Open Access
ARTICLE
Selenium Differentially Regulates Flavonoid Accumulation and Antioxidant Capacities in Sprouts of Twenty Diverse Mungbean ( (L.) Wilczek) Genotypes
1 Anhui Provincial Engineering Laboratory for Efficient Utilization of Featured Resource Plants, College of Life Sciences, Huaibei Normal University, Huaibei, 235000, China
2 Department of Soil Science, Federal Institute of Piaui, Uruçuí, Piauí, 64860-000, Brazil
3 The Second Affiliated Hospital of Guizhou University of Traditional Chinese Medicine, Guiyang, 550003, China
* Corresponding Authors: Li Xu. Email: ; Yongbo Duan. Email:
Phyton-International Journal of Experimental Botany 2024, 93(3), 611-625. https://doi.org/10.32604/phyton.2024.048295
Received 04 December 2023; Accepted 26 February 2024; Issue published 28 March 2024
Abstract
Seed germination with selenium (Se) is promising for producing Se-biofortified foods. Mungbean (Vigna radiata (L.) Wilczek) sprout is freshly eaten as a salad dressed with sauce, making it superior for Se biofortification. Since the Se safety range for the human body is extremely narrow, it is imperative to evaluate the genotypic responses of mungbean sprouts to Se. This study evaluated the Se enrichment capacity and interaction with flavonoids and antioxidant systems in sprouts of 20 mungbean germplasms. Selenium treatment was done by immersing mungbean seeds in 20 μM sodium selenite solution for 8 h. Afterward, the biomass, Se amounts, flavonoid (particularly vitexin and isovitexin) contents, antioxidant capacity, and key biosynthetic gene expressions were measured. Sprout Se content was 2.0–7.0 μg g−1 DW among the 20 mungbean germplasms. Selenium treatment differentially affected the biomass, total flavonoid, vitexin, isovitexin, antioxidant enzyme activities, and antioxidant capacities of the mungbean germplasms. Eight germplasms showed increased biomass (p < 0.05), the highest increasing by 127%, but 13 did not phenotypically respond to Se treatment. Seven and six germplasms showed varied levels of vitexin and isovitexin increment after Se treatment, the highest measuring 2.67- and 2.87-folds for vitexin and isovitexin, respectively. Two mungbean flavonoid biosynthesis genes, chalcone synthase (VrCHS) and chalcone isomerase (VrCHI) were significantly up-regulated in the germplasms with increased vitexin and isovitexin levels (p < 0.05). Moreover, Se enrichment capacity was significantly correlated with the vitexin, isovitexin, and antioxidant capacities. In conclusion, mungbean sprouts could be a useful Se-biofortified food, but the Se enrichment capacity and nutritional response must be determined for each germplasm before commercialization.
Keywords
Selenium (Se) is a key element for human health [1]. Human Se uptake is mainly through foods [2]. However, considering the decreasing soil Se content, over 10% of the global population would face Se deficiency in foods [3]. Nonetheless, spraying or irrigating external Se solution on plants could facilitate Se enrichment in crop foods [4–6]. Selenium has an extremely narrow safety range, and the recommended daily allowance (RDA) amounts are 60–70 µg/day, where <40 μg/d is a dietary deficiency and >400 μg/d are toxic levels [2,7], indicating the need to know the clear Se contents in biofortified foods.
Germinating seeds with an external Se solution provides a simple and efficient approach for the Se-enrichment of sprout-eatable crops, allowing the year-round production of Se-biofortified foods [8,9]. In soybean, most inorganic Se could be transformed into organic species within 24 h of germination [9]. Mungbean [Vigna radiata (L.) Wilczek] has a global plantation acreage of 6 million hectares [10]. The protein content of mungbean kernels is 20.97%–31.32%, substantially higher than that of other crops like soybean and corn [11]. The high protein content allows plants to absorb and metabolize more Se to form seleno-proteins, conferring a greater potential for Se enrichment of mungbean. Moreover, mungbean sprout is a popular fresh salad vegetable that retains most nutrients.
Mungbean can transform inorganic Se to the organic form during seed germination or foliar application [12–14]. A recent transcriptomic analysis of Se uptake mechanisms in mungbean sprouts identified transporters for Pi, sulfate, and nitrate [14], indicating that Se may share the transporters with these elements. Wang et al. [15] used foliar application of Se solution to identify three primary metabolites as biomarkers for nutritional evaluation. However, the interaction between Se enrichment capacity and the important secondary metabolites, particularly the major flavonoids vitexin and isovitexin [16], in mungbean remains unclear.
Previous studies reported that Se regulates flavonoid metabolism, antioxidant enzyme activities, and antioxidant capacities in plants [2,4,9,17]. However, the conclusions are difficult to compare and often contradictory because the datasets were generated from different plant species, growth stages, growing substrates, Se treatment methods, Se forms, and concentrations. Thus, single variable experiments, such as the genotypic response of the same plant species to Se treatment, should be conducted to provide substantial supporting data to draw an unambiguous conclusion.
In this study, twenty mungbean germplasms were employed to investigate the genotypic variations in Se enrichment capacity and respective biochemical responses. The Se enrichment capacity, phenotypic and antioxidant indices, and flavonoid (particularly vitexin and isovitexin) content were measured. Further, the flavonoid biosynthesis gene profiles were investigated to uncover the possible mechanism controlling flavonoid metabolism in diverse Se-treated mungbean germplasms.
