Open Access
REVIEW
Valorization of Aloe barbadensis Miller. (Aloe vera) Processing Waste
1
SISSTEM Program, Faculty of Arts and Science, University of Aruba, Oranjestad, Aruba
2
Department of Chemistry, KU Leuven, Leuven, Belgium
3
Department of Chemical Engineering, KU Leuven, Leuven, Belgium
* Corresponding Author: Pedro Fardim. Email:
(This article belongs to the Special Issue: Renewable Material from Agricultural Waste and By-Product and Its Applications)
Journal of Renewable Materials 2023, 11(3), 1031-1061. https://doi.org/10.32604/jrm.2022.023449
Received 26 April 2022; Accepted 23 June 2022; Issue published 31 October 2022
Abstract
Aloe vera plant is known worldwide for its medicinal properties and application in gel-based products such as shampoo, soap, and sunscreen. However, the demand for these gel-based products has led to a surplus production of Aloe vera processing waste. An Aloe vera gel processing facility could generate up to 4000 kg of Aloe vera waste per month. Currently the Aloe vera waste is being disposed to the landfill or used as fertilizer. A sustainable management system for the Aloe vera processing waste should be considered, due to the negative societal and environmental impacts of the currents waste disposal methods. Therefore, this review focuses on various approaches that can be used to valorize Aloe vera waste into value-added products, such as animal and aquaculture feeds, biosorbents, biofuel and natural polymers. Researchers have reported Aloe vera waste for environmental applications biosorbents used for wastewater treatment of various pollutants. Several studies have also reported on the valorization of Aloe vera waste for production of biofuels such as bioethanol, mixed alcohol fuels, biogas and syngas. Aloe vera waste could also be valorized through isolation and synthesis of natural polymers for application in wound dressing, tissue engineering and drug delivery systems. Aloe vera waste valorization was also reviewed through extraction of value-added bioactive compounds such as aloe-emodin, aloin and aloeresin. These value-added bioactive compounds have various applications in the cosmetics (non-steroidal anti-inflammatory, tyrosinase inhibitors) and pharmaceutical (anticancer agent and COVID 19 inhibitors) industry.Graphic Abstract
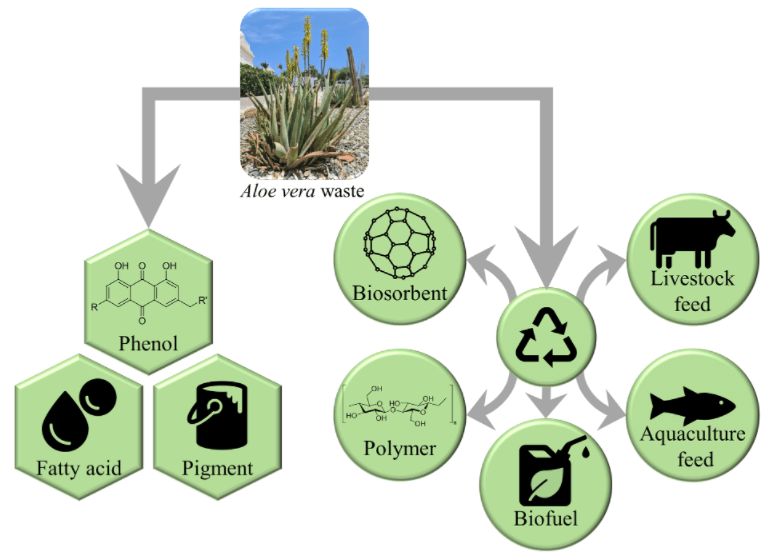
Keywords
Aloe species are perennial and drought resistant plants from the Aloaceae family. There are 558 Aloe species broadly distributed around the world [1]. Aloe species originated from South Africa about 19 million years ago, but migrated to Madagascar, West Africa, Saharan-Sudanian region and Arabian Peninsula due to bioclimatic changes in South Africa [2]. Aloe species in these regions were historically traded due to their medicinal properties [3]. The most popular among the medicinal Aloe species is the Aloe barbadensis Miller (Aloe vera), which was revealed by Grace et al. [2] to originate from the Arabian Peninsula.
