Open Access
ARTICLE
Physicochemical Properties of Combustion Ashes of Some Trees (Urban Pruning) Present in the Neotropical Region
1
Competitiveness and Sustainability for Development Research Group, Faculty of Engineering, Universidad Libre-Cúcuta Sectional,
Cúcuta, Colombia
2
Catalysis and Nanomaterials Research Group, Faculty of Mines, Universidad Nacional de Colombia-Medellín Sectional, Medellín,
Colombia
* Corresponding Author: John Freddy Gelves-Díaz. Email:
Journal of Renewable Materials 2023, 11(10), 3769-3787. https://doi.org/10.32604/jrm.2023.029270
Received 10 February 2023; Accepted 11 May 2023; Issue published 10 August 2023
Abstract
Secondary lignocellulosic biomass has proved to be useful as an energy source through its oxidation by means of combustion processes. In accordance with the above, in this paper, we wanted to study the ash from urban pruning residues that are generated in cities in the Neotropics. Species such as Licania tomentosa, Azadirachta indica, Ficus benjamina, Terminalia catappa, Leucaena leucocephala, Prosopis juliflora and Pithecellobium dulce were selected because they have been previously studied and showed potential for thermal energy generation. These materials were calcined in an oxidizing atmosphere and characterized by X-ray diffraction and fluorescence, scanning electron microscopy with microchemistry, BET surface area, thermal gravimetric analysis, and differential scanning calorimetry. The pH and apparent density were also established. The results show high basicity materials (average pH 10), a behavior associated with the presence of chemical elements such as calcium, potassium, magnesium, chlorine, phosphorus, and sulfur. Structurally, these materials have a very significant amorphous fraction (between 49% and 74.5%), the dominant crystalline phases are calcite, arcanite, sylvite, and hydroxyapatite. These ashes have low surface area and do not exceed 13 m2 /g. Two characteristic morphological aspects were observed in these ashes: a morphology of rounded grains where silicon content is highlighted, and lamellar morphologies where the presence of chlorine is highlighted. Thermally, these ashes show four significant mass loss events (400°C, 430°C, 680°C, and 920°C), causing mass losses that vary between 25% and 40%. Through this study, it was possible to establish that, from a chemical point of view, these ashes are less dangerous in comparison with those of a mineral coal that was used as a reference. However, they require additional treatments for their disposal due to their high basicity. Because of their composition, these ashes have the potential to be used in the ceramic and cement industries, and in the manufacture of fertilizers.Graphical Abstract
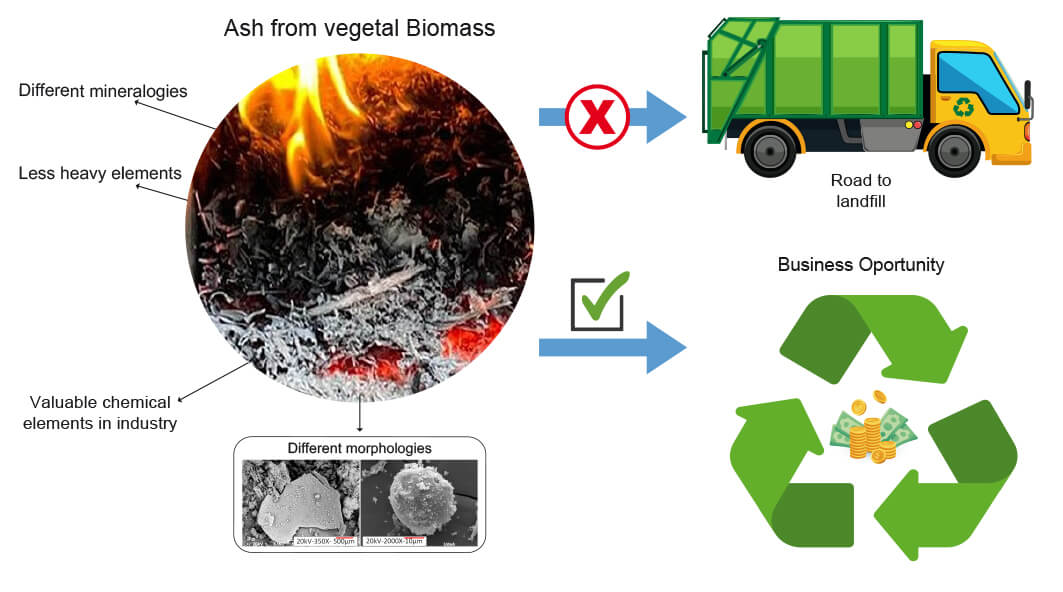
Keywords
Vegetable biomass is an alternative energy source of a renewable character, and considered within the category of neutral carbon. Due to these characteristics, it has been gaining ground as a substitute for fossil fuels such as oil and coal. One of the important factors that has contributed to this situation is the availability of plant biomass around the world from trees cultivated for this purpose, tree pruning from urban areas, and residues from agricultural activity such as rice husks, bagasse from cane, oil palm, corn crop residues, and coconut shells, among others [1–3].
Regardless of the source of origin of the plant biomass, these residues have shown the potential to generate thermal energy, in many cases through combustion processes, achieving the partial or total substitution of fossil fuel, especially coal [4–7]. In the Latin-American environment, these residues have been used in brick kilns, cement factories, and in industrial laundries for steam generation, consuming large amounts of biomass [8–10]. However, as is the case with mineral coal, a negative aspect originates, and it is the generation of ashes and unburned material [11,12].
Combustion ashes can become an environmental and financial problem for organizations that use biomass as fuel, which is why their characterization and the search for applications is important, in order to reduce or avoid expenses in their final disposition. The review of scientific literature shows that interest is increasing in the study of this class of materials, both in terms of environmental aspects and in terms of the possibilities of reusing them. In the Scopus bibliographic manager, for example, it was shown that by the year 2000, an average of 6 articles were published per year on biomass ash. This figure has been growing progressively, reaching 150 publications during 2022. Territories such as China, India, Germany, the United States, Spain, and Brazil are the most representative sources of study regarding the ashes.