2.1 Materials and Experimental Design
Twenty sprout-specialized germplasms of mungbean (Pinlv 211-6, Pinlv 2011-12, Jilv 0816, Jilv HNZ0810, Sulv 16-10, Sulv 15-11, Elv 5, Bailv 9, Baolv 200810-1, Bailv 10, Tong 1188326, Liaolv 10L708-5, 122-225, Baolv 201012-7, 1009-2-5, 142-139, Kelv 2, Weilv 11, Weilv 12, and Wanlv 2) were provided by Dr. Bin Zhou at the Crop Research Institute, Anhui Academy of Agricultural Sciences, China.
Sodium selenite (Na2SeO3, 99%) was obtained from Sigma-Aldrich (MO, USA), and standard Se solution (GSB 07-1253-2000) was purchased from the Institute for Reference Materials, Ministry of Environmental Protection (Beijing, China). The water used was deionized water (18.2 MΩ.cm, Millipore).
The culture was prepared following a previously published method [18]. For each germplasm, 10 g of cleaned and healthy mungbean seeds were disinfected with sodium hypochlorite solution (available chlorine, 2.5%) for 5 min and rinsed thrice using deionized water. Se treatment was conducted by immersing the disinfected seeds in 80 mL of 20 μM sodium selenite, which was reported to provide the highest ratio of organic form to total Se content as reported previously [14,19]. The seeds soaked in an equal volume of deionized water were used as control (CK). After the solution was completely absorbed (8 h), the seeds were evenly dispersed into plastic containers. The culture was performed in darkness at 25°C, 80% relative humidity, and sprayed with 15 mL of ddH2O every 6 h for adequate moisture. The uniform-sized sprouts for each treatment were collected four days later and weighed to record the weight. Some were frozen and stored at −80°C, and the others were freeze-dried and ground into powder.
Here, 100 mg of sprout powder was used to extract flavonoids using 1 mL of 80% methanol. The mixtures were violently shaken for 10 min and centrifuged to collect the supernatants. The pellets were re-extracted in 1 mL of 80% methanol. The supernatants were combined as the extracts for further analysis.
The total flavonoids were measured as reported [20] with some modifications. Briefly, 100 μL of extracts were added with 30 μL of 5% NaNO2 and 450 μL of ddH2O. Six minutes later, 30 μL of 10% AlCl3 was added to the mixture and vortexed well, and the mixture was left to stand for 5 min. Then 100 μL of 1 M NaOH was added to terminate the reaction, and A510 was determined accordingly. The total flavonoid content was obtained based on the standard curve of rutin (0, 0.2, 0.4, 0.6, 0.8, 1.0, and 1.2 mg mL−1). The content was denoted as mg rutin equivalent per 1 g sample (mg RE g−1).
Vitexin and isovitexin were quantified with an HPLC system provided with a C-18 column (4.6 mm × 250 mm, Agilent Technologies Inc., CA, USA) [16]. UV wavelength was 360 nm, and the deionized water with 0.2% acetic acid was solvent A, while acetonitrile was solvent B. The elution was initiated with 5% B, linear gradient to 50% B within 25 min, and 50%–85% B to 30 min. The flow rate was set at 1.0 mL/min, and the vitexin and isovitexin retention times were 13.91 and 14.62 min, respectively, compared to the corresponding standard. Vitexin or isovitexin peak volumes were quantified against the standard curves.
2.3 Antioxidant Enzyme Activity and Antioxidant Capacity Assays
Plant extracts were prepared from 100 mg of fresh sprout powder. The powder was dissolved in 1 mL of ice-cold extraction buffer (50 mM of phosphate-buffered saline (PBS) (pH 7.8), 0.2 mM of ethylenediaminetetraacetic acid (EDTA), and 0.3% (w/v) of Triton X-100). The reaction mixture was shaken violently, incubated on ice for 5 min, and centrifuged. The protein was measured following the Bradford method [21].
The antioxidant enzyme activities were measured as reported previously [22]. Briefly, the ascorbate peroxidase (APX) activity was deduced from the amount of reduced ascorbate (A290) in reaction solutions of PBS, reduced ascorbate, H2O2, and 100 μL of plant extract. Catalase (CAT) activity was detected via monitoring the H2O2 (A240) consumption rate in solutions containing 50 mM of PBS (pH 7.6), 0.1 mM of EDTA, 100 mM of H2O2, and 100 μL of plant extract. Additionally, the superoxide dismutase (SOD) was quantified via the ability of the plant extract to inhibit the generation of formazan (A560) caused by O2·−.
Two methods, including 2,2-diphenyl-1-picrylhydrazyl (DPPH) and ferric reducing/antioxidant power (FRAP), were employed to determine the antioxidant capacity [23]. Essentially, the DPPH method was done by detecting the ability of the extract to catalyze the DPPH oxidative reaction. The DPPH antioxidant capacity result was presented as mg ascorbic acid equivalent antioxidant capacity per 1 g sample (mg AEAC g−1). The FRAP assay was deduced from the ability of ferric to reduce the extract against a Fe (II) calibration curve, and the results were denoted as μM Fe2+ g−1.
