Open Access
REVIEW
Review on marine collagen peptides induce cancer cell apoptosis, necrosis, and autophagy by reducing oxidized free radicals
1 Department of Grass Science, College of Animal Science and Veterinary Medicine, Heilongjiang Bayi Agricultural University, Daqing, 163319, China
2 Department of Biochemistry and Molecular Biology, College of Life Science and Technology, Heilongjiang Bayi Agricultural University, Daqing, 163319, China
3 National Coarse Cereals Engineering Research Center, Daqing, 163319, China
* Corresponding Author: CHENGHAO JIN. Email:
BIOCELL 2023, 47(5), 965-975. https://doi.org/10.32604/biocell.2023.027729
Received 12 November 2022; Accepted 31 January 2023; Issue published 10 April 2023
Abstract
Marine collagen peptides (MCPs) are natural products prepared by hydrolyzing marine collagen protein through a variety of chemical methods or enzymes. MCPs have a range of structures and biological activities and are widely present in marine species. MCPs also have a small molecular weight, are easily modified, and absorbed by the body. These properties have attracted great interest from researchers studying antioxidant, anti-tumor, and anti-aging activities. MCPs of specific molecular weights have significant anti-tumor activity and no toxic side effects. Thus, MCPs have the potential use as anti-cancer adjuvant drugs. Free radicals produced by oxidation are closely related to human aging, cancer, arteriosclerosis, and other diseases, but their relationship with cancer is not well known. In this review, we focus on the antioxidant properties of MCPs in the treatment of cancer, highlighting their antioxidant molecular structure and potential for clinical practice.Keywords
The ocean occupies the largest area on the earth’s surface and hosts many types of marine creatures. The marine industry separates biologically active substances from fish bones and meat that are beneficial to human health to avoid wasting resources (Vedanjali and Mark, 2018). This process not only reduces pollution but also increases the value of by-products (Milica et al., 2020). Bioactive peptides derived from marine waste have aroused great interest in the pharmaceutical industry due to their broad spectrum of activities, including antioxidant, anti-cancer, and anti-hypertensive properties (Zhang et al., 2021).
The increase in aging populations, destruction of the ecological environment, unhealthy lifestyles, and food safety issues have contributed to the rising incidence of cancer. Cancer has become a public health issue and even a social issue that is of high concern (Kurian et al., 2018). China urgently needs to address concerns caused due to cancer; an even more serious issue is that the momentum in cancer incidence has not been effectively curbed (Wei et al., 2018). Research by the National Cancer Center predicts that the overall cancer death rate will continue to decline, and while the incidence will level off for men, it will increase slightly for women. These trends reflect demographic changes in cancer risk factors, use of screening tests, diagnostic practices, and treatment progress (Henley et al., 2020).
The marine biological proteins are much larger, and types and numbers than terrestrial biological proteins (Mooney et al., 2020). It was originally believed that free amino acids (aa) can be absorbed after a protein is hydrolyzed (MacDonald et al., 2019). However, subsequent research demonstrated that the body absorbs a mixture of oligopeptides and aa better than aa alone, particularly when the aa’s transport system malfunctions. Under the same concentration and composition of aa, the mixture of oligopeptides and aa indirectly improve absorption (Lourdes and Blanca, 2020). After chemotherapy and radiation therapy, the human digestive system is greatly affected, and the nutritional status of the patient is poor. Therefore, while supplying essential aa to the human body, appropriate supplementation of biologically active peptides, such as marine collagen peptides (MCPs), can enhance its absorption (Apostolopoulos et al., 2021). MCPs are a readily available resource extracted from the skin, bones, and scales of marine organisms. Among the many raw materials for extracting MCPs, fish scales are the best choice. Fish scales are the safest source among many raw materials because they are not distributed in vessels, thus minimizing the transfer of disease, pollution, or harmful toxins (Salvatore et al., 2020). MCPs extracted from deep-sea fish scales are more easily absorbed by the human body. The unique structure and sequence of aa in MCPs results in a range of biological activities, including antioxidant, anti-cancer, and anti-coagulant effects (Felician et al., 2019). In this review, we first describe the composition of MCPs, followed by their reducing and anti-oxidant properties. We then provide insights into how the arrangement of aa in MCPs leads to their anti-oxidant activity. Finally, we delve deeper into the anti-cancer effects of MCPs by exploring their autophagy, apoptosis, and necrosis effects on cancer cells.
Composition Characteristics of Marine Collagen and Marine Collagen Peptides
Marine collagens are an important protein that connects tissues, and primarily contain glycine (Gly), alanine (Ala), proline (Pro), and hydroxyproline (Hyp) (Lim et al., 2019). Marine collagens are mainly type I (most abundant) and type V collagens, and the molecule consists of three α-chains with a molecular weight of about 100 kDa (Rahman, 2019). Collagen is abundant in the fibrous connective tissues of marine animals, providing tensile strength and flexibility to tissues and organs (Song et al., 2019). Marine collagens contain sarcoplasmic peptide (30 kD), myofibrin peptide (including myosin heavy chain (192 kD), peptide (97.4 kD), tropomyosin peptide and troponin peptide (29 kD), and other functional protein peptides (Stammers et al., 2020). The aa composition of MCPs is similar to that of marine collagen. The periodic arrangement of GlyX-Y, Pro, and Hyp often appears in the marine collagen and MCPs structure, accounting for about 25% of the composition. Pro and Hyp are unique aa of marine collagens and MCPs at the X and Y positions, simultaneously, and they also contain the essential arginine (Arg) (Carrera et al., 2019). Compared with other terrestrial animal collagens, Hyp content is lower in marine collagens while methionine (Met) content is relatively high. Hyp plays a key role in linking polypeptides and stabilizing the triple helix structures, therefore, marine collagens possess a low denaturation temperature (Zhang et al., 2019). The most common structural feature of collagen peptides is the triple-helix structure, which comprises three α-polypeptide chains, each of which has a left-handed helix configuration. The three left-handed helical chain forks are entangled into a right-handed helical structure, which makes its molecular structure very stable, with low immunogenicity and good biocompatibility (Hu et al., 2017). Compared to marine collagens, MCPs have a lower molecular weight and strong affinity for water (hydrophilic group: -COOH, -OH, -NH2, Gly, Ala, aspartic acid (Asp), serine (Ser) and tyrosine (Tyr) residues). Furthermore, MCPs have a higher absorbed efficiency than marine collagens in the body and avoid absorption obstacles (edible absorption rate = 100%) (Pavlicevic et al., 2020).
Reducing Characteristics of Active Marine Collagen Peptides
Reactive oxygen species (ROS) is a general term for small molecular oxygen atoms with active chemical properties. Excessive ROS peroxidizes unsaturated fatty acids in lipids and destroys the structure of cell membranes (Giorgi et al., 2018; Saima et al., 2018; Tejero et al., 2019; Pospíšil et al., 2019). Under normal physiological conditions, cells clear excess ROS through a variety of antioxidant defense mechanisms and maintain the balance of intracellular ROS levels through enzymes catalase (CAT), superoxide dismutase (SOD), glutathione peptide per-oxidase (GSH-PX), and peroxidase (Prdxs), as well as non-enzymes vitamin C and E, glutathione (GSH), fatty acid, carotenoids, and iron chelators (Dania et al., 2015). Fe3+ or Fe3+ complexes can catalyze the formation of ROS and promote lipid peroxidation, thus causing damage to cells and tissues. The chelation of MCPs with metal ions can eliminate its catalytic effect. Giménez et al. (2009) employed a FRAP experiment (Fe2+ reduction ability) to show a significantly higher reducing ability of MCPs than that of marine collagen proteins (p < 0.05). The ability to remove Fe3+ may be hidden in marine collagen proteins, while the MCPs have their aa exposed through protease hydrolysis, thus enabling MCPs to exhibit stronger reducing ability. Recently, measurement of the scavenging rate of hydroxyl radicals after enzymatic hydrolysis revealed that MCPs are natural active oxygen scavengers (Stefania et al., 2019). Increasing concentrations of MCPs gradually increased the clearance rate of HO- (Yang et al., 2019). A recent study investigated the effect of digestion decomposition of monkfish to produce various MCPs in vitro and used free radical scavenging and lipid peroxidation assays to evaluate the antioxidant activity of MCPs. MCPs-mediated removal of HO- (41.32% ± 2.73%) and DPPH• (44.54% ± 3.12%) was superior to their removal by pepsin and trypsin. Another study showed that the low-molecular-weight angel fish collagen peptide has good anti-radical activity, attributed to its ability to remove HO-, DPPH, and O2-, with the removal of HO-being the highest (Jin et al., 2019).
