Open Access
ARTICLE
Application of Polygonum minus Extract in Enhancing Drought Tolerance in Maize by Regulating Osmotic and Antioxidant System
1 Department of Land Management, Faculty of Agriculture, Universiti Putra Malaysia, Serdang, 43400, Malaysia
2 Natural Medicines and Products Research Laboratory, Institute of Bioscience, Universiti Putra Malaysia, Serdang, 43400, Malaysia
3 Department of Cell and Molecular Biology, Faculty of Biotechnology and Biomolecular Sciences, Universiti Putra Malaysia, Serdang, 43400, Malaysia
* Corresponding Author: Susilawati Kasim. Email:
(This article belongs to the Special Issue: New Approaches to Mitigate Abiotic and Biotic Stresses for Improving Crop Productivity and Quality)
Phyton-International Journal of Experimental Botany 2024, 93(2), 213-226. https://doi.org/10.32604/phyton.2024.047150
Received 27 October 2023; Accepted 11 December 2023; Issue published 27 February 2024
Abstract
Drought stress is a major factor affecting plant growth and crop yield production. Plant extracts as natural biostimulants hold great potential to strengthen plants to overcome drought impacts. To explore the effect of Polygonum minus extract (PME) in enhancing drought tolerance in plants, a study was set up in a glasshouse environment using 10 different treatment combinations. PME foliar application were designed in CRD and effects were closely observed related to the growth, physiology, and antioxidant system changes in maize (Zea mays L.) under well-watered and drought conditions. The seaweed extract (SWE) was used as a comparison. Plants subjected to drought stress exhibited a significant reduction in fresh weight, dry weight, relative water content (RWC), and soluble sugar, but they stimulated the phenolic, flavonoid, proline, glutathione (GSH), malondialdehyde (MDA) and antioxidant enzyme (catalase, CAT; peroxidase, POD; superoxide dismutase, SOD) activities. Foliar application of PME improved fresh and dry weight (FW: 33.1%~41.4%; DW: 48.0%~43.1%), chlorophyll content (Chl b: 87.9%~100.76%), soluble sugar (23.6%~49.3%), and soluble protein (48.6%~56.9%) as well as antioxidant enzyme activities (CAT and POD) compared to CK under drought conditions. while decreasing the level of MDA. Notably, the mitigating effect of PME application with high concentration was more effective than those of SWE. Our study reveals that PME could alleviate drought stress by regulating osmoprotectant content and antioxidant defense system and can be used as an economical and environmentally friendly biostimulants for promoting maize growth under drought stress.Keywords
Maize (Zea mays L.), as an economically important food and forage crop, is widely grown all over the world under a various of climates and plays a prominent position in agricultural production [1]. However, the growth of maize is highly susceptible to various abiotic stresses. Studies have shown that maize yield is most sensitive to water deficit at the tasseling stage, because water deficit at the tasseling stage leads to a decline in pollen vigor, which also leads to loss in yield [2,3]. Besides, drought stress is the most crucial abiotic stress and about one-third of the world’s arable land has been restricted due to unpredictable and seasonal drought which severely threatens agricultural sustainability [4]. Water deficit causes the accumulation of Reactive Oxygen Species (ROS) in plants, and overproduces ROS affects a series of damage such as physiological disorders, stomatal conductance, and membrane lipid peroxidation [5–7].
Plants have developed intrinsic mechanisms to regulate photosynthesis and maintain water conditions by regulating physiological and biochemical functions in the face of abiotic stress conditions [8–12]. However, these endogenous physiological regulations are usually not sufficient to help plants effectively to resist abiotic stress. With these problem in mind, biostimulants, including amino acids, humid substances, plant extracts, protein hydrolysates and beneficial fungi, have been proposed as potential natural agents to promote nutrient absorption and plant development and enhance tolerance of abiotic and biotic stresses [13,14]. At the same time, biostimulants also can reduce the dependence on chemical fertilizer and pesticides [15].
Plant extract, as a kind of biostimulants, contain a variety of phytochemical compounds and nutrients, which improve physiological and biochemical processes of plant [16,17]. In recent years, seaweed extracts (SWE) have been widely used in vegetable and crop research, and study has indicated that application of SWE may increase phytochemical such as phenolic and flavoniods as well as antioxidant capacity [18,19]. Elansary et al. [20] reported that application of SWE can increase plant height and dry weight of Spiraea nipponica and Pittosporum eugenioides by regulating stomatal conductance and photosynthetic rate. Mutale-Joan et al. [21] found that microalgae liquid extracts improve root and shoot length and dry weight, meanwhile, the maximum uptake of nitrogen, phosphorus, and potassium were increased by 185.17%, 119.36%, and 78.04%, respectively, compared with non-treated plants. Besides, the use of natural extracts from various plant components such as licorice, cypress leaf, and pollen grains also has been reported to enhance plant tolerance to drought stress [16,17,22].