The Aloe vera has a triangular shape that consists of tubular yellow flowers and white spotted leaves, as shown in Fig. 1. The white spotted leaves are composed out of three layers: the leaf skin, latex, and gel [4]. The leaf skin is a thick green layer that shields the gel from outer damage [5]. The latex is a yellow bitter layer between the leaf skin and the gel, that is rich in anthraquinones [5,6]. The Aloe vera gel is made up of parenchyma cells that store water (99%) and various phytochemicals [5–9]. The phytochemical components have medicinal properties that can be used for wound healing, digestive issues, inflammation, and diabetes [5], which makes Aloe vera gel a valuable product to the industry. Therefore, the Aloe vera plant is cultivated worldwide for large scale commercial production. Researchers have reported that the phytochemical composition of the Aloe vera gel depends on several cultivation factors such as irrigation regime [10–12], light intensity [12,13] and fertilization [14–16]. Therefore, optimal cultivation conditions are required to maintain the quality of the Aloe vera plants for large-scale commercial production.
Figure 1: Aloe vera anatomy
Nowadays, Aloe vera is commercially cultivated worldwide (Fig. 2), from North America, Europe, Asia Pacific, Latin America, and Africa. However, the demand for Aloe vera gel-based products has led to the surplus production of Aloe vera solid and liquid waste. In Curaçao, the production of Aloe vera gel-based products generates 4000 kg Aloe vera leaf skin waste per month–as stated by Curaloa (The Aloe vera Plantation Curaçao). This Aloe vera leaf skin is currently considered an agricultural waste or used as fertilizer. Agricultural waste is traditionally managed via landfill disposal or incineration, which have negative environmental and societal impacts through the production of greenhouse gases (CH4, NO, NO2), and contamination of the soil and groundwater through leaching [17–20]. Therefore, a sustainable waste management system should be developed to valorize Aloe vera waste into value-added products that would contribute to the circular bioeconomy. The concept of a circular bioeconomy for Aloe vera would be integrating Aloe vera gel processing and waste valorization to reduce waste production and develop new economic sectors that would benefit the society and economy. The objective of this paper is to review multiple approaches that can be integrated into the circular bioeconomy for the valorization of Aloe vera waste.
Figure 2: Worldwide cultivation of Aloe vera
Aloe vera gel is a valuable product, due to its abundancy in phytochemicals, that can be applied in the cosmetic, food and pharmaceutical companies. For example, the food industry uses Aloe vera gel for application as a dietary supplement, antimicrobial agent, and a natural preservative [21]. In the cosmetics industry the gel is used due to its wound healing, anti-aging, and moisturizing effect [22]. The pharmaceutical industries use the Aloe vera gel as treatment for inflammation, ulcer, cancer, and diabetes [6]. Therefore, the Aloe vera industry uses harvesting, washing, filleting and product preparation techniques to preserve the phytochemical content of the Aloe vera gel [23–27], as described in Fig. 3.
Figure 3: Aloe vera gel processing and waste produced (red)
2.1 Harvesting, Washing Step and Draining
Aloe vera leaves are harvested after three years of growth [26]. The Aloe vera leaves are removed from the stem while maintaining the seal on the white base, which protects the gel against bacterial contamination and enzymatic degradation of the bioactive compounds [27,28]. After harvesting, the Aloe vera plant should be left with 15 to 18 leaves in order to sustain a high leaf yield [29]. The harvested Aloe vera leaves are transported to the processing facility, where the Aloe vera leaves are washed in a bactericidal solution to remove bacteria and dirt [24,25,27]. Researchers have reported the use of quaternary ammonium salt or sodium hypochlorite as disinfectants due to their antimicrobial activity [30,31]. Thereafter, the base of the Aloe vera leaves is cut to drain the latex (bitter yellow sap), because it contains aloin and anthraquinones with laxative properties [24,25].