Antioxidant Structural Characteristics of Active Marine Collagen Peptides
The generation of oxidative stress is attributed to the formation of several ROS, including alkyl, hydroxyl, superoxide, peroxide, and singlet oxygen. In the human body, ROS can mediate oxidative damage to cell membranes, proteins, and DNA. The ensuing oxidative stress is a result of the imbalance between free radicals and antioxidants (Sun et al., 2020). These damages can lead to a range of diseases, including inflammation, cancer, neuronal damage, and aging. Many studies have shown that MCPs derived from marine creatures can promote human health by reducing the risk of chronic diseases related to oxidative stress.
Peptides are the essential molecular structure of proteins and possess various physiological effects. Similarly, peptide efficacy also changes when the structure of the aa changes (Fatma et al., 2018). There are thousands of peptides with diverse structures. Researchers over the past decade have demonstrated that natural peptides are safer than chemically synthesized antioxidants. Therefore, naturally derived antioxidants have become the focus of research. MCPs are intermediates between proteins and aa, and form after the molecular chain of marine collagen disintegrates under the action of chemicals, bacteria, and enzymes (Subhadeep et al., 2018). The molecular weight of MCPs varies with different conditions such as reaction mode, time, and temperature. MCPs mainly consist of oligopeptides in a linear chain with a molecular weight range of 180–1000 Da (Chen et al., 2019).
Scholars have analyzed the aa composition and content of Lophius litulon collagen peptide, depicted in Table 1 (Silva et al., 2014; Lin et al., 2019; Tian et al., 2020). It can be seen that Gly, Pro, Hyp, Met, and other aa closely related to their properties have higher content. Like other sources of fish skin collagen(Nazeer et al., 2012; Zhu et al., 2012; Nguyen et al., 2020), Gly makes up the highest content (36.61%), followed by Pro (9.32%), Hyp (7.54%), and Met (6.09%). Phenylalanine (Phe) and cysteine (Cys) make up an extremely small (less than 1.1%) component of MCPs. Scholars found similar results for the composition and content of the anglerfish collagen peptide (Nagai et al., 2002; Hwang et al., 2007; Bernardini et al., 2011; You et al., 2011; Sun et al., 2013).
Further analysis has been conducted on the relationship between the composition of aa and the antioxidant properties of MCPs. Dávalos et al. (2004) found that tryptophan (Trp), Tyr, and Met have the strongest antioxidant properties, followed by Cys, Histidine (His), and Phe. The scavenging ability of oxidized free radicals by Met and Cys relies on their sulfhydryl groups as electron donors to terminate the chain reactions of free radicals. Lysine (Lys), Asp, and glutamic acid (Glu) can scavenge ROS because their amino or carboxyl side chain contains a proton donor (Rajapakse et al., 2005). Although some aa do not have the structure to scavenge oxidative free radicals, they play an auxiliary role. For example, leucine’s (Leu) hydrophobicity enables oligopeptides to smoothly cross the phospholipid bilayer so that the antioxidant effect occurs in intracellular space (Wang et al., 2020a). Met also plays an analogous role in the antioxidant activity of MCPs.
Correlation between AA Arrangement Order of Marine Collagen Peptides and Their Antioxidant Capacity
In addition to composition, the sequence of aa also has a significant impact on the antioxidant properties of MCPs (Chiara et al., 2016). Studies have shown that marine animals, mainly fish, produce MCPs by their degradation from various enzymes, which results in a diversity of peptides with different hydrophobicity and hydrophilicity. These MCPs are summarized in Table 2 (Mendis et al., 2005; Kim et al., 2007b; Kim et al., 2007a; Je et al., 2008; Ngo et al., 2010; Lee et al., 2011; Kumar et al., 2011; Sampath Kumar et al., 2012; Kumar and Nazeer, 2012; Zhang et al., 2012; Ko et al., 2013; Jeong et al., 2013; Cho et al., 2014; Jiang et al., 2014; Venkatesan et al., 2017; Hu et al., 2020; Yu et al., 2020; Li et al., 2021) and can be used as effective antioxidants.
Eresha et al. (2005) showed that the sequences of the two peptides in squid skin collagen peptides with high antioxidant activity are Asn-Gly-Pro-Leu-Gln-Ala-Gly-Gln-Pro-Gly-Gln-Arg (1241.59 Da) and Phe-Asp-Ser-Gly-Pro-Ala-Gly-Val-Leu (880.18 Da), and their resistance to oxidation is related to the position of Leu in the sequence. These two representative peptides had a strong inhibitory effect on lipid peroxidation, which was significantly higher than that of alpha-tocopherol. Furthermore, the peptides could also scavenge highly active free radicals in the oxidation system—hydroxyl radicals and carbon-centered free radicals (Cai et al., 2022). Guo et al. (2009) found that the Tyr residue showed strong scavenging of hydroxyl radicals and superoxide anions at the C-terminal of the peptide chain. It often appears in three dipeptides: Lys-Tyr, Arg-Tyr, and Tyr-Tyr. This ability may be due to the phenolic hydroxyl group acting as a proton donor binding free radicals and thus having a scavenging effect. A strong antioxidant activity is at the N-terminal of the peptide chain when the second aa residue is His (Ala-His, Val-His-His-Ala-Asn-Glu-Asn, Val-His-His). These findings indicate that the strength of antioxidant properties may be related to the sequence and arrangement of aa (Ucak et al., 2021).
The Anti-Cancer Effect of Marine Collagen Peptides Is Based on Their Antioxidant Activity
ROS is the main molecule produced during oxidative stress in the body and is considered an important factor in the occurrence, development, and recurrence of tumors (Kamesh and Tay, 2019). However, recent studies found that ROS can achieve the purpose of cancer treatment by accelerating tumor cell apoptosis. At present, drugs and methods are gradually being used clinically that are aimed at increasing the level of ROS in tumor cells.
In normal cells, the oxidation and antioxidant systems are maintained in a relatively balanced state. An increase in the level of pro-oxidation or a decrease in antioxidant capacity leads to the accumulation of ROS, which can cause a series of chronic diseases. At present, the cause of tumors is not fully understood but ROS damage to biological macromolecules can play a role in carcinogenesis due to radiation and chemicals, and cancer promotion. In the process of lipid peroxidation, a variety of free and non-free radicals can be produced to cause cell dysfunction, which is related to various diseases such as body aging, tumors, and inflammation (Ghoneum et al., 2020). In particular, lipid free radicals with moderate reactivity can easily penetrate and diffuse into the nucleus, directly attacking DNA or RNA. If the free radicals are not eliminated in time, they may cause DNA mutations and lead to cancer (Arfin et al., 2021). The cancer-promoting ability of cancer promoters is positively correlated with their ability to generate free radicals (Cao et al., 2020). Substances that can scavenge free radicals can also inhibit cancer-promoting effects. Increased levels of ROS and changes in cellular antioxidant enzymes can lead to the occurrence of tumors; patients with tumors usually show an imbalance in the body’s redox state. There is also an interaction between the tumor and the antioxidant system. DeNicola et al. (2011) used a mouse model to show for the first time that elevated ROS levels can increase resistance to certain cancers. The study also found that the regulation of the redox state seems to be a significant factor in determining tumor formation potential; therefore, its effective elimination of reactive oxygen free radicals may be an approach to treating cancer. MCPs have many advantages, such as being present in a wide variety and having remarkable antioxidant properties, which have become a research hotspot. Therefore, scholars are starting to pay attention to MCPs and other natural antioxidants for tumor prevention and treatment (Cheung et al., 2015).
