Open Access
ARTICLE
Vitamin D attenuates TGF-β1-induced lung fibroblast proliferation and migration through repression of RasGRP3
1 No. 23 Ward of Nursing Department, The First Affiliated Hospital of Wenzhou Medical University, Wenzhou, China
2 Department of Thoracic Surgery, Wenzhou Central Hospital, Dingli Clinical College of Wenzhou Medical University, Wenzhou, China
3 Department of Clinical Laboratory, The First Affiliated Hospital of Wenzhou Medical University, Wenzhou, China
* Corresponding Author: Xiaoxin Chen,
(This article belongs to the Special Issue: Biochemical and Epigenetics Changes in Health and Disease)
BIOCELL 2023, 47(6), 1243-1251. https://doi.org/10.32604/biocell.2023.027763
Received 14 November 2022; Accepted 31 January 2023; Issue published 19 May 2023
Abstract
Background: Transforming growth factor-β1 (TGF-β1) is a pleiotropic cytokine that plays a central role in the pathogenesis of idiopathic pulmonary fibrosis (IPF). While previous studies have revealed a cross-talk between vitamin D and TGF-β1 signaling, it is still unclear how they interact with each other to regulate the progression of IPF. Methods: In this work, we searched for a novel mediator of TGF-β1 activity in lung fibroblasts and examined its regulation by vitamin D. In addition, we investigated the mechanism underlying the interaction between vitamin D and TGF-β1 signaling in lung fibroblast activation. Bioinformatic analysis was performed to identify TGF-β1 downstream target genes. Knockdown and overexpression expression experiments were conducted to determine gene function in the regulation of lung fibroblast proliferation and migration. Results: Analysis of publicly available datasets revealed that RAS guanyl releasing protein 3 (RasGRP3) was upregulated in TGF-β1-treated lung fibroblasts and lung tissues from IPF patients relative to healthy controls. Our data confirmed the upregulation of RasGRP3 by TGF-β1 in human MRC5 lung fibroblasts. Overexpression of RasGRP3 enhanced MRC5 cell proliferation and migration. Knockdown of RasGRP3 blocked TGF-β1-induced MRC5 proliferation and migration. Vitamin D abolished TGF-β1-induced RasGRP3 upregulation, which was reversed by inhibition of the vitamin D receptor (VDR). Mechanistically, vitamin D promoted VDR enrichment and prevented mothers against decapentaplegic homolog (SMAD) 2 and 3 occupancy at the promoter of RasGRP3. Additionally, overexpression of RasGRP3 reversed the suppressive effect of vitamin D on MRC5 cell proliferation and migration. Conclusion: In conclusion, vitamin D antagonizes TGF-β1-induced lung fibroblast activation by repressing RasGRP3 transcription.Keywords
Idiopathic pulmonary fibrosis (IPF) is a progressive disease characterized by the excessive deposition of extracellular matrix (ECM) components in the lung, which can lead to lung dysfunction and death (Cui et al., 2022; Staab-Weijnitz, 2022). IPF has a high mortality, with overall 3-year and 5-year cumulative survival rates of 61.8% and 45.6%, respectively (Zheng et al., 2022). To date, no curative treatment options are available for IPF. Lung fibroblasts play a crucial role in the pathogenesis of IPF (Jeong et al., 2022). Injured lung epithelial cells release multiple cytokines and growth factors to promote myofibroblast differentiation and expansion of fibroblasts, consequently causing a pathological remodeling of lung architecture (Wang et al., 2022).
Transforming growth factor-β1 (TGF-β1) is an important regulator of tissue fibrosis (Meng et al., 2016; Wang et al., 2022). For example, TGF-β1 treatment stimulated fibroblast-myofibroblast transdifferentiation, as evidenced by increased expression of myofibroblast biomarkers α-smooth muscle actin (α-SMA) and collagen I (Jiang et al., 2022). In another work, TGF-β1-treated lung fibroblasts acquired a profibrogenic phenotype and showed collagen I deposition (Chen et al., 2022). TGF-β1 signaling has been reported to induce proliferation, migration, and myofibroblast transformation in lung fibroblasts (Wang et al., 2021). In a study, TGF-β1 binding promoted the assembly between TGF-β type II receptor (TβR2) and TβR1, which triggered the activation of intracellular signaling pathways by phosphorylating receptor-associated SMADs including SMAD2 and SMAD3. Further, the phosphorylated SMAD2 and SMAD3 underwent nuclear translocation and promoted gene transcription (Zhong et al., 2021). Inhibition of TGF-β1/SMAD signaling could elicit a strong anti-fibrotic effect on lung fibroblasts (Jiang et al., 2021). Thus, these observations suggest that targeting TGF-β1/SMAD signaling may benefit fibrosis treatment.
Vitamin D deficiency has been shown to have an impact on the progression of IPF. Faverio et al. (2022) enrolled IPF patients and found that 56.3% of the patients (n = 90) have a low vitamin D level. Vitamin D deficiency was significantly correlated with all-cause mortality in patients with IPF (Tzilas et al., 2019). In a high-fat-diet-induced obese mouse model, vitamin D supplementation attenuated obesity-associated lung fibrosis (Han et al., 2021). Another study reported that vitamin D prevented bleomycin-induced lung fibrosis in a mouse model (Chang et al., 2021). As a pleiotropic steroid hormone, vitamin D exerts its biological effects largely through interaction with the vitamin D receptor (VDR) (Rochel, 2022). VDR belongs to the nuclear receptor superfamily and can act as a heterodimer with retinoid X receptors (RXRs) to initiate gene transcription. VDR is expressed in a variety of cell types including lung fibroblasts (Stio et al., 1997). Despite these findings, the mechanism for the protective effect of vitamin D on lung fibrosis is still unclear.
