Open Access
ARTICLE
Modeling Date Palm Trunk Fibers (DPTF) Packed Bed Adsorption Performances for Cadmium Removal from Aqueous Wastewater
1
Mechanical Engineering Department, Faculty of Engineering Technology, Al-Balqa Applied University, Amman, 11134, Jordan
2
Chemical Engineering Department, Faculty of Engineering Technology, Al-Balqa Applied University, Amman, 11134, Jordan
* Corresponding Authors: Ahmad S. Awad. Emails: ;
Fluid Dynamics & Materials Processing 2023, 19(6), 1535-1549. https://doi.org/10.32604/fdmp.2023.024300
Received 25 May 2022; Accepted 09 August 2022; Issue published 30 January 2023
Abstract
In this study, the potential of a low-cost bio-adsorbent, taken directly from Date Palm Trunk Fibers (DPTF) agricultural wastes, for cadmium ions removal from wastewaters is examined. The performances of this adsorbent are evaluated by building breakthrough curves at different bed heights and flow rates while keeping other parameters, such as the initial feed concentration, pH, and particle size, constant. The results indicate that the maximum cadmium adsorption capacity of DTPF can be obtained from the Thomas model as 51.5 mg/g with the most extended mass transfer zone of 83 min at the lowest flow rate at 5 ml/min. The saturation concentrations (NO) and the rate constant (kab) obtained from the BDST (bed depth service time) model are 7022.16 mg/l and 0.0536 l/mg.min, respectively. Using the Yon-Nelsen Model, it is found that operating at a lower flow rate leads to a larger value of the elapsed needed time to reach a 50% breakthrough. The Wolborska model indicates that the bed capacity increases with decreasing the flow rate, and the adsorbent can achieve a greater external mass transfer kinetic coefficient (2.271/min) at a higher flow rate.Graphical Abstract
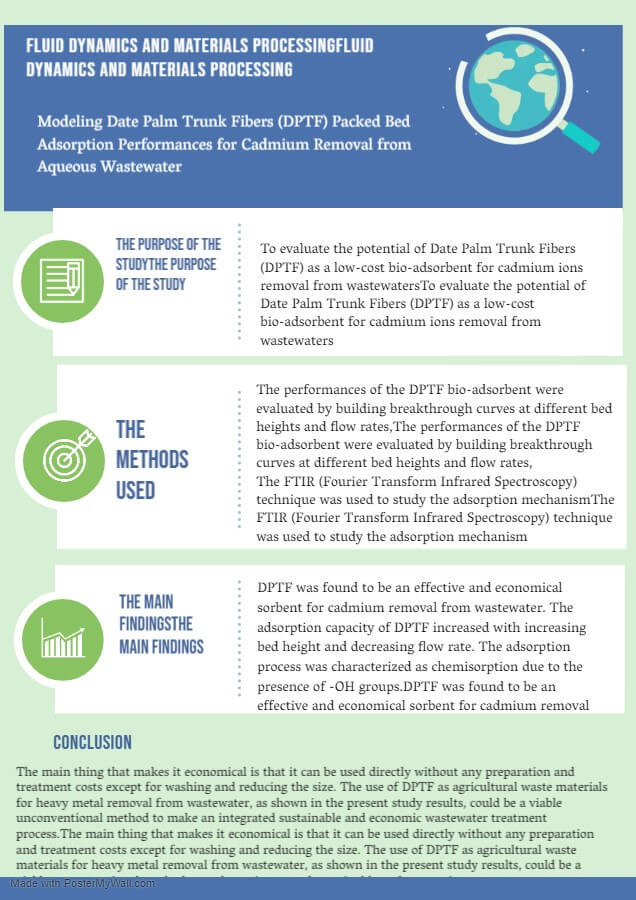
Keywords
A vast amount of date palm biomass wastes are collected yearly without proper usage; biomass is organic matter that can be attained from living organisms like plants, trees, algae, organic waste, etc. Annually, about 20 kg of organic waste is generated from only one date palm tree [1,2]. Often, date palm wastes are burned or buried in landfills; thus, this causes pollution issues. Its large quantities and natural availability make it attractive and competitive for different thermal and physical/chemical technologies for good utilization of date palm biomass in wastewater treatment, such used date palm fibers as promising adsorbents for unwanted materials removal [3].
As the world’s population increases, our clean water sources have decreased. This deficiency has moved our focus toward pollution reduction and control. In Jordan, which is classified as a semi-arid to arid country and considered one of the world’s poorest water resources, disposal of wastes and wastewater without treatment in lands and water bodies is no longer possible [4].
Wastewater contains toxic pollutants like heavy metals usually coming from industrial processes such as mining, petrochemical manufacturers, oil and gas refineries, dyes and pharmaceuticals [5], other sources from natural deposits, and discharges of domestic and agricultural wastewater [6,7]. Although the presence of heavy metals in traces concentrations (ppb range to less than 10 ppm) can cause severe damage to human health, agriculture, and the ecosystem [8,9]. Unlike organic pollutants, they do not degrade biologically because of complex physicochemical properties. Thus, they can be collected in living things when taken up and stored inside the body, causing diseases [9]. Heavy metals like barium, cadmium, chromium, lead, mercury, and silver can be found in water reservoirs [8]. However, water wasted with these hazardous substances should be treated separately before discharging to the larger water bodies [10].