Antioxidant activity of marine collagen peptides
Free radical damage to cells and tissues is the underlying cause of many diseases. The combination of DNA and protein under the action of free radicals can cause various forms of damage, and even induce the formation of tumors. As a natural antioxidant, MCPs can not only prolong life but also inhibit the occurrence and development of spontaneous tumors. Liang et al. (2010) showed that the MCPs obtained by enzymolysis from Oncorhynchus keta skin reacted with free radicals in the system to directly inhibit the formation of malondialdehyde (MDA), the product of lipid peroxidation, and maintain the redox balance in the cell. In 4.5% and 9% (wt/wt) MCP-treated male rats and 9% (wt/wt) MCP-treated female rats, tumor growth rate and overall incidence were inhibited in Sprague-Dawley rats. Wang et al. (2020b) extracted six kinds of bioactive peptides with antioxidant properties from fish collagen. All of them exhibited good scavenging activity of 2, 2-diphenyl-1-picrylhydrazyl (DPPH•) free radicals. Among them, three kinds of bioactive peptides could protect hepatocytes from H2O2-induced oxidative damage by reducing ROS and MDA levels and activating intracellular antioxidant enzymes SOD, CAT, and GSH-Px. Li et al. (2022) showed that MCPs exerted antioxidant activity by activating the Keap1/Nrf2-ARE signaling pathway. The antioxidant mechanism of MCPs is shown in Fig. 1.
Figure 1: Marine collagen peptides (MCPs) exert antioxidant activity by activating the Kelch-like ECH-associated protein 1 (Keap1)/NF-E2-related factor 2 (Nrf2)-antioxidant responsive element (ARE) signaling pathway.
Keap1 binds to Nrf2 in the cytoplasm in an inactive state. After stimulation, the conformation of Keap1 changes, the binding of Keap1-Nrf2 is unstable. Nrf2 is released and transferred to the nucleus, binds with ARE, activates the transcription of downstream genes, and then translates a series of related proteins to play physiological functions. MCPs significantly increase the expression of Nrf2 and decrease the expression of Keap1. The increased Nrf2 enters the nucleus and initiates the Nrf2/ARE signal transduction pathway, which increases the contents of SOD, CAT, and GSH-Px in the body, decreases the levels of ROS and MDA, and finally exerts antioxidant activity.
Marine collagen peptides provide negative ions to inhibit carcinogenesis
One of the main causes of carcinogenesis of human cells is the removal of electrons. ROS (unsaturated electrons) competes for electrons, causing the cells to lose electrons. During carcinogenesis, cancerous cells snatch electrons from surrounding cells, and cause a vicious circle. MCPs, small molecular protein peptides, provide negative ions to the human body (Zhao et al., 2019). After the human body receives negative ions, the negative ions have excess electrons, which can provide a large number of electrons. By blocking the vicious cycle of electron removal, cancer cells formation can be prevented or inhibited. The results of one study indicated that the antioxidant activity of MCPs has clinical significance for tumor treatment (Zorov et al., 2014).
Marine collagen peptides regulate ROS to promote tumor cells apoptosis, necrosis, and autophagy
Solid tumors are unable to provide sufficient O2 and nutrients to the growing tumor tissues due to their abnormal and deformed vascular system. Moreover, the available O2 is consumed by the rapidly proliferating cells around the tumor, which leads to serious O2 shortages, resulting in a severely hypoxic microenvironment (Tas et al., 2016). Most solid tumors have varying degrees of hypoxia, and this hypoxic microenvironment is also considered to be one of the main obstacles to current cancer treatment. Abnormal and deformed blood vessels make it difficult for a variety of chemotherapeutic drugs to reach the lesion in the tumor (Caplazi et al., 2015; Mateen et al., 2016; Galligan et al., 2016). Similarly, it is also a major obstacle to the delivery of nutrients (Dai et al., 2019). Severe hypoxia prevents the uptake of nutrients deep inside the tumor tissue. Accordingly, peripheral tumor tissues jointly adopt photodynamic/sonodynamic therapy, which has been rapidly developed by tumor tissues in recent years and is expected to become an effective means of cancer treatment (Zhou et al., 2020). Based on the understanding of tumor hypoxia, researchers have begun to try a variety of antioxidant methods to treat tumors. The most common approaches include two strategies: one is dedicated to correcting the abnormal characteristics and improving the tumor microenvironment, dredging capillaries so that anti-cancer drugs can be delivered to the lesions, to create better conditions for cancer treatment. The other is dedicated to the design of antioxidant substances to create a hypoxic environment at the tumor site; reducing the tumor’s access to nutrients and oxygen, can achieve the purpose of treatment by accelerating tumor cell death (Zonyane et al., 2020). MCPs are natural antioxidants extracted from marine animals and plants. In recent years, an increasing number of researchers have discovered that MCPs can effectively scavenge active oxygen free radicals and specifically kill and inhibit tumor cells, thereby inhibiting tumor activity and protecting human health. The study also found that the regulation of the redox state seems to be an important factor in determining its tumor formation potential; therefore, MCPs may be a potential therapeutic target. Since then, the role of MCPs has attracted increasing attention in tumor treatment. At the moment, various drugs that cause cell oxidative stress by regulating ROS levels are often used clinically, and they often have different degrees of toxic side effects. However, there is still a lack of in-depth research on the role of anti-cancer drugs in regulating oxidative stress in tumor treatment. Consequently, MCPs are of great significance in the treatment of tumors; however, the specific mechanism of action of MCPs in tumor treatment needs to be explored further. To better understand how MCP regulation of ROS can have anti-cancer effects, we discuss in the following sections the relationship between ROS and tumor cell apoptosis, necrosis, and autophagy.
Relationship between reactive oxygen species and apoptosis
The apoptosis receptor Fas-FasL pathway and the mitochondrial pathway are interrelated and depend on the ROS levels. The exogenous and endogenous pathways of apoptosis are related to each other, as shown in Fig. 2.
Figure 2: The exogenous and endogenous pathways of apoptosis are related to each other.
After FasL binds to Fas, the trimerized death receptor Fas recruits adaptor proteins such as Fas-related death domain through the death domain. The adaptor protein forms a complex with the pro-caspase-8 through the death effect domain, which is called the death-inducing signaling complex (DISC). In the cytoplasm, the activated caspase-8 can break the Bid into tBid, which transfers to the mitochondria, and induces the release of cytochrome C from the mitochondria into the cytoplasm. In addition, caveolin-l-Y14 (Cav-1-Y14), an internal protein located on the cell surface, is phosphorylated under the action of ROS. Cav-1-Y14 regulates apoptosis by interacting with Fas and Bid and connects the death receptor pathway and the mitochondrial pathway. This interaction is achieved by ROS-mediated regulation of Cav-1 Y14 phosphorylation.
Relationship between reactive oxygen species and necrosis
Cell necrosis and apoptosis can occur simultaneously in cells and are two ways of causing cell death. Studies have shown that excessive levels of ROS induce apoptosis, and a large amount of ROS can cause necrosis. Tumor cells are more sensitive to ROS than normal cells, allowing ROS to selectively kill tumor cells. Aleman et al. (2011) studied the effects of different enzymatic hydrolyzed squid protein peptides on the growth of malignant tumor cell lines MCF-7 and U87. The results showed that hydrolyzing squid protein peptides with different proteases resulted in the necrosis of MCF-7 and U87 cells in a ROS-dependent manner. At 72 h, the inhibitory effects of the hydrolysate of preprotease on the cytotoxicity of MCF-7 and U87 were the highest (96.6 ± 0.5)% and (91.2 ± 2.7)%, respectively. In addition, in the above-mentioned study, squid protein peptides can inhibit the expression of malignant tumor cell mRNA, thereby preventing the expansion of cancer cells.
Relationship between reactive oxygen species and autophagy
Autophagy describes programmed cell death. Oxidative stress can induce autophagy under certain conditions. Recent studies have found that ROS produced as a signal molecule plays an important role in the process of autophagy in mitochondria. It has a dual effect on the cell. ROS-produced signaling molecules can reduce oxidative stress and thus have a self-protection effect. However, the signaling molecules can have a destructive effect by stimulating autophagy cell death (Montani et al., 2019).