In the present study, we explored the cross-talk between vitamin D/VDR and TGF-β1 signaling in lung fibroblasts and investigated how vitamin D modulated TGF-β1-induced lung fibroblast proliferation and migration.
Human MRC5 pulmonary fibroblasts were obtained from the American Type Culture Collection (Rockville, MD, USA) and cultured in Eagle’s Minimum Essential Medium (EMEM) supplemented with 10% fetal bovine serum (FBS; Sigma-Aldrich, St. Louis, MO, USA).
For TGF-β1 treatment, MRC5 cells were exposed to different concentrations of TGF-β1 (0, 2, 5, 10, and 20 ng/mL; Peprotech, Hamburg, Germany) for 24 h. Unless stated otherwise, 10 ng/mL TGF-β1 was used. At the concentration, TGF-β1 was shown to effectively promote the proliferation of MRC5 fibroblasts (Ballester et al., 2021). In some experiments, 1,25-dihydroxy vitamin D [1,25(OH)2D], the active form of vitamin D (100 nM; Sigma-Aldrich) (Karkeni et al., 2015) was added 24 h before exposure to TGF-β1 for another 24 h. 1,25(OH)2D was dissolved in 99.8% ethanol (Sigma-Aldrich).
Quantitative real-time polymerase chain reaction analysis
After the above treatment, MRC5 cells were lysed in Trizol reagent (Invitrogen, Carlsbad, CA, USA), and total RNA was isolated. Reverse transcription from total RNA was performed using the RevertAid First Strand cDNA Synthesis Kit (Thermo Fisher Scientific, Waltham, MA, USA). The levels of IGF1, MYH11, SERPINE2, PLN, RasGRP3, and CCDC82 transcripts were measured via the quantitative real-time polymerase chain reaction (qRT-PCR) using the 2−ΔΔCT method (Schmittgen and Livak, 2008). GAPDH was used as an internal control. The primers are shown as follows: IGF1 forward, 5′-GC TCTTCAGTTCGTGTGTGGA-3′ and reverse, 5′-GCCTCC TTAGATCACAGCTCC-3′; MYH11 forward, 5′-GTCCA GGAGATGAGGCAGAAAC-3′ and reverse, 5′-GTCTGCG TTCTCTTTCTCCAGC-3′; SERPINE2 forward, 5′-TTTGCA AAAATAACAACAGGGTCA-3′ and reverse, 5′-GCTGCTG AAGCTTTGGTTCC-3′; PLN forward, 5′-CAGGTCTTCACC AAGTATCA-3′ and reverse, 5′-TCCATACTTGATTCTC ATCA-3′; RasGRP3 forward, 5′-TCAGCCTCATCGACATAT CCA-3′ and reverse, 5′-TCAGCCAATTCAATG GGCTCC-3′; CCDC82 forward, 5′-GAGCTTGATAGTGAAGAATTT-3′ and reverse, 5′-CCGTTGCCAGAGTTAAT GAG-3′; SMAD2 forward, 5′-GGAGCAGAATACCGAA GGCA-3′ and reverse, 5′-CTTGAGCAACGCACTGAAGG-3′; SMAD3 forward, 5′-AGAAGACGGGGCAGCTGGAC-3′ and reverse, 5′-GAC ATCGGATTCGGGGATAG-3′; VDR forward, 5′-CGAC CCCACCTACTCCGACTT-3′ and reverse, 5′-GGCTCCC TCCACCATCATTC-3′; GAPDH forward, 5′-GGAGCG AGATCCCTCCAAAAT-3′ and GAPDH reverse, 5′-GGCT GTTGTCATACTTCTCATGG-3′.
Cells were lysed with RIPA Lysis and Extraction Buffer (Thermo Fisher Scientific) supplemented with the Protease Inhibitor Cocktail (Cell Signaling Technology, Beverly, MA, USA). Protein concentration was determined using the BCA Protein Assay Kit (Cell Signaling Technology). Protein samples were separated by sodium dodecyl sulfate-polyacrylamide gel electrophoresis and transferred to a polyvinylidene fluoride membrane. The membrane was then incubated at 4°C overnight with primary antibodies recognizing RasGRP3 (#3334; Cell Signaling Technology) or GAPDH (#5174; Cell Signaling Technology). After washing, the membrane was re-probed with secondary antibodies conjugated with horseradish peroxidase. Western blot bands were visualized using enhanced chemiluminescence reagents (Amersham GE Healthcare, Chicago, IL, USA) and subjected to densitometric analysis.
Plasmid details, small interfering RNAs, and cell transfection
Full-length human RasGRP3 cDNA was cloned in pcDNA3.1(+) vector. Small interfering RNAs (siRNAs) specifically targeting human RasGRP3, SMAD2, SMAD3, and VDR and non-specific control siRNAs were purchased from Santa Cruz Biotechnology (Santa Cruz, CA, USA).
For transient transfection, MRC5 cells were seeded into 6-well plates (1 × 106 cells/well) 24 h before transfection. The RasGRP3-expressing plasmid and siRNAs (50 nM) were transfected to MRC5 cells using Lipofectamine 3000 (Invitrogen) in accordance with the manufacturer’s protocol. After 24 h of transfection, the cells were subjected to gene expression analysis, proliferation, and migration assays.