2.2 Aloe vera gel and Whole Leaf Processing
The Aloe vera industry is mainly interested in Aloe vera gel, which accounts for around 60%–66% of the Aloe vera leaf weight [32–34]. Aloe vera gel is extracted from the leaves by hand or through mechanical filleting [24–28]. Hand filleting involves removing the spikes, base, tip, and skin from the Aloe vera leaves with a sharp knife [23]. The mechanical filleting can be done using various types of leaf-splitting units, e.g., units equipped with roller and blades [34–36]. Aloe vera leaves that are processed through the leaf-splitting units are split into halves and then directed to the rollers for the gel extraction [34]. Dinesha et al. [34] reported higher gel recoveries for hand filleting (57.78%) compared to mechanical filleting (43.57%). Researchers reported that for maximum Aloe vera gel extraction from 20–35 mm thick leaves, the roller speed of the leaf-splitting unit should be operated at 75 rpm (revolutions per minute) [35,36]. Gajbhiye et al. [36] reported a gel recovery of 59.7% by mechanically filleting 20 mm Aloe vera leaves at 75 rpm. A recent study done on Aloe vera whole leaf extraction through mechanical filleting by Naik et al. [37] revealed that gel recoveries of 98.13% could be achieved using >25 mm thick leaves by operating the rollers at 100 rpm. The extracted Aloe vera gel is subjected to depulping to remove cellulosic fibers. The extracted gel is added to a depulping extractor with a pore size of 200 μm [23], which only allows particles smaller than 200 μm to pass through.
Another way to process the Aloe vera leaves is through the whole leaf processing. After the washing and draining the latex, the leaves are cut into fragments and transferred to a grinding unit where the leaf fragments are grounded into a liquid suspension [25,27]. The liquid suspension contains a high cellulosic fiber content and is therefore treated with cellulase. The cellulase breaks down the cellulosic fibers and then is passed through a press filtration system that removes solid particles [26,38]. The solid particles can also be removed through centrifugation as described by Chandegara et al. [39], which reported that up to 70.28% of Aloe vera gel was recovered at optimal conditions of 10.000 rpm, 5°C for 30 min.
2.3 Activated Carbon Treatment
The food industry uses Aloe vera gel to formulate their beverages, yoghurt, and dietary supplements and therefore it is important to reduce the content of aloin and anthraquinones. The Aloe vera industry standards are set to less than 10 ppm aloin and anthraquinone for oral consumption–as established by IASC (International Aloe Science Council). Xia et al. [22] reported a 100x reduction in aloin from 8 mg/g to 0.08 mg/g aloin with 1% w/w activated carbon filtration. A later study by Martínez et al. [40] reported that a 465x reduction in aloin content from 46.5 ppm to 0.1 ppm was achieved by treating the Aloe vera gel with a 1.5% activated carbon concentration and stirred for 45 min at 40oC. The downside of activated carbon treatment is that there is a loss of 19%–23% complex polysaccharides [41]. After the treatment, the activated carbon is removed by facilitating the gel through 3 μm and 0.3 μm cellulose press filters [40]. Waller et al. [23] reported that the Aloe vera industry adds 0.05%–2% w/v activated carbons to 2000–20.000 L of gel for 15–60 min. The activated carbon is removed via a press filter with a pore size of 20 μm [23,25]. Alternatively, the aloin and anthraquinone content is reduced by 99.9% by passing the Aloe vera gel through a filter treated with activated carbon [21]. These active carbon treated filters also remove solid residues transferred from the washing, filleting and depulping process [21].
Thereafter, Aloe vera gel is pasteurized to avoid microbial contamination [23,24]. The standard pasteurization conditions for Aloe vera gel are at 65°C for 15 min [23]. However, researchers describe the High-temperature Short-time (HTST) pasteurization at 85°C–95°C for 1–2 min as the best technique to pasteurize the Aloe vera gel [23,24,42]. Saberian et al. [43] later reported that pasteurization at 90°C for 1 min led to a decrease in vitamin C and antioxidant activity within the Aloe vera gel by 16% and 57%, respectively [43].