Marine Collagen Peptides Play an Anti-Tumor Role by Targeting Reactive Oxygen Species
The rapid growth of solid tumors creates a demand for oxygen and nutrients, and subsequently causes a microenvironment of ischemia and hypoxia in the tumors (LaGory and Giaccia, 2016). MCPs can bind to tumor genes and regulatory factors, thereby exerting an anti-cancer effect. Specifically, MCPs can kill and inhibit tumor cell lines MCF-7 and U87 growth and development. In addition, MCPs have good antioxidant activity and can eliminate ROS to achieve anti-tumor effects and also remove free radicals in normal tissues to play a role in anti-aging and other health care. For example, squid skin collagen peptides are rich in D-amino acids, hydroxyl acids, α-amino acids, and β-amino acids (Raman and Mathew, 2014). These small peptides can inhibit tumor cell proliferation. The research results of Huang et al. (2017) showed that the MCPs isolated and purified from squid can inhibit the proliferation of prostate cancer PC-3 cells. Further study showed that PC-3 cells treated with MCPs (5, 10, and 15 mg/mL) increased the percentage of early apoptotic cells (from 8.85% to 29%) for 24 h. The research also found that squid skin MCPs SP1 (>10,000 Da), SP2 (6000~10,000 Da), and SP3 (2000~6000 Da) could significantly inhibit melanoma B16 cells (Peng et al., 2020). Among them, the inhibitory effect of SP2 is the most obvious. The tyrosinase-mediated inhibition rate of 20 g/L fish MCPs reached more than 30%, which is much stronger than similar products on the market. Japanese sea cucumber MCPs can significantly inhibit melanin synthesis and tyrosinase activity, as well as down-regulate the expression of tyrosinase mRNA (Kim et al., 2001). The inhibitory effect of MCPs with a molecular weight of 6000~10,000 Da was the most apparent. MCPs with antitumor activity extracted from sea cucumber could inhibit the proliferation of Lewis lung cancer (LLC) cells and inhibit the migration of lung cancer cells by inhibiting the adhesion of LLC cells to extracellular matrix proteins (Qiao et al., 2021).
MCPs can activate c-FOS protein through the endoplasmic reticulum (ER) to induce cell apoptosis. Similarly, MCPs isolated from groupers show strong anti-tumor activity by activities from the corresponding proteins of the ER. The results showed that MCPs inhibited the proliferation of human leukemia cells U937, and the inhibitory effect was the best when the concentration of MCPs was 2–5 μg/mL. After treating U937 cells with 3 μg/ml MCPs for 24 h, the ADP/ATP ratio increased and the expression of apoptosis-related proteins was activated. Apoptosis was observed in U937 cells treated with 4 μg/mLMCPs (Chen et al., 2009). Prasun et al. (2013) purified a glycopeptide from cod (named TFD100), which binds β-galactoside-binding lectin (gal3) to block gal3-mediated angiogenesis, and subsequent tumor-endothelial cell interactions and the metastasis of prostate cancer cells. The results showed that 3.5 nM TFD100 inhibited angiogenesis by 70–75%, which was consistent with the effect of 50 μM lactose. Further evaluation of the antiangiogenic activity of TFD100 in vivo showed that VEGF-induced angiogenesis was enhanced by 33% in the presence of 0.03 μM exogenous gal3, but inhibited by 83% in the presence of 2 nM TFD100.
Anti-tumor MCPs can be divided into three types: linear peptides, cyclic peptides, and marine protein hydrolysates according to their size and structure. Discodermins are the first end-to-end novel cyclic dipeptides isolated from the marine sponge. All discodermins had cytotoxic effects on human lung cells with IC50 values ranging from 0.02 to 20 μg/mL (Wu et al., 1994). The IC50 values of MCPs cyclic octapeptide extracted from sponge stalagmite against myeloma PMI-8226 cells and gastric MGC-803 cells were 4.9 and 9.7 μM, respectively (Zhan et al., 2014). MCPs use their antioxidant properties to achieve anti-cancer effects. MCPs have good anti-oxidant activity in normal tissues or cells and play a role in protecting against damage and delaying aging. Fazli’s research showed that fish scale collagen peptide (FSCP) down-regulates key pro-inflammatory cytokines tumor necrosis factor-α, interleukin (IL)-1β, IL-8, and inducible nitric oxide synthase-mediated mechanisms to increase the viability of HaCaT cells and improve cellular oxidative damage (Subhan et al., 2017). In addition, FSCP also prevents cell senescence by inhibiting Bax expression, caspase-3 activity, and cyto-C, and upregulating Bcl-2 protein levels in CoCl2-stimulated HaCaT cells. The inhibitory effect of FSCP on cytotoxicity was found to be related to ROS, mitogen activated protein kinase (MAPK), and nuclear factor kappa B (NF-κB) signaling pathways. Another experiment showed that the purified MCPs (GM2-2-3) treated with lipopolysaccharide (LPS)-induced damage to liver cells, the cell oxidative damage was significantly reduced, and the intracellular SOD, CAT, and GSH-PX activities increased. Compared with the injured group, the GM2-2-treated group demonstrated a more stretched morphology of liver cells, more aggregation of cell chromatin, reduced nuclear shrinkage phenomenon, and reduced early apoptosis rate. Another study showed that different concentrations of cod skin collagen peptides could repair damage induced by LPS in liver cells (Ngo et al., 2011). The mechanism of MCPs to exert anticancer activity is summarized in Fig. 3.
Figure 3: Marine collagen peptides (MCPs) exert anticancer activity by inducing cell apoptosis and inhibiting cell migration.
MCPs increase the expression level of pro-apoptotic protein Bax and reduce the expression level of anti-apoptotic protein Bcl-2 by regulating the expression of MAPK, NF-κB, and tumor suppressor gene p53, so as to promote cell apoptosis. In addition, MCPs inhibit cell adhesion to ECM protein by regulating the expression levels of fibronectin, laminin, vitronectin, and collagen, and ultimately play an anticancer role together.
Current and Future Developments
Free radicals are related to human aging, cancer, arteriosclerosis, and other diseases. At present, research has confirmed that aquatic polypeptides have good antioxidant activity in vivo and in vitro and have shown certain application prospects. The role of ROS in tumor treatment has attracted increasing attention. Various drugs used in clinical treatment cause oxidative stress by regulating the level of ROS. However, there is still a lack of a unified understanding of the role of anti-cancer drugs in regulating oxidative stress during tumor treatment. Therefore, the application of antioxidant drugs still needs further research for tumor treatment, and its specific mechanism of anti-tumor effect also needs to be further explored.
Proteins and peptides, derived from marine animals, have attracted wide attention due to their diversified biological activities and natural abundance. Years of research has revealed that MCPs have more hydrophilic groups after hydrolysis. Therefore, collagen peptides have many excellent biological properties, including anti-oxidation and anti-cancer, antibacterial, immune regulation, and blood sugar lowering effects. These properties are coupled with good biocompatibility, non-toxicity, and high absorption rate, thereby making MCPs a safe choice for the development of health products.
With the improvement in quality of life, there is a higher demand for daily health care. Because of their inherent biological activity, MCPs can be used as therapeutic or preventive agents. In addition, MCPs, as small molecules, can chelate metal ions through coordinated covalent bonding or adsorption bonding. The chelate has the advantages of high bioavailability, safety, and biological activity. In basic research, the ability of MCPs to inhibit tumor growth and protect normal tissues has shown promising effects. In different types of cancer, the objective response time to exert the drug effect varies, which indicates the need for real-time monitoring of patients. Therefore, the development of reliable anti-cancer drugs remains a challenge. Concurrently, the structure of the human body is very complicated; after the MCPs enter the human body, their action is affected by many factors, such as bacterial flora and virus in vivo. Even though MCPs are used more frequently as health products, a common mechanism for MCPs in regulating ROS has not yet been discovered. This may be a focus for future research to better understand the anti-cancer effects that MCPs exert.
Acknowledgement: We thank LetPub (www.letpub.com) for its linguistic assistance during the preparation of this manuscript.
Funding Statement: This work was supported by the Central Government Supports Local College Reform and Development Fund Talent Training Projects [Grant Number 2020GSP16] and the Heilongjiang Touyan Innovation Team Program [Grant Number 2019HTY078].
Author Contributions: Chenghao Jin and Yinghua Luo conceived and designed the review. Yu Zhang and Tong Zhang drafted the manuscript. Yannan Li, Hui Xue, and Jinglong Cao made substantial contributions to the design of the figures. Wenshuang Hou, Jian Liu, Yuhe Cui, and Ting Xu interpreted the data for the work. All authors read and approved the final version of the manuscript.
Availability of Data and Materials: The datasets generated during and/or analyzed during the current study are available from the corresponding author upon reasonable request.
Ethics Approval: Not applicable.
Conflicts of Interest: The authors declare that they have no conflicts of interest to report regarding the present study.