For short term proliferation assays, 1 × 104 cells transfected with indicated constructs were seeded onto 12-well plates and cultured with or without TGF-β1 or vitamin D. After 3 or 5 days, the cells were harvested and counted using an automated cell counter.
For the assessment of cell migration, an in vitro wound-healing assay was performed. MRC5 cells were seeded onto 6-well plates and cultured to confluence. A sterile 10-μL pipette tip was used to make an artificial gap at the central part of the culture monolayer. The scratched cells were photographed at 0 and 24 h after scratching.
Transcription factor prediction
Putative transcription factors binding to the promoter region of the RasGRP3 gene were predicted using the Animal Transcription Factor DataBase (AnimalTFDB) version 3.0 (http://bioinfo.life.hust.edu.cn/AnimalTFDB/#!/) (Hu et al., 2019).
Chromatin immunoprecipitation (ChIP) assay
ChIP assays were performed as described previously (Liu et al., 2019). Briefly, MRC5 cells with indicated treatments were cross-linked with 1% formaldehyde and sonicated on ice to yield 200–500 bp DNA fragments. The fragmented chromatin was incubated with anti-SMAD2/SMAD3 (#5678; Cell Signaling Technology), anti-VDR (ab3508; Abcam, Cambridge, MA, USA), or isotype control IgG (Cell Signaling Technology). The precipitated chromatin DNA was recovered and subjected to qRT-PCR analysis. The PCR primers are shown as follows: RasGRP3 promoter, forward: 5′-CCATC CTGGCTAACATGGTGA-3′, and reverse: 5′-CGCTCATTA GCTAAACATGCT-3′.
Data are expressed as mean ± standard deviation (SD). All statistical analyses were performed using the Student’s t-test or one-way analysis of variance with a Tukey’s test. p < 0.05 was considered to be significant.
TGF-β1 stimulates the expression of RasGRP3 in human lung fibroblasts
To search for novel downstream genes mediating TGF-β1 profibrotic effects, we analyzed 2 publicly available datasets, i.e., GSE97829 and GSE110147. The GSE110147 dataset includes RNA profiling data from 22 IPF patients vs. 11 healthy controls. The GSE97829 dataset contains RNA sequencing data from human MRC5 lung fibroblasts treated with or without TGF-β1. The Venn diagram showed 54 differentially expressed genes (DEGs) commonly detected in both datasets (Fig. 1A). Among them, 6 genes (i.e., IGF1, MYH11, SERPINE2, PLN, RasGRP3, and CCDC82) were consistently upregulated in lung tissues from IPF patients and TGF-β1-treated fibroblasts. To validate the bioinformatic analysis, we performed qRT-PCR experiments using MRC5 cells after treatment with different concentrations of TGF-β1. While IGF1, MYH11, PLN, RasGRP3, and CCDC82 were induced by 1.6–5.1 fold upon TGF-β1 treatment, SERPINE2 levels remained unchanged (Fig. 1B and Suppl. Fig. S1). In this study, we focused on RasGRP3 because it showed the highest fold change after TGF-β1 treatment. Western blot analysis also confirmed the upregulation of RasGRP3 by TGF-β1 (Figs. 1C and 1D). These results suggest that RasGRP3 may mediate the effect of TGF-β1 on lung fibroblasts.
Figure 1: TGF-β1 stimulates the expression of RasGRP3 in human lung fibroblasts. (A) Venn diagram showing 54 differentially expressed genes commonly detected in the 2 publicly available datasets. (B) Expression of RasGRP3 was measured by qRT-PCR in MRC5 cells treated with TGF-β1. *p < 0.05 using one-way analysis of variance with a Tukey’s test (n = 3). (C) Western blot analysis of RasGRP3 protein levels in MRC5 cells treated with TGF-β1. Images were representative of three experiments. (D) Bar graph showing densitometry analysis of RasGRP3 protein in (C). Data are expressed as the fold change relative to 0 ng/mL TGF-β1. *p < 0.05 using one-way analysis of variance with a Tukey’s test (n = 3). Key: TGF-β1-transforming growth factor beta-1; RasGRP3: RAS guanyl releasing protein 3; qRT-PCR: quantitative real-time polymerase chain reaction.
RasGRP3 is required for TGF-β1-induced lung fibroblast proliferation and migration
Next, we investigated the role of RasGRP3 in modulating the behavior of lung fibroblasts. Overexpression of RasGRP3 enhanced the proliferation and migration of MRC5 cells (Figs. 2A–2C). Previous studies (Jin et al., 2019; Wang et al., 2021) have shown that treatment with TGF-β1 led to a significant increase in MRC5 cell proliferation and migration. Of note, the knockdown of RasGRP3 (Fig. 2D) reversed this TGF-β1-dependent proliferation and migration in MRC5 cells (Figs. 2E and 2F). These results indicate that RasGRP3 is capable of inducing a profibrotic phenotype in terms of increased cell proliferation and migration in lung fibroblasts.