2.4 RNA Isolation, cDNA Synthesis, and Realtime Fluorescence Quantitative PCR
Total RNA isolation was done using a Plant RNA Extraction Kit (TaKaRa, Kusatsu, Japan) [18], and cDNA was synthesized with the PrimeScript cDNA Synthesis Kit (TaKaRa). The PCR was done on the ABI7500 fast Real-Time PCR system (Applied Biosystems, MA, USA) with a 2 × AceQ qPCR SYBR Green Master Mix (Vazyme, NJ, USA). VrActin was used as the reference gene, and the analyses involved three biological and three technical replicates per gene. Relative gene expression was calculated using the 2−ΔΔCT method [24]. The primer sequences are listed in Table S1.
2.5 Determining Se Content Using Atomic Fluorescence Spectroscopy
Selenium accumulation in mungbean sprouts was measured following previously published methods [25,26]. For each sample, 1 g of the dried powder was acid-digested and analyzed using a double-channel atomic fluorescence spectrometer (Jinsuokun SK-2002AZ, Beijing, China). Then, Se content was calculated based on the fluorescence intensity of the extract against the calibration curve of a commercial Se standard.
The data is presented as the means ± SD of three biological replicates per sample. IBM SPSS Statistics 20.0 with Duncan’s multiple-range test (p < 0.05) was used to determine the difference between the CK (germinated normally without Se treatment) and Se treatment for each germplasm. Heatmaps generated using the “corrplot” in R Software Package (version 4.2.2) show the Pearson correlations between the parameters (p < 0.05). The “corr” and “cor. mtest” functions of the “corrplot” package were used to establish the coefficient and p-value matrices, respectively. Finally, the analysis of variance (ANOVA) was employed to determine the statistically significant correlations represented by the asterisks in the heatmaps.
3.1 Mungbean Germplasms Display Differential Phenotypic Response to Se
Under normal growth conditions, the germplasms varied in growth vigor. The biomass was 0.13–0.37 g/plant (Fig. 1A) four days after germination, and the highest was 142-139. Biomass is key for evaluating the genetic diversity of crops [27] and is usually included for screening diverse germplasm collections [28]. In this study, the difference in biomass between the highest and lowest germplasm reached three-fold, suggesting that the mungbean germplasms tested are diverse.
Figure 1: The biomass (A) and phenotypes (B) of mungbean sprouts treated with 20 μM of Na2SeO3 (right) and without Na2SeO3 (left) at four days after germination. The error bars represent the standard error of the mean (n = 20). Bar, 1 cm. CK, sprouts germinated normally without Se treatment. Se, sprouts germinated with 20 μM Se. Values marked by asterisks significantly differ between CK and Se-treated samples, respectively (p < 0.05). 1, Pinlv 211-6; 2, Pinlv 2011-12; 3, Jilv 0816; 4, Jilv HNZ0810; 5, Sulv 16-10; 6, Sulv 15-11; 7, Elv 5; 8, Bailv 9; 9, Baolv 200810-1; 10, Bailv 10; 11, Tong 1188326; 12, Liaolv 10L708-5; 13, 122-225; 14, Baolv 201012-7; 15, 1009-2-5; 16, 142-139; 17, Kelv 2; 18, Weilv 11; 19, Weilv 12; 20, Wanlv 2
Selenium (20 μM) significantly increased the biomass of eight germplasms (p < 0.05), decreased one (Sulv 16-10), and showed a non-significant change on the others (Fig. 1A). Wanlv2 showed the highest Se-driven increment (127%, p < 0.05). Selenium promotes growth in different plant species [29,30]. However, the germplasms in this study showed significantly different phenotypic responses to Se (Figs. 1A, 1B). The possible reason is that the previous studies tested only one or two germplasms, while this study evaluated genotypic response using a diverse collection of 20 germplasms. Furthermore, just 20 μM Se was used in our study, the proper Se concentration may vary among different mungbean germplams.
3.2 Mungbean Germplasms Vary in Se Accumulation during Seed Germination
External Se treatment significantly enhanced Se accumulation in mungbean, reaching 2.0–7.0 μg g−1 DW (Fig. 2). The highest Se content was observed in 122–225, and the lowest was in Kelv 2. These values were 118–210-fold higher than those in mungbean germinated without external Se (0.014–0.035 μg g−1 DW) (p < 0.05), indicating that mungbean efficiently accumulates Se during germination, as previously reported [12,13]. Different plant species are known to vary in abilities to metabolize Se [31,32]. This study further demonstrates a >3-fold genotypic difference in the Se enrichment capacity of mungbean. The difference in Se enrichment may correlate with the differential expression of SeO32− transporter Pht1 (phosphate transporter 1) and aquaporins since Pht1 consumed SeO32− via aquaporins [33]. These results indicate the feasibility of supplementing external Se for mungbean sprout biofortification, but the genotypic variation should be noted. However, we just measured the total Se content, the Se speciation in different mungbean germplasms deserves further study.
Figure 2: Selenium accumulation in mungbean sprouts with or without 20 μM Na2SeO3. The data were collected four days after germination. Error bars represent the standard error of the mean (n = 3). CK, sprouts germinated normally without Se treatment. Se, sprouts germinated with 20 μM Se. 1, Pinlv 211-6; 2, Pinlv 2011-12; 3, Jilv 0816; 4, Jilv HNZ0810; 5, Sulv 16-10; 6, Sulv 15-11; 7, Elv 5; 8, Bailv 9; 9, Baolv 200810-1; 10, Bailv 10; 11, Tong 1188326; 12, Liaolv 10L708-5; 13, 122-225; 14, Baolv 201012-7; 15, 1009-2-5; 16, 142-139; 17, Kelv 2; 18, Weilv 11; 19, Weilv 12; 20, Wanlv 2. Values marked by asterisks significantly differ between CK and Se-treated samples, respectively (p < 0.05)
Recent research indicated that the organic to total Se ratio increases to 90% on the 4th day of mungbean seed germination [14]. Accordingly, we harvested mungbean sprouts on the 4th day for Se accumulation and nutritional analysis. The RDA for Se (60–70 µg/d) [2] indicates that daily consumption of approximately 210–735 g fresh mungbean sprout could supplement enough Se.