Preservatives such as citric acid are added to Aloe vera gel to preserve the shelf-life of various Aloe vera based products [26]. In the food industry, vitamin C (ascorbic acid) is used to fortify and to preserve the shelf-life of food, beverages, and diet supplements [25]. These Aloe vera-based food products contain dissolved oxygen that makes the ascorbic acid susceptible to oxidative degradation that can be prevented by removing dissolved oxygen through the application of deaeration [44].
Quality control of Aloe vera gel is important to ensure that the Aloe vera-based products on the market are of the highest quality. The quality control of the Aloe vera gel was done by analyzing organic compounds such as acetylated polysaccharides, glucose, malic acid, lactic acid and acetic acid [45–47]. Jiao et al. [47] suggested that the polysaccharides in the Aloe vera gel vary based on the cultivation conditions, harvesting, processing conditions and storage. The presence of malic acid is an indicator of gel freshness and quality, while lactic acid and acetic acid can be an indication of microbial or enzymatic degradation [45].
Aloe vera-based products are made from the gel or dried concentrates [26]. The main Aloe vera gel-based products are derived from the Aloe vera gel, concentrate and powder [21]. The Aloe vera concentrate and powder require additional drying processes such as freeze-drying, spray-drying tray drying or microwave-assisted drying [23,26]. However, drying of the Aloe vera gel can compromise the bioactive compounds of the Aloe vera gel [48–51]. To maintain the same quality product as the Aloe vera gel, the drying methods used to obtain the concentrate and powder should be further investigated.
3.1 Quantity of Aloe vera Waste
The Aloe vera industry generates a surplus of solid and liquid waste during cultivation and gel extraction process, as described in Table 1. Each Aloe vera plant produces two flowers and 10 suckers per plant per month–according to Curaloa. Regarding Curaloa, the waste generated are based on a plantation containing 50.000 Aloe vera plants. Therefore, during cultivation there are 100.000 flowers and 500.000 suckers being generated as waste per month. The flowers and suckers are removed so that the Aloe vera plant concentrates its energy on growth. The suckers are used for plant propagation or are composted. In addition, 30–50 dead plants are produced per month by Curaloa. The Aloe vera roots are generated post-harvest of Aloe vera plant [52].
3.1.1 Aloe vera gel and Whole Leaf Processing
During gel extraction, each Aloe vera leaf generates 40%–45% gel, 1.0%–1.5% latex and 45%–55% leaf skin [31]. Therefore, for every 1000 kg Aloe vera leaves there are 400–450 kg gel, 10–15 kg latex and 440–535 kg leaf skin produced [31]. In Curaçao, 8000 kg Aloe vera leaves are harvested monthly for gel extraction by Curaloa (The Aloe vera Plantation Curaçao). From the 8000 kg leaves there are 2000 L gel and 4000 kg leaf skin produced monthly. In the depulper, cellulosic fibers are removed, which make up to 20% of the gel produced during extraction–according to Curaloa. In regards to the whole leaf processing, Martinez et al. [31] reported that for every Aloe vera leaf there is 88%–92% liquid suspension, 1.0%–1.5% latex and 8%–12% solid waste particles produced. Therefore, for every 1000 kg Aloe vera leaves there are 880–920 kg liquid suspension, 10–15 kg latex and 70–115 kg solid waste particles produced [31].
3.1.2 Activated Carbon Treatment
Aloe vera industries that utilize activated carbon treatments produce spent activated carbon containing aloin and anthraquinone [31,40]. Currently spent activated carbons are either disposed to the landfill, incinerated or recycled [53]. Waller et al. [23] suggest recovering aloin and anthraquinones absorbed onto the spent activated carbons with organic solvents. Spent activated carbons can be also be recycled through biological, chemical, thermal, steam and microwave treatment [53–55].