Efforts that were carried out revealed investigations concerning aspects of physicochemical characterization [2,13], the effects of calcination temperature on the properties of this class of materials [14], the classification methodologies due to the great diversity of species [15], the affectations of ashes on biomass combustion systems [16–18], the ecotoxicity of these residues [19,20] and a large amount of very valuable work aimed at reusing these residues. Regarding this last aspect, their use as a raw material in the manufacture of traditional cementitious materials [21], in the manufacture of cementitious geopolymers [22] and adsorbent geopolymers [23], as raw material in the ceramic industry [24], in the synthesis of zeolites [25], for fertilization [26], in the capture of carbon dioxide [27], in the support and active phase of catalysts [28,29], as filters [30] and directly as adsorbents [31], stands out. The secondary biomass associated with the urban pruning process has been highlighted in scientific literature as an interesting source of energy, especially in territories such as the Neotropics, where climatic conditions are sustained throughout the year and continuous pruning of trees is necessary due to electrical network maintenance, for the proper visualization of traffic lights, and in ornamentation efforts. In Colombia and other neotropical countries, the existence of trees in street dividers and sidewalks of homes is very common. The city of Cúcuta, for example, which is an intermediate city, has about 400,000 specimens, which can generate about 2,000 tons of vegetable biomass per month as a product of pruning [32], which is not a negligible quantity, and which allows the feeding of many steam generation boilers of companies in said territory. Now it is expected that much larger quantities will be obtained in larger cities such as Bogotá, which has about 1,200,000 trees, a material that could be very useful for the energy needs of many organizations and even for domestic use [33,34].
This amount of pruning waste used as fuel brings with it the generation of at least 12,000 tons of ash per year. There have been problems regarding their disposal or applicability, due to the little knowledge their is about their physicochemical properties. Although many efforts have been carried out that have made it possible to characterize and find applications for these combustion residues [35–37], few studies have been carried out regarding the ashes of species that grow in urban areas of the Neotropics, in countries such as Colombia, Venezuela, Ecuador, Panama, or Mexico, especially of species such as Licania tomentosa, Azadirachta indica, Ficus benjamina, Terminalia catappa, Leucaena leucocephala, Prosopis juliflora, and Pithecellobium dulce [37–41]. Due to the little knowledge that we have concerning the ashes of the aforementioned species, the objective of this study has been aimed at characterizing them in order to know their chemical and structural composition, to identify if there are components that can affect the environment, as well as to infer possible applications based on the characterization results. The high content of leaves, branches, and twigs, in this biomass is distinctive.
Plant biomass from the pruning and ornamentation process in the metropolitan area of Cúcuta (Colombia) was collected for the study. The material was collected directly from the grinding process carried out by companies dedicated to this work in the city. The high content of leaves, branches, and twigs, in this biomass is distinctive.
The relevant species that were characterized and their identification codes are shown in Table 1.
The main physicochemical characteristics were considered in a previous study [42]. However, Table 2 presents some of the relevant aspects that could be useful for discussing the results of the ash, which is the object of study of this research.
The chemical composition of a bituminous mineral coal that was extracted and marketed in the area of influence where this study was carried out [43], was used as a reference to make a comparative analysis on the generation and impact of pruning ash.
The samples of the different species were initially exposed to natural drying for 1 week. Subsequently, they were subjected to artificial drying in an oven at a temperature of 110°C for 8 h. Next, 700 g of each species were taken and subjected to a combustion process in the open air in order to reduce the volume of pruning residue, since there was no container to calcinate all the material directly in the electric oven. The material resulting from the combustion of each residue, still rich in organic matter, was placed in crucibles and simultaneously loaded into an electric furnace (27 liters and an oxidizing atmosphere) in order to complete the calcination. The heating rate was 5°C/min up to 650°C, maintaining this last temperature for 1.5 h. These conditions were established in order to eliminate all organic matter from the samples and try to reduce losses due to potassium volatilization [44]. This temperature was established based on previous results of thermal analysis and heat flux (TG/DSC), where it was shown that, at this temperature, there was no more mass loss associated with organic matter in any of the residues of the eight species that were studied. In the same way, it was considered that this temperature was not far from that which is recommended in the ASTM D 1102-84 standard for determining the ash content in wood samples. From the material that was obtained, a fraction was taken which was ground until the sample passed the 200 Tyler mesh, this in order to perform X-ray diffraction, X-ray fluorescence, thermogravimetric analysis (TG) analysis. and differential scanning calorimetry (DSC). For the development of pH measurements, scanning electron microscopy (SEM), BET surface area and density, the material was used without modifying the granulometry.
The chemical characterization was carried out using the XRF technique, using a Bruker model S8 Tiger 4 kW dispersive wavelength X-ray fluorescence spectrometer. The structural characterization was carried out in a Bruker model D8 Advance powder diffractometer with DaVinci Geometry in the conditions of 40 kV and 40 mA, with a measurement range between 3.5° to 70°, and a counting time of 0.6 s per step. Quantitative analysis was performed by Rietveld refinement, using an internal standard (20% corundum) to determine the amorphous phase. The morphological and microchemical aspects were studied using a JEOL JSM-6490LV model scanning electron microscope. A sputtering type gold coating system was used with Denton Vacuum Desk IV equipment. The surface area (BET) was established with ASAP 2020 micromeritics equipment using nitrogen at −196°C (77 K) as a molecule for adsorption, and degassing was performed at 250°C for 12 h. The TG/DSC analysis was carried out in TA Instrument model SDT Q-600 equipment, using a mass of 10.0 mg in an alumina crucible, a maximum analysis temperature of 1100°C, heating rate of 10°C/min, and dry air flow of 50 ml/min. The pH measurements were made using a Hanna Instruments model HI 99121 pH meter for soils. The apparent density was carried out using a 25 ml cylindrical cylinder as a containment element, with the help of an Ohaus brand analytical scale with a resolution of 0.01 to determine the mass.