Polygonum minus (P. minus), commonly known as ‘kesum’ in Malaysia, is widely used in cooking and for its medicinal properties, particularly in treating stomach-related illnesses [23]. Previous pharmacological studies have revealed P. minus have a high antioxidant content, making it as a subject of considerable interest [24]. However, studies using P. minus extract (PME) as a biostumulants to enhance stress tolerance in maize have not been conducted. Thus, we hypothesize that PME is associated with antioxidant activities and may have beneficial effect in promoting plant tolerance to abiotic stresses and this will stabilize the plant growth. To the best of our knowledge, this study needs to be investigated by treating maize with foliar spraying different concentrations of PME. The effects on morphology, physiology, and antioxidant activity of maize under drought condition were identified, and SWE was used as a comparison. This study aim to utilize PME as a drought mitigator for maize planted using sustainable agriculture approach.
2.1 Preparation and Analysis of SWE and PME
The Polygonum minus was purchased from a local supplier. The materials were extracted as details in Taha et al. [22] method with modification. Each 10 g of materials were mixed with 80% methanol using an optimum ratio of 1/8 (w/v) sample weight to solvent volume. Thereafter, the mixtures were continuously shaken at 150 rpm for 24 h using an orbital shaker. The extraction samples were filtered with Whatman No.1 filter paper, and the alcohol and excess water were evaporated under vacuum at 30°C using a rotary evaporator. The Polygonum minus extract (PME) obtained was made as an extract at a concentration of 1.0 g/L by dissolving in 10 L distilled water. The extracts were kept in refrigerator at 4°C until use. The seaweed extract (SWE) was purchased from Maize Star Resources Sdn. Bhd., Malaysia, and concentration was configured according to the instructions: 1 g seaweed extract was dissolved in 1 L of distill water. The chemical constituents of SWE and PME (on a dry weight basis) are shown in Table 1.
2.2 Plant Material and Growing Conditions
This experiment was carried out from March to June 2023 in a glasshouse at Faculty of Agriculture (2° 98′ N, 101° 73′ E), University Putra Malaysia (UPM), Selangor, Malaysia. Maize seeds (Zea mays L., Hybrid F1 316, Malaysia) were brought from local supplier. Prior to germination step, growth medium using mixed soil was prepared using sand and mineral soil (Oxisols, pass 2 mm sieve) at 1 to 4 ratio. Later, four seeds were sown in plastic pots (24 cm in diameter, 28 cm in depth) filled with mixed soil (14 kg). The basic properties for mixed soil are: total carbon 1.55 g kg−1, total nitrogen 2.10 g kg−1, total phosphorus 3.87 g kg−1, available phosphorus 0.53 g kg−1, total potassium 3.40 g kg−1, CEC 4.51 cmol kg−1. All pots were placed in a completely randomized design (CRD) and the equal amount of water was added to the pot until imposition of drought stress. After 2 weeks, save only one of the best quality seedlings in each pot. A total of 15 g compound fertilizer (N:P:K, 15:15:15) was applied to each pot: 5 g at sowing, after 28 and 56 days, respectively. The average day and night net glasshouse temperature was 38.80°C ± 2.60°C and 27.52°C ± 1.39°C, whereas average day and night net relative humidity was 40.14% ± 4.45% and 82.00% ± 4.42%, respectively.
2.3 PME Treatments and Experimental Design
The plants grew under normal water condition until tasseling (56 days) before they were divided into well-watered (75% soil field capacity) and drought (35% soil field capacity) conditions. Total 10 treatments were examined as follows: well-watered, well-watered with SWE, well-watered with PME1, well-watered with PME3, well-watered with PME5, drought, drought with SWE, drought with PME1, drought with PME3, and drought with PME5 (1, 3 and 5 indicate different concentrations). The concentration of 1 and 5 g L−1 based on Taha et al. [22] and Pourghasemian et al. [16] recommendation, and the middle value (3 g L−1) was established as a comparison value. Each treatment was performed 4 repetitions. SWE and PME application were applied by using foliar spraying once a week and at each application, it is important to ensures that all leaves were sprayed evenly. The moisture of each pot was monitored everyday by soil moisture meter and maintained to desired soil water conditions by adding appropriate volumes of water. The top leaves of maize were collected after the last spray (84 days) and stored at −80°C until analysis of plant physiology, antioxidant as well as enzyme activity.
2.4 Morphological Parameters and RWC
The fresh plant weight is measured immediately, and the dry weight is measured after the sample is placed in the oven to constant weight. Plant height was measured by ruler.
