Open Access
ARTICLE
Blue LED promotes the chemosensitivity of human hepatoma to Sorafenib by inducing DNA damage
1 Department of Pharmacy (The University Key Laboratory of Drug Research, Heilongjiang Province), The Second Affiliated Hospital of Harbin Medical University, Harbin, China
2 Department of General Surgery, The First Affiliated Hospital of Harbin Medical University, Harbin, China
3 National Key Laboratory of Frigid Cardiovascular Disease, Harbin, China
4 Department of Pharmacology (The State-Province Key Laboratories of Biomedicine Pharmaceutics of China, Key Laboratory of Cardiovascular Research, Ministry of Education), College of Pharmacy, Harbin Medical University, Harbin, China
5 Department of Clinical Pharmacology, College of Pharmacy, Harbin Medical University, Harbin, China
* Corresponding Authors: LINQIANG LI. Email: ; YE YUAN. Email:
# Tong Wang and Jinhuan Hong contributed equally to this work
(This article belongs to the Special Issue: Application of Deep Learning in Cancer)
BIOCELL 2023, 47(8), 1811-1820. https://doi.org/10.32604/biocell.2023.029120
Received 01 February 2023; Accepted 14 April 2023; Issue published 28 August 2023
Abstract
Background: Phototherapies based on sunlight, infrared, ultraviolet, visible, and laser-based treatments present advantages like high curative effects, small invasion, and negligible adverse reactions in cancer treatment. We aimed to explore the potential therapeutic effects of blue light emitting diode (LED) in human hepatoma cells and decipher the underlying cellular and molecular mechanisms. Methods: Wound healing and transwell assays were employed to probe the inhibition of the invasion and migration of hepatocellular carcinoma cells in the presence of blue LED. The sphere-forming test was used to evaluate the effect of LED blue light irradiation on cancer stem cell properties. Immunofluorescence and western blotting were used to detect the changes in γ-H2AX. The Cell Counting Kit-8 assay, 5-ethynyl-2′-deoxyuridine staining, and colony formation assay were used to detect the combined effect of blue LED and sorafenib on cell proliferation inhibition. Results: We demonstrated that the irradiation of blue LED light in hepatoma cells could lead to cell proliferation reduction along with the increase of cell apoptosis. Simultaneously, blue LED irradiation also markedly suppressed the migration and invasion ability of human hepatoma cells. Sphere formation analysis further revealed the decreased cancer stemness of hepatoma cells upon blue LED irradiation. Mechanistically, blue LED irradiation significantly promoted the expression of the phosphorylation of the core histone protein H2AX (γ-H2AX), a sensitive molecular marker of DNA damage. In addition, we found that the combined treatment of blue LED irradiation and sorafenib increased cancer cell sensitivity to sorafenib. Conclusion: Collectively, we demonstrated that blue LED irradiation exhibited anti-tumor effects on liver cancer cells by inducing DNA damage and could enhance chemosensitivity of cancer cells, which represents a potential approach for human hepatoma treatment.Graphic Abstract
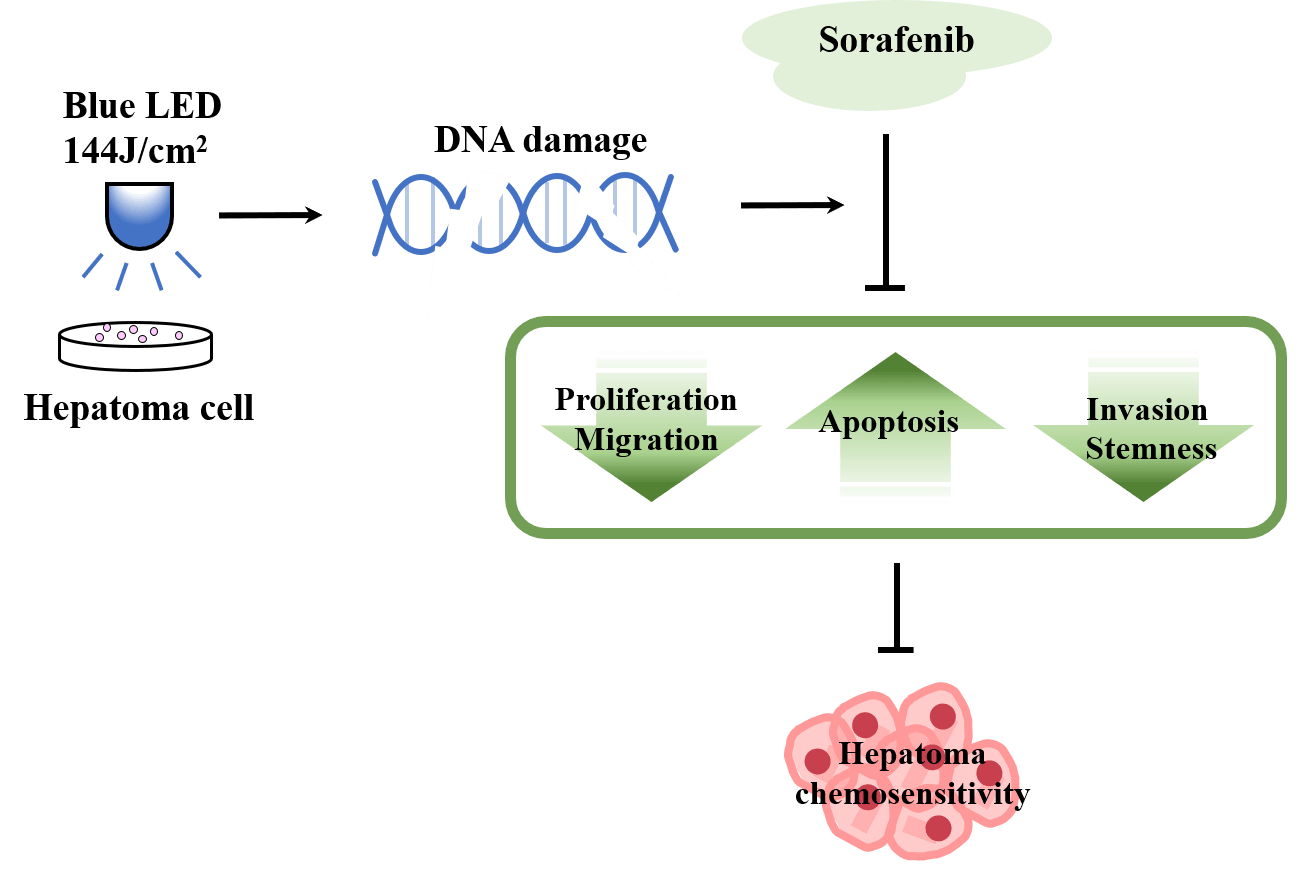
Keywords