3.1 Chemical and Structural Aspects
The first aspect to report has to do with the fraction recovered as ash, after the heating process (650°C) in an oxidizing atmosphere in the electric furnace. The results are presented in Table 3.
Table 3 shows the variability in the amount of ash obtained for each residue. The higher ash content generated by the Ficus benjamina species, and, in the opposite case, the lower ash values provided by Leucaena pruning is worth highlighting. If the ash is considered as just a residue and, incidentally, this material must be disposed of in a landfill to avoid environmental impacts associated with the basicity of the soil and/or leaching of polluting elements [2,11,12], the pruning of the species Ficus would be a bigger problem compared to the other biomass residues associated with other tree species. Now, if these ashes have a technological and commercial value, the situation would be the opposite, where it would be advisable to use the species that generate the highest ash content. That is why it is important to have knowledge of the chemical and structural composition of these residues, in order to be able to make more accurate decisions about the final disposal methods, about how to handle them during combustion, and about the potential use of the ashes under the guidelines of the circular economy [45]. The chemical and structural information obtained for the study samples are presented in Tables 4 and 5.
From the information presented in Table 4, it is evident that in all the ashes obtained from pruning residues (see Table 1), calcium is the main element, followed by potassium. In the case of calcium, its existence is related to the presence of carbonated crystalline phases such as calcite, dolomite, and fairchildite, and also in phases such as hydroxyapatite. However, there must be a large amount of this element in the non-crystalline phase, due to the high amorphous content evidenced in all the samples through the Rietveld refinement that was performed (see Table 5). Regarding potassium, something similar happens: its existence is correlated with the presence of crystalline phases such as arcanite and sylvite, and, to a lesser extent, by the phosphide and potassium sulfide phases. Its existence in the amorphous fraction is also possible due to its flux role [46].
Other representative elements in the samples are silicon, magnesium, phosphorus and sulfur, but their representativeness is strongly influenced by the type of species. In the case of silicon, its presence is favored in the species Licania tomentosa, Ficus benjamina, Terminalia catappa, and Pithecellobium dulce, being structurally related to the presence of phases such as quartz, hydroxyapatite, and the amorphous phase, which is rich in silicon and aluminum (see Table 5). Regarding phosphorus, its presence is related to the existence of phases such as hydroxyapatite, potassium phosphide, and traces of iron and nickel phosphate. The highest concentration of this element is favored in the specie Pithecellobium dulce. In the case of magnesium, its presence is favored in the species Terminalia catappa and, once again, in Pithecellobium dulce. Structurally, it can be associated with the presence of carbonates such as dolomite and the periclase phase, according to the X-ray diffraction analysis presented in Table 5. Finally, in the case of sulfur, its presence is related to that described for phosphorus, and, structurally, this sulfur can be related to the presence of arcanite and, to a lesser extent, to the presence of zinc sulfide and potassium sulfide.
As for minor elements, there is the presence of chlorine, iron, aluminum, and sodium. Chlorine concentrations are evident in all the ashes, and its content varies according to the species, highlighting, in general terms, a greater presence of this element with respect to wood biomass [15]. One explanation for this situation has to do with the fact that pruning residues have a high fraction of material associated with leaves and it is there that a large amount of this element tends to accumulate [47]. Structurally, chlorine is related to the presence of sylvite in the ashes, according to the results in Table 5. Iron and aluminum are quite scarce in the ashes, as can be seen in Table 4. Terminalia catappa is the species with the highest concentration of these elements as well as sodium, which turns out to be a special case within the materials that were analyzed, due to its high concentration, which is higher than that which is found in many industrial minerals containing this element [48]. The iron in the ash is related to the presence of iron oxide and iron phosphate. In the case of aluminum and sodium, it is very likely that their presence is more related to the amorphous phase, although in the case of Pithecellobium dulce, sodium was found as sodium peroxide.
As trace elements, Table 4 shows around 23 elements, where strontium, zinc, manganese, copper, titanium, and rubidium are present in all materials. However, it is important to highlight that, out of all the elements that were identified, greater attention should be paid to those that are considered heavy or toxic [11,12], highlighting in this article the presence of zinc, copper, nickel, chromium, lead, barium, and manganese present in plant biomass ashes. It is relevant to highlight the non-presence of other elements that are more harmful than if they are present in the coal ashes, as in the case of arsenic, vanadium, yttrium and cobalt.
Regarding the trace elements that have a polluting role (see Table 4), it is evident that in the studied ashes, they can be found in low concentrations, and could hardly become harmful in the landfills where they are deposited. In the case of barium, in scientific literature, dangerous concentrations above 500 mg/kg [49] are expressed, values that are not obtained in the biomass ashes that were studied, but in the reference coal. In the case of manganese, dangerous concentrations for plants are around 1500 mg/kg [49], it is once again evident that none of the ashes under study exceed this reference value. In the case of copper, the concentrations in the ashes are between 200 and 400 mg/kg. These values are above the typical values in soils [49], where values of up to 100 mg/kg are expected. Therefore, one can conclude that actions should be taken in order to treat these ashes, for example, its mixture with other soils in order to reduce its concentration. In relation to nickel in the ashes studied, Table 4 shows values between 30 and 100 mg/kg. These values are within the range established in soils, which is between 1 and 200 mg/kg [49]. In the case of zinc, concentrations between 400 and 700 mg/kg are evident in the ashes, and its concentration is higher than that reported in soils, which ranges from 10 to 300 mg/kg [49]. Once again, one can conclude that there is a need for the treatment of these ashes before being discarded, if this is the case. Finally, regarding the content of lead, scientific literature reports concentrations in soil between 3 and 189 mg/kg [49,50], and the data in the ashes that were studied does not exceed 60 mg/kg, which generates some peace of mind concerning its impact on the ground.