Leaf relative water content (RWC) was assessed using the methods described by Wang et al. [25]. Briefly, the leaves were measured to fresh weight (FW) and immersed in distilled water for 24 h to record the turgid weight (TW). After that, the leaves were oven-dried to record the dry weight (DW). The RWC was expressed as follows:
RWC (%) = [(FW − DW)/(TW − DW)] × 100%
Chlorophyll and carotenoid contents were assessed and then calculated as detailed described in Pourghasemian et al. [16]. Samples of 0.3 g fresh leaves were extracted in 20 mL acetone (80%) and left in the dark. After 24 h of the incubation, absorbance (A) was recorded at wavelengths of 646.8, 663.2 and 470 nm. Chl a, Chl b and carotenoids were calculated using the following equation:
2.6 Leaf Total Phenolic and Flavonoid Content
The leaf extracts were obtained using methanol method [22] with modification. Briefly, 0.3 g dry leaf samples were cut into small pieces and extracted in 10 mL 80% methanol solution for 24 h at room temperature. After centrifuged at 10,000 × g at 4°C, the supernatant was used to determine the total phenolic and flavonoid content using the Folin-Ciocalteu colorimetric method and the method described by Miliauskas et al. [26], using gallic acid and rutin as the reference, respectively.
2.7 Osmoprotectants (Proline and Soluble Sugar)
Soluble sugar content was measured by the anthrone colorimetric method [27]. Briefly, 0.3 g fresh maize samples were homogenized with 10 mL ethanol (80%) and then centrifuged at 10,000 × g at 4°C for 10 min. The extract was mixed with 5 mL of anthrone reagent and then heated at 100°C in water bath for 10 min, and the absorbance was read at 630 nm after cooling to room temperature. Proline quantification was determined following the method of Bates et al. [28] and the absorbance was read at 520 nm.
2.8 Glutathione and Lipid Peroxidation
Glutathione (GSH) contents were quantified using the method described by Griffth [29]. Briefly, 0.3 g fresh leaves were extracted with 10 mL 5 % trichloroacetic acid (TCA) and centrifuged at 4,000 × g for 15 min. The 2 mL of extract was mixed with 0.4 mL of 5, 5’-dithiobis (2-nitrobenzoate) (DTNB), the absorbance was read at 412 nm. Lipid peroxidation was indicated by Malondialdehyde (MDA) following de Vos et al. [30] method. The same supernatant (same as GSH) was used to determine MDA. The 2 mL of extract was mixed with 2 mL of 0.6% 2-thiobarbituric acid (TBA), and the mixture was heated at 95°C for 15 min and cooled immediately in an ice bath. The absorbance of supernatant was read at 532 nm after centrifugation at 4,000 × g for 10 min.
2.9 Antioxidant Enzyme Activities and Protein
Fresh maize samples were homogenized with potassium buffer at pH 7.8 to assess the antioxidant enzyme activities and protein. The mixture was then centrifuged at 12,000 × g for 10 min at 4°C. The supernatant was used to measure antioxidant enzyme activities using the method described by Zafari et al. [31]. The activity of superoxide dismutase (SOD) was quantified by measuring the inhibition of nitro blue tetrazolium (NBT) reduction under light. The peroxidase (POD) activity was determined by assessing the oxidation of guaiacol after the addition of H2O2. The catalase (CAT) activity was determined by assay kits purchased by Nanjing Jiancheng Bioengineering Institute, China. The protein content was measured according to Bradford [32] using bovine serum albumin as the standard.
Data were analyzed using SPSS 25.0 (IBM, New York, NY, USA). Significant differences between treatments were calculated by one-way analysis of variance (ANOVA) with LSD test at p < 0.05. The regression relationships between all parameters and treatments (well-watered, drought, PME and SWE application) were analyzed by unitary linear regression. Figures and heat map were created by Origin 2018 software (OriginLab, Northampton, MA, USA).
Drought stress caused a significant impact on fresh weight (FW), dry weight (DW) and number of leaves (Fig. 1). FW and DW were affected by foliar spraying under both well-watered and drought conditions. The result shows that SWE and PME treatments significantly increased FW and DW under drought condition, and in all PME treatments evaluation parameters, except height, all were significantly higher than CK. Generally, the SWE, and PME5 recorded the highest value, exhibiting a 41.4% and 53.1% higher than CK, respectively (p < 0.05). Additionally, it is worth noting that PME3 and PME5 showed significantly increased in FW by 8.6% and 9.3% and DW by 15.1% and 16.4%, respectively, compared to CK under well-watered condition. However, there were no significant differences observed in plant height among all foliar spraying treatments.
Figure 1: The analysis of variance of the effects of water conditions (well-watered and drought) and foliar application of SWE and PME treatments (1, 3 and 5 as concentration) on growth of maize. Different lowercase letters indicate significant influences between the same water conditions with different treatments (n = 4, p < 0.05)
3.2 Photosynthetic Pigments and RWC
Chlorophyll a, chlorophyll b and carotenoids were significantly affected by foliar spraying and the drought stress condition (Fig. 2). Under well-watered condition, the PME5 treatment exhibited the highest content of chlorophyll a, chlorophyll b and carotenoids with significant increases of 91.2%, 113.7% and 100.0% compared to the control, respectively (p < 0.05). Under drought conditions, SWE and different concentrations of PME treatments able to increase the contents of chlorophyll a, b and carotenoids in different extents compared to the control. Among them, chlorophyll b and carotenoids showed the most significant differences. As for chlorophyll a, only SWE and PME1 showed significant improvements of 30.4% and 16.8% compared to CK, respectively. Furthermore, the results in Fig. 2 indicated that foliar spraying has no obvious influence on relative water content (RWC) under well-watered condition. However, under drought stress, both SWE and PME3 treatments were significantly higher than CK by 21.9% and 26.1%, respectively (p < 0.05).