In the Aloe vera industry, liquid waste is generated via the washing and drying steps. The washing step generates 3–12 L of wastewater per kg Aloe vera gel produced [56]. Wastewater is also produced throughout the Aloe vera gel drying process to 10x concentrated and powder for. The gel is 10x concentrated by drying the gel until it has lost 90% water weight and then dried further until 87% of its weight is reduced to form the powder [31]. For example, for every 400–450 kg Aloe vera gel extracted there is 40–45 kg 10x concentrated gel (10%) and 360–410 kg wastewater (90%) produced. And for every 40–45 kg 10x concentrated gel dried there is 4.5–6.2 kg powder (13%) and 35–38 kg wastewater (87%) produced. The wastewater accumulated during the gel extraction is discarded to the environment by the Aloe vera industry [56].
Aloe vera waste in the form of leaf skin, flowers, latex and roots are either disposed as agricultural waste or used as a fertilizer. Researchers have found that Aloe vera waste is rich in carbohydrates, amino acids, minerals, vitamins, lipids, and phenolic compounds (Table 2) including the anthraquinones (Fig. 4). Thus, Aloe vera waste could potentially be a valuable feedstock for valorization.
Figure 4: Anthrones, anthraquinones and aloesin present in Aloe vera
After Aloe vera gel extraction, the leaf skin is obtained which accounts for 45%–55% of the Aloe vera leaf weight [4,31,33,34]. Aloe vera leaf skin is also a source of soluble sugars such as glucose, but their concentration is determined by the drying methods used [83]. Ma et al. [83] investigated the impact of heat, air, vacuum freeze and microwave drying on the soluble sugar content of Aloe vera leaf skin. The study showed that microwave drying conditions set at medium output for 3 min followed by 2 min air drying achieved the highest sugar content of 9.35 g/100 g fresh weight. Heat (60°C, 24 h), air (48 h) and vacuum freeze (−50°C, 24 h) drying led to a lower sugar content of 8.27, 8.46 and 8.46 g/100 g fresh weight, respectively. Ma et al. [83] suggested that microwave drying might positively impact the enzyme that is involved in regulating the soluble sugar content. The presence of soluble sugar and cellulose in the Aloe vera leaf skin might make it a potential feedstock for bioethanol production.
The Aloe vera leaf skin was also rich in vitamin C [63], which has been reported to have antioxidant properties. The Aloe vera leaf skin has a higher antioxidant activity compared to the flower (Table 3). Lòpez et al. [81] suggested that the higher antioxidant activity exhibited by the Aloe vera leaf skin might be to its higher phenolic content. The Aloe vera leaf skin is rich in phenolic compounds such as phenolic acids, flavonoids, chromones and anthrones. Researchers have investigated the ethanol and methanol extracts and found that the Aloe vera leaf skin contained a higher phenolic concentration and antioxidant capacity in comparison to the gel and flowers [58,81]. For example, Lòpez et al. [81] analyzed methanol extracts and reported that Aloe vera leaf skin extracts had a higher phenolic content (3.07 mg/g). Similar findings were reported by Añibarro-Ortega et al. [63], who used 80% ethanol-extracts and showed that the leaf skin contained a higher phenolic content (105 mg/g) compared to the flowers (4.78 mg/g). Both these studies correlated the high phenolic concentration with the high antioxidant activity exhibited by Aloe vera leaf skin [63,81]. Previous studies on the antioxidant activity of Aloe vera by Lucini et al. [58] found that the leaf skin also had a higher antioxidant activity than the gel. However, the reported phenolic concentrations for the Aloe vera leaf skin differed between the previously mentioned studies, which might be due to the difference in extraction methods. The methanol extracts of the Aloe vera leaf skin were rich in catechin, sinapic acid and quercetin [81], while the ethanol extracts were rich in aloesin B and malonyl aloin A [63].