There are several works in scientific literature that have laid the foundations for classifying biomass combustion ashes. The works of Zhai and his team [15] and of Vassilev and co-workers [3,16] are highlighted, which have been taken as a guide to carry out the analysis.
In the case of the work of Zhai and his co-workers, they present four categories to classify biomass ashes according to their origin, which are called: hardwoods, softwoods, cereal crop residues, and crop residues other than cereals. The first category is related to the use of the stem of the plant, characterized by having a high concentration of calcium (average of 37.4%) and low silicon content (2.7% on average). In the case of Softwoods, although they are also stems, they are characterized by having a lower calcium content compared to that of hardwoods (average of 32.0%), in which case the presence of silicon is favored (average of 20.1%). The other categories have the particularity that they have a lower calcium content (less than 20%) than wood and higher chlorine content (greater than 7%). However, there is no common pattern for the other relevant elements such as silicon, potassium, sulfur. Pasture residues and small crop branches tend to have a higher potassium content, while agro-industrial crop residues tend to have higher silicon contents in their composition, rice husks being a representative case of this last group [18].
In general terms, and in accordance with the data in Table 4, the materials studied cannot be strictly categorized into one of the four categories mentioned, due to the fact that fractions of wood, branches, and leaves are included, which means that there are high contributions of the calcium associated with the thicker branches of the trees, and of the potassium that seems to be more associated with the fine branches and leaves that are present, which are quite representative in the vast majority of species. This last argument also explains the chlorine content.
3.2 Density, Surface Area, and pH Measurements of the Residues
Other important aspects of the ashes have to do with their basicity or acidity, a very important aspect to take into account for final disposal, for handling, and even for the potential use that can be given to this class of materials. In the same way, it is valuable to know the apparent density of these materials, considering its future applicability and its transportation. Likewise, it is valuable to know its surface area, considering its physical and chemical surface interrelationship with other species, and the possibility of acting as a support for other phases, as in the case of catalysts. The results obtained are presented in Table 6.
From the information in Table 6, it is evident that all the materials studied have a high basicity, especially the ashes of Ficus benjamina. These values have been taken while dry, and an increase of this parameter is very probable when moisture is added, due to the formation of OH groups [51]. These values that were obtained do not differ significantly from those reported for other biomass ashes according to scientific literature [12]. This result makes it possible to prove the need to carry out actions in case the ash is only disposed of in a landfill, since it would affect the natural pH of the soil, which in many cases has a slight acid tendency due to the high content of silicon and aluminum, hence both the surrounding flora and fauna would be affected. From the point of applicability, these ashes could play an important role as a conditioner for acidified soils, acting as a neutralizer as well as providing fertilizing elements such as potassium, phosphorus, chlorine and sulfur, as it has been previously mentioned.
Regarding surface area (BET), it is evident in Table 6 that all the ashes from the biomass that underwent heat treatment have quite low values, and even tend to be lower than those reported for other ashes in the same group, such as rice, coffee, or wood [52,53]. This behavior could be explained by the high presence of potassium, which, at the combustion temperatures used to obtain the ashes, ends up acting as a flux, favoring the formation of the glassy phase, which significantly reduces the porosity, as it occurs in the ceramic industry [48]. This undoubtedly reduces the surface area with which the probe molecule can act. It is noteworthy that the higher values of surface area in Licania tomentosa and Pithecellobium dulce could have a correlation with the higher content of silicon and phosphorus present in these materials, according to the information in Table 4. These results obtained reduce the possibilities of using these ashes in applications where other materials of the same nature have found applications, such as absorption/adsorption of chemical species, especially in environmental remediation, phase separation, and catalyst design [54,55]. Despite the low surface area values, this class of residues could have interesting adsorption applications, such as those described by Liu et al. [27]. These authors highlight the synergy of biochar and ash from organic materials in order to capture carbon dioxide, despite having a low surface area (less than 12 m2/g), noting that ash plays a very relevant role in the process due to their high calcium oxide content.
Regarding apparent density, it is highlighted that the lowest values were obtained in Pithecellobium dulce and Ficus benjamina, and their greater pore volume makes the apparent density lower. Although there should be some direct relationship with the surface area parameter, this is not appreciated in the case of the Ficus species. The higher concentration of elements lighter than calcium, such as silicon and phosphorus, the residual carbon, and even the low concentration of calcium and iron, could explain this behavior in some way. In terms of applicability and even final disposal of the ashes found in Pithecellobium dulce and Ficus benjamina, it could be a problem concerning transportation from the combustion/gasification site to the site where it will be reused or the final landfill, since it occupies more volume, and it may therefore require making more routes in the cargo system, or hiring more containers to transport said mass.
3.3 Thermogravimetric and Heat Flow Analysis
This analysis is valuable in order to know the mass changes during heating, and to identify reaction and/or transformation events, as well as the heat fluxes as the temperature increases. The results that were obtained are presented in Fig. 1, as well as in Table 7.