Figure 2: The analysis of variance of the effects of water conditions (well-watered and drought) and foliar application of SWE and PME treatments (1, 3 and 5 as concentration) on chlorophyll and relative water content of maize. Different lowercase letters indicate significant influences between the same water conditions with different treatments (n = 4, p < 0.05)
3.3 Total Phenolic and Flavonoid Content
Drought stress caused an increase of total phenolic and flavonoid concentration in maize, however, both SWE and PME treatments showed no significant differences compared to CK (Fig. 3). Among them, the PME5 treatment exhibited the highest concentration of total phenolic and flavonoid with increase of 6.7% and 4.8% compared to CK, respectively.
Figure 3: The analysis of variance of the effects of water conditions (well-watered and drought) and foliar application of SWE and PME treatments (1, 3 and 5 as concentration) on total phenolic and flavonoid of maize. Different lowercase letters indicate significant influences between the same water condition with different treatments (n = 4, p < 0.05)
Proline content increased by 50.6% under drought conditions, whereas soluble sugar content decreased by 31.5% as shown in Fig. 4. All PME treatments had no significant effect on proline, but SWE treatment significantly decreased 25.9% compared to the CK under drought condition (p < 0.05). Regarding soluble sugar content, both SWE and PME treatments led to an increase of the value compared to CK. The highest concentration was observed in SWE treatment under well-watered condition, which was significantly higher by 11.3%. Under drought conditions, the soluble content of SWE, PME1, PME3 and PME5 was significantly increased by 46.4%, 23.6%, 32.9% and 49.3%, respectively (p < 0.05).
Figure 4: The analysis of variance of the effects of water conditions (well-watered and drought) and foliar application of SWE and PME treatments (1, 3 and 5 as concentration) on proline, soluble sugar, GSH and MDA of maize. Different lowercase letters indicate significant influences between the same water conditions with different treatments (n = 4, p < 0.05)
3.5 Glutathione and Lipid Peroxidation
Glutathione (GSH) and Malondialdehyde (MDA) concentration were significantly affected by both drought stress and foliar spraying, as depicted in Fig. 4. Under drought stress condition, GSH and MDA accumulation increased by 308.3% and 13.5%, respectively, compared to the well-watered condition. Both SWE and PME treatments led to a significant increase in GSH content compared to the control under well-watered and drought conditions (p < 0.05). Notably, under drought condition, PME treatments have the largest improvement compared to CK, where the enhancements ranging from 108.2% to 138.8%. On the contrary, MDA levels displayed a decreasing trend in foliar spraying (Fig. 4). Among the treatments, PME3 treatment had significant difference under well-watered condition, while both SWE, PME3 as well as PME5 treatments had significant differences under drought stress condition (p < 0.05).
3.6 Antioxidant Enzyme Activities and Protein
The data showed that antioxidant enzymes activities (CAT, SOD and POD) were affected by both drought stress and foliar spraying of SWE and PME (Fig. 5). All the antioxidant enzyme activities were increased when they received changes amount of water from well-watered and drought stress condition. Under drought condition, both SWE and PME treatments significantly increased CAT activity compared to CK, while there was no significant difference under well-watered condition. The SOD activity in all foliar spraying treatments, except PME5, was lower than CK. Specifically, under drought stress, SWE, PME1 and PME3 treatments showed a significant reduction in SOD activity by 15.9%, 19.4% and 21.4%, respectively (p < 0.05). Furthermore, foliar spraying of both SWE and PME increased POD levels compared to CK treatment, and PME5 significantly increased 22.6% and 15.3%, respectively, under well-watered and drought stress conditions (p < 0.05).
Figure 5: The analysis of variance of the effects of water conditions (well-watered and drought) and foliar application of SWE and PME treatments (1, 3 and 5 as concentration) on antioxidant enzyme activity and protein of maize. Different lowercase letters indicate significant influences between the same water condition with different treatments (n = 4, p < 0.05)
Protein was significantly affected by foliar spraying of SWE and PME and all treatments were higher in drought stress than in well-watered condition (Fig. 5). Under drought condition, PME treatments have the largest improvement compared to CK, with enhancements ranging from 108.2% to 138.8%. Under the drought condition, the protein content was improved obviously after treated with foliar spraying. Among all treatments, SWE, PME1, PME3 and PME5 were significantly higher than CK by 57.3%, 55.5%, 56.9% and 48.6%, respectively (p < 0.05).