Phototherapy is a promising medical technique, where sunlight or artificial light including infrared, ultraviolet, visible light, and laser radiations are used to prevent and treat diseases or promote physical rehabilitation. Light emitting diode (LED) is being studied as the preferred light source for phototherapy as a result of its affordability and none or minimal side effects across various wavelengths. Previous studies have shown that various levels of light exposure would cause different biological effects (Migliario et al., 2018; Costa et al., 2019; Oh and Jeong, 2019). Recent reports also discovered that LED treatment could significantly ameliorate lung inflammation, inhibit human gingiva fibroblast cell proliferation, and facilitate post-surgical recovery and wound healing (Barolet and Boucher, 2008; Erdle et al., 2008; Yoshida et al., 2013; Siqueira et al., 2017). Notable among the various types of LED is the blue LED including wavelengths of 430–490 nm, which has shown excellent antitumor activity in vitro and in vivo experiments. Blue light could effectively inhibit the tumor progression of skin tumors, melanoma, leukemia, and colon cancer mainly by the accumulation of intracellular reactive oxygen species (ROS) (Oh et al., 2017; Ohara et al., 2002, 2003, 2004). Consistent with these findings, our previous study demonstrated that blue LED could cause autophagic cell death in human osteosarcoma through increasing ROS generation and dephosphorylating epidermal growth factor receptor (EGFR) (He et al., 2021). Furthermore, blue LED irradiation could enhance the anti-tumor effects of arsenic trioxide on human osteosarcoma and cause cell death in colorectal cancer by increasing ROS accumulation and DNA damage-mediated p53 activation (Yan et al., 2018; Feng et al., 2019). Although blue LED showed such promising anti-tumor effects in the above studies, the potential effects of blue LED irradiation on liver cancer remain unclear.
Liver cancer, the second leading cause of cancer mortality in the world, is most prevalent in Asia and Africa with over forty percent of new cases and deaths occurring in China (Bray et al., 2018; Chen et al., 2018; Petrick et al., 2020). Earlier research has illuminated that the aberrant activation of the wnt/β-catenin signaling pathway, the mammalian target of rapamycin (mTOR) pathway, metabolic pathways, and liver tumor microenvironment (TME) play important roles in significantly the molecular pathogenesis of liver cancer (Ringelhan et al., 2018; Satriano et al., 2019; Jung et al., 2021; Lu et al., 2021). However, the limitation of current treatments for liver cancer and the phenomenon of resistance to sorafenib are becoming increasingly common, suggesting that further exploration of the molecular mechanisms of hepatocarcinogenesis and chemoresistance is urgently needed to develop new treatment strategies.
In the current study, we explored the role of various intensities of blue LED on hepatoma cells including HepG2 and Hep3B cell lines. We found that blue LED could suppress the proliferation, aggregation, migration, and invasion of HepG2 and Hep3B cells along with the promotion of cell apoptosis via DNA damage, impacting the cell sensitivity to sorafenib.
Hepatoma carcinoma cell lines Hep3B and HepG2 were purchased from Fenghui Biotechnology, China. The passage number of cells used for the study was within 15 passages. Cells were cultivated in Dulbecco’s modified Eagle medium (DMEM) (Life Technologies Corporation, California, USA) containing 4,500 mg/L glucose supplemented with 10% fetal bovine serum (FBS). Sorafenib (5 μM) was purchased from MCE (HY-10201, USA).
Light emitting diode irradiation
At room temperature, blue light (peak at 470 nm) was irradiated at 0, 72, 144, 216 and 288 J/cm2 for 15, 30, 45 and 60 min, respectively.