References
Aleman A, Santín E, Bordenave S, Arnaudin I, Gómez Guillén MC, Montero García P (2011). Squid gelatin hydrolysates with antihypertensive, anticancer and antioxidant activity. Food Research International 44: 1044–1051. https://doi.org/10.1016/j.foodres.2011.03.010 [Google Scholar] [CrossRef]
Apostolopoulos V, Bojarska J, Chai TT, Elnagdy S, Kaczmarek K et al. (2021). A global review on short peptides: Frontiers and perspectives. Molecules 26: 430. https://doi.org/10.3390/molecules26020430 [Google Scholar] [PubMed] [CrossRef]
Arfin S, Jha N, Jha S, Kesari KK, Ruokolainen J, Roychoudhury S, Rathi B, Kumar D (2021). Oxidative stress in cancer cell metabolism. Antioxidants 10: 642–670. https://doi.org/10.3390/antiox10050642 [Google Scholar] [PubMed] [CrossRef]
Bernardini R, Harnedy P, Bolton D, Kerry J, O’Neill E, Mullen AM, Hayes M (2011). Antioxidant and antimicrobial peptidic hydrolysates from muscle protein sources and by-products. Food Chemistry 124: 1296–1307. https://doi.org/10.1016/j.foodchem.2010.07.004 [Google Scholar] [CrossRef]
Cai G, Moffitt K, Navone L, Zhang Z, Robins K, Speight R (2022). Valorisation of keratin waste: Controlled pretreatment enhances enzymatic production of antioxidant peptides. Journal of Environmental Management 301: 113945. https://doi.org/10.1016/j.jenvman.2021.113945 [Google Scholar] [PubMed] [CrossRef]
Cao Y, Wang J, Tian H, Fu GH (2020). Mitochondrial ROS accumulation inhibiting JAK2/STAT3 pathway is a critical modulator of CYT997-induced autophagy and apoptosis in gastric cancer. Journal of Experimental & Clinical Cancer Research: CR 39: 119. https://doi.org/10.1186/s13046-020-01621-y [Google Scholar] [PubMed] [CrossRef]
Caplazi P, Baca M, Barck K, Carano RA, DeVoss J, Lee WP, Bolon B, Diehl L (2015). Mouse models of rheumatoid arthritis. Veterinary Pathology 52: 819–826. https://doi.org/10.1177/0300985815588612 [Google Scholar] [PubMed] [CrossRef]
Carrera M, Ezquerra J, Aubourg S (2019). Characterization of the jumbo squid (Dosidicus gigas) skin by-product by shotgun proteomics and protein-based bioinformatics. Marine Drugs 18: 31. https://doi.org/10.3390/md18010031 [Google Scholar] [PubMed] [CrossRef]
Chen J, Gao K, Liu S, Wang S, Elango J, Bao B, Dong J, Liu N, Wu W (2019). Fish collagen surgical compress repairing characteristics on wound healing process in vivo. Marine Drugs 17: 33. https://doi.org/10.3390/md17010033 [Google Scholar] [PubMed] [CrossRef]
Chen J, Lin W, Wu J, Her GM, Hui CF (2009). Epinecidin-1 peptide induces apoptosis which enhances antitumor effects in human leukemia U937 cells. Peptides 30: 2365–2373. https://doi.org/10.1016/j.peptides.2009.08.019 [Google Scholar] [PubMed] [CrossRef]
Cheung R, Ng T, Wong J (2015). Marine peptides: Bioactivities and applications. Marine Drugs 13: 4006–4043. https://doi.org/10.3390/md13074006 [Google Scholar] [PubMed] [CrossRef]
Chiara D, Elena V, Maxim V, Papacharalambous M, Truhanov AI, Korkina LG (2016). Skin antiageing and systemic redox effects of supplementation with marine collagen peptides and plant-derived antioxidants: A single-blind case-control clinical study. Oxidative Medicine and Cellular Longevity 216: 4389410. https://doi.org/10.1155/2016/4389410 [Google Scholar] [PubMed] [CrossRef]
Cho JK, Jin YG, Rha SJ, Kim SJ, Hwang JH (2014). Biochemical characteristics of four marine fish skins in Korea. Food Chemistry 159: 200–207. https://doi.org/10.1016/j.foodchem.2014.03.012 [Google Scholar] [PubMed] [CrossRef]
Dai Q, Wang M, Li Y, Li J (2019). Amelioration of CIA by asarinin is associated to a downregulation of TLR9/NF-κB and regulation of Th1/Th2/Treg expression. Biological & Pharmaceutical Bulletin 42: 1172–1178. https://doi.org/10.1248/bpb.b19-00083 [Google Scholar] [PubMed] [CrossRef]
Dania C, Peter H, Werner J, Grefte S (2015). Interactions between mitochondrial reactive oxygen species and cellular glucose metabolism. Archives of Toxicology 89: 1209–1226. https://doi.org/10.1007/s00204-015-1520-y [Google Scholar] [PubMed] [CrossRef]
DeNicola G, Karreth F, Humpton T, Gopinathan A, Wei C et al. (2011). Oncogene-induced Nrf2 transcription promotes ROS detoxification and tumorigenesis. Nature 475: 106–109. https://doi.org/10.1038/nature10189 [Google Scholar] [PubMed] [CrossRef]
Dávalos A, Miguel M, Bartolome B, López-Fandiño R (2004). Antioxidant activity of peptides derived from egg white proteins by enzymatic hydrolysis. Journal of Food Protection 67: 1939–1944. https://doi.org/10.4315/0362-028X-67.9.1939 [Google Scholar] [PubMed] [CrossRef]
Eresha M, Rajapakse N, Byun H (2005). Investigation of jumbo squid (Dosidicus gigas) skin gelatin peptides for their in vitro antioxidant effects. Life Sciences 77: 2166–2178. https://doi.org/10.1016/j.lfs.2005.03.016 [Google Scholar] [PubMed] [CrossRef]
Fatma G, Berna S, Elif O (2018). Membrane active peptides and their biophysical characterization. Biomolecules 8: 77. https://doi.org/10.3390/biom8030077 [Google Scholar] [PubMed] [CrossRef]
Felician FF, Yu RH, Li MZ, Li CJ, Chen HQ, Jiang Y, Tang T, Qi WY, Xu HM (2019). The wound healing potential of collagen peptides derived from the jellyfish Rhopilema esculentum. Chinese Journal of Traumatology = Zhonghua Chuang Shang Za Zhi 22: 12–20. https://doi.org/10.1016/j.cjtee.2018.10.004 [Google Scholar] [PubMed] [CrossRef]
Galligan C, Keystone E, Fish E (2016). Fibrocyte and T cell interactions promote disease pathogenesis in rheumatoid arthritis. Journal of Autoimmunity 69: 38–50. https://doi.org/10.1016/j.jaut.2016.02.008 [Google Scholar] [PubMed] [CrossRef]
Ghoneum A, Abdulfattah A, Warren B, Shu J, Said N (2020). Redox homeostasis and metabolism in cancer: A complex mechanism and potential targeted therapeutics. International Journal of Molecular Sciences 21: 3100. https://doi.org/10.3390/ijms21093100 [Google Scholar] [PubMed] [CrossRef]
Giménez B, Gómez E, Alemán A, Gómez-Guillén MC, Montero MP (2009). Improvement of the antioxidant properties of squid skin gelatin films by the addition of hydrolysates from squid gelatin. Food Hydrocolloids 23: 1322–1327. https://doi.org/10.1016/j.foodhyd.2008.09.010 [Google Scholar] [CrossRef]
Giorgi C, Marchi S, Simoes ICM, Ren Z, Morciano G et al. (2018). Mitochondria and reactive oxygen species in aging and age-related diseases. International Review of Cell and Molecular Biology 340: 209–344. https://doi.org/10.1016/bs.ircmb.2018.05.006 [Google Scholar] [PubMed] [CrossRef]
Guo H, Kouzuma Y, Yonekura M (2009). Structures and properties of antioxidative peptides derived from royal jelly protein. Food Chemistry 113: 238–245. https://doi.org/10.1016/j.foodchem.2008.06.081 [Google Scholar] [CrossRef]
Henley SJ, Ward EM, Scott S, Ma J, Anderson RN et al. (2020). Annual report to the nation on the status of cancer, part I: National cancer statistics. Cancer 126: 2225–2249. https://doi.org/10.1002/cncr.32802 [Google Scholar] [PubMed] [CrossRef]