Figure 2: RasGRP3 is required for TGF-β1-induced lung fibroblast proliferation and migration. (A) RasGRP3 protein levels determined by Western blot analysis in MRC5 cells transfected with vector or RasGRP3-expressing plasmid. Left: images were representative of three experiments. Right: densitometry analysis of RasGRP3 protein. *p < 0.05 using the Student’s t-test (n = 3). (B) Cell proliferation assay. *p < 0.05 using the Student’s t-test (n = 3). (C) Wound-healing assay was performed to measure MRC5 cell migration. *p < 0.05 using the Student’s t-test (n = 3). (D) qRT-PCR analysis of RasGRP3 mRNA levels in MRC5 cells transfected with control siRNA (siCtrl) or RasGRP3-targeting siRNAs (siRasGRP3). *p < 0.05 using the Student’s t-test (n = 3). (E) Cell proliferation assay. *p < 0.05 using one-way analysis of variance with a Tukey’s test (n = 3). (F) Cell migration assay. *p < 0.05 using one-way analysis of variance with a Tukey’s test (n = 3). Key: TGF-β1-transforming growth factor beta-1; RasGRP3: RAS guanyl releasing protein 3; qRT-PCR: quantitative real-time polymerase chain reaction; siRNA: small interfering RNA.
TGF-β1-SMAD2/3 signaling drives the transcription of RasGRP3
Since TGF-β1 treatment augmented the expression of RasGRP3 transcript (Fig. 1B), we hypothesized whether TGF-β1 signaling can directly initiate the transcription of RasGRP3. Using the AnimalTFDB 3.0 software, we predicted that the promoter region of the RasGRP3 gene harbored binding sites for 72 transcription factors. Intriguingly, there was one binding site for SMAD2/SMAD3: AAATACATG (-3446/-3437 bp) (Fig. 3A). ChIP assay confirmed the enrichment of SMAD2/SMAD3 at the promoter region of RasGRP3 (Fig. 3B). Knockdown of SMAD2 or SMAD3 (Fig. 3C) significantly impaired the TGF-β1-induced upregulation of RasGRP3 (Fig. 3D). These data indicate that TGF-β1 promotes RasGRP3 expression through SMAD2/SMAD3-dependent transcription.
Figure 3: TGF-β1-SMAD2/3 signaling drives the transcription of RasGRP3. (A) Using the AnimalTFDB 3.0 software, the promoter region of the RasGRP3 gene was predicted to have 1 binding site for SMAD2/SMAD3. (B) ChIP assays of SMAD2/SMAD3 on the promoter of RasGRP3 in MRC5 cells. *p < 0.05 using the Student’s t-test (n = 3). (C) Knockdown of SMAD2 and SMAD3 using specific siRNAs. *p < 0.05 using the Student’s t-test (n = 3). (D) qRT-PCR analysis of RasGRP3 mRNA in MCR5 cells with indicated treatments. *p < 0.05 using one-way analysis of variance with a Tukey’s test (n = 3). Key: TGF-β1-transforming growth factor beta-1; RasGRP3: RAS guanyl releasing protein 3; qRT-PCR: quantitative real-time polymerase chain reaction; ChIP: chromatin immunoprecipitation; siRNA: small interfering RNA.
Vitamin D blocks TGF-β1-mediated RasGRP3 upregulation through the VDR
Vitamin D has been shown to modulate TGF-β1/SMAD signaling in different biological settings (Subramaniam et al., 2001; Tao et al., 2015). Thus, we checked whether vitamin D could regulate the expression of RasGRP3. Interestingly, we found that vitamin D significantly inhibited the upregulation of RasGRP3 in TGF-β1-treated MRC5 cells (Fig. 4A). ChIP assay demonstrated that the enrichment of SMAD2/SMAD3 at the promoter region of RasGRP3 was impaired when treat MRC5 cells were treated with vitamin D (Fig. 4B). One putative VDR binding site, AATCAGAAA (-3250/-3241 bp) localized nearby the predicted SMAD2/SMAD3 binding site at the RasGRP3 promoter (Fig. 3A). Notably, vitamin D treatment promoted the enrichment of the VDR at the RasGRP3 promoter (Fig. 4C). In addition, the depletion of the VDR using specific siRNAs (Fig. 4D) abolished the suppression of RasGRP3 by vitamin D (Fig. 4E). Taken together, vitamin D prevents TGF-β1-induced RasGRP3 upregulation likely by interfering with the binding of SMAD2/SMAD3 to the RasGRP3 promoter.
Figure 4: Vitamin D blocks TGF-β1-mediated RasGRP3 upregulation through VDR. (A) MCR5 cells were treated with 100 nM 1,25(OH)2D or 99.8% ethanol (vehicle) 24 h before exposure to 10 ng/mL TGF-β1 for another 24 h. RasGRP3 mRNA levels were determined by qRT-PCR analysis. *p < 0.05 using one-way analysis of variance with a Tukey’s test (n = 3). (B) ChIP assays of SMAD2/SMAD3 on the promoter of RasGRP3 in MRC5 cells treated as in (A). *p < 0.05 using one-way analysis of variance with a Tukey’s test (n = 3). (C) ChIP assays of VDR on the promoter of RasGRP3 in MRC5 cells treated as in (A). *p < 0.05 using one-way analysis of variance with a Tukey’s test (n = 3). (D) Knockdown of VDR using specific siRNAs. *p < 0.05 using the Student’s t-test (n = 3). (E) qRT-PCR analysis of RasGRP3 mRNA in MCR5 cells with indicated treatments. *p < 0.05 using one-way analysis of variance with a Tukey’s test (n = 3). Key: TGF-β1-transforming growth factor beta-1; RasGRP3: RAS guanyl releasing protein 3; qRT-PCR: quantitative real-time polymerase chain reaction; ChIP: chromatin immunoprecipitation; siRNA: small interfering RNA; VDR: vitamin D receptor.