Cadmium Pollution from industrial wastewater and potable water is considered a severe problem and one of the most current environmental issues [11]. Many plants and water life animals can absorb cadmium. It is widely known that cadmium is non-biologically degraded, so its absorption by the human body will lead to long-term accumulation [12]. Cadmium aggregation in body organisms could cause harmful, risky diseases like kidney tissue damage due to the deposition of necrotic protein [13,14]. Moreover, it has been reported to have severe adverse effects on liver tissues.
Furthermore, much literature investigated cadmium accumulation’s adverse effects on lung tissues and the related respiratory problems, as well as its effect on immunity suppression [15]. Consequently, developing processes for removing cadmium from industrial wastewaters and drinking water becomes essential [16]. According to FAO/WHO permitted pollution levels, the limit of cadmium should be less than 0.005 mg/l.
There are several processes used for wastewater polluted with heavy metal ions like chemical precipitation techniques [17,18], membrane processes [19,20], electro dialysis [21], ion exchange process [22], flotation [23], extraction [24], and adsorption [25,26]. Adsorption is considered a meaningful and good alternative from an economic view due to its simplicity, availability, and low cost [27,28]. Many adsorbents can be used in adsorption methods, including plant leaves, roots, fruit shells, agricultural wastes, etc. [29]. It is apparent that many researchers investigated the possibility of applying low–cost adsorbents derived from natural resources and agricultural wastes for various metals ions and found its effectiveness and economic feasibility [30–33]. Agricultural wastes like Date palm trunk fibers (DPTFs) are composed of organic components such as pectins, cellulose, terpenes, and lignins. These compounds contain –COOH and –OH functional groups and have a high metal ions adsorption capacity utilizing ion exchange or chelation [34,35].
Al Shayeb et al. [36] considered the date palm a bio-monitoring of lead, zinc, copper, nickel, chromium, and lithium. Results showed that Pb was effectively attached to the surface of leaflets. Chromium and lithium. Results showed that Pb was effectively attached to the surface of leaflets. Also, Cr (II) was strongly bonded with the leaflet’s surface. All other metals understudies showed different bonded with different ratios. Alfarooq et al. [37] used date palm fiber as a precursor to synthesize a low-cost powder-activated carbon (nPAC) to remove aluminum (Al3+) from solutions by carbonization and activation of KOH. The authors found the optimum synthesis condition for both time and temperature at 1.09 h and 650oC. At the same time, the removal capacity was up to 99.5% and 9.958 mg.g−1. Ahmad et al. [38] investigated the removal of PO43− ions from an aqueous solution using date palm fibers. Results showed that the optimum conditions for maximum removal (capacity of 4.35 mg P/g) were at pH 6.8, with the adsorbent dosage of 6 g/L at a constant temperature of 18°C ± 02. Furthermore, the aqueous solution reached equilibrium within 120 min of exposure time.
Al-Shawabkeh et al. [39] studied the removal of cadmium using a date palm fiber with a fixed-bed column, and the authors investigated the influence of particle size; results showed that higher adsorption capacity (20.9 mg/g) was encountered for smaller size date palm fibers. However, a lack of studies used (DPTFs) as a continuous fixed bed columns application under different conditions. In this study (DPTFs) were demonstrated as a potential low-cost adsorbent for the separation of chromium [Cd(VI)] from industrial effluent wastewater in a continuous fixed-bed column. The effect of particle size was focused.
The Date Palm Trunk Fibers (DPTF) were collected from local farms located in the Al-Agwaar region in the Jordan Valley area of the Balqa governorate (44° 43.574′ N; 122° 41.9052′ W). Fig. 1 shows a sample of the raw fibers.
Figure 1: A sample of the collected raw Date Palm Trunk Fibers (DPTF)
The available raw fibers were primarily subjected to intensive cleaning by washing them several times using tap water to get rid of all the solid impurities attached to the fibers, such as fine grains of dust, sand, and any foreign things stuck between their fibers. After the cleaning water became clear and free of impurities, a final cleaning process was carried out three times using distilled water to ensure that all impurities were removed and that the fibers were clean and could be used for experiments. Afterward, the DPTF was set aside in an electrical furnace for 15 h at 95°C to produce clean, dry fibers. The DPTF were milled using a multi-purpose grinder. A sieving process was then performed for three hours to classify the sizes and choose the appropriate size to prepare the fixed bed adsorbent. After obtaining the appropriate size, the adsorbent is kept at 105°C to keep it dry and away from moisture. The samples are then cooled and stored in closed glass containers to be ready for preparing the fixed bed adsorbent. The artificial wastewater contaminated with cadmium ions was prepared by dissolving cadmium nitrate in deionized water. A standard solution (Concentration = 1.0 mg/l) was prepared by adding 2.74 g of a high purity grade (p. a.) Cd (NO3)2.4H2O to one liter of deionized water. The required concentration of cadmium ions solution was achieved by diluting the standard solution with deionized water.