Figure 1: Thermal profiles of the studied residues. (a) Derivate weight loss, (b) Heat flow. A: Licania tomentosa; B: Azadirachta indica; C: Ficus benjamina; D: Terminalia catappa; E: Leucaena leucocephala; F: Prosopis juliflora; G: Pithecellobium dulce
Based on the information in Fig. 1a, it is possible to show how all the ashes are affected by heating in relation to their mass. This figure shows events of mass loss at different temperatures, with signals of greater intensity near 425°C, 675°C, and 922°C. Table 7 shows the consolidation of said events for the ashes of each of the species studied. Low temperature events around 130°C could be related to physically adsorbed water [56] which is only evident in three species (Licania tomentosa, Pithecellobium dulce and Prosopis juliflora); regarding the other ashes. The mass losses in this zone were in the range of 1.4% to 5.3%, the highest value being that obtained by Licania tomentosa while the smallest was given by Terminalia catappa. Events between 390°C and 440°C are associated with the decomposition of residual organic matter. This is supported by the heat flow results shown in Fig. 1b, where exothermic events are observed in this area (downward concavity). The ashes of Licania tomentosa, Prosopis juliflora, and Pithecellobium dulce are the materials that still contain residual organic matter, which would be associated with a greater resistance to oxidation, since all the ashes were calcined simultaneously and under the same preparation conditions. Mass losses in this region were between 0.9% and 22%, Leucaena leucocephala being the ash with the lowest organic matter content, while Licania tomentosa was the material with the highest losses. The explanation for this behavior is quite complex. One possible hypothesis could be related to the structural composition of the residues (see Table 2), and its stability to thermal oxidation. The results of mass spectrometry coupled to a thermal analyzer presented in a previous study [42] demonstrate that most of these residues show a CO2 formation event in the region where lignin usually decomposes. On the other hand, some of the residues, such as Prosopis juliflora and Pithecellobium dulce do not present a clear signal in the region of hemicellulose and cellulose decomposition. This is related to the formation of carbon monoxide and the difficulty of thermal oxidation of these structural phases in some plant biomasses, which hinders the transition of carbon from a solid state to a gas. From Table 2, for example, it is noticeable that the pruning residues of Licania tomentosa, Prosopis juliflora and Pithecellobium dulce are precisely those with the highest cellulose and even hemicellulose content. Mass loss events above 600°C (see Table 7) are of the endothermic type, according to the information shown in Fig. 1b. The explanation for this mass loss would be associated with the decomposition of crystalline and possibly amorphous carbonate phases, which can be observed in the microstructural analysis presented in Table 5. This includes magnesite and kutnohorite in events close to 700°C, and dolomite and calcite with decompositions above 800°C [57]. The decomposition of sylvite and arcanite occurs near 1100°C. Fig. 1 only showed one event around 1080°C [17]. The mass losses in this last region are between 10.6% and 37.0%, the lowest value being that of Licania tomentosa and the highest being that of Leucaena leucocephala. Species such as Azadiracta indica (29.8%), Terminalia catappa (29.6%) and Prosopis juliflora (31.9%) also reach high values of mass loss in this region, which shows the large amount of carbonates that are present, which can be both in a crystalline and amorphous state, as described in Table 5. The total mass losses of the ashes within the evaluated temperature range are quite significant, reaching values close to 40%, as shown in Table 7. It is noted that the lowest total mass loss in the ashes is obtained in the species Ficus benjamina and Pithecellobium dulce, which would be the ones that would generate the highest yield (in terms of mass) in case this residue is used as a raw material in some application with high temperature treatment, and in turn would not be releasing carbon dioxide, which is known to contribute to the phenomenon of global warming [1].
3.4 Morphological and Microchemical Analysis of the Ash by Electron Microscopy
The morphological and microchemical characterization can be valuable in order to explain some behaviors evidenced with previous techniques. It is also useful to find new similarities or differences between samples. The most representative results are presented in Figs. 2 and 3, as well as in the information in Table 8.
Figure 2: Micrographs of the ashes obtained by SEM. (a) Licania tomentosa, (b) Azadirachta indica, (c) Ficus benjamina, (d) Terminalia catappa, (e) Leucaena leucocephala, (f) Prosopis juliflora, (g) Pithecellobium dulce
Figure 3: Differentiating morphologies of the ashes obtained by SEM. (a) and (b) being spherical morphologies, (c) and (d) being lamellar and fibrous morphologies
The information in Fig. 2 shows the presence of lamellar morphologies and fibers that can be associated with the presence of residual organic matter. This assertion is based on a preliminary microchemical review and on the correlation that occurs with the results of the Figure, where it was possible to show that the greatest number of these morphologies occurred in the ashes of the species Licania tomentosa and Prosopis juliflora. The morphological characteristics of these fibers and sheets could explain the surface area results, associated with their greater porosity, as seen in the micrograph of the Licania tomentosa species. On the other end are the morphological characteristics of the ashes of species such as Azadirachta indica and Leucaena, where no residual organic matter was found. These ashes are characterized by the presence of agglomerates ranging from 5 to about 250 micrometers, which present formation of a glassy phase, most likely associated with the high potassium and phosphorus contents in all the ashes and their combination with silicon and calcium. This behavior contributes to reducing the open porosity, as it was already explained in Section 3.2, thus leaving less available surface area, which explains the results shown in Table 6.
An additional characteristic evidenced in the ashes that were studied was the presence of two morphologies that differ in their chemical composition. In Fig. 3, four images of different ashes have been included where this behavior was shown. There, it is possible to observe spherical and lamellar and fibrous morphologies. In the case of spherical morphologies (Figs. 3a and 3b), their microchemistry (see Table 8) is characterized by having a high silicon content, and they were seen more frequently in the ashes of Ficus benjamina and Licania tomentosa. This same type of morphology is usually found in some bottom and fly ashes from mineral coal, where the predominant chemical element is precisely silicon. The other type of morphology (Figs. 3c and 3d) that was observed is characterized by having a high chlorine content in its composition. There, it is possible to find the presence of carbon in its composition, it has less oxygen content compared to the first type of morphology, and it lacks silicon in its composition. This information from Table 8 reveals the influence of the chemical composition on the morphology of the ashes.