Cells were plated at a density of 1 × 106 glass bottom cell culture dish (NEST, Hong Kong, China). When the cell confluence reached about 70%, blue light irradiation was performed for 15, 30, 45 and 60 min at 0, 72, 144, 216 and 288 J/cm2, respectively. The cells were digested by trypsin to prepare a single-cell suspension. The cell suspension was mixed with 0.4% trypan blue solution (Biosharp, BL627A, Shanghai, China) at a 9:1 ratio, and the living and dead cells were counted respectively in 3 min. Microscopically, dead cells are stained with a blue color, while living cells are colorless and transparent. The formula used was: Dead cell rate (%) = total number of dead cells/(total number of living cells + total number of dead cells) × 100% (Feng et al., 2019).
5-ethynyl-2′-deoxyuridine (EdU) cell proliferation assay
The EdU Apollo DNA in vitro kit (Ribobio, #C10310-1, Guangzhou, China) was used for this staining. Cells were plated into a glass-bottom cell culture dish (NEST, #801002, Hong Kong, China) at a density of 2.0 × 105 cells/mL. Irradiation with 0 and 144 J/cm2 blue light was done for 30 min, or treatment with sorafenib 5 μM for 24 h was done. The cells were fixed with 4% paraformaldehyde (m/v) for 30 min and followed by incubation with 30 μM of EdU at 37°C for 90 min. After the cells were permeabilized in 0.5% Triton X-100, the Apollo staining solution was added into the cell culture medium for 30 min in the dark. Finally, the cells were incubated with 20 μg/mL 4′,6-diamidino-2-phenylindole (DAPI) for 10 min. Finally, cells were placed under the confocal laser scanning microscope (FV10i, Olympus, Tokyo, Japan) for imaging. The EdU index (%) was the average ratio of EdU-positive cells to total cells in 5 randomly selected regions (Yuan et al., 2021).
Cells were plated into 6-well culture plates at a density of 2.5 × 105 cells/mL. When the confluence of cells reached 70%, a wound was created by scraping the cells with a 200 μL pipette tip, and three scratches were evenly distributed in each well plate. The cells were rinsed with PBS and then the medium was replaced with fresh medium. Cells were kept in a humidified incubator. Subsequently, cells were treated with blue LED irradiation. Wound healing was monitored at 0, 24, 48, and 72 h with standard light microscopy (Eclipse TS100, Nikon, Japan). The wound area was measured using ImageJ software (National Institutes of Health (NIH), USA) (He et al., 2021).
HepG2 cells and Hep3B cells were seeded in 6-well cell culture plates at 1500 cells/well, respectively, and suspended in a medium containing 10% FBS and incubated for 24 h. According to the experimental requirements, the experimental groups were treated with blue light for 30 min or sorafenib for 24 h when the cell confluence reached 70%. The indicated cells were cultured in a humidified atmosphere containing 5% CO2 at a constant temperature of 37°C to form colonies for 14 days. Colonies were stained with crystal violet after being fixed with formaldehyde. Different colony morphologies were captured under a light microscope (Eclipse TS100, Nikon, Japan) (Zhao et al., 2018).
Logarithmic growth stage cells were seeded in a 96-well plate at a density of 1500 cells per well at 37°C, 5% CO2 and cultured for 24 h. Treatments included the sorafenib group, the sorafenib combined with the blue LED group, and the LED group. After 24 h, treated cells were then incubated with 10 μL CCK8 for 90 min. The absorbance was read at 450 nm using a spectrophotometer (Tecan, Infinite 200 PRO, Männedorf, Switzerland) (Du et al., 2020).
Propidium iodide (PI)/hoechst 33342 staining
PI/Hoechst 33342 staining was performed using the Hoechst 33342/PI Double Staining Kit (Solarbio Science, CA1120, Beijing China). Cells were plated into a glass-bottom cell culture dish (NEST, Hong Kong, China) at a density of 2.0 × 105 till the cells were evenly grown on the bottom of the culture dish. They were irradiated with blue LED at room temperature for 30 min at 0 and 144 J/cm2. Samples were collected for staining on the third day after cell inoculation. Cells were fixed with paraformaldehyde, and stained with the dye solution at room temperature and away from light. Finally, images of the staining were captured by a confocal laser scanning microscope (FV10i, Olympus, Tokyo, Japan) (Yu et al., 2019).
A 24 mm Transwell® chamber (Corning, #3412, USA) was used to detect cell invasion abilities. Matrigel was diluted with PBS (1:8) and coated on the upper surface of the membrane at the bottom of the chamber. After 12 h of cell starvation, the cells were digested with trypsin and resuspended in serum-free medium at a cell density of 5 × 104 cells/mL. Further, 1 × 104 cells were added to the upper compartment, and 300 μL of DMEM medium with 10% FBS was added to the lower compartment and subsequent blue light irradiation of 0 and 144 J/cm2. After 24 h, cells that migrated through the membrane were stained with 0.1% crystal violet (Beyotime Biotechnology, #C0121, China) and counted using light microscopy (Eclipse TS100, Nikon, Japan) (Yuan et al., 2021).
A total of 1000 hepatoma cells were plated in ultralow attachment plates. The cells were cultured for 10 days in DMEM/F12 medium (Invitrogen, Shanghai, China) supplemented with 4 mg/mL insulin (Sigma, Shanghai, China), B27 (1:50, Gibco, Shanghai, China), 20 ng/mL epidermal growth factor (EGF) (Sigma, Shanghai, China) and 20 ng/mL basic fibroblast growth factor (FGF) (Sigma, Shanghai, China). Liver cancer cells in good condition were digested with trypsin, centrifuged, washed with PBS, and resuspended with the prepared stem cell culture medium. The cells were cultured in six-well plates with ultra-low attachment surface for 10 days, during which the cells were irradiated with blue light at 0 and 144 J/cm2. Finally, they were counted using light microscopy (Eclipse TS100, Nikon, Japan) (Park et al., 2020).