Overexpression of RasGRP3 antagonizes the anti-fibrotic effect of vitamin D on lung fibroblasts
Supplementation of vitamin D significantly attenuated the proliferation and migration of MRC5 cells treated with TGF-β1 (Figs. 5A and 5B). The exogenous expression of RasGRP3 restored MRC5 cell proliferation and migration in the presence of vitamin D (Figs. 5A–5C). These results suggest that the anti-fibrotic effect of vitamin D involves the modulation of RasGRP3.
Figure 5: Overexpression of RasGRP3 antagonizes the anti-fibrotic effect of vitamin D on lung fibroblasts. (A) Assessment of the proliferation of MRC5 cells with indicated treatments. *p < 0.05 using one-way analysis of variance with a Tukey’s test (n = 3). (B) Wound-healing assays were performed to determine the migration of MRC5 cells with indicated treatments. *p < 0.05 using one-way analysis of variance with a Tukey’s test (n = 3). (C) qRT-PCR analysis of RasGRP3 mRNA in MCR5 cells with indicated treatments. *p < 0.05 using one-way analysis of variance with a Tukey’s test (n = 3). Key: TGF-β1-transforming growth factor beta-1; RasGRP3: RAS guanyl releasing protein 3.
TGF-β1 is a potent cytokine that shows the ability to promote lung fibrosis (Mackinnon et al., 2012; Boutanquoi et al., 2020). Treatment with TGF-β1 is able to elicit genome-wide expression changes in a variety of cell types including lung fibroblasts (Lu et al., 2020). In this respect, it is important to screen for DEGs upon TGF-β1 treatment. In the current study, we show that RasGRP3 is stimulated by TGF-β1 in lung fibroblasts. Analysis of publically available data from 22 IPF patients vs. 11 healthy controls suggests that RasGRP3 is upregulated in lung tissues from IPF patients relative to healthy controls. This upregulation of RasGRP3 may contribute to IPF progression. RasGRP3 belongs to the RASGRP family of guanine nucleotide exchange factors (GEFs). Many studies have reported the role of RasGRP3 in malignant diseases (Yang et al., 2010; Lee et al., 2015). For example, RasGRP3 was highly expressed in glioblastoma and accelerated cell migration and invasion (Lee et al., 2015). Yang et al. (2010) reported that the knockdown of RasGRP3 inhibited prostate cancer cell proliferation and migration. RasGRP3 also regulates the behaviors of nonmalignant cells such as endothelial cells (Randhawa et al., 2011) and macrophages (Tang et al., 2014). Randhawa et al. (2011) reported that activation of RasGRP3 impairs endothelial cell migration. Using lung fibroblasts, we demonstrate in this work that RasGRP3 mediates the TGF-β1-induced profibrotic phenotype. Knockdown of RasGRP3 attenuated the proliferation and migration of lung fibroblasts stimulated by TGF-β1. Therefore, our work highlights the importance of RasGRP3 in regulating the phenotype of lung fibroblasts. However, it should be noted that the cell line MRC5 used in this study is a fetal fibroblast cell line. Given that IPF is a condition affecting older adults, the present results seen in MRC5 cells should be validated in adult human lung fibroblasts.
Bioinformatic analysis reveals that the promoter of the RasGRP3 gene contains a putative binding site for SMAD2/SMAD3. When SMAD2 or SMAD3 is depleted, TGF-β1-induced upregulation of RasGRP3 is reversed. These data suggest that TGF-β1 stimulates RasGRP3 upregulation likely through SMAD2/SMAD3-dependent transcription. Vitamin D has been reported to antagonize TGF-β signaling in multiple cell types such as human hepatic stellate cells (Beilfuss et al., 2015), fibroblasts, and epithelial cells (Ramirez et al., 2010). Xu et al. (2020) provided evidence for the physical interaction between VDR and SMAD3. Our data demonstrate that vitamin D treatment suppresses TGF-β1-induced upregulation of RasGRP3. Moreover, vitamin D prevents SMAD2/SMAD3 binding to the promoter of RasGRP3. Instead, the enrichment of the VDR at the promoter of RasGRP3 is potentiated by vitamin D. Ablation of the VDR reverses the suppression of RasGRP3 by vitamin D. These results suggest that activated VDR may interfere with SMAD2/SMAD3 binding to the promoter of RasGRP3, thus inhibiting RasGRP3 transcription.
A number of molecular mechanisms have been suggested to be involved in the anti-fibrotic effects of vitamin D (Liu et al., 2014; Chang et al., 2021; Zhu et al., 2021). Zhu et al. (2021) reported that VDR binding to the promoter of PSAT1 leads to the downregulation of phosphoserine amino transferase 1 (PSAT1), consequently inhibiting the mitogen-activated protein kinase (MAPK) pathway and preventing lung fibrosis. Chang et al. (2021) demonstrated that vitamin D suppresses lung fibrosis through the regulation of the local renin-angiotensin system in the lung. In this study, our data show a novel mechanism by which vitamin D regulates lung fibroblast activation. We demonstrated that vitamin D suppresses the transcription of RasGRP3 to block lung fibroblast proliferation and migration. Ectopic expression of RasGRP3 reverses the anti-fibrotic effect of vitamin D on lung fibroblasts. Vitamin D-mediated suppression of RasGRP3 is dependent on VDR. Ding et al. (2013) reported a receptor-dependent mechanism for the suppression of liver fibrosis by vitamin D. They found that in the presence of vitamin D, VDR binding interferes with SMAD3 occupancy at the promoter of profibrotic genes, thus inhibiting liver fibrosis. Our results reveal a similar mechanism in the context of lung fibroblast activation. Taken together, vitamin D counteracts TGF-β1-induced lung fibroblast proliferation and migration through VDR-mediated repression of RasGRP3 transcription.