3.7 Correlation Analysis of the Physiological Responses after Applying Biostimulants under Drought
In order to investigate potential correlations among various physiological and biochemical characteristics influenced by SWE and PME under drought-induced stress, a heat map be constructed (Fig. 6). The heat map visually represents the physiological and antioxidant-related responses of maize crop under two sets of conditions: well-watered conditions (with CK, SWE and PME treatments) and drought stress conditions (with CK, SWE and PME treatments). It can be seen from the heat map that plant height, FW, DW, number of leaves, RWC and sugar exhibited negative correlations with drought stress. Additionally, under drought stress condition, foliar spraying of PME resulted in significantly positive correlations with FW, DW, Chl b, carotenoid, RWC, sugar, GSH, protein and CAT activity, meanwhile the MDA and SOD activity exhibited a significant negative correlation. The heat map also shown that different physiological responses were increased with SWE treatment.
Figure 6: Overall relationship analysis of plant growth and physiological parameters in exogenous biostimulants application under drought stress; *p < 0.05; **p < 0.01
Drought stress has a detrimental impact on plant growth and development, influencing various physiological and biochemical characteristics [33,16]. The choice of maize as the subject of this study because of it is sensitive to water availability, making it a pivotal model for both physiological and agronomic studies. The adverse effects of drought stress on morphological aspects such as plant height and biomass weight and grain yield parameters have been reported in maize [34]. This study also indicated that drought stress reduced morphological parameters (plant height, FW, DW and number of leaves) compared with well-watered plants (Fig. 1). This is because water deficit causes stomatal closure and reduction of CO2 intake which result in decrease of photosynthesis [25]. Besides, under well-watered and drought stress condition, foliar spraying of PME promoted almost all the morphological parameters compared to the control. This result is similar to that of the Rady et al. [35] and Anjum et al. [34] studies. According to the fact, Polygonum minus extract (PME) is rich in osmoprotectants (i.e., proline and soluble sugar), phytochemical compounds (i.e., phenolic and flavonoid), mineral nutrients (i.e., N, P, K, Ca, Mg, Fe, Cu and so on) and glutathione (Table 1). The presence of these key components makes PME potentially beneficial to plant growth. Furthermore, these components might be attributed to the improvement of chlorophyll levels (Fig. 2), osmoprotectant and antioxidant content (Fig. 4). Typically, RWC exhibit a decline when leaves face drought stress due to its strong correlation with water uptake through the roots and water transpiration [36]. This study showed that application of PME leads to an increase in RWC under drought condition, which might be explained by reduced stomatal closure and increased efficiency of water uptake.
Drought stress primarily leads to the changes in plant water status that subsequently affects the transpiration rate and the stomatal conductance in leaves, limits CO2 acquisition, and finally inhibits photosynthesis [37]. Interestingly, without the foliar application, drought still increased chlorophyll content (Fig. 2), which due to the concentrating effect caused by the limitation in plant growth [38]. Previous study has reported that foliar and drench application of SWE can improve stomatal conductance, transpiration and photosynthesis rate through reducing stomatal closure and increasing gas exchange values [20]. Our findings showed that the application of PME not only increased chlorophyll content under drought conditions, but also significantly increased chlorophyll content at higher concentrations (PME3 and PME5) under well-water condition (Fig. 2), confirming the ability of SWE to diffuse CO2 and increase plant photosynthesis rate. This was possibly due to application of PME results in the accumulation of high levels of compatible solutes, decreased ROS levels and improved activities of chlorophyll synthesising enzymes to reduce chlorophyll degradation [39]. Additionally, the enrichment of growth stimulants such as amino acids, phytohormons and many mineral nutrient elements within PME contributes to the maintenance of the photosynthetic machinery through the regulation of the antioxidant defense system [40]. Phenolic and flavonoid compounds are major plant secondary metabolites that have strong antioxidant activities and important function in stress resistance [20]. Proline content has been associated with the accumulation of free radicals generated by abiotic stress factors [41]. In this study, total phenolic, flavonoid and proline content in maize leaves was observed to be higher under drought stress compared to well-watered condition, whereas the improvement effect of SWE and PME was not significant (Fig. 3). This result is not in accordance with Elansary et al. [20], who reported that phenolic and flavoniod content of Spiraea and Pittosporum were significantly increased with application of SWE. Therefore, the response of phytochemical compound and proline accumulation to exogenous biostimulants is not constant, and the extent of its accumulation may depends on the degree of drought and plant species or genetic factors. In addition, this study showed that the application of PME remarkably increased soluble sugar and protein in maize plants compared to the control under drought stress (Figs. 4 and 5). Similar results have been reported by Wang et al. [25], who revealed the sugar possessing key function in maintaining cell osmotic potential when plant be subjected to drought stress. Consequently, this result further indicated the adjustment ability of PME to osmolyte accumulation in response to stress. GSH as an antioxidant molecule and plays a critical function in maintaining the cellular redox balance and scavenging ROS in plant cells [42]. Our results also showed that foliar spraying of PWE can significantly increase GSH content compared to the control in both well-watered and drought stress conditions (Fig. 4), thus confirming the positive effect on antioxidant defense system. Although maize plants exhibited an increase in MDA levels under drought stress, application of PME significantly reduced MDA concentration compared to the control. MDA is used to characterize the degree of free radical damage to cell membrane caused by oxidative stress. This finding comprehensively elucidated that PME at a certain concentration, have a significant effect in protecting lipid peroxidation under drought stress.