2.2 Fixed Bed Adsorption Experiments
Fixed bed column experiments were held using the apparatus shown in Fig. 2. The apparatus was designed to measure the breakthrough curves during the continuous adsorption of cadmium ions from an aqueous solution using the DPTF adsorbent. The setup has a 20 L well stirred and closed feed tank to store the prepared artificial wastewater contaminated with cadmium ions at a specific concentration for the intended experiment. The column was made from glass material with a 1.1 cm internal diameter and a length of 50 cm (1 cm2 cross-sectional area). Fiberglass wool layers were fixed at the top and bottom of the packed bed adsorbent to keep constant bed height, support the bed, and protect it from escaping the flowing solution. This support was retained using a fixed stainless steel sieve during the whole experiment.
Figure 2: The experimental setup was used to obtain measurements of the breakthrough curves for the fixed bed cadmium adsorption from an aqueous solution on a DPT
A peristaltic pump (Heidolph, Germany) was used to force the liquid to flow from the feed tank to the top of the column. The flow rate was kept constant during all experiments using the installed valve and the flow meter. The DPTF adsorbent was packed inside the column until the anticipated bed height. The column effluent was collected in a graduated beaker, and samples were taken at steady time intervals to be analyzed for concentration measurements. The residual concentration of cadmium ions in the aqueous solution from the effluents was measured using atomic absorption spectroscopy.
2.3 Fixed-Bed Column Adsorption Studies
The separation efficiency of cadmium ions from contaminated queues solutions using a fixed-bed adsorption column was investigated by measuring breakthrough curves under different operating conditions. The variation of the effluent concentration with time, the time to reach the breakthrough, and the time to reach bed exhaustion are significant parameters used in the design and operation of the adsorption columns. Continuous fixed-bed column runs were conducted to determine the operating parameters’ influence on the adsorption process of cadmium ions onto the DPTF adsorbent. Several breakthrough curves were measured under controlled experimental conditions. A systematic experimental investigation was performed by keeping the feed concentration and the particle size constant in all experiments and varying the feed flow rate at fixed bed height. Another series of experiments were conducted at different bead heights and fixed flow rates. The experimental conditions are summarized in Table 1.
3 Analyses and Theoretical Modeling of Packed Bed Column Data
This research studied the practical applicability of DPTF as an adsorbent in a fixed bed column operating with continuous flow conditions to remove cadmium from aqueous solutions. The performance of the fixed bed adsorption process is determined by analyzing experimentally measured breakthrough curves at different operating conditions for a given inlet concentration (C0) of the adsorbate. The breakthrough curve represents the variation of the effluent concentration (Ct) from the fixed bed column or the normalized concentration (Ct/C0) with time (t) or effluent volume (Veff). The shape of the breakthrough curve, the time to reach the breakthrough, and the time to reach bed exhaustion are significant characteristics used to determine the operation, dynamic behavior, separation efficiency, and design of the fixed bed adsorption column.
In the fixed bed adsorption process, the concentration of adsorbate in the fluid and solid phases varies with time and with the bed’s location. Initially, the mass transfer mainly occurs near the inlet of the bed, wherever fluid contacts fresh adsorbent. If the adsorbent contains no adsorbate initially, the concentration in the fluid decreases exponentially with the position in the bed to zero before the end of the bed is reached. The section of the bed where adsorbate is essentially adsorbed or the area where the maximum decrease in concentration occurs is recognized as the zone of mass transfer (MTZ).
The breakthrough points are usually recognized as the points where the ratio of the effluent concentration to the inlet concentration (Ct/C0) lies between 0.01 at the breakthrough time (tb) and 0.99 at the exhaustion time (te). The difference between tb and te is the mass transfer zone (MTZ) according to the relation:
3.1 Modeling of Fixed-Bed Column Studies
Various mathematical models and theories have been investigated to predict the breakthrough curves, estimate the design parameters of adsorption columns, and evaluate the dynamic behavior and column performances. Among these models used in this study: are the Thomas model, Adams-Bohart model, Yoon-Nelson model, and Wolborska model.
Thomas model is one of the most commonly applied models used in predicting the breakthrough curves and the dynamic behavior and the performance of the adsorption-backed bed column. It is based on the hypothesis that no axial dispersion with a plug flow pattern in the fixed bed adsorbent. Also, this model assumes that the rate driving force in the adsorption process is governed by second-order reversible reaction kinetics and Langmuir kinetics. It is often used to determine the adsorption capacity of the adsorbent. The linearized form of the Thomas equation is given by the relation [40]:
where (C0) is the influent concentration of adsorbate, i.e., pollutant (mg/l), (Ct) is the effluent concentration of the pollutant (mg/l), F is the volumetric influent flow rate (L/min), KTh is Thomas rate parameter (L/min.mg), t is the time (min), Qe is the maximum (equilibrium) adsorption capacity (mg/g) of the adsorbent. M is the mass of adsorbent in the fixed bed column (g). Hence, the kinetic coefficient KTh and q can be estimated from the slope and intercept of the straight-line plotting of
The Adams-Bohart model is routinely used to describe fixed-bed column breakthrough behavior at its initial stages [42]. The simplified equation of the Adams-Bohart model linearized form is as follows [43,44]:
where N0 is the bed capacity (mg/l), υ is the linear velocity (cm/min), Kab is Adams-Bohart model rate constant for the (l/mg.min), Z is the bed height of column (cm), t is the breakthrough time, is the breakthrough concentration. Applying Bohart–Adams method requires no less than several individual column tests that need to be performed to obtain the necessary experimental data, which is time-wasting. However, an approach has been developed by Hutchins [45], which involves fewer fixed column runs to obtain the required data. This method, known as the bed depth service time (BDST) model, where the Adams-Bohart equation is rewritten with the following linear equation [45,46]:
where
where tb, the breakthrough time is when the normalized concentration (Ct/C0) reaches the value of 0.01. The BDST model was established by assuming that the adsorption rate is governed by the surface reaction between the adsorbate and the residual capacity of the adsorbent.