3.5 Possible Applications of Ashes from Pruning Residues
Based on the characterization results that were obtained, possible applications for each of the residues are proposed. The results that were obtained are shown in Table 9.
Based on the results shown in Table 9, it can be seen that all residues may have applicability in some processese, highlighting the greater versatility of Licania tomentosa, Ficus benjamina and Pithecellobium dulce ashes. More detailed studies must be carried out in order to corroborate if the approaches presented here are really valid, since the analysis has been carried out based on the results of previous works found in scientific literature [13,21–27].
The investigation that was carried out made it possible to obtain relevant information which could be of interest for future investigations, since there are no characterization reports of many of the ashes of the species studied. It was possible to show that there are important differences between the ashes from pruning residues and other ashes from other biomasses. The ash contents in the species studied vary between 3% and 10% (weight). The study of the chemical and structural aspects showed that these ashes have low concentrations of polluting elements in comparison with other references, such as mineral coal. However, treatments are required for the ash disposal, especially regarding the management of basicity, which is very high (with values greater than 10). In the same way, the potential of some of the species to store some type of chemical element in particular was shown. For example, Terminalia catappa is characterized by its high sodium content, which is not common in the other biomass ashes that were studied. It was possible to demonstrate that these ashes are affected by being subjected to heating. Some of them still retain some residual organic phase, and similarly, the loss of mass associated with the decomposition of carbonate species was demonstrated, as seen by the X-ray diffraction analysis. Morphological and microchemical analysis showed two types of characteristic morphologies in the ashes studied, which are related to the highest silicon (spherical) or chlorine (lamellar) content. Finally, using the information from the characterization carried out, it could be deduced that these ashes could have application potential in the cement industry, ceramic industry, and fertilizers.
Funding Statement: The authors thank the Ministry of Science, Technology and Innovation of Colombia through the “Fondo Francisco José de Caldas” National Financing Fund for Science, Technology and Innovation for the financing provided for the development of the project (Project 120885272102, Call 852 of 2019). They also express their gratitude to the Universidad Libre and the Universidad Nacional de Colombia for the support provided for the development of this research.
Author Contributions: The authors declare that all the efforts made for the construction of this work were carried out jointly or in groups, it is not possible to discriminate the role performed by each of the researchers.
Conflicts of Interest: The authors declare that they have no conflicts of interest to report regarding the present study.
References
1. Panwar, N. L., Kaushik, S. C., Kothari, S. (2011). Role of renewable energy sources in environmental protection: A review. Renewable and Sustainable Energy Reviews, 15(3), 1513–1524. [Google Scholar]
2. Vassilev, S. V., Vassileva, C. G., Vassilev, V. S. (2015). Advantages and disadvantages of composition and properties of biomass in comparison with coal: An overview. Fuel, 158(2), 330–350. [Google Scholar]
3. Nunes, L. J. R., Causer, T. P., Ciolkosz, D. (2020). Biomass for energy: A review on supply chain management models. Renewable and Sustainable Energy Reviews, 120(2), 109658. [Google Scholar]
4. Klingenberg, D., Nolasco, A. M., Júnior, A. F. D., Candaten, L., Cavalcante, A. K. L. et al. (2020). Energy potential of wood waste from a tropical urban forest. Research, Society and Development, 9(9), e451997478. [Google Scholar]
5. Klingenberg, D., Nolasco, A. M., Candaten, L., Cavalcante, A. K. L., Aguiar, D. L. et al. (2021). Drying wastes from urban forestry as an option for their recovery and use in furniture and small wooden objects. Environment, Development and Sustainability, 24(10), 11615–11625. [Google Scholar]
6. Demirbas, A. (2005). Potential applications of renewable energy sources, biomass combustion problems in boiler power systems and combustion related environmental issues. Progress in Energy and Combustion Science, 31(2), 171–192. [Google Scholar]
7. De Meira, A. M., Nolasco, A. M., Klingenberg, D., de Souza, E. C., Dias Júnior, A. F. (2021). Insights into the reuse of urban forestry wood waste for charcoal production. Clean Technologies and Environmental Policy, 23(10), 2777–2787. [Google Scholar]
8. de Souza, J. E. A., Paes Filho, F. D. A., da Silva, B. P. C., Tenório, N. V. N., de Sousa, F. J. et al. (2012). Biomass residues as fuel for the ceramic industry in the state of Alagoas: Brazil. Waste and Biomass Valorization, 3(2), 191–196. [Google Scholar]
9. Da Costa, G. G., dos Santos, I. F. S., Barros, R. M., Tiago Filho, G. L., de Oliveira, M. et al. (2022). Mapping and energy analysis of Brazilian bioenergy power potential for three agricultural biomass byproducts. Journal of Cleaner Production, 349(6), 131466. [Google Scholar]