3.3 Selenium Treatment Increases Mungbean Flavonoids
The total flavonoid content was 3.85–7.14 mg RE g−1 DW under normal conditions. There is a 1.85-fold discrepancy between the highest and the lowest values (Fig. 3A). Treatment with 20 μM of Na2SeO3 significantly enhanced the flavonoids in Sulv 16-10, Bailv 9, 122-225 and 1009-2-5 (p < 0.05), but not other germplasms. The highest increment was 2.2 folds in 122-225.
Figure 3: Total flavonoids (A), vitexin (B), and isovitexin (C) contents of mungbean treated with or without 20 μM Na2SeO3. The data were collected four days after germination. Error bars represent the standard error of the mean (n = 3). CK, sprouts germinated normally without Se treatment. Se, sprouts germinated with 20 μM Se. Values marked by asterisks significantly differ between CK and Se-treated samples, respectively (p < 0.05). 1, Pinlv 211-6; 2, Pinlv 2011-12; 3, Jilv 0816; 4, Jilv HNZ0810; 5, Sulv 16-10; 6, Sulv 15-11; 7, Elv 5; 8, Bailv 9; 9, Baolv 200810-1; 10, Bailv 10; 11, Tong 1188326; 12, Liaolv 10L708-5; 13, 122-225; 14, Baolv 201012-7; 15, 1009-2-5; 16, 142-139; 17, Kelv 2; 18, Weilv 11; 19, Weilv 12; 20, Wanlv 2
The major flavonoids, vitexin, and isovitexin, were quantified via HPLC (Fig. S1). The vitexin content was 0.48–1.20 mg g−1 DW in sprouts of 20 mungbean germplasms germinated normally (Fig. 3B). Selenium treatment differentially influenced vitexin metabolism among germplasms, which increased vitexin accumulation by 1.43, 2.67, 1.79, and 1.76 folds respectively in Bailv 9, 122-225, 1009-2-5 and Weilv 12 but decreased it in Pinlv2011-12 and Jilv 0816 (p < 0.05). The isovitexin content was 0.68–2.07 mg g−1 DW in mungbean germplasms without Se treatment (Fig. 3C), with a 3-fold difference between the highest and the lowest values. Furthermore, Na2SeO3 treatment significantly increased the isovitexin content of Jilv 0816, Sulv 16-10, Tong 1188326, 122-225, Weilv 11, and Weilv 12 (p < 0.05), with a 2.87-fold increase in 122-225. Comprehensively considering the three indicators, Sulv 16-10, Bailv 9, 122-225, and 1009-2-5 are potential candidates for Se-biofortification and flavonoid enrichment.
Vitexin and isovitexin have important pharmacological activities against various disorders, including diabetes [34], endometrial cancer [35], and renal injury [36]. However, vitexin and isovitexin, the major flavonoids of mungbean [37,38], decrease during germination [16,39]. To our knowledge, this is the first time to report that Se enrichment could regulate the accumulation of vitexin and isovitexin in plants. Moreover, the 20 mungbean germplasms demonstrated differential flavonoid accumulation under Se treatment (Fig. 3). These results amend previous reports that Se increases flavonols in soybean sprouts [40] and isoflavonols in chickpea sprouts [41] via germination. The difference is possibly attributed to the experimental materials since just one or two germplasms were used in those studies while 20 diverse germplasm collections were investigated here.
3.4 Se Influences Antioxidant Capacities in Mungbean
Three antioxidant enzymes varied in activity among germplasms (Fig. 4). Under normal conditions, the genotypic differences between the highest and the lowest germplasms were 2.0-, 2.2-, and 1.9-fold for SOD, CAT, and APX activities, respectively.
Figure 4: The activities of antioxidant enzymes: SOD (A), CAT (B), APX (C) in treated (20 μM Na2SeO3) and untreated (CK) mungbean four days after germination. Error bars represent the standard error of the mean (n = 3). CK, sprouts germinated normally without Se treatment. Se, sprouts germinated with 20 μM Se. Values marked by asterisks significantly differ between CK and Se-treated samples, respectively (p < 0.05). 1, Pinlv 211-6; 2, Pinlv 2011-12; 3, Jilv 0816; 4, Jilv HNZ0810; 5, Sulv 16-10; 6, Sulv 15-11; 7, Elv 5; 8, Bailv 9; 9, Baolv 200810-1; 10, Bailv 10; 11, Tong 1188326; 12, Liaolv 10L708-5; 13, 122-225; 14, Baolv 201012-7; 15, 1009-2-5; 16, 142-139; 17, Kelv 2; 18, Weilv 11; 19, Weilv 12; 20, Wanlv 2
When germinated with Se, 20 germplasms presented different responses in antioxidant enzymes (Fig. 4). Se treatment decreased SOD activity in Sulv 15-11 and Weilv 12 but increased it in 7 germplasms (p < 0.05), i.e., Pinlv 211-6, Pinlv 2011-12, Bailv 9, 122-225, Baolv201012-7 and Wanlv 2. CAT activity was boosted in Bailv 9, 122-225, 1009-2-2, and Wanlv2, while it was decreased in other 4 germplasms when treated with Se (p < 0.05). For APX, 5 germplasms, i.e., Sulv 16-10, Bailv 9, 122-225, 1009-2-5, and Wanlv 2 showed increased activities, while Tong 1188326 presented a decreased activity.