The Yoon-Nelson model depends on the principle that the possibility of adsorbate adsorption and breakthrough of adsorbate on the adsorbent is proportionate to the rate of decrees in the adsorption probability for every adsorbate species [47]. The linearized form of the Yoon-Nelson model is [48]:
where KYN and τ, the rate parameter (L/min) and the elapsed time to reach a 50% breakthrough (min), the value of KYN is influenced by the column operating conditions such as the influent concentration of pollutants, height of the bed and rate of flow. The values of KYN and τ can be evaluated from the intercepts and slopes of the straight-line Plotting of
The model developed by Wolborska is based on the general equations of mass transfer describing diffusion mechanisms in the low concentration range breakthrough curves. The linearized form of this model is expressed by the relation [49]:
Results and Discussion Where βa is the external mass transfer coefficient (1/min). N0 is the adsorption capacity of the bed per unit volume (mg/L). The values of the parameters of the Wolborska model can be determined from the Plot of
4 Characterization of DPTF Adsorbent
The FTIR spectra of DPTF before and after Cd (VI) adsorption are shown in Fig. 3. Comparing the two spectra shows differences in the absorbance peak’s appearance. The infrared spectrum of pristine DPTF showed a broad peak at 3380.08 cm–1 related to the O–H stretching vibrations of hemicelluloses, cellulose, lignin, and pectin components [50], after the biosorption of Cd (VI). The asymmetrical stretching vibration was significantly deformed at 3332.55 cm–1, suggesting chemical interactions occurred between the hydroxyl groups and the metal ions on the surface of the bio–sorbent [51]. Furthermore, the peak at 1744 cm–1 shifted to 1640 cm–1 can be attributed to the OH involved in Cd (VI) [52]. Date palm fiber before and after adsorption showed peaks at (1593.65 cm−1) and (1596.91), which could be referred to as the stretching vibrations of the presence of the (N–H) amide group; the sharp, more intense peak appeared for date palm fiber after adsorption at 1036.93 cm–1 could be due to stretching vibration of C–OH of alcohols and carboxylic acids [53]. Mild shifting was observed on the C–O band (1103.07 to 1010.63 cm–1) [54]. A minor shifting of wavelengths is absorbed can be noted, suggesting the adsorption of Cd (VI) on the DPTF surface.
Figure 3: FTIR spectra of DPF before and after adsorption
In the first stage of removal investigations in the continuous flow fixed-bed column with DPTF adsorbent, the flow rate varied from 5 to 15 ml/min, whereas the inlet Concentration of cadmium ions in the feed water was kept constant at 115 mg/l and the mass of the adsorbent was 11 g corresponds to 10 cm bed height. The plots of experimental measurements of breakthrough curves as normalized cadmium concentration (Ct/C0) against time (t) at different flow rates are depicted in Fig. 4. Fig. 4 shows a typical S-shaped form at different flow rates. This is substantial evidence that resistance to bulk diffusion mass transfer and the bonding of molecules into the pore surface play a vital role during the adsorption process of cadmium onto DPTF. The shown variation of Ct/C0 with time in Fig. 4 results from the downwards movement of the interface layer between the mass transfer zone (MTZ) and the saturated zone. This causes decay in the MTZ and growth in the saturation zone with time, thus a continuous increase in the (C/C0) value. The measured breakthrough data were fitted to the Thomas model using linear regression analysis to determine the Thomas rate constant (KTh) and maximum sorption capacity (Qe) at the different feed flow rates. Table 2 shows that increasing the flow rate leads to a decrease in the maximum (equilibrium) adsorption capacity (Qe) and an increase in the values of the Thomas rate constant (KTh). The values of R2 of the kinetic model alternated from 0.9371 to 0.9598, demonstrating good linearity and that the experimental data can be described by this model to a high level of accuracy, with relatively high (R2) values.
Figure 4: Experimental and predicted (Thomas model) breakthrough curves at different flow rates for cadmium adsorption onto DPTF
As depicted in Fig. 4, at the fewest flow rate (5 ml/min), comparatively increased uptake performance was noticed for cadmium ions sorption to DPTF. This is a consequence of the strong adsorption driving force between the cadmium ions on the DPTF and the cadmium ion in the solution; thus, better column performance was observed. Generally sharper breakthrough curves (i.e., shorter mass transfer zone) were measured at elevated rates of flow. The breakpoint time as well as total maximum (equilibrium) adsorption capacity (mg/g) of the adsorbent (see Table 2) also reduced with the rising flow rate. This attitude can be interpreted by the evidence that cadmium adsorption is influenced by the inadequate residence time of the cadmium ions in the column. This inadequate time reduces the bonding potential of the cadmium ions onto DPTF. While shorter MTZ was detected at the higher flow rate, the adsorption capacity and cadmium ions uptake were higher at lower flow rates and thus better column performance. The results presented in Table 2 show that a maximum cadmium adsorption capacity on DTPF was obtained as 51.5 mg/g (favorable) with the longest mass transfer zone of 83 min (unfavorable) at the lowest flow rate at 5 ml/min.