Despite these in vitro findings, there is no direct evidence for the role of RasGRP3 in animal models of IPF yet. It is still unclear whether RasGRP3 could be used as a biomarker for IPF diagnosis and prognosis. In addition, it remains to be explored in future work how RasGRP3 regulates lung fibrosis.
In summary, our data suggest RasGRP3 as a novel mediator of TGF-β1 profibrotic activity in lung fibroblasts. Vitamin D can reduce TGF-β1-induced lung fibroblast activation, which is at least partially mediated through inhibition of SMAD2/SMAD3 occupancy and VDR enrichment at the promoter of RasGRP3. The significance of RasGRP3 in IPF deserves further investigation.
Funding Statement: This work was supported by the Basic Scientific Research Project of Wenzhou of China (Y20210068) and the Collaborative Education Project of Industry-University Cooperation of the Ministry of Education of China (202101160012).
Author Contributions: GQH, RSH, and XXC designed the study and analyzed the data. GQH, RSH, LLL, and QSP conducted experiments and collected data. GQH was responsible for manuscript drafting. All authors read and approved the final draft of the manuscript.
Availability of Data and Materials: All data generated or analyzed during this study are included in this published article.
Ethics Approval: Not applicable.
Conflicts of Interest: The authors declare that they have no conflicts of interest to report regarding the present study.
References
Ballester B, Milara J, Montero P, Cortijo J (2021). MUC16 is overexpressed in idiopathic pulmonary fibrosis and induces fibrotic responses mediated by transforming growth factor-β1 canonical pathway. International Journal of Molecular Sciences 22: 6502. https://doi.org/10.3390/ijms22126502 [Google Scholar] [PubMed] [CrossRef]
Beilfuss A, Sowa JP, Sydor S, Beste M, Bechmann LP et al. (2015). Vitamin D counteracts fibrogenic TGF-β signalling in human hepatic stellate cells both receptor-dependently and independently. Gut 64: 791–799. https://doi.org/10.1136/gutjnl-2014-307024 [Google Scholar] [PubMed] [CrossRef]
Boutanquoi PM, Burgy O, Beltramo G, Bellaye PS, Dondaine L et al. (2020). TRIM33 prevents pulmonary fibrosis by impairing TGF-β1 signalling. The European Respiratory Journal 55: 1901346. https://doi.org/10.1183/13993003.01346-2019 [Google Scholar] [PubMed] [CrossRef]
Chang J, Nie H, Ge X, Du J, Liu W, Li X, Sun Y, Wei X, Xun Z, Li YC (2021). Vitamin D suppresses bleomycin-induced pulmonary fibrosis by targeting the local renin-angiotensin system in the lung. Scientific Reports 11: 16525. https://doi.org/10.1038/s41598-021-96152-7 [Google Scholar] [PubMed] [CrossRef]
Chen CY, Tung HY, Tseng YF, Huang JS, Shi LS, Ye YL (2022). Verbascoside and isoverbascoside ameliorate transforming growth factor β1-induced collagen expression by lung fibroblasts through Smad/non-Smad signaling pathways. Life Sciences 308: 120950. https://doi.org/10.1016/j.lfs.2022.120950 [Google Scholar] [PubMed] [CrossRef]
Cui H, Xie N, Banerjee S, Dey T, Liu RM et al. (2022). CD38 mediates lung fibrosis by promoting alveolar epithelial cell aging. American Journal of Respiratory and Critical Care Medicine 206: 459–475. https://doi.org/10.1164/rccm.202109-2151OC [Google Scholar] [PubMed] [CrossRef]
Ding N, Yu RT, Subramaniam N, Sherman MH, Wilson C et al. (2013). A vitamin D receptor/SMAD genomic circuit gates hepatic fibrotic response. Cell 153: 601–613. https://doi.org/10.1016/j.cell.2013.03.028 [Google Scholar] [PubMed] [CrossRef]
Faverio P, Fumagalli A, Conti S, Madotto F, Bini F et al. (2022). Nutritional assessment in idiopathic pulmonary fibrosis: A prospective multicentre study. ERJ Open Research 8: 00443-2021. https://doi.org/10.1183/23120541.00443-2021 [Google Scholar] [PubMed] [CrossRef]
Han H, Chung SI, Park HJ, Oh EY, Kim SR, Park KH, Lee JH, Park JW (2021). Obesity-induced vitamin D deficiency contributes to lung fibrosis and airway hyperresponsiveness. American Journal of Respiratory Cell and Molecular Biology 64: 357–367. https://doi.org/10.1165/rcmb.2020-0086OC [Google Scholar] [PubMed] [CrossRef]
Hu H, Miao YR, Jia LH, Yu QY, Zhang Q, Guo AY (2019). AnimalTFDB 3.0: A comprehensive resource for annotation and prediction of animal transcription factors. Nucleic Acids Research 47: D33–D38. https://doi.org/10.1093/nar/gky822 [Google Scholar] [PubMed] [CrossRef]