Only two germplasms (122-225 and Wanlv 2) had significantly increased activities of three antioxidant enzymes when treated with Se. Six germplasms including Jilv 0816, Elv 5, Baolv 200810-1, 142-139, Kelv 2, and Weilv 11 did not show significant changes in all three antioxidant enzymes. These results suggest that there are genotypic variations in antioxidant enzyme activities, though Se treatment may fortify the mungbean antioxidant system.
The antioxidant capacities (evaluated using the FRAP and DPPH methods) varied among mungbean germplasms in response to Se treatment (Fig. 5). Five germplasms, i.e., Sulv 16-10, Bailv 9, 122-225, 1009-2-5, and Wanlv 2, presented significantly increased FRAP and DPPH values after Na2SeO3 treatment (p < 0.05). The other germplasms gave increased values in one indicator or insignificant changes in both FRAP and DPPH.
Figure 5: Antioxidant capacities: FRAP (A), DPPH (B) of mungbean treated with or without 20 μM Na2SeO3. The data were collected four days after germination. Error bars represent the standard error of the mean (n = 3). CK, sprouts germinated normally without Se treatment. Se, sprouts germinated with 20 μM Se. Values marked by asterisks significantly differ between CK and Se-treated samples, respectively (p < 0.05). 1, Pinlv 211-6; 2, Pinlv 2011-12; 3, Jilv 0816; 4, Jilv HNZ0810; 5, Sulv 16-10; 6, Sulv 15-11; 7, Elv 5; 8, Bailv 9; 9, Baolv 200810-1; 10, Bailv 10; 11, Tong 1188326; 12, Liaolv 10L708-5; 13, 122-225; 14, Baolv 201012-7; 15, 1009-2-5; 16, 142-139; 17, Kelv 2; 18, Weilv 11; 19, Weilv 12; 20, Wanlv 2
Selenium has been reported to elicit the physiological, biochemical, and antioxidant defense systems of plants [42]. Comparing the Se-accumulator and non-accumulator, the higher the biochemical Se tolerance in plants, the higher the antioxidant enzymes and more non-enzymatic substances are, confirming that the Se accumulator had a higher antioxidant capacity [19]. However, this conclusion is based on different plant species, our results further extended the inter-specific characteristic to intra-specific in mungbean.
3.5 Se-Promoted Flavonoid Accumulation Is Associated with Flavonoid Biosynthesis Genes
We examined the expression of synthase genes (i.e., VrCHS, VrCHI, VrF3H, and VrFLS) from the biosynthetic pathway to understand flavonoid accumulation after Se treatment. Two high (Bailv 9 and 122-225) and two low (Pinlv 2011-12 and Baolv 200810-1) Se response germplasms were chosen for gene expression analysis (Fig. 6A). VrCHS and VrCHI were significantly up-regulated (>4 fold) in Bailv 9 and 122-225 (p < 0.05). In contrast, their expression was not significantly different in Pinlv 2011-12 and Baolv 200810-1 (p > 0.05). Chalcone synthase (CHS) and chalcone isomerase (CHI) are key synthases for flavonoid biosynthesis (Fig. 6B), and their expressions were consistent with flavonoid accumulation (Fig. 6C). Unlike VrCHS and VrCHI, the other two flavonoid biosynthesis genes VrF3H and VrFLS did not present an expression profile consistent with flavonoid accumulation pattern in mungbean sprout. The F3H gene was up-regulated in Pinlv 2011-12 but down-regulated in Bailv 9, Baolv 200810-1, and 122-225, while the FLS gene was up-regulated in all four germplasms. These results demonstrate that the Se-activated flavonoid is probably attributed to CHS and CHI, but not F3H and FLS, in mungbean sprouts.
Figure 6: Relative expression of genes from the flavonoid biosynthetic pathway. (A) mRNA transcripts of biosynthetic genes. The qRT-PCR techniques quantified transcripts from mungbean germplasm with a high and low response to Se treatment (20 μM Na2SeO3). Non-treated Pinlv 2011-12 samples were considered as the reference calculating the relative gene expression level of other samples, and the data are averages of four technical and three biological replicates. CK, sprouts germinated normally without Se treatment. Se, sprouts germinated with 20 μM Se. Values marked with asterisks significantly differ between plants with high and low Se levels, treated and non-treated samples, respectively (p < 0.05; n = 3). (B) The flavonoid biosynthesis pathway in plants. (C) Fold change of each gene with or without Se treatment. The expression of each gene in Pinlv 2011-12 without Se treatment was conversed to 1 for comparison. 2, Pinlv 2011-12; 8, Bailv 9; 9, Baolv 200810-1; 13, 122-225
In plants, flavonoid biosynthesis is well understood, though the genes catalyzing naringenin into vitexin/isovitexin are unclear [43]. Two key biosynthetic genes (CHI and CHS) were up-regulated in two high- but not in two low-response germplasms. The up-regulation of upstream flavonoid synthases [44], CHI, and CHS by Se treatment augmented the precursor influx into flavonoid metabolism. However, correlation analysis indicated that Se enrichment capacity significantly correlates with vitexin and isovitexin but not with total flavonoids. These results are possibly explained by the unknown genes that catalyze naringenin into vitexin/isovitexin, probably up-regulating vitexin/isovitexin. Genotypically, the varied flavonoids in Se-germinated mungbean are probably correlated with the regulatory elements in CHI and CHS promoters of different germplasms, but this perception needs further examination.