Hu X, Yang X, Wang T, Li L, Wu Y, Zhou Y, You L (2020). Purification and identification of antioxidant peptides from round scad (Decapterus maruadsi) hydrolysates by consecutive chromatography and electrospray ionization-mass spectrometry. Food and Chemical Toxicology 135: 110882. https://doi.org/10.1016/j.fct.2019.110882 [Google Scholar] [PubMed] [CrossRef]
Hu Z, Yang P, Zhou C, Li S, Hong P (2017). Marine collagen peptides from the skin of nile tilapia (Oreochromis niloticusCharacterization and wound healing evaluation. Marine Drugs 15: 102. https://doi.org/10.3390/md15040102 [Google Scholar] [PubMed] [CrossRef]
Huang F, Jing Y, Ding G, Yang Z (2017). Isolationand purification of novel peptides derived from Sepiaink: Effect apoptosis of prostate cancer cell PC-3. Molecular Medicine Reports 16: 4222–4228. https://doi.org/10.3892/mmr.2017.7068 [Google Scholar] [PubMed] [CrossRef]
Hwang J, Mizuta S, Yokoyama Y, Yoshinaka R (2007). Purification and characterization of molecular species of collagen in the skin of skate (Raja). Food Chemistry 99: 921–925. https://doi.org/10.1016/j.foodchem.2005.10.046 [Google Scholar] [CrossRef]
Je JY, Qian ZJ, Lee SH, Byun HG, Kim SK (2008). Purification and antioxidant properties of bigeye tuna (Thunnus obesus) dark muscle peptide on free radical-mediated oxidative systems. Journal of Medicinal Food 11: 629–637. https://doi.org/10.1089/jmf.2007.0114 [Google Scholar] [PubMed] [CrossRef]
Jeong HS, Venkatesan J, Kim SK (2013). Isolation and characterization of collagen from marine fish (Thunnus obesus). Biotechnology & Bioprocess Engineering 18: 1185–1191. https://doi.org/10.1007/s12257-013-0316-2 [Google Scholar] [CrossRef]
Jiang H, Tong T, Sun J, Xu Y, Zhao Z, Liao D (2014). Purification and characterization of antioxidative peptides from round scad (Decapterus maruadsi) muscle protein hydrolysate. Food Chemistry 154: 158–163. https://doi.org/10.1016/j.foodchem.2013.12.074 [Google Scholar] [PubMed] [CrossRef]
Jin HX, Xu HP, Li Y, Zhang QW, Xie H (2019). Preparation and evaluation of peptides with potential antioxidant activity by microwave assisted enzymatic hydrolysis of collagen from sea cucumber acaudina molpadioides obtained from Zhejiang Province in China. Marine Drugs 17: 169. https://doi.org/10.3390/md17030169 [Google Scholar] [PubMed] [CrossRef]
Kamesh R, Tay Y (2019). The Yin-Yang regulation of reactive oxygen species and microRNAs in cancer. International Journal of Molecular Sciences 20: 5335. https://doi.org/10.3390/ijms20215335 [Google Scholar] [PubMed] [CrossRef]
Kim SY, Je JY, Kim SK (2007a). Purification and characterization of antioxidant peptide from hoki (Johnius belengerii) frame protein by gastrointestinal digestion. The Journal of Nutritional Biochemistry 18: 31–38. https://doi.org/10.1016/j.jnutbio.2006.02.006 [Google Scholar] [PubMed] [CrossRef]
Kim S, Kim Y, Byun H, Nam KS, Joo DS, Shahidi F (2001). Isolation and characterization of antioxidative peptides from gelatin hydrolysate of Alaska pollack skin. Journal of Agricultural and Food Chemistry 49: 1984–1989. https://doi.org/10.1021/jf000494j [Google Scholar] [PubMed] [CrossRef]
Kim SS, Shim MS, Chung J, Lim DY, Lee BJ (2007b). Purification and characterization of antimicrobial peptides from the skin secretion of Rana dybowskii. Peptides 28: 1532–1539. https://doi.org/10.1016/j.peptides.2007.07.002 [Google Scholar] [PubMed] [CrossRef]
Ko JY, Lee JH, Samarakoon K, Kim JS, Jeon YJ (2013). Purification and determination of two novel antioxidant peptides from flounder fish (Paralichthys olivaceus) using digestive proteases. Food and Chemical Toxicology 52: 113–120. https://doi.org/10.1016/j.fct.2012.10.058 [Google Scholar] [PubMed] [CrossRef]
Kumar NSS, Nazeer RA (2012). Wound healing properties of collagen from the bone of two marine fishes. International Journal of Peptide Research and Therapeutics 18: 185–192. https://doi.org/10.1007/s10989-012-9291-2 [Google Scholar] [CrossRef]
Kumar NS, Nazeer R, Jaiganesh R (2011). Purification and biochemical characterization of antioxidant peptide from horse mackerel (Magalaspis cordyla) viscera protein. Peptides 32: 1496–1501. https://doi.org/10.1016/j.peptides.2011.05.020 [Google Scholar] [PubMed] [CrossRef]
Kurian KM, Jenkinson MD, Brennan PM, Grant R, Jefferies S et al. (2018). Brain tumor research in the United Kingdom: Current perspective and future challenges. A strategy document from the NCRI Brain Tumor CSG. Neuro-Oncology Practice 5: 10–17. https://doi.org/10.1093/nop/npx022 [Google Scholar] [PubMed] [CrossRef]
LaGory E, Giaccia A (2016). The ever expanding role of HIF in tumour and stromal biology. Nature Cell Biology 18: 356–365. https://doi.org/10.1038/ncb3330 [Google Scholar] [PubMed] [CrossRef]
Lee WS, Jeon JK, Byun HG (2011). Characterization of a novel antioxidative peptide from the sand eel Hypoptychus dybowskii. Process Biochemistry 46: 1207–1211. https://doi.org/10.1016/j.procbio.2011.02.001 [Google Scholar] [CrossRef]
Li J, Li Y, Lin S, Zhao W, Chen Y, Jin H (2022). Collagen peptides from Acaudina molpadioides prevent CCl4-induced liver injury via Keap1/Nrf2-ARE, PI3K/AKT, and MAPKs pathways. Journal of Food Science 87: 2185–2196. https://doi.org/10.1111/1750-3841.16142 [Google Scholar] [PubMed] [CrossRef]
Li D, Ren JW, Xu T, Li L, Liu P, Li Y (2021). Effect of bovine bone collagen oligopeptides on wound healing in mice. Aging 13: 9028–9042. https://doi.org/10.18632/aging.202750 [Google Scholar] [PubMed] [CrossRef]
Liang J, Pei XR, Wang N, Zhang ZF, Wang JB, Li Y (2010). Marine collagen peptides prepared from chum salmon (Oncorhynchus keta) skin extend the life span and inhibit spontaneous tumor incidence in Sprague-Dawley Rats. Journal of Medicinal Food 13: 757–770. https://doi.org/10.1089/jmf.2009.1279 [Google Scholar] [PubMed] [CrossRef]
Lim YS, Ok YJ, Hwang SY, Kwak JY, Yoon S (2019). Marine collagen as a promising biomaterial for biomedical applications. Marine Drugs 17: 467. https://doi.org/10.3390/md17080467 [Google Scholar] [PubMed] [CrossRef]
Lin X, Chen Y, Jin H, Zhao Q, Liu C et al. (2019). Collagen extracted from bigeye tuna (Thunnus obesus) skin by isoelectric precipitation: Physicochemical properties, proliferation, and migration activities. Marine Drugs 17: 261. https://doi.org/10.3390/md17050261 [Google Scholar] [PubMed] [CrossRef]
Lourdes A, Blanca H (2020). Current evidence on the bioavailability of food bioactive peptides. Molecules 25: 4479. https://doi.org/10.3390/molecules25194479 [Google Scholar] [PubMed] [CrossRef]
MacDonald A, Singh RH, Rocha JC, van Spronsen FJ (2019). Optimising amino acid absorption: Essential to improve nitrogen balance and metabolic control in phenylketonuria. Nutrition Research Reviews 32: 70–78. https://doi.org/10.1017/S0954422418000173 [Google Scholar] [PubMed] [CrossRef]
Mateen S, Zafar A, Moin S, Khan AQ, Zubair S (2016). Understanding the role of cytokines in the pathogenesis of rheumatoid arthritis. Clinica Chimica Acta 455: 161–171. https://doi.org/10.1016/j.cca.2016.02.010 [Google Scholar] [PubMed] [CrossRef]