Jeong SH, Son ES, Lee YE, Kyung SY, Park JW, Kim SH (2022). Histone deacetylase 3 promotes alveolar epithelial-mesenchymal transition and fibroblast migration under hypoxic conditions. Experimental & Molecular Medicine 54: 922–931. https://doi.org/10.1038/s12276-022-00796-y [Google Scholar] [PubMed] [CrossRef]
Jiang S, Gu L, Hu Y, Ren Y, Yang Z, Chai C, Yu B, Ge H, Cao Z, Zhao F (2022). Inhibition of TRPC6 suppressed TGFβ-induced fibroblast-myofibroblast transdifferentiation in renal interstitial NRK-49F cells. Experimental Cell Research 421: 113374. https://doi.org/10.1016/j.yexcr.2022.113374 [Google Scholar] [PubMed] [CrossRef]
Jiang H, Zhao Y, Tang H, Duan S, Li M et al. (2021). Tetraethylthiuram disulphide alleviates pulmonary fibrosis through modulating transforming growth factor-β signalling. Pharmacological Research 174: 105923. https://doi.org/10.1016/j.phrs.2021.105923 [Google Scholar] [PubMed] [CrossRef]
Jin J, Togo S, Kadoya K, Tulafu M, Namba Y et al. (2019). Pirfenidone attenuates lung fibrotic fibroblast responses to transforming growth factor-β1. Respiratory Research 20: 119. https://doi.org/10.1186/s12931-019-1093-z [Google Scholar] [PubMed] [CrossRef]
Karkeni E, Marcotorchino J, Tourniaire F, Astier J, Peiretti F, Darmon P, Landrier JF (2015). Vitamin D limits chemokine expression in adipocytes and macrophage migration in vitro and in male mice. Endocrinology 156: 1782–1793. https://doi.org/10.1210/en.2014-1647 [Google Scholar] [PubMed] [CrossRef]
Lee HK, Finniss S, Cazacu S, Xiang C, Poisson LM, Blumberg PM, Brodie C (2015). RasGRP3 regulates the migration of glioma cells via interaction with Arp3. Oncotarget 6: 1850–1864. https://doi.org/10.18632/oncotarget.2575 [Google Scholar] [PubMed] [CrossRef]
Liu C, Chang HM, Yi Y, Fang Y, Zhao F, Leung PCK, Yang X (2019). ALK4-SMAD2/3-SMAD4 signaling mediates the activin A-induced suppression of PTX3 in human granulosa-lutein cells. Molecular and Cellular Endocrinology 493: 110485. https://doi.org/10.1016/j.mce.2019.110485 [Google Scholar] [PubMed] [CrossRef]
Liu X, Nelson A, Wang X, Farid M, Gunji Y, Ikari J, Iwasawa S, Basma H, Feghali-Bostwick C, Rennard SI (2014). Vitamin D modulates prostaglandin E2 synthesis and degradation in human lung fibroblasts. American Journal of Respiratory Cell and Molecular Biology 50: 40–50. https://doi.org/10.1165/rcmb.2013-0211OC [Google Scholar] [PubMed] [CrossRef]
Lu F, Sun X, Xu X, Jiang X (2020). SILAC-based proteomic profiling of the suppression of TGF-β1-induced lung fibroblast-to-myofibroblast differentiation by trehalose. Toxicology and Applied Pharmacology 391: 114916. https://doi.org/10.1016/j.taap.2020.114916 [Google Scholar] [PubMed] [CrossRef]
Mackinnon AC, Gibbons MA, Farnworth SL, Leffler H, Nilsson UJ et al. (2012). Regulation of transforming growth factor-β1-driven lung fibrosis by galectin-3. American Journal of Respiratory and Critical Care Medicine 185: 537–546. https://doi.org/10.1164/rccm.201106-0965OC [Google Scholar] [PubMed] [CrossRef]
Meng XM, Nikolic-Paterson DJ, Lan HY (2016). TGF-β: The master regulator of fibrosis. Nature Reviews Nephrology 12: 325–338. https://doi.org/10.1038/nrneph.2016.48 [Google Scholar] [PubMed] [CrossRef]
Ramirez AM, Wongtrakool C, Welch T, Steinmeyer A, Zügel U, Roman J (2010). Vitamin D inhibition of pro-fibrotic effects of transforming growth factor beta1 in lung fibroblasts and epithelial cells. The Journal of Steroid Biochemistry and Molecular Biology 118: 142–150. https://doi.org/10.1016/j.jsbmb.2009.11.004 [Google Scholar] [PubMed] [CrossRef]
Randhawa PK, Rylova S, Heinz JY, Kiser S, Fried JH et al. (2011). The Ras activator RasGRP3 mediates diabetes-induced embryonic defects and affects endothelial cell migration. Circulation Research 108: 1199–1208. https://doi.org/10.1161/CIRCRESAHA.110.230888 [Google Scholar] [PubMed] [CrossRef]
Rochel N (2022). Vitamin D and its receptor from a structural perspective. Nutrients 14: 2847. https://doi.org/10.3390/nu14142847 [Google Scholar] [PubMed] [CrossRef]
Schmittgen TD, Livak KJ (2008). Analyzing real-time PCR data by the comparative C(T) method. Nature Protocols 3: 1101–1108. https://doi.org/10.1038/nprot.2008.73 [Google Scholar] [PubMed] [CrossRef]
Staab-Weijnitz CA (2022). Fighting the fiber: Targeting collagen in lung fibrosis. American Journal of Respiratory Cell and Molecular Biology 66: 363–381. https://doi.org/10.1165/rcmb.2021-0342TR [Google Scholar] [PubMed] [CrossRef]