3.6 Se Enrichment Capacity Is Correlated with Vitexin and Isovitexin and Antioxidant-Related Attributes
Fig. 7A shows the correlations between Se enrichment capacity and vitexin, isovitexin, and other examined parameters. Selenium content was positively correlated with biomass, vitexin, and isovitexin, and negatively correlated with APX (p < 0.05), indicating that Se influenced their accumulations in mungbean. The total flavonoids were positively correlated with DPPH and FRAP. These results suggest that Se enrichment promotes the increase of biomass, vitexin, and isovitexin in mungbean sprouts. However, Se enrichment is not significantly correlated with total flavonoids (Fig. 7A). Previous studies have reported that flavonoids could be turned into other non-flavonoid compounds, such as stilbenes and bibenzyls [45], which may explain the poor correlation between total flavonoids and Se enrichment capacity.
Figure 7: The heatmap of the Pearson correlation coefficients generated from the variables of 20 mungbean germplasms germinated with 20 μM Na2SeO3. (A) Correlation between parameters analyzed in mungbean plants. * indicates a significant correlation (p < 0.05). (B) PCA biplot showing each genotype score (points) and group (circles). 1, Pinlv 211-6; 2, Pinlv 2011-12; 3, Jilv 0816; 4, Jilv HNZ0810; 5, Sulv 16-10; 6, Sulv 15-11; 7, Elv 5; 8, Bailv 9; 9, Baolv 200810-1; 10, Bailv 10; 11, Tong 1188326; 12, Liaolv 10L708-5; 13, 122-225; 14, Baolv 201012-7; 15, 1009-2-5; 16, 142-139; 17, Kelv 2; 18, Weilv 11; 18, Weilv 12; 20, Wanlv 2
Principal component analysis (PCA) is an efficient tool that can simplify the information of observations from several inter-correlated quantitative dependent variables [46]. It was used to describe and elucidate the sources of variance among mungbean genotypes (Fig. 7B and Table S2). In this study, PC1 and PC2 explained 29.2% and 19.5% of the total variations (Fig. 7B), mainly representing Se enrichment capacity, vitexin, isovitexin, and antioxidant-related indicators (Table S2). The results indicated that the Se treatment mainly influences flavonoid metabolisms, antioxidant enzymes and antioxidant capacities, implying that these parameters need to be investigated for each mungbean germplasm for Se biofortification.
This study reports the differential responses of mungbean germplasms to Se treatment during germination. The information enlightens that Se enrichment could improve the flavonoid metabolism and antioxidant system in mungbean during germination, but the detailed response of each mungbean germplasm to Se should be clarified before commercial application.
Acknowledgement: None.
Funding Statement: This study was supported by the Key Project of Natural Science Research for Colleges and Universities in Anhui Province (KJ2021A0533; 2023AH050345), and the Excellent Scientific Research and Innovation Team of Universities in Anhui Province (2022AH010029).
Author Contributions: The authors confirm their contribution to the paper as follows: study conception and design: Fenglan Zhao, Yongbo Duan, Li Xu; data collection: Fenglan Zhao, Jizhi Jin, Meng Yang; analysis and interpretation of results: Fenglan Zhao, Franklin Eduardo Melo Santiago, Jianping Xue, Li Xu; draft manuscript preparation: Fenglan Zhao, Li Xu, Yongbo Duan; funding acquisition: Fenglan Zhao, Jianping Xue, Yongbo Duan. All authors have reviewed the results and approved the final version of the manuscript for submission.
Availability of Data and Materials: The data that support the findings of this study are available on request from the corresponding author upon reasonable request.
Ethics Approval: Not applicable.
Conflicts of Interest: The authors declare that they, as well as the Second Affiliated Hospital of Guizhou University of Traditional Chinese Medicine have no conflict of interest to report regarding the present study.