Fig. 5 displays the measured breakthrough curves at different bed heights under the same operating conditions. It can be observed that a reduction in the slope of the breakthrough curve as the bed height is enlarged from 5 to 15 cm. This indicates a rapid mass transfer zone.
Figure 5: Breakthrough curves at different adsorbent bed heights for cadmium adsorption
In this study, the bed depth service time (BDST) approach will be used to estimate the parameters N0 and kab from the slope and intercept of the linear Plot of tb vs. Z using the experimentally measured breakthrough curves at different bed heights shown in Fig. 5.
Fig. 6 shows the BDST plot using the experimental data of cadmium ions adsorption onto DPTF at different bed heights (Fig. 5) for a volumetric flow rate of 10 ml/min (linear velocity = 10.53 cm/min). The linear relationship obtained from the BDST plot for an initial concentration of 115 mg/l is expressed in Eq. (9):
Figure 6: Bed depth service time plot (Eq. (4)) for the adsorption of cadmium ions onto DPTF
The saturation concentrations (N0) and the rate constant (kab) obtained from BDST is 7022.16 mg/l and 0.0536 l/mg. min, respectively. DTPF has the advantage of a high value of saturation concentration, allowing more solutions to be processed, resulting in a reduced adsorbent exhaustion rate. The linear correlation coefficient is R2 = 0.9619, indicating a high degree of linearity and thus an excellent fitting of the experimental data to the BDST model. The estimated BDST model parameters can be useful to scale up to the industrial process and at other different flow rates and concentrations without extra experimental efforts.
4.3 Analysis Using the Yoon-Nelson Model
The values of KYN and τ are evaluated from the intercepts and slopes of the straight-line Plot of
Figure 7: Measured and predicted from the Yoon and Nelson models breakthrough curves for the sorption of Cd(II) ions onto DPTF as a function of flow rate
4.4 Application of Wolborska Model
The measured breakthrough curves were analyzed applying the Wolborska model (Eq. (8)) to improve the understanding of the role of column operating flow rate on the mass transfer mechanism. This model depicts the diffusion mechanism in adsorption systems of low concentration generated from the mass transfer equation. Fig. 8 shows the linear fitting of the breakthrough data concerning Eq. (8), and the estimated Wolborska model parameters are listed in Table 3.
Figure 8: The linear fitting of the breakthrough data according to the Wolborska model (Eq. (8))
Regarding the parameters of the Wolborska model given in Table 2, the values of the bed capacity increase with decreasing the flow rate. However, it is noteworthy that the external mass transfer coefficient (βa) changes considerably by changing the flow rate. The adsorbent has a more significant external mass transfer kinetic coefficient (2.271/min) at a higher flow rate. This result designates an improved external mass transfer rate at a higher flow rate which does not agree with the measured improved performance and high capacity of the fixed bed at a low flow rate, as can be predicted from the breakthrough data. This can be attributed to the fact that the internal mass transfer process mainly influences the mass transfer; the binding to the adsorbent inside the pores is affected by the flow rate and contact time. Predictably, a low flow rate provides more contact time, thus a slight resistance for cadmium ions to be adsorbed to the active sites within the pores of DPTF. This indicates that pores diffusion and binding to the active sites is the controlling mechanism in the adsorption process of cadmium ions onto the DPTF.
Date Palm Trunk Fibers (DPTF) which are low-cost, renewable agricultural waste, environmentally friendly, and available in large amounts, were found to be an effective sorbent for the removal of cadmium from wastewater. The FTIR studies showed that the adsorption of cadmium ions on date palm fibers could be characterized as a chemisorption process due to the presence of –OH groups. An increase in bed height resulted in improved sorption performance. This study showed that variation in bed heights of DPTF bio-adsorbent expands the bed performance at both breakpoint and exhaustion point. The BDS model parameters that can be used in the design of industrial adsorption columns were estimated. The Thomas model was successfully used to fit the column data at different flow rates, and the constants were evaluated. The sorption capacity exhibited by DPTF was found to be high (51.5 mg.g−1 at a low flow rate) compared to other bio-sorbents. The main thing that makes it economical is that it can be used directly without any preparation and treatment costs except for washing and reducing the size. The use of DPTF as agricultural waste materials for heavy metal removal from wastewater, as shown in the present study results, could be a viable unconventional method to make an integrated sustainable and economic wastewater treatment process.
Funding Statement: The authors received no specific funding for this study.
Conflicts of Interest: The authors declare that they have no conflicts of interest to report regarding the present study.