Recently the chemical composition of developing Aloe vera flowers was investigated by Martinez-Sanchez et al. [66]. Development of the flowers was categorized in three stages: the immature, mature closed and mature open flower buds. The study showed that the flowers were rich in amino acids, vitamins, fatty acids, and phenolic compounds, but their concentration depended on phase of maturation. For example, the amino acids aspartate, phenylalanine and tyrosine concentration decreased as the flowers matured. On the other hand, the mature flowers contained a higher concentration of alanine and glutamine than the immature flowers. This might be due to metabolic processes that are involved in the development of Aloe vera flowers, resulting in certain amino acids being more prominent during the catalytic or synthesis process [66]. The Aloe vera flowers were also rich in vitamin A (α-and β-carotene), vitamin C (ascorbic acid and dehydroascorbic acid) and vitamin E (α-tocopherol) [62,66]. Martinez-Sanchez et al. [66] observed that the vitamin A and vitamin C were most abundant in the immature flower stage and decreased in concentration as the flower developed. Regarding vitamin E, Lòpez-Cervantes et al. [62] reported that the α-tocopherol concentration of the Aloe vera flower was 47.01 μg/g dry weight, which was higher than the concentration reported for the fresh Aloe vera gel (0.81 μg/g dry weight) by Añibarro-Ortega et al. [63]. Aloe vera flowers are also rich in various polyunsaturated fatty acids that are essential for health such as α-linolenic acid and linoleic acids [66]. Regarding the phenolic compounds, Aloe vera flowers were characterized by phenolic acids and flavonoids [61,63,76,81]. However, the phenolic content of the Aloe vera flowers varied based on the studies, which might be due to the extraction methods used or due to the flower development as described by Martinez-Sanchez et al. [66]. Therefore, depending on the development of the flowers, desirable compounds can be selected without negatively impacting the plant growth and to further valorize the Aloe vera waste.
The Aloe vera gel extraction produces 1.0%–1.5% Aloe vera latex for every Aloe vera leaf drained [66]. The main components of Aloe vera latex sap are anthraquinone glycoside (aloin A and aloin B), chromone glycosides (aloesin and aloeresin A), polyphenols, 8-hydroxyl substituted anthranoids and the aloe-emodin [67,71,74]. Aloin is found in the Aloe vera leaf skin and latex. Sánchez-Machado et al. [85] the aloin content in fresh and dry samples of Aloe vera gel and latex using phosphate buffer saline at pH 3. The fresh (199.76 mg/g) and dry (176.26 mg/g) latex had a higher aloin concentration in comparison to the fresh (7.87 mg/g) and dry (5.11 mg/g) gel. The lower aloin content in the dry samples was likely a result of the drying process at 70°C which caused aloin degradation. In a later study, Lotfizadeh et al. [86] investigated the aloin recovery from dried and liquid Aloe vera latex using ultrasonic and stirring extraction with ethyl acetate. The dried samples were dried using a freeze-drying method for 24 h. The study found that the highest aloin recovery occurred for the dried latex. The study suggests that by freeze-drying the latex, the enzymes involved in aloin degradation activity are inhibited. The dried latex also exhibited a higher antioxidant activity in comparison to the liquid latex, which was correlated with the higher aloin content in the dried samples [86]. As described previously, a higher phenolic content (aloin) is correlated with a higher antioxidant activity. A later study by Nakiguli et al. [87] found that aqueous and PBS extracts from dried and fresh latex had higher total phenolic content and antioxidant activity in comparison to the fresh and dried gel extracts.