Stio M, Celli A, Lunghi B, Raugei G, Modesti A, Treves C (1997). Vitamin D receptor in IMR-90 human fibroblasts and antiproliferative effect of 1,25-dihydroxyvitamin D3. Biochemistry and Molecular Biology International 43: 1173–1181. https://doi.org/10.1080/15216549700205011 [Google Scholar] [PubMed] [CrossRef]
Subramaniam N, Leong GM, Cock TA, Flanagan JL, Fong C, Eisman JA, Kouzmenko AP (2001). Cross-talk between 1,25-dihydroxyvitamin D3 and transforming growth factor-beta signaling requires binding of VDR and Smad3 proteins to their cognate DNA recognition elements. The Journal of Biological Chemistry 276: 15741–15746. https://doi.org/10.1074/jbc.M011033200 [Google Scholar] [PubMed] [CrossRef]
Tang S, Chen T, Yu Z, Zhu X, Yang M, Xie B, Li N, Cao X, Wang J (2014). RasGRP3 limits toll-like receptor-triggered inflammatory response in macrophages by activating Rap1 small GTPase. Nature Communications 5: 4657. https://doi.org/10.1038/ncomms5657 [Google Scholar] [PubMed] [CrossRef]
Tao Q, Wang B, Zheng Y, Jiang X, Pan Z, Ren J (2015). Vitamin D prevents the intestinal fibrosis via induction of vitamin D receptor and inhibition of transforming growth factor-beta1/Smad3 pathway. Digestive Diseases and Sciences 60: 868–875. https://doi.org/10.1007/s10620-014-3398-6 [Google Scholar] [PubMed] [CrossRef]
Tzilas V, Bouros E, Barbayianni I, Karampitsakos T, Kourtidou S, Ntassiou M, Ninou I, Aidinis V, Bouros D, Tzouvelekis A (2019). Vitamin D prevents experimental lung fibrosis and predicts survival in patients with idiopathic pulmonary fibrosis. Pulmonary Pharmacology & Therapeutics 55: 17–24. https://doi.org/10.1016/j.pupt.2019.01.003 [Google Scholar] [PubMed] [CrossRef]
Wang F, Wang S, Zhang C, Tian X, Zhou Y, Xuan W, Matteson EL, Luo F, Tschumperlin DJ, Vassallo R (2022). Non-canonical JAK1/STAT3 interactions with TGFβ modulate myofibroblast trans-differentiation and fibrosis. American Journal of Physiology. Lung Cellular and Molecular Physiology 323: L698–L714. https://doi.org/10.1152/ajplung.00428.2021 [Google Scholar] [PubMed] [CrossRef]
Wang Y, Yang H, Su X, Cao A, Chen F, Chen P, Yan F, Hu H (2021). TGF-β1/SMOC2/AKT and ERK axis regulates proliferation, migration, and fibroblast to myofibroblast transformation in lung fibroblast, contributing with the asthma progression. Hereditas 158: 47. https://doi.org/10.1186/s41065-021-00213-w [Google Scholar] [PubMed] [CrossRef]
Xu S, Zhang ZH, Fu L, Song J, Xie DD, Yu DX, Xu DX, Sun GP (2020). Calcitriol inhibits migration and invasion of renal cell carcinoma cells by suppressing Smad2/3-, STAT3- and β-catenin-mediated epithelial-mesenchymal transition. Cancer Science 111: 59–71. https://doi.org/10.1111/cas.14237 [Google Scholar] [PubMed] [CrossRef]
Yang D, Kedei N, Li L, Tao J, Velasquez JF et al. (2010). RasGRP3 contributes to formation and maintenance of the prostate cancer phenotype. Cancer Research 70: 7905–7917. https://doi.org/10.1158/0008-5472.CAN-09-4729 [Google Scholar] [PubMed] [CrossRef]
Zheng Q, Cox IA, Campbell JA, Xia Q, Otahal P, de Graaff B, Corte TJ, Teoh AKY, Walters EH, Palmer AJ (2022). Mortality and survival in idiopathic pulmonary fibrosis: A systematic review and meta-analysis. ERJ Open Research 8: 00591-2021. https://doi.org/10.1183/23120541.00591-2021 [Google Scholar] [PubMed] [CrossRef]
Zhong FJ, Sun B, Cao MM, Xu C, Li YM, Yang LY (2021). STMN2 mediates nuclear translocation of Smad2/3 and enhances TGFβ signaling by destabilizing microtubules to promote epithelial-mesenchymal transition in hepatocellular carcinoma. Cancer Letters 506: 128–141. https://doi.org/10.1016/j.canlet.2021.03.001 [Google Scholar] [PubMed] [CrossRef]
Zhu W, Ding Q, Wang L, Xu G, Diao Y, Qu S, Chen S, Shi Y (2021). Vitamin D3 alleviates pulmonary fibrosis by regulating the MAPK pathway via targeting PSAT1 expression in vivo and in vitro. International Immunopharmacology 101: 108212. https://doi.org/10.1016/j.intimp.2021.108212 [Google Scholar] [PubMed] [CrossRef]
Supplementary Materials
Figure S1: Effect of TGF-β1 on the expression of indicated genes. Quantification of indicated transcripts in MRC5 cells treated with different concentrations of TGF-β1 using qRT-PCR assays. *p < 0.05 using one-way analysis of variance with a Tukey’s test (n = 3).
Cite This Article
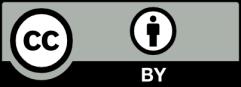