Micro propagation of Pterocarpus santalinoides using three different growth media. J Adv Biol Biotechnol. 2019;21(3):1–9. [Google Scholar]
35. Loganathan K, Bai VN. High frequency in vitro plantlet regeneration and antioxidant activity of Enicostema axillare (Lam.) Raynal ssp. littoralis (Blume) Raynal: an important medicinal plant. Asian Pac J Reprod. 2014;3(3):241–8. [Google Scholar]
36. Otroshy M, Moradi K, Nekouei MK. The effect of different cytokenins in propagation of Capsicum annuum L. by in vitro nodal cutting. Trakia J Sci. 2011;9(3):21–30. [Google Scholar]
37. Thirugnanasampandan R, Mahendran G, Bai VN. High frequency in vitro propagation of Isodon wightii (Benthan) H. Hara. Acta Physiol Plant. 2010;32(2):405–9. [Google Scholar]
38. Fattorini L, Falasca G, Kevers C, Rocca LM, Zadra C, Altamura MM. Adventitious rooting is enhanced by methyl jasmonate in tobacco thin cell layers. Planta. 2009;231(1):155–68. [Google Scholar] [PubMed]
39. Zhou X, Zhu Y, Gui S, Li Y, Nie G. Study on inducing roots from Polygonatum cyrtonema plantlets. Nonwood For Res. 2015;33(4):102–5. [Google Scholar]
40. Zhang G, Shi X, Pan H, Dai T. Study on callus induction and tissue culture regeneration in vitro of Dracocephalum Rupestre. Bot Res. 2019;8(6):525–32. [Google Scholar]
Cite This Article
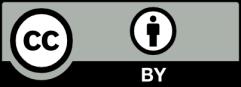