Most of the research done on the Aloe vera roots has been on identifying its antibacterial, antifungal antiviral and cytotoxic activities. Haq et al. [88] investigated the antifungal and antimicrobial activity of ethanol extracts from Aloe vera roots. The ethanol extracts of roots showed growth inhibition against gram positive (Staphylococcus aureus, Bacillus Subtitis, Bacillus cereus, Enterococcus faecalis) and gram negative (Escherichia coli, Proteus vulgaris, Acinetobacter baumanni, Psedomonas aeruginosa). However, the ethanol extract did not show growth inhibition against the plant pathogenic fungi Pucciniales [88]. Ethanol extraction likely did not yield the bioactive compounds that are necessary for growth inhibition of the pathogenic fungi. Canche-Escamilla et al. [52] extracted Aloe vera roots with ethyl acetate to obtain its anthraquinones and anthrones to test for antimicrobial activity. Deoxyerythrolaccin and lacaic acid D methyl ester were the only bioactive compounds that exhibited antimicrobial activity against Xanthomonas campestris. The bioactive components contained within the Aloe vera roots also exhibit cytotoxic activities against a breast cancer cell line, which were aloesaponarin I, aloesaponarin-II, and deoxyerythrolaccin [89].
4 Aloe vera Waste Valorization
Researchers have investigated various processes for the valorization of Aloe vera waste through animal feed, biosorbents, biofuels, natural polymers, and extraction of bioactive compounds, as stated in Table 4.
Animal feed from agricultural waste has been considered by various researchers to improve livestock health and reduce the environmental impact [118]. Aloe vera waste is a source of multiple bioactive components that have antioxidant, antimicrobial and anti-inflammatory properties that may improve livestock performance (feed digestibility and volatile fatty acid production) and product quality (milk composition and production). Various researchers have focused on in vitro and in vivo studies to evaluate whole plant extracts of Aloe spp. as potential animal feed for livestock [119–121]. For example, Sirohi et al. [119] reported that acetone-extracts of Aloe vera leaves increased in vitro feed digestibility compared to the methanol and aqueous-extracts. The acetone-extracts likely contained secondary metabolites that improve feed digestibility [119]. Calabrò et al. [120] investigated the effect of Aloe arborescens plant extract on in vitro rumen fermentations and revealed that increasing the plant extract dose to 120 mg/L did not impact feed digestibility but did increase the volatile fatty acid production [120]. Bani et al. [121] also studied the impact of Aloe arborescens homogenate on in vitro rumen fermentations. Similar results were obtained as reported by Calabrò et al. [120], whereby increasing the A. arborescens dose to 500 g caused an increase in total volatile fatty acid concentration. In regards to in vivo studies, researchers have focused on Aloe vera gel and whole plant extracts as animal feed for calves [122,123], goats [124], horses [125], poultry [126–128] and weaned pigs [129]. Aloe vera gel and whole plant extracts have shown a positive impact on growth and against animal diseases such as equine squamous gastric disease [125], swine fever virus [129] and parasitic disease coccidiosis [126,127].
Singh et al. [90] investigated the Aloe vera waste (AVW) generated during the gel processing for its use in in vitro and in vivo studies involving ruminal fermentation, milk production and methane production on lactating cows. The in vitro organic matter and fiber digestibility in lactating cows were increased when using AVW in animal feed. This might be due to the bioactive components present in the AVW, resulting in a diverse microbial community that improves ruminal fermentation [90]. Furthermore, the in vitro methane production decreased from 34.8 to 31.9 mL CH4/g dry matter (DM) with an increasing dose of 10 to 40 g/kg AVW. The in vivo study also revealed that the methane production decreased in lactating cows fed with 20 g/kg AVW (19.9 g/kg DM) in comparison to feeding without AVW (23.9 g/kg DM). The in vivo study also revealed that the milk production and its fats, proteins, and lactose content were increased for lactating cows fed with AVW. Singh et al. [90] suggested that the increase in milk production and its constituents could be due to the increase in nutrient digestibility of the lactating cows fed with AVW. Research into utilization of Aloe vera aqueous extracts as animal feed for lactating goats also found an increase in milk production, fat, protein and lactose [124]. However, Banakar et al. [124] reported that there was a higher fermentation efficiency for the lactating goats fed with Aloe vera aqueous extracts that resulted in higher milk production. The AVW and Aloe vera aqueous extracts likely contain bioactive components that are improving milk production and quality in lactating cows and goats. The lower methane production combined with the increased milk production resulted in a 15% reduction of the carbon footprint of milk production [90]. The use of Aloe vera waste as an animal feed should be considered for a large-scale study due to its potential in reducing the carbon-footprint of milk without compromising on rumen health and product quality.