Immunofluorescence experiments
Cells were plated at a density of 2 × 105 cells/mL. The next day, cells were irradiated by blue LED of 0 and 144 J/cm2 for 30 min. Samples were fixed with 4% paraformaldehyde, followed by permeabilization with 0.1% Triton X-100 at room temperature, and sealed with goat serum albumin. The cells were incubated with primary antibody (γ-H2AX; Affinity, AF6823, China) and secondary antibody (ZSGB-BIO, ZF-0511, China) and stained with DAPI in the dark for 20 min. After staining, the images were observed under a laser confocal microscope (FV10i, Olympus, Tokyo, Japan) and randomly selected for imaging. Cells were quantified using Image-Pro Plus software (Media Cybernetics, Silver Spring, MD) (Feng et al., 2019).
Cells were uniformly plated (4 × 105 cells/mL) at the bottom of a cell bottle for adherent growth for 24 h. The next day after cell irradiation by blue light of 0 and 144 J/cm2 for 30 min, the protein content was extracted with RIPA buffer (Beyotime Biotechnology, P0013B, China). Protein fractions were collected by centrifugation at 13,500 rpm for 15 min, and then supernatants were heated with sodium dodecyl sulfate (SDS) buffer at 100°C for 4 min. Proteins were separated by 10% sodium dodecyl sulfate polyacrylamide gel electrophoresis and transferred to a polyvinylidene difluoride membrane. The blots were then blocked with 5% non-fat milk powder in PBST buffer for 1 h and incubated at 4°C overnight with the appropriate primary antibodies at appropriate dilutions. After washing, the blots were incubated with the HRP-conjugated secondary antibody for 1 h at room temperature. The primary antibody used was phosphorylation of the core histone protein H2AX (γ-H2AX; ABclonal, AP0099, China). β-actin was used as the internal control (Abcam, ab6276, China) (Cenni et al., 2020).
Data are expressed as mean ± SEM. Statistical analysis was performed using GraphPad Prism8 software and analyzed with Student’s t-test (two-tailed). All experiments were independently repeated at least three times and similar results were obtained. The p-values were as follows: *p < 0.05; **p < 0.01; ***p < 0.001.
Blue light emitting diode irradiation inhibited cell viability and promoted apoptosis of hepatocellular carcinoma (HCC) cells
To investigate whether the irradiation of blue LED could affect HCC cell growth, the morphologic changes of HCC cells were observed. HepG2 and Hep3B cell lines were irradiated with different doses of blue LEDs of 0, 72, 144, 216, and 288 J/cm2. As exhibited in Fig. 1A, with the higher light intensity, the more damaged cell morphology was observed. This was seen as a decrease in viable cells, as well as an increase in the ratio of dead cells upon the treatment of blue LED irradiation (Fig. 1B).
Figure 1: Blue light emitting diode (LED) exposure reduced the viability of hepatoma cells. (A) The representative images of hepatoma cells irradiated with blue LED light. (B) The number of live cells was counted by Trypan blue exclusion. Hep3B cells and HepG2 cells were irradiated with blue LED for 0 and 144 J/cm2, respectively (Scale bar: 200 μm). Data are expressed as the mean ± SEM. *p < 0.05; **p < 0.01; ***p < 0.001.
Furthermore, the EdU staining assay was used to assess the effects of blue LED irradiation on the cell proliferation ability of HepG2 and Hep3B cells with or without blue LED irradiation. Compared with non-irradiated control cells, EdU-positive cells in the irradiation group decreased from about 40% to about 10%, indicating significant suppression of the proliferative ability of HepG2 and Hep3B cells under the blue LED irradiation (Figs. 2A and 2B). The PI/Hoechst staining assay was performed to detect cell apoptosis. The 144 J/cm2-irradiated group showed a significant increase of PI-positive cells, indicating that LED blue light irradiation had a role in promoting apoptosis of hepatoma cells (Figs. 2C and 2D).
Figure 2: Blue light emitting diode (LED) light inhibited proliferation and promoted apoptosis in hepatoma cells. (A and B) The proliferation of hepatoma cells was determined by 5-ethynyl-2′-deoxyuridine (EdU) staining (Scale bar: 25 μm). (C and D) The apoptosis in hepatoma cells was detected by Hoechst/propidium iodide (PI) staining after blue LED light exposure. Hep3B cells and HepG2 cells with blue LED exposure at 0 and 144 J/cm2, respectively (Scale bar: 25 μm). Data are expressed as the mean ± SEM. *p < 0.05; **p < 0.01; ***p < 0.001.
Blue light emitting diode irradiation inhibited the migration and invasion of hepatoma cells
Subsequently, wound healing and transwell assays were then employed to evaluate whether cell invasion and migration were affected by blue LED irradiation on HCC cells. As shown in Figs. 3A and 3B, the wound healing assay revealed that the size of the wounds in the control group decreased over time compared to the LED blue light irradiated group (144 J/cm2) at 24, 48, and 72 h, and the speed of wound healing could be used to measure cell migration. Compared with the non-irradiated control group, wound closure was significantly inhibited in the irradiated group. The cells were then further evaluated for changes in their ability to migrate by staining as crystal violet is a basic dye that can bind to DNA in the nucleus, thereby staining the nucleus. The cell migration through matrigel-coated membranes was markedly inhibited in blue LED-treated groups compared with control groups (Figs. 3C and 3D).