Oxidative damage is closely related to the antioxidant defense mechanism, and plants with abiotic stress tolerance exhibited higher antioxidant enzyme activity [43]. Our observation showed that maize leaves exposed to drought stress displayed higher activity of the antioxidant enzymes (CAT, POD and SOD) compared to well-watered condition, and foliar sparing of PME further increased CAT and POD activities (Fig. 5), suggesting PME has the potential to mitigate oxidative damage by stimulating the activities of antioxidant enzymes. CAT plays a key role in the plant cell and convert H2O2 to water and oxygen, which leads to protecting photosynthesis against drought stress [44]. Similarly, application of exogenous pollen grains extract, which contain amino acids, sugars, and nutrient elements, can increase the activities of CAT and SOD in Ocimum basilicum under drought stress [22]. In addition, maize crop was able to maintain POD activity for detoxifying ROS in response to water deficit [34]. Summarily, under both well-watered and drought stress conditions, maize was performed well with PME and SWE. In addition, PME is easy to prepare, extract and it is inexpensive. Therefore, it can be used as a natural bioactive mitigator to help plants to enhance their tolerance to drought stress.
Foliar application of PME exhibits notable biostimulatory effects and significantly improves maize growth performance by increasing chlorophyll and osmoprotectant content and stimulating antioxidant under drought stress. In addition, PME application significantly reduced oxidative damage caused by drought stress via improving accumulation of low molecule antioxidants and increasing antioxidant enzyme activities. Our findings clearly indicate that PME is an effective bioactive stimulant to alleviate negative effects of drought stress and has potential to improve crop yield. Nevertheless, the yield-related parameters and molecular mechanisms by which PME regulates drought resistance are not known and will be further validated in future field trials.
Acknowledgement: Not applicable.
Funding Statement: This paper was supported by Universiti Putra Malaysia, Innohub Grant Scheme (Vote No. 9005004), and D’Khairan Farm Sdn Bhd (Vote No. 6300349).
Author Contributions: The authors confirm contribution to the paper as follows: Study conception and design: Mingzhao Han, Susilawati Kasim; data collection: Effyanti Mohd Shuib; analysis and interpretation of results: Zhongming Yang, Xi Deng and Md Kamal Uddin; draft manuscript preparation: Mingzhao Han and Noor Baity Saidi. All authors reviewed the results and approved the final version of the manuscript.
Availability of Data and Materials: The data that support this study will be shared upon reasonable request to the corresponding author.
Ethics Approval: Not applicable.
Conflicts of Interest: The authors declare that they have no conflicts of interest to report regarding the present study.
References
1. Cairns JE, Prasanna BM. Developing and deploying climate-resilient maize varieties in the developing world. Curr Opin Plant Biol. 2018;45:226–30. [Google Scholar] [PubMed]
2. Fuad-Hassan AVAN, Tardieu F, Turc O. Drought-induced changes in anthesis silking interval are related to silk expansion: a spatio-temporal growth analysis in maize plants subjected to soil water deficit. Plant Cell Environ. 2008;31(9):1349–60. [Google Scholar] [PubMed]
3. Wang J, Guo S, Kang S, Wang Y, Du T, Tong L. Joint optimization of irrigation and planting. pattern to guarantee seed quality, maximize yield, and save water in hybrid maize seed production. Eur J Agron. 2020;113:125970. [Google Scholar]
4. Chaves MM, Oliveira MM. Mechanisms underlying plant resilience to water deficits: prospects for water-saving agriculture. J Exp Bot. 2004;55(407):2365–84. [Google Scholar] [PubMed]
5. Hussein Y, Amin G, Azab A, Gahin H. Induction of drought stress resistance in sesame (Sesamum indicum L.) plant by salicylic acid and kinetin. J Plant Sci. 2015;10(4):128–41. [Google Scholar]
6. Batra NG, Sharma V, Kumari N. Drought-induced changes in chlorophyll fluorescence, photosynthetic pigments, and thylakoid membrane proteins of Vigna radiata. J Plant Interact. 2014;9(1):712–21. [Google Scholar]