References
1. Genchi G, Lauria G, Catalano A, Sinicropi MS, Carocci A. Biological activity of selenium and its impact on human health. Int J Mol Sci. 2023;24(3):2633. [Google Scholar] [PubMed]
2. Duborská E, Šebesta M, Matulová M, Zverina O, Urík M. Current strategies for selenium and iodine biofortification in crop plants. Nutr. 2022;14(22):4717. [Google Scholar]
3. Jones GD, Droz B, Greve P, Gottschalk P, Poffet D, McGrath SP, et al. Selenium deficiency risk predicted to increase under future climate change. Proc Natl Acad Sci USA. 2017;114(11):2848–53. [Google Scholar] [PubMed]
4. Pilon-Smits EAH. On the ecology of selenium accumulation in plants. Plants. 2019;8(7):197. [Google Scholar] [PubMed]
5. Sun MF, Hui XR, Tong CL, Yuan LY, Zhang DJ. The physiological and molecular responses of exogenous selenium to selenium content and fruit quality in walnut. Phyton. 2023;92(3):851–60. [Google Scholar]
6. Tangjaidee P, Swedlund P, Xiang JQ, Yin HQ, Quek SY. Selenium-enriched plant foods: selenium accumulation, speciation, and health functionality. Front Nutr. 2023;9:962312. [Google Scholar] [PubMed]
7. D’Amato R, Regni L, Falcinelli B, Mattioli S, Benincasa P, Dal Bosco A, et al. Current knowledge on selenium biofortification to improve the nutraceutical profile of food: a comprehensive review. J Agric Food Chem. 2020;68:4075–97. [Google Scholar] [PubMed]
8. Deng B, Tian S, Li S, Guo M, Liu H, Li Y, et al. A simple, rapid and efficient method for essential element supplementation based on seed germination. Food Chem. 2020;325:126827. [Google Scholar] [PubMed]
9. Huang Y, Lei N, Xiong Y, Liu Y, Tong L, Wang F, et al. Influence of selenium biofortification of soybeans on speciation and transformation during seed germination and sprouts quality. Foods. 2022;11:1200. [Google Scholar] [PubMed]
10. Hou D, Yousaf L, Xue Y, Hu J, Wu J, Hu X, et al. Mung bean (Vigna radiata L.bioactive polyphenols, polysaccharides, peptides, and health benefits. Nutr. 2019;11:1238. [Google Scholar]
11. Hadidi M, Jafarzadeh S, Ibarz A. Modified mung bean protein: optimization of microwave-assisted phosphorylation and its functional and structural characterizations. LWT. 2021;151:112119. [Google Scholar]
12. Tie M, Gao Y, Xue Y, Zhang A, Yao Y, Sun J, et al. Determination of selenium species and analysis of methyl-seleno-l-cysteine in Se-enriched mung bean sprouts by HPLC-MS. Anal Methods. 2016;8:3102–8. [Google Scholar]
13. Cao L, Zhu J, Li N. Selenium-agarose hybrid hydrogel as a recyclable natural substrate for selenium-enriched cultivation of mung bean sprouts. Int J Biol Macromol. 2022;194:17–23. [Google Scholar] [PubMed]
14. Cheng H, Li L, Dong JZ, Wang SY, Wu S, Rao S, et al. Transcriptome and physiological determination reveal the effects of selenite on the growth and selenium metabolism in mung bean sprouts. Food Res Int. 2023;169:112880. [Google Scholar] [PubMed]
15. Wang K, Yuan Y, Luo X, Shen Z, Huang Y, Zhou H, et al. Effects of exogenous selenium application on nutritional quality and metabolomic characteristics of mung bean (Vigna radiata L.). Front Plant Sci. 2022;13:961447. [Google Scholar] [PubMed]
16. Yao Y, Cheng XZ, Ren GX. Contents of D-chiro-inositol, vitexin, and isovitexin in various varieties of mung bean and its products. J Integr Agri. 2011;10:1710–5. [Google Scholar]
17. Skrypnik L, Feduraev P, Golovin A, Maslennikov P, Styran T. The integral boosting effect of selenium on the secondary metabolism of higher plants. Plants. 2022;11:3432. [Google Scholar] [PubMed]
18. Zhou T, Wang P, Gu Z, Ma M, Yang R. Spermidine improves antioxidant activity and energy metabolism in mung bean sprouts. Food Chem. 2020;309:125759. [Google Scholar] [PubMed]
19. Santiago FEM, Silva MLDS, Cardoso AADS, Duan YB, Guilherme LRG, Liu JP, et al. Biochemical basis of differential selenium tolerance in arugula (Eruca sativa Mill.) and lettuce (Lactuca sativa L.). Plant Physiol Biochem. 2020;157:328–38. [Google Scholar] [PubMed]
20. Yang J, Shi W, Li B, Bai Y, Hou Z. Preharvest and postharvest UV radiation affected flavonoid metabolism and antioxidant capacity differently in developing blueberries (Vaccinium corymbosum L.). Food Chem. 2019;301:125248. [Google Scholar] [PubMed]
21. Bradford M. A rapid and sensitive method for the quantitation of microgram quantities of protein utilizing the principle of protein-dye binding. Anal Biochem. 1976;2:248–54. [Google Scholar]
22. Kim DK, Jeong SC, Gorinstein S, Chon SU. Total polyphenols, antioxidant and antiproliferative activities of different extracts in mungbean seeds and sprouts. Plant Food Hum Nutr. 2012;67:71–5. [Google Scholar] [PubMed]
23. Paula LC, Lemes AC, Valencia-Mejía E, Moreira BR, Oliveira TS, Campos ITN, et al. Effect of extrusion and autoclaving on the biological potential of proteins and naturally-occurring peptides from common beans: antioxidant and vasorelaxant properties. Food Chem X. 2022;13:100259. [Google Scholar] [PubMed]