Figure 3: Blue light emitting diode (LED) light impedes the migration and invasion of hepatoma cells. (A and B) The migration of hepatoma cells was assessed by the wound healing assay (A), (Scale bar: 200 μm). The ratio of wound healing in migrated cells (B). (C and D) The representative images from hepatoma cells stained with crystal violet (C) (Scale bar: 50 μm) and transwell assays showing the invasion ability (D). Data are expressed as the mean ± SEM. *p < 0.05; **p < 0.01; ***p < 0.001.
Blue light emitting diode irradiation inhibited the stem cell potential of hepatocellular carcinoma cells
Essentially, cancer stem cells maintain the vitality of tumor cell populations through self-renewal and unlimited proliferation; Cancer stem cells can be in a dormant state for a long time with a variety of drug resistance approaches and are not sensitive to external physical and chemical factors that kill tumor cells, resulting in tumor recurrence, and migration. The sphere-forming test of tumor cells (sphere-forming size and number) is the gold standard for measuring the stemness of tumor cells. Hence, we next evaluated the effect of LED blue irradiation on the functional properties of cells by studying their ability to form colonies and spheres after they were irradiated with blue light. All HCC cell lines in the 144 J/cm2 LED blue irradiation group had significantly reduced colony-forming and sphere-forming abilities. In contrast, the control group was not affected (Fig. 4).
Figure 4: Blue light emitting diode (LED) light reduces the potential of stem cells in liver cancer. Tumor sphere formation of hepatoma cells after blue LED light irradiation at 0 and 144 J/cm2, respectively (Scale bar: 200 μm).
Blue light emitting diode irradiation exhibited anti-tumor effects on liver cancer by inducing DNA damage
Our study so far fully demonstrated the potential of blue LED irradiation to inhibit hepatoma cells. Next, we sought to clarify the potential regulatory mechanisms of such blue light irradiation-induced cancer cell death. Among the many types of DNA damage, DNA double-strand breaks (DSBs) are considered to be the most serious type of damage because they affect the double helix structure of DNA. Phosphorylating histone H2AX (phosphorylated H2AX histones are called γ-H2AX) accumulates at double-stranded breaks to form foci formed by the accumulation of large amounts of γ-H2AX. The number of γ-H2AX foci in cells can be used to evaluate DNA double-strand breaks, which can then be used to evaluate the mutagenic ability of genotoxic factors. Our immunofluorescence results indicated that γ-H2AX was dramatically increased after fluorescence irradiation both in HepG2 and Hep3B (Figs. 5A and 5B). Consistently, western blotting results also showed that the total γ-H2AX content in HepG2 and Hep3B cells was significantly increased after LED blue light irradiation, which means that blue light irradiation severely damaged the DNA of hepatoma cells (Figs. 5C and 5D).
Figure 5: Blue light emitting diode (LED) exposure increased γ-H2AX expression in liver cancer cells. Hep3B cells and HepG2 cells treated with blue LED irradiation at 0 and 144 J/cm2 respectively. (A and B) Representative images from γ-H2AX immunostained liver cancer cells (Scale bar: 25 μm). (C and D) The protein levels of γ-H2AX after exposure to blue LED light. Data are expressed as the mean ± SEM. *p < 0.05; **p < 0.01; ***p < 0.001.
Sorafenib combined with blue light emitting diode irradiation enhanced the sensitivity of liver cancer cells to sorafenib
Being a multi-target kinase inhibitor, sorafenib can block tumor cell proliferation by inhibiting the activity of Raf-1, B-Raf and other kinases in the Ras/Raf/Mitogen-activated protein kinase/extracellular-signal-regulated kinase (Ras/Raf/MEK/ERK) signaling pathway. According to previous clinical trials, sorafenib is significantly effective in patients with advanced hepatocellular carcinoma. Due to the genetic heterogeneity of hepatocellular carcinoma, a significant proportion of patients are insensitive to sorafenib. Hence, limiting the number of patients who benefit from sorafenib treatment. The CCK-8 assay, EdU staining and colony formation assay were used to analyze the effect of the blue light LED and sorafenib combined treatment on cell proliferation. While CCK8 results showed that cell growth was inhibited in the sorafenib group, cell growth was further lowered in the sorafenib combined with LED group (Fig. 6A). EdU staining and colony formation assay experiments further showed that HepG2 and Hep3B cells in the combined sorafenib and the LED irradiation group had a decreased colony-forming ability compared with the other groups (Figs. 6B and 6E). Given that the proliferation process was inhibited, it indicated that the cells had increased sensitivity to sorafenib.
Figure 6: Combined blue light emitting diode (LED) irradiation treatment and sorafenib enhanced the sensitivity of liver cancer cells to sorafenib. (A) The viability of HepG2 and Hep3B cells and the effect on half maximal inhibitory concentration (IC50) were detected by the Cell Counting Kit-8 (CCK-8) assay. (B and C) 5-ethynyl-2′-deoxyuridine (EdU) staining was used to detect cell proliferation before and after treatment (Scale bar: 25 μm). (D and E) In the presence of blue LED irradiation, the effect of sorafenib was enhanced on colony formation by HepG2 and Hep3B cell lines. Data are expressed as the mean ± SEM. *p < 0.05; **p < 0.01; ***p < 0.001.