7. Sleimi N, Bankaji I, Touchan H, Corbineau F. Effects of temperature and water stresses on germination of some varieties of chickpea (Cicer arietinum). Afr J Biotech. 2013;12(17):2201–6. [Google Scholar]
8. Hossain MS, ElSayed AI, Moore M, Dietz KJ. Redox and reactive oxygen species network in acclimation for salinity tolerance in sugar beet. J Exp Bot. 2017;68(5):1283–98. [Google Scholar] [PubMed]
9. Ganie SA, Ahammad GJ. Dynamics of cell wall structure and related genomic resources for drought tolerance in rice. Plant Cell Rep. 2021;40:437–59. [Google Scholar] [PubMed]
10. Ganie SA, Reddy AS. Stress-induced changes in alternative splicing landscape in rice: functional significance of splice isoforms in stress tolerance. Biol. 2021;10(4):309. [Google Scholar]
11. Sghaier DB, Bankaji I, Sylvia P, Caçador I, Sleimi N. Photosynthetic behaviour and mineral nutrition of Tamarix gallica cultivated under aluminum and NaCl combined stress. Phyton-Int J Exp Bot. 2019;88(3):239–52. [Google Scholar]
12. Bouslimi H, Ferreira R, Dridi N, Brito P, Martins-Dias S, Caçador I, et al. Effects of Barium stress in Brassica juncea and Cakile maritima: the indicator role of some antioxidant enzymes and secondary metabolites. Phyton-Int J Exp Bot. 2021;90(1):145–58. [Google Scholar]
13. Bulgari R, Franzoni G, Ferrante A. Biostimulants application in horticultural crops under abiotic stress conditions. Agron. 2019;9(6):306. [Google Scholar]
14. Mrid RB, Benmrid B, Hafsa J, Boukcim H, Sobeh M, Yasri A. Secondary metabolites as biostimulants and bioprotectant agents: a review. Sci Total Environ. 2021;777:146204. [Google Scholar]
15. Cuadrado CJL, Pinillos EO, Tito R, Mirones CS, Gamarra Mendoza NN. Insecticidal properties of capsaicinoids and glucosinolates extracted from Capsicum chinense and Tropaeolum tuberosum. Insects. 2019;10(5):132. [Google Scholar]
16. Pourghasemian N, Moradi R, Naghizadeh M, Landberg T. Mitigating drought stress in sesame by foliar application of salicylic acid, beeswax waste and licorice extract. Agric Water Manag. 2020;231:105997. [Google Scholar]
17. ElSayed AI, Rafudeen MS, Ganie SA, Hossain MS, Gomaa AM. Seed priming with cypress leaf extract enhances photosynthesis and antioxidative defense in zucchini seedlings under salt stress. Sci Hortic. 2022;293:110707. [Google Scholar]
18. Xu C, Leskovar DI. Effects of A. nodosum seaweed extracts on spinach growth, physiology and nutrition value under drought stress. Sci Hortic. 2015;183:39–47. [Google Scholar]
19. Fan D, Hodges DM, Zhang J, Kirby CW, Ji X, Locke SJ, et al. Commercial extract of the brown seaweed Ascophyllum nodosum enhances phenolic antioxidant content of spinach (Spinacia oleracea L.) which protects Caenorhabditis elegans against oxidative and thermal stress. Food Chem. 2011;124(1):195–202. [Google Scholar]
20. Elansary HO, Skalicka-Wozniak K, King IW. Enhancing stress growth traits as well as phytochemical and antioxidant contents of Spiraea and Pittosporum under seaweed extract treatments. Plant Physiol Biochem. 2016;105:310–20. [Google Scholar] [PubMed]
21. Mutale-Joan C, Redouane B, Najib E, Yassine K, Lyamlouli K, Laila S, et al. Screening of microalgae liquid extracts for their bio stimulant properties on plant growth, nutrient uptake and metabolite profile of Solanum lycopersicum L. Sci Rep. 2020;10(1):2820. [Google Scholar] [PubMed]
22. Taha RS, Alharby HF, Bamagoos AA, Medani RA, Rady MM. Elevating tolerance of drought stress in Ocimum basilicum using pollen grains extract; a natural biostimulants by regulation of plant performance and antioxidant defense system. S Afr J Bot. 2020;128:42–53. [Google Scholar]
23. Vikram P, Chiruvella KK, Ripain IHA, Arifullah M. A recent review on phytochemical constituents and medicinal properties of kesum (Polygonum minus Huds). Asian Pac J Trop Biomed. 2014;4(6):430–5. [Google Scholar] [PubMed]
24. Christapher PV, Parasuraman S, Christina JMA, Asmawi MZ, Vikneswaran M. Review on Polygonum minus. Huds, a commonly used food additive in Southeast Asia. Pharmacogn Res. 2015;7(1):1–6. [Google Scholar]
25. Wang W, Zheng W, Lv H, Liang B, Jin S, Li J, et al. Animal-derived plant biostimulants alleviates drought stress by regulating photosynthesis, osmotic adjustment, and antioxidant systems in tomato plants. Sci Hortic. 2022;305:111365. [Google Scholar]