References
1. Faiad, A., Alsmari, M., Ahmed, M. M., Bouazizi, M. L., Alzahrani, B. et al. (2022). Date palm tree waste recycling: Treatment and processing for potential engineering applications. Sustainability, 14(3), 1134. DOI 10.3390/su14031134. [Google Scholar] [CrossRef]
2. Martis, R., Al-Othman, A., Tawalbeh, M., Alkasrawi, M. (2020). Energy and economic analysis of date palm biomass feedstock for biofuel production in UAE: Pyrolysis, gasification and fermentation. Energies, 13(22), 5877. DOI 10.3390/en13225877. [Google Scholar] [CrossRef]
3. Ahmad, T., Danish, M., Rafatullah, M., Ghazali, A., Sulaiman, O. et al. (2012). The use of date palm as a potential adsorbent for wastewater treatment: A review. Environmental Science and Pollution Research, 19(5), 1464–1484. DOI 10.1007/s11356-011-0709-8. [Google Scholar] [CrossRef]
4. Saidan, M., Al-Addous, M., Al-Weshah, R., Ibrahim, O., Alkasrawi, M. et al. (2020). Wastewater reclamation in major Jordanian industries: A viable component of a circular economy. Water, 12(5), 1276. DOI 10.3390/W12051276. [Google Scholar] [CrossRef]
5. Khulbe, K., Matsuura, T. (2018). Removal of heavy metals and pollutants by membrane adsorption techniques. Applied Water Science, 8(1), 1–30. DOI 10.1007/s13201-018-0661-6. [Google Scholar] [CrossRef]
6. Fergusson, J. E. (1990). The heavy elements: Chemistry, environmental impact and health effects, pp. 85–547. Oxford, New York: Pergamon Press. [Google Scholar]
7. Kabata-Pendia, A. (2001). Trace elements in soils and plants. 3rd edition. FL: CRC, Boca Raton. [Google Scholar]
8. Sincero, A., Sincero, G. (2002). Physical-chemical treatment of water and wastewater. USA: CRC Press. [Google Scholar]
9. Choudhury, T., Pathan, K., Amin, M., Ali, M., Quraishi, S. et al. (2012). Adsorption of Cr (III) from aqueous solution by groundnut shell. Journal of Environmental Science and Water Resources, 1(6), 144–150. [Google Scholar]
10. Gupta, V. K., Gupta, M., Sharma, S. (2001). Process development for the removal of lead and chromium from aqueous solutions using red mud--An aluminum industry waste. Water Research, 35(5), 1125–1134. DOI 10.1016/s0043-1354(00)00389-4. [Google Scholar] [CrossRef]
11. Mishra, P., Patel, R. (2009). Removal of lead and zinc ions from water by low cost adsorbents. Journal of Hazardous Materials, 168(1), 319–325. DOI 10.1016/j.jhazmat.2009.02.026. [Google Scholar] [CrossRef]
12. Dixit, S., Singh, D. (2014). Role of free living, immobilized and non-viable biomass of nostoc muscorum in removal of heavy metals: An impact of physiological state of biosorbent. Cellular and Molecular Biology, 60(5), 110–118. [Google Scholar]
13. Gunay, A., Arslankaya, E., Tosun, I. (2007). Lead removal from aqueous solution by natural and pretreated clinoptilolite: Adsorption equilibrium and kinetics. Journal of Hazardous Materials, 146(1–2), 362–371. DOI 10.1016/j.jhazmat.2006.12.034. [Google Scholar] [CrossRef]
14. Altaher, H., Alghamdi, A., Omar, W. (2015). Innovative biosorbent for the removal of cadmium ions from wastewater. Environmental Engineering and Management Journal, 14(4), 793–800. DOI 10.30638/eemj.2015.088. [Google Scholar] [CrossRef]
15. Sarkar, A., Ravindran, G., Krishnamurthy, V. (2013). A brief review on the effect of cadmium toxicity: From cellular to organ level. International Journal of Bio-Technology and Research, 3(1), 17–36. [Google Scholar]
16. Lakherwal, D. (2014). Adsorption of heavy metals: A review. International Journal of Environmental Research and Development, 4(1), 41–48. [Google Scholar]
17. Abdullah, A., Naushada, Mu., Tansir, A., Mohammad, A., Ahmed, A. et al. (2020). Removal of highly toxic Cd(II) metal ions from aqueous medium using magnetic nanocomposite: Adsorption kinetics, isotherm and thermodynamics. Desalination and Water Treatment, 181, 355–361. DOI 10.5004/dwt.2020.25108. [Google Scholar] [CrossRef]
18. Aziz, H., Adlan, M., Ariffin, S. (2008). Heavy metals (Cd, Pb, Zn, Ni, Cu and Cr (III)) removal from water in Malaysia: Post treatment by high quality limestone. Bioresource Technology, 99(6), 1578–1583. DOI 10.1016/j.biortech.2007.04.007. [Google Scholar] [CrossRef]
19. Saffaj, N., Loukil, H., Younssi, S., Albizane, A., Bouhria, M. et al. (2004). Filtration of solution containing heavy metals and dyes by means of ultrafiltration membranes deposited on support made of morrocan clay. Desalination, 168, 301–306. DOI 10.1016/j.desal.2004.07.013. [Google Scholar] [CrossRef]