In this study, we discovered for the first time that blue LED irradiation documented an anti-tumor effect on liver cancer cells which was strongly supported by the promotion of cell apoptosis and the inhibition of cell proliferation, cell migration, and cell invasion. Additionally, blue LED irradiation could also significantly destroy the stemness characteristic of human liver cancer HepG2 and Hep3B stem-like cells. Furthermore, we identified that DNA damage maker γ-H2AX was also dramatically up-regulated under blue LED treatment and that the combination of blue LED irradiation with sorafenib increased the sensitivity of cells to the drug. This study further demonstrated that the antitumor ability of blue LED irradiation for liver cancer was modulated by DNA damage, which provides a new approach and strategy for the clinical treatment of human liver cancer.
Phototherapy has successfully documented a good therapeutic effect on many diseases, such as spinal cord injury (Hu et al., 2020), acne (Posadzki and Car, 2018), vitiligo (Bae et al., 2017), to name a few. Our previous studies have explored the anti-tumor function of blue LED in human osteosarcoma through the regulation of ROS production and DNA damage (Yan et al., 2018). Additionally, we also confirmed that the combined treatment of blue LED irradiation and arsenic trioxide in human osteosarcoma played coordinated roles, which improved and strengthened the anti-tumor effect of arsenic trioxide (Feng et al., 2019). However, the role of blue LED irradiation in human liver cancer was unclear. Thus, we detected cell viability and apoptosis in liver cancer cell lines HepG2 and Hep3B under blue LED irradiation at 0 and 144 J/cm2, respectively. Results of our analyses indicated that blue LED irradiation exhibited a powerful lethal action on the assayed human liver cancer cells. Cancer metastasis is a multi-step process including cell migration and invasion, which represents the advanced stage of malignancy. Consisting with our results, a recent work also showed that blue LED could inhibit cell migration and invasion of colon cancer cells and fibrosarcoma cells (Oh et al., 2017).
Cancer stem cells are a small part of cells within tumors, which are classified as cancer-initiating cells and have characteristics of self-renewal, differentiation, and tumorigenicity (Clevers, 2011). Liver cancer has been reported to be derived from liver stem cells occurring in adult liver tissue with an origin that can be endogenous or exogenous to the liver. Sphere-formation analysis is a common method used to identify the properties of cancer stem cells in vitro. In the present study, blue LED irradiation strongly targeted the liver cancer cell capacity of generating liver tumor nodules.
DNA damage is a permanent change in DNA nucleotide sequence that occurs during replication and results in changes in genetic characteristics. DNA damage triggers a series of cellular responses and stimulates cell cycle checkpoints (Roos et al., 2016). Cell cycle checkpoints can repair the damage before it interferes with the DNA replication machinery, which will cause cell death if it fails. Core histone protein H2AX (termed γ-H2AX) persistence is a strong molecular marker of DNA damage. Consistently, our immunofluorescence staining results significantly showed that blue LED irradiation increased the expression of γ-H2AX in HepG2 and Hep3B cells, which represents the accumulation of DNA damage.
The multikinase inhibitor sorafenib is currently the only small molecule targeted agent effective against advanced hepatocellular carcinoma, but the phenomenon of resistance is becoming more common, and differences in patient response to sorafenib have aroused increasing concern. Recent studies have shown that glucose metabolism, tumor microenvironment, and epigenetics affect the development of sorafenib action and its drug resistance. Using the CCK8 assay, we found that blue light irradiation further enhanced the inhibitory effect of sorafenib on cell viability and decreased the half maximal inhibitory concentration (IC50) value. The EdU staining assay and the colony formation assay demonstrated that the combination of the two enhanced the sensitivity of cells to sorafenib.
In conclusion, blue LED irradiation leads to the inhibition of cell proliferation, migration, invasion, induction of cell death, and increase of drug sensitivity by inducing DNA damage in liver cancer cells. Therefore, our study will provide a better understanding of blue LED irradiation in liver cancer therapy.
Acknowledgement: Not applicable.
Funding Statement: This work was supported by grants from the National Key Research and Development Program of China (2017YFB0403802), the Outstanding Youth Project of the Natural Science Foundation of Heilongjiang Province (YQ2020H019) and the Huaier Fund in 2023 from Chen Xiao-Ping Foundation for the Development of Science and Technology of Hubei Province.
Author Contributions: The authors confirm their contribution to the paper as follows: T.W., J.H.H., J.J.X., Q.L., J.R.Y., X.T.H., S.Y.G., T.L. and G.X.L. performed research; Y.Y., T.W., J.H.H., analyzed data; Y.Y., L.Q.L., and designed the study and wrote the manuscript. All authors reviewed the results and approved the final version of the manuscript.
Availability of Data and Materials: The datasets used and/or analyzed during the current study are available from the corresponding author upon reasonable request.
Ethics Approval: Not applicable.
Conflicts of Interest: The authors declare that they have no conflicts of interest to report regarding the present study.