10. Silva, D. A. L., Filleti, R. A. P., Musule, R., Matheus, T. T., Freire, F. (2022). A systematic review and life cycle assessment of biomass pellets and briquettes production in Latin America. Renewable and Sustainable Energy Reviews, 157, 112042. [Google Scholar]
11. Munawar, M. A., Khoja, A. H., Naqvi, S. R., Mehran, M. T., Hassan, M. et al. (2021). Challenges and opportunities in biomass ash management and its utilization in novel applications. Renewable and Sustainable Energy Reviews, 150, 111451. [Google Scholar]
12. Barbosa, R., Dias, D., Lapa, N., Lopes, H., Mendes, B. (2013). Chemical and ecotoxicological properties of size fractionated biomass ashes. Fuel Processing Technology, 109, 124–132. [Google Scholar]
13. Vassilev, S. V., Baxter, D., Andersen, L. K., Vassileva, C. G. (2013). An overview of the composition and application of biomass ash. Part 1. Phase-mineral and chemical composition and classification. Fuel, 105(12–13), 40–76. [Google Scholar]
14. Xiao, R., Chen, X., Wang, F., Yu, G. (2011). The physicochemical properties of different biomass ashes at different ashing temperature. Renewable Energy, 36(1), 244–249. [Google Scholar]
15. Zhai, J., Burke, I. T., Mayes, W. M., Stewart, D. I. (2021). New insights into biomass combustion ash categorisation: A phylogenetic analysis. Fuel, 287, 119469. [Google Scholar]
16. Vassilev, S. V., Baxter, D., Vassileva, C. G. (2014). An overview of the behaviour of biomass during combustion: Part II. Ash fusion and ash formation mechanisms of biomass types. Fuel, 117(3), 152–183. [Google Scholar]
17. Mlonka-Mędrala, A., Magdziarz, A., Gajek, M., Nowińska, K., Nowak, W. (2020). Alkali metals association in biomass and their impact on ash melting behaviour. Fuel, 261(2), 116421. [Google Scholar]
18. Du, S., Yang, H., Qian, K., Wang, X., Chen, H. (2014). Fusion and transformation properties of the inorganic components in biomass ash. Fuel, 117, 1281–1287. [Google Scholar]
19. Mierzwa-Hersztek, M., Gondek, K., Jewiarz, M., Dziedzic, K. (2019). Assessment of energy parameters of biomass and biochars, leachability of heavy metals and phytotoxicity of their ashes. Journal of Material Cycles and Waste Management, 21(4), 786–800. [Google Scholar]
20. Carlson, C. L., Adriano, D. C. (1993). Environmental impacts of coal combustion residues. Journal of Environmental Quality, 22(2), 227–247. [Google Scholar]
21. Kim, K. W., Park, K. T., Ates, F., Kim, H. G., Woo, B. H. (2023). Effect of pretreated biomass fly ash on the mechanical properties and durability of cement mortar. Case Studies in Construction Materials, 18, e01754. [Google Scholar]
22. Thomas, B. S., Yang, J., Mo, K. H., Abdalla, J. A., Hawileh, R. A. et al. (2021). Biomass ashes from agricultural wastes as supplementary cementitious materials or aggregate replacement in cement/geopolymer concrete: A comprehensive review. Journal of Building Engineering, 40(7), 102332. [Google Scholar]
23. Novais, R. M., Carvalheiras, J., Tobaldi, D. M., Seabra, M. P., Pullar, R. C. et al. (2019). Synthesis of porous biomass fly ash-based geopolymer spheres for efficient removal of methylene blue from wastewaters. Journal of Cleaner Production, 207, 350–362. [Google Scholar]
24. Labaied, I., Douzane, O., Lajili, M., Promis, G. (2022). Bricks using clay mixed with powder and ashes from lignocellulosic biomass: A review. Applied Sciences, 12(20), 10669. [Google Scholar]
25. Belviso, C. (2018). State-of-the-art applications of fly ash from coal and biomass: A focus on zeolite synthesis processes and issues. Progress in Energy and Combustion Science, 65(1), 109–135. [Google Scholar]
26. Silva, F. C., Cruz, N. C., Tarelho, L. A., Rodrigues, S. M. (2019). Use of biomass ash-based materials as soil fertilisers: Critical review of the existing regulatory framework. Journal of Cleaner Production, 214, 112–124. [Google Scholar]
27. Liu, J., Zhang, W., Jin, H., Li, Z., Liu, G. et al. (2023). Exploring the carbon capture and sequestration performance of biochar-artificial aggregate using a new method. Science of the Total Environment, 859, 160423. [Google Scholar] [PubMed]
28. He, Q., Yu, J., Song, X., Ding, L., Wei, J. et al. (2020). Utilization of biomass ash for upgrading petroleum coke gasification: Effect of soluble and insoluble components. Energy, 192(8), 116642. [Google Scholar]
29. Maneerung, T., Kawi, S., Wang, C. H. (2015). Biomass gasification bottom ash as a source of CaO catalyst for biodiesel production via transesterification of palm oil. Energy Conversion and Management, 92, 234–243. [Google Scholar]
30. Lanzerstorfer, C. (2015). Chemical composition and physical properties of filter fly ashes from eight grate-fired biomass combustion plants. Journal of Environmental Sciences, 30(9), 191–197. [Google Scholar]
31. Saldarriaga, J. F., Montoya, N. A., Estiati, I., Aguayo, A. T., Aguado, R. et al. (2021). Unburned material from biomass combustion as low-cost adsorbent for amoxicillin removal from wastewater. Journal of Cleaner Production, 284(7), 124732. [Google Scholar]
32. Gelves, J., Rozo, S., Monroy, R., Romero, Y., Dorkis, L. (2022). Residuos de poda en el Área Metropolitana de Cúcuta: Potencial energético de algunas especies presentes en el territorio. In: Investigación, desarrollo tecnológico e innovación en ingeniería y administración. Cúcuta: Editorial Servicio Nacional de Aprendizaje (in Spanish). [Google Scholar]
33. Dobbs, C., Hernández-Moreno, Á., Reyes-Paecke, S., Miranda, M. D. (2018). Exploring temporal dynamics of urban ecosystem services in Latin America: The case of Bogota (Colombia) and Santiago (Chile). Ecological Indicators, 85(9), 1068–1080. [Google Scholar]