20. Trivunac, K., Stevanovic, S. (2006). Removal of heavy metal ions from water by complexation-assisted ultrafiltration. Chemosphere, 64(3), 486–491. DOI 10.1016/j.chemosphere.2005.11.073. [Google Scholar] [CrossRef]
21. Jakobsen, M., Rasmussen, J., Nielsen, S., Ottosen, L. (2004). Electrodialytic removal of cadmium from wastewater sludge. Journal of Hazardous Materials, 106(2–3), 127–132. DOI 10.1016/j.jhazmat.2003.10.005. [Google Scholar] [CrossRef]
22. Fang, X., Jia, L., Chai, Y., Chen, X. (2016). Study on the treatment of wastewater containing Cu (II) by D851 ion exchange resin. Desalination and Water Treatment, 57(8), 3597–3605. DOI 10.1080/19443994.2014.986528. [Google Scholar] [CrossRef]
23. Zak, S. (2012). Treatment of the processing wastewaters containing heavy metals with the method based on flotation. Ecological Chemistry and Engineering’s, 19(3), 433–438. DOI 10.2478/v10216-011-0033-8. [Google Scholar] [CrossRef]
24. Berrama, T., Benaouag, N., Kaouah, F., Bendjama, Z. (2013). Application of full factorial design to study the simultaneous removal of copper and zinc from aqueous solution by liquid–liquid extraction. Desalination and Water Treatment, 51(10–12), 2135–2145. DOI 10.1080/19443994.2013.734678. [Google Scholar] [CrossRef]
25. Sha, L., Xueyi, G., Ningchuan, F., Qinghua, T. (2009). Adsorption of Cu2+ and Cd2+ from aqueous solution by mercator–acetic acid modified orange peel. Colloids and Surfaces B: Biointerfaces, 73(1), 10–14. DOI 10.1016/j.colsurfb.2009.04.021. [Google Scholar] [CrossRef]
26. Nirmala, N., Shriniti, V., Aasresha, K., Arun, J., Gopinath, K. et al. (2022). Removal of toxic metals from wastewater environment by graphene-based composites: A review on isotherm and kinetic models, recent trends, challenges and future directions. Science of the Total Environment, 20(840), 156–164. DOI 10.1016/j.scitotenv.2022.156564. [Google Scholar] [CrossRef]
27. Kannapan, P., Gopinath, D. -V., Dhakshinamoorthy, G., Prakash, A., Adithya, J. et al. (2021). Environmental applications of carbonbased materials: A review. In: Environmental chemistry letters, vol. 19, no. 1, pp. 557–582. Springer Science and Business Media Deutschland GmbH. Springer Nature Switzerland AG. DOI 10.1007/s10311-020-01084-9. [Google Scholar] [CrossRef]
28. Sadanand, P., Namgyu, S., Misook, K. (2022). Synergistic sorption performance of karaya gum crosslink poly(acrylamide-co-acrylonitrile) @ metal nanoparticle for organic pollutants. International Journal of Biological Macromolecules, 15(210), 300–314. DOI 10.1016/j.ijbiomac.2022.05.019. [Google Scholar] [CrossRef]
29. Pandey, S., Do, J., Kim, J., Kang, M. (2020). Fast and highly efficient catalytic degradation of dyes using κ-carrageenan stabilized silver nanoparticles nanocatalyst. Carbohydrate Polymers, 230. DOI 10.1016/j.carbpol.2019.115597. [Google Scholar] [CrossRef]
30. Cazetta, A., Vargas, A., Nogami, E., Kunita, M., Guilherme, M. et al. (2011). NaOH–activated carbon of high surface area produced from coconut shell: Kinetics and equilibrium studies from the methylene blue adsorption. Chemical Engineering Journal, 174(1), 117–125. DOI 10.1016/j.cej.2011.08.058. [Google Scholar] [CrossRef]
31. Aghababaei, A., Ncibi, M., Sillapää, M. (2017). Optimized removal of ox tetracycline and cadmium from contaminated waters using chemically-activated and pyrolyzed biochars from forest and wood-processing residues. Bioresource Technology, 239, 28–36. DOI 10.1016/j.biortech.2017.04.119. [Google Scholar] [CrossRef]
32. Cutillas-Barreiro, L., Paradelo, R., Igrexas-Soto, A. (2016). Valorization of biosorbent obtained from a forestry waste: Competitive adsorption, desorption and transport of Cd, Cu, Ni, Pb and Zn. Ecotoxicology and Environmental Safety, 131, 118–126. DOI 10.1016/j.ecoenv.2016.05.007. [Google Scholar] [CrossRef]
33. Ahmad, T., Rafatullah, M., Ghazali, A., Sulaiman, O., Hashim, R. (2011). Oil palm biomass based adsorbents for the removal of water pollutants—A review. Journal of Environmental Science and Health, 29(3177–222. [Google Scholar]
34. Al-Ghamdi, A., Altaher, H., Omar, W. (2013). Application of date palm trunk fibers as adsorbents for removal of Cd+2 ions from aqueous solutions. Journal of Water Reuse and Desalination, 3(1), 47–54. DOI 10.2166/wrd.2013.031. [Google Scholar] [CrossRef]
35. Pyrzynska, K. (2019). Removal of cadmium from wastewaters with low-cost adsorbents. Journal of Environmental Chemical Engineering, 7(1), 102795. DOI 10.1016/j.jece.2018.11.040. [Google Scholar] [CrossRef]