References
Bae JM, Jung HM, Hong BY, Lee JH, Choi WJ, Lee JH, Kim GM (2017). Phototherapy for vitiligo: A systematic review and meta-analysis. JAMA Dermatology 153: 666–674. https://doi.org/10.1001/jamadermatol.2017.0002 [Google Scholar] [PubMed] [CrossRef]
Barolet D, Boucher A (2008). LED photoprevention: Reduced MED response following multiple LED exposures. Lasers in Surgery and Medicine 40: 106–112. https://doi.org/10.1002/(ISSN)1096-9101 [Google Scholar] [CrossRef]
Bray F, Ferlay J, Soerjomataram I, Siegel RL, Torre LA, Jemal A (2018). Global cancer statistics 2018: GLOBOCAN estimates of incidence and mortality worldwide for 36 cancers in 185 countries. CA: A Cancer Journal for Clinicians 68: 394–424. https://doi.org/10.3322/caac.21492 [Google Scholar] [PubMed] [CrossRef]
Cenni V, Squarzoni S, Loi M, Mattioli E, Lattanzi G, Capanni C (2020). Emerin phosphorylation during the early phase of the oxidative stress response influences emerin-BAF interaction and BAF nuclear localization. Cells 9: 1415. https://doi.org/10.3390/cells9061415 [Google Scholar] [PubMed] [CrossRef]
Chen W, Sun K, Zheng R, Zeng H, Zhang S, Xia C, Yang Z, Li H, Zou X, He J (2018). Cancer incidence and mortality in China, 2014. Chinese Journal of Cancer Research 30: 1–12. [Google Scholar] [PubMed]
Clevers H (2011). The cancer stem cell: Premises, promises and challenges. Nature Medicine 17: 313–319. https://doi.org/10.1038/nm.2304 [Google Scholar] [PubMed] [CrossRef]
Costa DF, Mendes LP, Torchilin VP (2019). The effect of low- and high-penetration light on localized cancer therapy. Advanced Drug Delivery Reviews 138: 105–116. https://doi.org/10.1016/j.addr.2018.09.004 [Google Scholar] [PubMed] [CrossRef]
Du J, Zhou Y, Li Y, Xia J, Chen Y et al. (2020). Identification of Frataxin as a regulator of ferroptosis. Redox Biology 32: 101483. https://doi.org/10.1016/j.redox.2020.101483 [Google Scholar] [PubMed] [CrossRef]
Erdle BJ, Brouxhon S, Kaplan M, Vanbuskirk J, Pentland AP (2008). Effects of continuous-wave (670-nm) red light on wound healing. Dermatologic Surgery 34: 320–325. [Google Scholar] [PubMed]
Feng C, Gong R, Zheng Q, Yan G, He M et al. (2019). Synergistic anti-tumor effects of arsenic trioxide and blue LED irradiation on human osteosarcoma. International Journal of Biological Sciences 15: 386–394. https://doi.org/10.7150/ijbs.28356 [Google Scholar] [PubMed] [CrossRef]
He M, Yan G, Wang Y, Gong R, Lei H et al. (2021). Blue LED causes autophagic cell death in human osteosarcoma by increasing ROS generation and dephosphorylating EGFR. Journal of Cellular and Molecular Medicine 25: 4962–4973. https://doi.org/10.1111/jcmm.16412 [Google Scholar] [PubMed] [CrossRef]
Hu D, Moalem-Taylor G, Potas JR (2020). Red-light (670 nm) therapy reduces mechanical sensitivity and neuronal cell death, and alters glial responses following spinal cord injury in rats. Journal of Neurotrauma 37: 2244–2260. https://doi.org/10.1089/neu.2020.7066 [Google Scholar] [PubMed] [CrossRef]
Jung YS, Stratton SA, Lee SH, Kim MJ, Jun S et al. (2021). TMEM9-v-ATPase activates Wnt/β-Catenin Signaling via APC lysosomal degradation for liver regeneration and tumorigenesis. Hepatology 73: 776–794. [Google Scholar] [PubMed]
Lu X, Paliogiannis P, Calvisi DF, Chen X (2021). Role of the mTOR pathway in liver cancer: From molecular genetics to targeted therapies. Hepatology 73: 49–61. [Google Scholar] [PubMed]
Migliario M, Sabbatini M, Mortellaro C, Renò F (2018). Near infrared low-level laser therapy and cell proliferation: The emerging role of redox sensitive signal transduction pathways. Journal of Biophotonics 11: e201800025. https://doi.org/10.1002/jbio.201800025 [Google Scholar] [PubMed] [CrossRef]
Oh PS, Jeong HJ (2019). Therapeutic application of light emitting diode: Photo-oncomic approach. Journal of Photochemistry and Photobiology B: Biology 192: 1–7. https://doi.org/10.1016/j.jphotobiol.2019.01.003 [Google Scholar] [PubMed] [CrossRef]
Oh PS, Kim HS, Kim EM, Hwang H, Ryu HH, Lim S, Sohn MH, Jeong HJ (2017). Inhibitory effect of blue light emitting diode on migration and invasion of cancer cells. Journal of Cellular Physiology 232: 3444–3453. https://doi.org/10.1002/jcp.25805 [Google Scholar] [PubMed] [CrossRef]