34. Escobedo, F. J., Clerici, N., Staudhammer, C. L., Feged-Rivadeneira, A., Bohorquez, J. C. et al. (2018). Trees and crime in Bogota, Colombia: Is the link an ecosystem disservice or service? Land Use Policy, 78(6), 583–592. [Google Scholar]
35. Cruz, N. C., Silva, F. C., Tarelho, L. A., Rodrigues, S. M. (2019). Critical review of key variables affecting potential recycling applications of ash produced at large-scale biomass combustion plants. Resources, Conservation and Recycling, 150, 104427. [Google Scholar]
36. Paula, L., Trugilho, P. F., Napoli, A., Bianchi, M. L. (2011). Characterization of residues from plant biomass for use in energy generation. Cerne, 17(2), 237–246. [Google Scholar]
37. Maurcio, L., Fearnside, P. M., Cerri, C. C. (1999). Burning of Amazonian forest in Ariquemes, Rondônia, Brazil: Biomass, charcoal formation and burning efficiency. Forest Ecology and Management, 120(1–3), 179–191. [Google Scholar]
38. Klingenberg, D., Nolasco, A. M., Candaten, L., Cavalcante, A. K. L., Aguiar, D. L. et al. (2022). Drying wastes from urban forestry as an option for their recovery and use in furniture and small wooden objects. Environment, Development and Sustainability, 24(10), 11615–11625. [Google Scholar]
39. Pérez-Arévalo, J. J., Velázquez-Martí, B. (2018). Evaluation of pruning residues of Ficus benjamina as a primary biofuel material. Biomass and Bioenergy, 108(5), 217–223. [Google Scholar]
40. Nugroho, A., Pambudi, N. A., Harjanto, B., Febryanto, A., Firdaus, R. A. et al. (2019). Production of solid fuel by hydrothermal treatment using Terminalia catappa peels waste as renewable energy sources. Journal of Physics: Conference Series, 1153(1), 012083. [Google Scholar]
41. Bandara, W., Ranasinghe, O., Perera, P., Vlosky, R., Kizha, A. R. (2022). Potential to use invasive plants in biomass energy production: A case study Prosopis juliflora in coastal wetlands of Sri Lanka. Trees, Forests and People, 10(1), 100330. [Google Scholar]
42. Gelves, J., Rozo, S., Monroy, R., Camargo, G., Dorkis, L. (2023). Urban plant biomass residues from the neotropics and their potential for thermal energy generation. Journal of Renewable Materials (in Press), 11(9), 3547–3566. https://doi.org/10.32604/jrm.2023.029267 [Google Scholar] [CrossRef]
43. Sánchez, J., Corpas, F., Álvarez, D. (2018). Aplicaciones de los nutrientes tecnológicos en la industria cerámica del área metropolitana de Cúcuta. San José de Cúcuta: Editorial Universidad Francisco de Paula Santander (in Spanish). [Google Scholar]
44. Thy, P., Jenkins, B. M., Grundvig, S., Shiraki, R., Lesher, C. (2006). High temperature elemental losses and mineralogical changes in common biomass ashes. Fuel, 85(5–6), 783–795. [Google Scholar]
45. Sherwood, J. (2020). The significance of biomass in a circular economy. Bioresource Technology, 300, 122755. [Google Scholar] [PubMed]
46. Iloghalu, F. I., Nnuka, E. E. (2014). Evaluation of the fluxing potentials of okene potassium feldspar for silicate ceramics production. Evaluation, 1(4), 288–299. [Google Scholar]
47. Zalesny, J. A., Zalesny, R. S., Wiese, A. H., Sexton, B., Hall, R. B. (2008). Sodium and chloride accumulation in leaf, woody, and root tissue of Populus after irrigation with landfill leachate. Environmental Pollution, 155(1), 72–80. [Google Scholar] [PubMed]
48. Alvarez-Rozo, D., Sánchez-Molina, J., Gelves, J. F. (2017). Influence of raw materials and forming technique in the manufacture of stoneware ceramic. Ingeniería y competitividad, 19(2), 93–105. [Google Scholar]
49. Macias, F., Calvo, R. (2009). Niveles genéricos de referencia de metales pesados y otros elementos traza en suelos de Galicia. Santiago de Compostela: Editorial Xunta de Galicia (in Spanish). [Google Scholar]
50. Kabata-Pendias, A., Pendias, H. (2001). Trace elements in soils and plants. Boca Raton: CRC Press Inc. [Google Scholar]
51. Supancic, K., Obernberger, I., Kienzl, N., Arich, A. (2014). Conversion and leaching characteristics of biomass ashes during outdoor storage-results of laboratory tests. Biomass and Bioenergy, 61(1/2), 211–226. [Google Scholar]
52. Bakar, R. A., Yahya, R., Gan, S. N. (2016). Production of high purity amorphous silica from rice husk. Procedia Chemistry, 19, 189–195. [Google Scholar]
53. Trivedi, N. S., Mandavgane, S. A., Mehetre, S., Kulkarni, B. D. (2016). Characterization and valorization of biomass ashes. Environmental Science and Pollution Research, 23(20), 20243–20256. [Google Scholar] [PubMed]
54. Gokhale, N. A., Trivedi, N. S., Mandavgane, S. A., Kulkarni, B. D. (2020). Biomass ashes as potent adsorbent for pesticide: Prediction of adsorption capacity by artificial neural network. International Journal of Environmental Science and Technology, 17(6), 3209–3216. [Google Scholar]
55. Herman, A. P., Yusup, S., Shahbaz, M., Patrick, D. (2016). Bottom ash characterization and its catalytic potential in biomass gasification. Procedia Engineering, 148, 432–436. [Google Scholar]
56. Cheng, K., Winter, W. T., Stipanovic, A. J. (2012). A modulated-TGA approach to the kinetics of lignocellulosic biomass pyrolysis/combustion. Polymer Degradation and Stability, 97(9), 1606–1615. [Google Scholar]
57. Földvári, M. (2011). Handbook of thermogravimetric system of minerals and its use in geological practice. Budapest: Geological Institute of Hungary. [Google Scholar]
Cite This Article
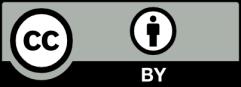