36. Al-Shayeb, S., Al-Rajhi, M., Seaward, M. (1995). The date palm (Phoenix dactylifera L.) as a biomonitor of lead and other elements in arid environments. Science of the Total Environment, 168(1), l–10. DOI 10.1016/0048-9697(95)04556-G. [Google Scholar] [CrossRef]
37. Alfarooq, B., Marlia, H., Alsaadi, M., Al-Douri, Y., Al-Raad, A. (2021). Synthesis and optimization of high surface area mesoporous date palm fiber-based nanostructure powder activated carbon for aluminum removal. Chinese Journal of Chemical Engineering, 32(4), 472–484. [Google Scholar]
38. Ahmad, T., Rafatullah, M., Ghazali, A., Sulaiman, O., Hashim, R., Ahmad, A. (2010). Removal of pesticides from water and wastewater by different adsorbents: A review. Journal of Environmental Science and Health, 28, 231–271. [Google Scholar]
39. Al-Shawabkeh, A., Hasseine, A., Al-Amayreh, M., Omar, W. (2021). Experimental study of the application of date palm trunk fiber (DPTF) as biosorbent for removal cadmium using a fixed bed column: Investigation of the influence of particle size. Desalination and Water Treatment, 223, 328–334. DOI 10.5004/dwt.2021.27153. [Google Scholar] [CrossRef]
40. Ayoob, S., Gubta, A., Bhakat, P. (2007). Analysis of breakthrough developments and modeling of fixed bed adsorption system for As(V) removal from water by modified calcined bauxite (MCB). Separation and Purification Technology, 52(3), 430–438. DOI 10.1016/j.seppur.2006.05.021. [Google Scholar] [CrossRef]
41. Chu, K. (2010). Fixed bed sorption: Setting the record straight on the Bohart-Adams and Thomas models. Journal of Hazardous Materials, 177(1–3), 1006–1012. DOI 10.1016/j.jhazmat.2010.01.019. [Google Scholar] [CrossRef]
42. Bohart, G., Adams, E. (1920). Some aspect of the behavior of charcoal with respect to chlorine. Journal of the American Chemical Society, 42(3), 523–544. DOI 10.1021/ja01448a018. [Google Scholar] [CrossRef]
43. Kumar, U., Bandyopadhyay, M. (2006). Fixed bed column study for Cd (II) removal from wastewater using treated rice husk. Journal of Hazardous Materials, 129(1–3), 253–259. DOI 10.1016/j.jhazmat.2005.08.038. [Google Scholar] [CrossRef]
44. Volesky, B., Prasetyo, I. (1994). Cadmium removal in a biosorption column. Biotechnology and Bioengineering, 43(11), 1010–1015. DOI 10.1002/(ISSN)1097-0290. [Google Scholar] [CrossRef]
45. Hutchins, R. (1980). Liquid phase adsorption: Maximizing performance. Chemical Engineering Journal, 87(1), 1–110. [Google Scholar]
46. Markovska, L., Meshko, V., Novak, V. (2001). Adsorption of basic dyes in a fixed bed column. Korean Journal of Chemical Engineering, 18(2), 190–195. DOI 10.1007/BF02698458. [Google Scholar] [CrossRef]
47. Yoon, Y., Nelson, J. (1984). Application of Gas adsorption kinetics. I. A theoretical odel for respirator cartridge service life. American Industrial Hygiene Association Journal, 45(8), 509–516. DOI 10.1080/15298668491400197. [Google Scholar] [CrossRef]
48. Walker, G., Weatherly, L. (1997). Adsorption of acid dyes onto granular activated carbon in fixed beds. Water Research, 31(8), 2093–2101. DOI 10.1016/S0043-1354(97)00039-0. [Google Scholar] [CrossRef]
49. Wolborska, A. (1989). Adsorption on activated carbon of p-nitro phenol from aqueous solution. Water, 23(1), 85–91. [Google Scholar]
50. Ayoob, S., Gupta, A. (2007). Sportive response profile of an adsorbent in the defluoridation of drinking water. Chemical Engineering Journal, 133, 273–281. [Google Scholar]
51. Rahman, M., Ali, M., Saha, I., Al Raihan, M., Moniruzzaman, M. et al. (2017). Date palm fiber as a potential low-cost adsorbent to uptake chromium (VI) from industrial wastewater. Desalination and Water Treatment, 88, 169–178. [Google Scholar]
52. Marín, A., Aguilar, M., Ortuño, J., Meseguer, V., Sáez, J. et al. (2010). Biosorption of Zn (II) by orange waste in batch and packed-bed systems. Chemical Technology and Biotechnology, 85(10), 1310–1318. DOI 10.1002/jctb.2432. [Google Scholar] [CrossRef]
53. Mandina, S., Chigondo, F., Shumba, M., Nyamunda, B., Sebata, E. (2013). Removal of chromium (VI) from aqueous solution using chemically modified orange (citrus cinensis) peel. Journal of Applied Chemistry, 6(2), 66–75. [Google Scholar]
54. Wu, J., Yu, H. (2008). Biosorption of 2, 4-dichlorophenol from aqueous solutions by immobilized phanerochaete chrysosporium biomass in a fixed bed column. Chemical Engineering Journal, 138(1–3), 128–135. DOI 10.1016/j.cej.2007.05.051. [Google Scholar] [CrossRef]
Cite This Article
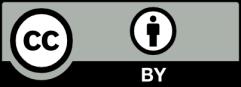