Ohara M, Kawashima Y, Kitajima S, Mitsuoka C, Watanabe H (2003). Blue light inhibits the growth of skin tumors in the v-Ha-ras transgenic mouse. Cancer Science 94: 205–209. https://doi.org/10.1111/j.1349-7006.2003.tb01420.x [Google Scholar] [PubMed] [CrossRef]
Ohara M, Kawashima Y, Watanabe H, Kitajima S (2002). Effects of blue-light-exposure on growth of extracorporeally circulated leukemic cells in rats with leukemia induced by 1-ethyl-1-nitrosourea. International Journal of Molecular Medicine 10: 407–411. https://doi.org/10.3892/ijmm.10.4.407 [Google Scholar] [CrossRef]
Ohara M, Kobayashi M, Fujiwara H, Kitajima S, Mitsuoka C, Watanabe H (2004). Blue light inhibits melanin synthesis in B16 melanoma 4A5 cells and skin pigmentation induced by ultraviolet B in guinea-pigs. Photodermatology, Photoimmunology and Photomedicine 20: 86–92. https://doi.org/10.1111/j.1600-0781.2004.00077.x [Google Scholar] [PubMed] [CrossRef]
Park JM, Kim YJ, Park S, Park M, Farrand L et al. (2020). A novel HSP90 inhibitor targeting the C-terminal domain attenuates trastuzumab resistance in HER2-positive breast cancer. Molecular Cancer 19: 161. https://doi.org/10.1186/s12943-020-01283-6 [Google Scholar] [PubMed] [CrossRef]
Petrick JL, Florio AA, Znaor A, Ruggieri D, Laversanne M, Alvarez CS, Ferlay J, Valery PC, Bray F, McGlynn KA (2020). International trends in hepatocellular carcinoma incidence, 1978–2012. International Journal of Cancer 147: 317–330. https://doi.org/10.1002/ijc.32723 [Google Scholar] [PubMed] [CrossRef]
Posadzki P, Car J (2018). Light therapies for acne. JAMA Dermatology 154: 597–598. https://doi.org/10.1001/jamadermatol.2018.0110 [Google Scholar] [PubMed] [CrossRef]
Ringelhan M, Pfister D, O’Connor T, Pikarsky E, Heikenwalder M (2018). The immunology of hepatocellular carcinoma. Nature Immunology 19: 222–232. https://doi.org/10.1038/s41590-018-0044-z [Google Scholar] [PubMed] [CrossRef]
Roos WP, Thomas AD, Kaina B (2016). DNA damage and the balance between survival and death in cancer biology. Nature Reviews Cancer 16: 20–33. https://doi.org/10.1038/nrc.2015.2 [Google Scholar] [PubMed] [CrossRef]
Satriano L, Lewinska M, Rodrigues PM, Banales JM, Andersen JB (2019). Metabolic rearrangements in primary liver cancers: Cause and consequences. Nature Reviews Gastroenterology & Hepatology 16: 748–766. https://doi.org/10.1038/s41575-019-0217-8 [Google Scholar] [PubMed] [CrossRef]
Siqueira VPC, Evangelista MIS, Dos Santos A, Marcos RL, Ligeiro-de-Oliveira AP, Pavani C, Damazo AS, Lino-Dos-Santos-Franco A (2017). Light-emitting diode treatment ameliorates allergic lung inflammation in experimental model of asthma induced by ovalbumin. Journal of Biophotonics 10: 1683–1693. https://doi.org/10.1002/jbio.201600247 [Google Scholar] [PubMed] [CrossRef]
Yan G, Zhang L, Feng C, Gong R, Idiiatullina E et al. (2018). Blue light emitting diodes irradiation causes cell death in colorectal cancer by inducing ROS production and DNA damage. The International Journal of Biochemistry & Cell Biology 103: 81–88. https://doi.org/10.1016/j.biocel.2018.08.006 [Google Scholar] [PubMed] [CrossRef]
Yoshida A, Yoshino F, Makita T, Maehata Y, Higashi K, Miyamoto C, Wada-Takahashi S, Takahashi SS, Takahashi O, Lee MCI (2013). Reactive oxygen species production in mitochondria of human gingival fibroblast induced by blue light irradiation. Journal of Photochemistry and Photobiology B: Biology 129: 1–5. https://doi.org/10.1016/j.jphotobiol.2013.09.003 [Google Scholar] [PubMed] [CrossRef]
Yu LM, Zhang WH, Han XX, Li YY, Lu Y et al. (2019). Hypoxia-induced ROS contribute to myoblast pyroptosis during obstructive sleep apnea via the NF-κB/HIF-1α signaling pathway. Oxidative Medicine and Cellular Longevity 2019: 4596368. https://doi.org/10.1155/2019/4596368 [Google Scholar] [PubMed] [CrossRef]
Yuan Y, Yan G, He M, Lei H, Li L et al. (2021). ALKBH5 suppresses tumor progression via an m6A-dependent epigenetic silencing of pre-miR-181b-1/YAP signaling axis in osteosarcoma. Cell Death & Disease 12: 60. https://doi.org/10.1038/s41419-020-03315-x [Google Scholar] [PubMed] [CrossRef]
Zhao W, Hu JX, Hao RM, Zhang Q, Guo JQ et al. (2018). Induction of microRNA‐let‐7a inhibits lung adenocarcinoma cell growth by regulating cyclin D1. Oncology Reports 40: 1843–1854. https://doi.org/10.3892/or.2018.6593 [Google Scholar] [PubMed] [CrossRef]
Cite This Article
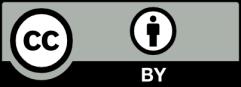