26. Miliauskas G, Venskutonis PR, Van Beek TA. Screening of radical scavenging activity of some medicinal and aromatic plant extracts. Food Chem. 2004;85(2):231–7. [Google Scholar]
27. Dubois M, Gilles KA, Hamilton JK, Rebers PA, Smith FAJN. A colorimetric method for the determination of sugars. Nat. 1951;168(4265):167. [Google Scholar]
28. Bates LS, Waldren RA, Teare ID. Rapid determination of free proline for water-stress studies. Plant Soil. 1973;39(1):205–7. [Google Scholar]
29. Griffth OW. Determination of glutathione and glutathione disulfide using glutathione reductase and 2-vinylpyridine. Anal Biochem. 1980;106(1):207–12. [Google Scholar] [PubMed]
30. de Vos CHR, Schat HMAM, de Waal MAM, Vooijs R, Ernst WHO. Increased resistance to copper-induced damage of the root cell plasmalemma in copper tolerant Silene cucubalus. Physiol Plant. 1991;82(4):523–8. [Google Scholar]
31. Zafari M, Ebadi A, Jahanbakhsh S, Sedghi M. Safflower (Carthamus tinctorius) biochemical properties, yield, and oil content affected by 24-epibrassinosteroid and genotype under drought stress. J Agric Food Chem. 2020;68(22):6040–7. [Google Scholar] [PubMed]
32. Bradford MM. A rapid and sensitive for the quantitation of microgram quantities of protein utilizing the principle of protein dye binding. Anal Biochem. 1976;72(1–2):248–54. [Google Scholar] [PubMed]
33. Sharma S, Chen C, Khatri K, Rathore MS, Pandey SP. Gracilaria dura extract confers drought tolerance in wheat by modulating abscisic acid homeostasis. Plant Physiol Biochem. 2019;136(3):143–54. [Google Scholar] [PubMed]
34. Anjum SA, Wang L, Farooq M, Xue L, Ali S. Fulvic acid application improves the maize performance under well-watered and drought conditions. J Agron Crop Sci. 2011;197(6):409–17. [Google Scholar]
35. Rady MM, Desoky ESM, Elrys AS, Boghdady MS. Can licorice root extract be used as an effective natural biostimulants for salt-stressed common bean plants? S Afr J Bot. 2019;121:294–305. [Google Scholar]
36. Nayyar H, Gupta D. Differential sensitivity of C3 and C4 plants to water deficit stress: association with oxidative stress and antioxidants. Environ Exp Bot. 2006;58(1–3):106–13. [Google Scholar]
37. Gupta A, Rico-Medina A, Caño-Delgado AI. The physiology of plant responses to drought. Sci. 2020;368(6488):266–9. [Google Scholar]
38. Abo Gamar MI, Kisiala A, Emery RN, Yeung EC, Stone SL, Qaderi MM. Elevated carbon dioxide decreases the adverse effects of higher temperature and drought stress by mitigating oxidative stress and improving water status in Arabidopsis thaliana. Planta. 2019;250:1191–214. [Google Scholar] [PubMed]
39. Ma X, Zheng J, Zhang X, Hu Q, Qian R. Salicylic acid alleviates the adverse effects of salt stress on Dianthus superbus (Caryophyllaceae) by activating photosynthesis, protecting morphological structure, and enhancing the antioxidant system. Front Plant Sci. 2017;8:600. [Google Scholar] [PubMed]
40. Desoky ESM, EI-maghraby LMM, Awad AE, Abdo AI, Rady MM, Semida WM. Fennel and ammi seed extracts modulate antioxidant defence system and alleviate salinity stress in cowpea (Vigna unguiculata). Sci Hortic. 2020;272:109576. [Google Scholar]
41. Rai AC, Singh M, Shah K. Effect of water withdrawal on formation of free radical, proline accumulation and activities of antioxidant enzymes in ZAT12-transformed transgenic tomato plants. Plant Physiol Biochem. 2012;61:108–14. [Google Scholar]
42. Koh YS, Wong SK, Ismail NH, Zengin G, Duangjai A, Saokaew S, et al. Mitigation of environmental stress-impacts in plants: role of sole and combinatory exogenous application of glutathione. Front Plant Sci. 2021;12:791205. [Google Scholar] [PubMed]
43. Razi K, Muneer S. Drought stress-induced physiological mechanisms, signaling pathways. and molecular response of chloroplasts in common vegetable crops. Crit Rev Biotechnol. 2021;41(5):669–91. [Google Scholar] [PubMed]
44. Zayed BA, Salem AKM, El-Sharkawy HM. Effect of different micronutrient treatments on rice (Oriza sativa L.) growth and yield under saline soil conditions. World J Agric Sci. 2011;7(2):179–84. [Google Scholar]
Cite This Article
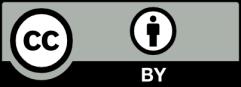