Mendis E, Rajapakse N, Kim SK (2005). Antioxidant properties of a radical-scavenging peptide purified from enzymatically prepared fish skin gelatin hydrolysate. Journal of Agricultural and Food Chemistry 53: 581–587. https://doi.org/10.1021/jf048877v [Google Scholar] [PubMed] [CrossRef]
Milica P, Elena M, Marta M (2020). Marine bioactive peptides—an overview of generation, structure and application with a focus on food sources. Marine Drugs 18: 424. https://doi.org/10.3390/md18080424 [Google Scholar] [PubMed] [CrossRef]
Montani MSG, Santarelli R, Granato M, Gonnella R, Torrisi MR, Faggioni A, Cirone M (2019). EBV reduces autophagy, intracellular ROS and mitochondria to impair monocyte survival and differentiation. Autophagy 15: 652–667. https://doi.org/10.1080/15548627.2018.1536530 [Google Scholar] [PubMed] [CrossRef]
Mooney TA, Di Iorio L, Lammers M, Lin TH, Nedelec SL, Parsons M, Radford C, Urban E, Stanley J (2020). Listening forward: Approaching marine biodiversity assessments using acoustic methods. Royal Society Open Science 7: 201287. https://doi.org/10.1098/rsos.201287 [Google Scholar] [PubMed] [CrossRef]
Nagai T, Araki Y, Suzuki N (2002). Collagen of the skin of ocellate puffer fish (Takifugu rubripes). Food Chemistry 78: 173–177. https://doi.org/10.1016/S0308-8146(01)00396-X [Google Scholar] [CrossRef]
Nazeer R, Sampath K, Ganesh R (2012). In vitro and in vivo studies on the antioxidant activity of fish peptide isolated from the croaker (Otolithes ruber) muscle protein hydrolysate. Peptides 35: 261–268. https://doi.org/10.1016/j.peptides.2012.03.028 [Google Scholar] [PubMed] [CrossRef]
Ngo DH, Qian ZJ, Ryu B, Park JW, Kim SK (2010). In vitro antioxidant activity of a peptide isolated from Nile tilapia (Oreochromis niloticus) scale gelatin in free radical-mediated oxidative systems. Journal of Functional Foods 2: 107–117. https://doi.org/10.1016/j.jff.2010.02.001 [Google Scholar] [CrossRef]
Ngo D, Ryu B, Vo T, Himaya SW, Wijesekara I, Kim SK (2011). Free radical scavenging and angiotensin-I converting enzyme inhibitory peptides from Pacific cod (Gadus macrocephalus) skin gelatin. International Journal of Biological Macromolecules 49: 1110–1116. https://doi.org/10.1016/j.ijbiomac.2011.09.009 [Google Scholar] [PubMed] [CrossRef]
Nguyen TT, Heimann K, Zhang W (2020). Protein recovery from underutilised marine bioresources for product development with nutraceutical and pharmaceutical bioactivities. Marine Drugs 18: 391. https://doi.org/10.3390/md18080391 [Google Scholar] [PubMed] [CrossRef]
Pavlicevic M, Maestri E, Marmiroli M (2020). Marine bioactive peptides—An overview of generation, structure and application with a focus on food sources. Marine Drugs 18: 424. https://doi.org/10.3390/md18080424 [Google Scholar] [PubMed] [CrossRef]
Peng Z, Chen B, Zheng Q, Zhu G, Cao W, Qin X, Zhang C (2020). Ameliorative effects of peptides from the oyster (Crassostrea hongkongensis) protein hydrolysates against UVB-induced skin photodamage in mice. Marine Drugs 18: 288. https://doi.org/10.3390/md18060288 [Google Scholar] [PubMed] [CrossRef]
Pospíšil P, Prasad A, Rác M (2019). Mechanism of the formation of electronically excited species by oxidative metabolic processes: Role of reactive oxygen species. Biomolecules 9: 258. https://doi.org/10.3390/biom9070258 [Google Scholar] [PubMed] [CrossRef]
Prasun G, Engin K, Gargi B, Kaczanowska S, Davila E, Thompson K, Martin SS, Kalvakolanu DV, Vasta GR, Ahmed H (2013). Cod glycopeptide with picomolar affinity to galectin-3 suppresses T-cell apoptosis and prostate cancer metastasis. Proceedings of the National Academy of Sciences of the United States of America 110: 5052–5057. https://doi.org/10.1073/pnas.1202653110 [Google Scholar] [PubMed] [CrossRef]
Qiao R, Xiao R, Chen Z, Jiang J, Yuan C, Ning S, Wang J, Zhou Z (2021). Cloning, expression and inhibitory effects on lewis lung carcinoma cells of rAj-Tspin from sea cucumber (Apostichopus japonicus). Molecules 30: 229. https://doi.org/10.3390/molecules27010229 [Google Scholar] [PubMed] [CrossRef]
Rahman M (2019). Collagen of extracellular matrix from marine invertebrates and its medical applications. Marine Drugs 17: 118. https://doi.org/10.3390/md17020118 [Google Scholar] [PubMed] [CrossRef]
Rajapakse N, Mendis E, Byun H, Kim SK (2005). Purification and in vitro antioxidative effects of giant squid muscle peptides on free radical-mediated oxidative systems. Journal of Nutritional Biochemistry 16: 562–569. https://doi.org/10.1016/j.jnutbio.2005.02.005 [Google Scholar] [PubMed] [CrossRef]
Raman M, Mathew S (2014). Study of chemical properties and evaluation of collagen in mantle, epidermal connective tissue and tentacle of Indian Squid, Loligo duvauceli Orbigny. Journal of Food Science & Technology 51: 1509–1516. https://doi.org/10.1007/s13197-012-0671-6 [Google Scholar] [PubMed] [CrossRef]
Saima K, Feng W, Cui H (2018). The role of mitochondria in reactive oxygen species generation and its implications for neurodegenerative diseases. Cells 7: 274. https://doi.org/10.3390/cells7120274 [Google Scholar] [PubMed] [CrossRef]
Salvatore L, Gallo N, Natali ML, Campa L, Lunetti P, Madaghiele M, Blasi FS, Corallo A, Capobianco L, Sannino A (2020). Marine collagen and its derivatives: Versatile and sustainable bio-resources for healthcare. Materials Science and Engineering: C, Materials for Biological Applications 113: 110963. https://doi.org/10.1016/j.msec.2020.110963 [Google Scholar] [PubMed] [CrossRef]
Sampath Kumar NS, Nazeer RA, Jaiganesh R (2012). Purification and identification of antioxidant peptides from the skin protein hydrolysate of two marine fishes, horse mackerel (Magalaspis cordyla) and croaker (Otolithes ruber). Amino Acids 42: 1641–1649. https://doi.org/10.1007/s00726-011-0858-6 [Google Scholar] [PubMed] [CrossRef]
Silva TH, Moreira-Silva J, Marques AL, Domingues A, Bayon Y, Reis RL (2014). Marine origin collagens and its potential applications. Marine Drugs 12: 5881. https://doi.org/10.3390/md12125881 [Google Scholar] [PubMed] [CrossRef]
Song WK, Liu D, Sun LL, Li BF, Hou H (2019). Physicochemical and biocompatibility properties of type I collagen from the skin of nile tilapia (Oreochromis niloticus) for biomedical applications. Marine Drugs 17: 137. https://doi.org/10.3390/md17030137 [Google Scholar] [PubMed] [CrossRef]
Stammers M, Ivanova IM, Niewczas IS, Segonds-Pichon A, Streeter M, Spiegel DA, Clark J (2020). Age-related changes in the physical properties, cross-linking, and glycation of collagen from mouse tail tendon. The Journal of Biological Chemistry 295: 10562–10571. https://doi.org/10.1074/jbc.RA119.011031 [Google Scholar] [PubMed] [CrossRef]
Stefania D, Gianluca D, Mélanie P, Piraino S, Leone A (2019). Barrel jellyfish (Rhizostoma pulmo) as source of antioxidant peptides. Marine Drugs 17: 134. https://doi.org/10.3390/md17020134 [Google Scholar] [PubMed] [CrossRef]
Subhadeep C, Snigdha G, Kaustav M (2018). Food-derived bioactive peptides in human health: Challenges and opportunities. Nutrients 10: 1738. https://doi.org/10.3390/nu10111738 [Google Scholar] [PubMed] [CrossRef]