24. Pfaffl MW. A new mathematical model for relative quantification in real-time RT-PCR. Nucleic Acids Res. 2001;29:9. [Google Scholar]
25. Deng X, Li R, Deng S. Determination of the total content of arsenic, antimony, selenium and mercury in Chinese herbal food by chemical vapor generation-four-channel non-dispersive atomic fluorescence spectrometry. J Fluoresc. 2020;30:949–54. [Google Scholar] [PubMed]
26. Zagrodzki P, Pasko P, Galanty A, Tyszka-Czochara M, Wietecha-Posluszny R, Rubio PS, et al. Does selenium fortification of kale and Kohlrabi sprouts change significantly their biochemical and cytotoxic properties? J Trace Elem Med Bio. 2020;59:126466. [Google Scholar] [PubMed]
27. Springborn MR, Faig A, Dedrick A, Baskett ML. Beyond biomass: valuing genetic diversity in natural resource management. Am J Agr Econ. 2020;102:607–24. [Google Scholar]
28. Duan Y, Santiago FEM, Reis ARD, de Figueiredo MA, Zhou SP, Thannhauser TW, et al. Genotypic variation of flavonols and antioxidant capacity in broccoli. Food Chem. 2021;338:127997. [Google Scholar] [PubMed]
29. Boldrin PF, de Figueiredo MA, Yang Y, Luo HM, Giri S, Hart JJ, et al. Selenium promotes sulfur accumulation and plant growth in wheat (Triticum aestivum). Physiol Plantarum. 2016;158:80–91. [Google Scholar]
30. Araujo MAD, Melo AARD, Silva VM, Reis ARD. Selenium enhances ROS scavenging systems and sugar metabolism increasing growth of sugarcane plants. Plant Physiol Biochem. 2023;201:107798. [Google Scholar] [PubMed]
31. Trippe RCIII, Pilon-Smits EAH. Selenium transport and metabolism in plants: phytoremediation and biofortification implications. J Hazard Mater. 2021;404:124178. [Google Scholar] [PubMed]
32. Bañuelos GS, Freeman JL, Arroyo IS. Selenium content and speciation differences in selenium enriched soups made from selenium biofortified plants. J Food Compos Anal. 2022;105:104255. [Google Scholar]
33. Li HF, McGrath SP, Zhao FJ. Selenium uptake, translocation and speciation in wheat supplied with selenate or selenite. New Phytol. 2008;178:92–102. [Google Scholar] [PubMed]
34. Tao MF, Li R, Zhang Z, Wu T, Xu TT, Zogona D, et al. Vitexin and isovitexin act through inhibition of insulin receptor to promote longevity and fitness in Caenorhabditis elegans. Mol Nutr Food Res. 2022;66:e2100845. [Google Scholar] [PubMed]
35. Liang CX, Jiang YJ, Sun LZ. Vitexin suppresses the proliferation, angiogenesis and stemness of endometrial cancer through the PI3K/AKT pathway. Pharm Biol. 2023;61:581–9. [Google Scholar] [PubMed]
36. Tseng CY, Yu PR, Hsu CC, Lin HH, Chen JH. The effect of isovitexin on lipopolysaccharide-induced renal injury and inflammation by induction of protective autophagy. Food Chem Toxicol. 2023;172:113581. [Google Scholar] [PubMed]
37. Li H, Cao D, Yi J, Cao J, Jiang W. Identification of the flavonoids in mungbean (Phaseolus radiatus L.) soup and their antioxidant activities. Food Chem. 2012;135:2942–6. [Google Scholar] [PubMed]
38. Tang D, Dong Y, Ren H, Li L, He C. A review of phytochemistry, metabolite changes, and medicinal uses of the common food mung bean and its sprouts (Vigna radiata). Chem Cent J. 2014;8:4. [Google Scholar] [PubMed]
39. Zhang AW, Fu LX, Zuo F, Qian LL. HPLC analysis of vitexin and isovitexin content changes during mung bean germination. J Food Meas Charac. 2022;16:3302–9. [Google Scholar]
40. Lazo-Vélez MA, Guardado-Félix D, Avilés-González J, Romo-López I, Sergio O, Serna-Saldívar SO. Effect of germination with sodium selenite on the isoflavones and cellular antioxidant activity of soybean (Glycine max). LWT. 2018;93:64–70. [Google Scholar]
41. Guardado-Félix D, Serna-Saldivar SO, Cuevas-Rodriguez EO, Jacobo-Velazquez DA, Gutierrez-Uribe JA. Effect of sodium selenite on isoflavonoid contents and antioxidant capacity of chickpea (Cicer arietinum L.) sprouts. Food Chem. 2017;226:69–74. [Google Scholar]
42. Ikram M, Raja NI, Mashwani ZUR, Omar AA, Mohamed AH, Satti SH, et al. Phytogenic selenium nanoparticles elicited the physiological, biochemical, and antioxidant defense system amelioration of Huanglongbing-infected ‘Kinnow’ mandarin plants. Nanomaterials. 2017;12:356. [Google Scholar]
43. Wen W, Alseekh S, Fernie AR. Conservation and diversification of flavonoid metabolism in the plant kingdom. Curr Opin Plant Biol. 2020;55:100–8. [Google Scholar] [PubMed]
44. Shen N, Wang TF, Gan Q, Liu SA, Wang L, Jin B. Plant flavonoids: classification, distribution, biosynthesis, and antioxidant activity. Food Chem. 2022;383:132531. [Google Scholar] [PubMed]
45. Davies KM, Jibran R, Zhou Y, Albert NW, Brummell DA, Jordan BR, et al. The evolution of flavonoid biosynthesis: a bryophyte perspective. Front Plant Sci. 2020;11:7. [Google Scholar] [PubMed]
46. Sharifi P, Astereki H, Pouresmael M. Evaluation of variations in chickpea (Cicer arietinum L.) yield and yield components by multivariate technique. Ann Agr Sci. 2018;16:136–42. [Google Scholar]
Supplementary Materials
Figure S1: The HPLC chromatograms for quantification of vitexin and isovitexin
Cite This Article
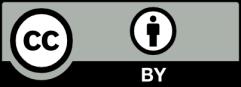