Subhan F, Kang H, Lim Y, Ikram M, Baek SY, Jin S, Jeong YH, Kwak JY, Yoon S (2017). Fish scale collagen peptides protect against CoCl2/TNF-α-induced cytotoxicity and inflammation via inhibition of ROS, MAPK, and NF-κB pathways in HaCaT cells. Oxidative Medicine and Cellular Longevity 2017: 9703609. https://doi.org/10.1155/2017/9703609 [Google Scholar] [PubMed] [CrossRef]
Sun Y, Lu Y, Saredy J, Wang X, Drummer Iv C et al. (2020). ROS systems are a new integrated network for sensing homeostasis and alarming stresses in organelle metabolic processes. Redox Biology 37: 101696. https://doi.org/10.1016/j.redox.2020.101696 [Google Scholar] [PubMed] [CrossRef]
Sun L, Zhang Y, Zhuang Y (2013). Antiphotoaging effect and purification of an antioxidant peptide from tilapia (Oreochromis niloticus) gelatin peptides. Journal of Functional Foods 5: 154–162. https://doi.org/10.1016/j.jff.2012.09.006 [Google Scholar] [CrossRef]
Tas S, Maracle C, Balogh E (2016). Targeting of proangiogenic signalling pathways in chronic inflammation. Nature Reviews Rheumatology 12: 111–122. https://doi.org/10.1038/nrrheum.2015.164 [Google Scholar] [PubMed] [CrossRef]
Tejero J, Shiva S, Mark T (2019). Sources of vascular nitric oxide and reactive oxygen species and their regulation. Physiological Reviews 99: 311–379. https://doi.org/10.1152/physrev.00036.2017 [Google Scholar] [PubMed] [CrossRef]
Tian X, Zheng J, Xu B, Ye J, Yang Z, Yuan F (2020). Optimization of extraction of bioactive peptides from monkfish (Lophius litulon) and characterization of their role in H2O2-induced lesion. Marine Drugs 18: 468. https://doi.org/10.3390/md18090468 [Google Scholar] [PubMed] [CrossRef]
Ucak I, Afreen M, Montesano D, Carrillo C, Tomasevic I, Simal-Gandara J, Barba FJ (2021). Functional and bioactive properties of peptides derived from marine side streams. Marine Drugs 19: 71. https://doi.org/10.3390/md19020071 [Google Scholar] [PubMed] [CrossRef]
Vedanjali G, Mark T (2018). Marine natural product peptides with therapeutic potential: Chemistry, biosynthesis, and pharmacology. Biochimica et Biophysica Acta—General Subjects 1862: 81–196. https://doi.org/10.1016/j.bbagen.2017.08.014 [Google Scholar] [PubMed] [CrossRef]
Venkatesan J, Anil S, Kim SK, Shim MS (2017). Marine fish proteins and peptides for cosmeceuticals: A review. Marine Drugs 15: 143. https://doi.org/10.3390/md15050143 [Google Scholar] [PubMed] [CrossRef]
Wang J, Liu J, Guo Y (2020a). Cell growth stimulation, cell cycle alternation, and anti-apoptosis effects of bovine bone collagen hydrolysates derived peptides on MC3T3-E1 cells ex vivo. Molecules 25: 2305. https://doi.org/10.3390/molecules25102305 [Google Scholar] [PubMed] [CrossRef]
Wang WY, Zhao YQ, Zhao GX, Chi CF, Wang B (2020b). Antioxidant peptides from collagen hydrolysate of redlip croaker (Pseudosciaena polyactis) scales: Preparation, characterization, and cytoprotective effects on H2O2-damaged HepG2 cells. Marine Drugs 18: 156. https://doi.org/10.3390/md18030156 [Google Scholar] [PubMed] [CrossRef]
Wei D, Xu J, Liu XY, Chen ZN, Bian H (2018). Fighting cancer with viruses: Oncolytic virus therapy in China. Human Gene Therapy 29: 151–159. https://doi.org/10.1089/hum.2017.212 [Google Scholar] [PubMed] [CrossRef]
Wu YN, Gadina M, Tao-Cheng JH, Youle RJ (1994). Retinoic acid disrupts the Golgi apparatus and increases the cytosolic routing of specific protein toxins. The Journal of Cell Biology 125: 743–753. https://doi.org/10.1083/jcb.125.4.743 [Google Scholar] [PubMed] [CrossRef]
Yang XR, Zhao YQ, Qiu YT, Chi CF, Wang B (2019). Preparation and characterization of gelatin and antioxidant peptides from gelatin hydrolysate of skipjack tuna (Katsuwonus pelamis) bone stimulated by in vitro gastrointestinal digestion. Marine Drugs 17: 78. https://doi.org/10.3390/md17020078 [Google Scholar] [PubMed] [CrossRef]
You L, Zhao M, Regenstein J, Ren JY (2011). In vitro antioxidant activity and in vivo anti-fatigue effect of loach (Misgurnus anguillicaudatus) peptides prepared by papain digestion. Food Chemistry 124: 188–194. https://doi.org/10.1016/j.foodchem.2010.06.007 [Google Scholar] [CrossRef]
Yu X, Su Q, Shen T, Chen Q, Wang Y, Jia W (2020). Antioxidant peptides from sepia esculenta hydrolyzate attenuate oxidative stress and fat accumulation in caenorhabditis elegans. Marine Drugs 18: 490. https://doi.org/10.3390/md18100490 [Google Scholar] [PubMed] [CrossRef]
Zhan K, Jiao W, Yang F, Li J, Wang SP, Li YS, Han BN, Lin HW (2014). Reniochalistatins A–E, cyclic peptides from the marine sponge Reniochalina stalagmitis. Journal of Natural Products 77: 2678–2684. https://doi.org/10.1021/np5006778 [Google Scholar] [PubMed] [CrossRef]
Zhang Y, Duan X, Zhuang Y (2012). Purification and characterization of novel antioxidant peptides from enzymatic hydrolysates of tilapia (Oreochromis niloticus) skin gelatin. Peptides 38: 13–21. https://doi.org/10.1016/j.peptides.2012.08.014 [Google Scholar] [PubMed] [CrossRef]
Zhang QT, Liu ZD, Wang Z, Wang T, Wang N, Wang N, Zhang B, Zhao YF (2021). Recent advances in small peptides of marine origin in cancer therapy. Marine Drugs 19: 115. https://doi.org/10.3390/md19020115 [Google Scholar] [PubMed] [CrossRef]
Zhang JB, Zhao YQ, Wang YM, Chi CF, Wang B (2019). Eight collagen peptides from hydrolysate fraction of spanish mackerel skins: Isolation, identification, and in vitro antioxidant activity evaluation. Marine Drugs 17: 224. https://doi.org/10.3390/md17040224 [Google Scholar] [PubMed] [CrossRef]
Zhao R, Jiang S, Zhang L, Yu ZB (2019). Mitochondrial electron transport chain, ROS generation and uncoupling (Review). International Journal of Molecular Medicine 44: 3–15. https://doi.org/10.3892/ijmm.2019.4188 [Google Scholar] [PubMed] [CrossRef]
Zhou X, Liu Q, Zhou X, Zhang J, Liu W, Zhao X, Luo N (2020). THH relieves CIA inflammation by reducing inflammatory-related cytokines. Cell Biochemistry and Biophysics 78: 367–374. https://doi.org/10.1007/s12013-020-00911-8 [Google Scholar] [PubMed] [CrossRef]
Zhu BW, Dong X, Zhou D, Gao Y, Yang JF et al. (2012). Physicochemical properties and radical scavenging capacities of pepsin-solubilized collagen from sea cucumber Stichopus japonicus. Food Hydrocolloids 28: 182–188. https://doi.org/10.1016/j.foodhyd.2011.12.010 [Google Scholar] [CrossRef]
Zonyane S, Fawole O, Grange C, Stander MA, Opara UL, Makunga NP (2020). The implication of chemotypic variation on the anti-oxidant and anti-cancer activities of Sutherlandia frutescens (L.) R.Br. (Fabaceae) from different geographic locations. Antioxidants 9: 152–173. https://doi.org/10.3390/antiox9020152 [Google Scholar] [PubMed] [CrossRef]
Zorov D, Juhaszova M, Sollott S (2014). Mitochondrial reactive oxygen species (ROS) and ROS-induced ROS release. Physiological Reviews 94: 909–950. https://doi.org/10.1152/physrev.00026.2013 [Google Scholar] [PubMed] [CrossRef]
Cite This Article
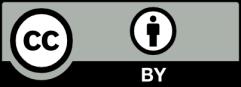