The aquaculture industry is looking into developing sustainable feed additives that provide health and quality to aquatic animals. Various researchers have focused on using Aloe vera extracts as animal feed for rainbow trout [130], Siberian sturgeon [131], GIFT-tilapia [132], African catfish [133–135], common carp [136] and Nile tilapia [137]. Aloe vera extracts are considered as animal feed due to their numerous health benefits. Various studies have reported on the health properties of Aloe vera waste and should therefore be considered as animal feed for aquaculture. Quirós-Pozo et al. [91] investigated the possibility to use Aloe vera solid waste and gel generated during the press filtration step of gel extraction as potential feed additives for cultivation of the golden mullet. Using 2%–6% AVW 2%–6% or 2% Aloe vera gel as a feed additive did not lead to significant growth for the golden mullet compared to the control. In previous studies on the common carp [138] and GIFT-tilapia [132] it was reported that incorporating 2% Aloe vera was sufficient to increase growth. However, there have been reports that using 1% Aloe vera as feed additive was sufficient to increase growth in African fish [133–135] and nile tilapia [137]. The differences among the studies could be attributed to differences in fish species, feed additive preparation, animal feed ingredients and aquaculture cultivation methods. Quirós-Pozo et al. [91], also found that the golden mullet that were fed with AVW showed a different fatty acid profile. For example, omega-3 fatty acid was present in higher levels in golden mullet fed with AVW in comparison to Aloe vera gel and control. The muscles of golden mullets fed with AVW contained a higher content of arachidonic acid, an essential fatty acid. While the liver of the golden mullets fed with AVW contained higher levels of linoleic acid, a polyunsaturated essential fatty acid (PUFA). The AVW is known to contain various phenolic compounds that have antioxidant properties. The antioxidants remove free radicals and protect the PUFA against lipid oxidation [139,140].
Biosorbents are biological materials that can be used for the removal of pollutants from wastewater. Various researchers have reported the use of Aloe vera agricultural waste to prepare biosorbents for the removal of heavy metals, dyes, and miscellaneous pollutants (Table 5).
The adsorption capacity of biosorbents depends on various factors among which the preparation methods [93]. Malik et al. [141] investigated biosorbents prepared with raw and H3PO4-treated Aloe vera waste (AVW) for their removal potential of lead (II) from aqueous solutions. The batchwise adsorptions were carried out under optimum conditions with contact time of 30 min, absorbent dose of 1.5 g/L, initial absorbate concentration of 0.3 g/L and temperature at 308 K. The results showed that the maximum adsorption of lead (II) was achieved at pH 4 and pH 4.5 for raw and H3PO4-treated AVW, respectively. The H3PO4-treated biosorbent showed a higher adsorption of 96.2 mg/g compared to the adsorption by raw biosorbent of 86.4 mg/g [141]. Another study on biosorbents prepared with raw, H3PO4-and NaOH-treated AVW was investigated by Noli et al. [142] for their capacity to remove uranium (VI) and cadmium (II) from aqueous solutions. The batchwise adsorption for uranium (VI) and cadmium (II) was carried out under optimum conditions with contact time of 6 h, absorbent dose of 1.5 g/L, initial absorbate concentration of 1 g/L. The highest maximum adsorption of uranium (VI) and cadmium (II) was reached at pH 4 and 5–6, respectively. The study revealed that the NaOH-treated biosorbent had a higher adsorption capacity compared with the biosorbents based on raw and acid-treated biosorbents. Noli et al. [142] suggest that the higher absorption of NaOH-treated biosorbents is due to the higher porosity that exposes the functional groups and aids metal intake. The biosorbents reported by Noli et al. [142] have shown higher removal capacities compared to activated carbons prepared with alternative agricultural waste such as green algae, grape stalks and citrus lemon peel [142].
The adsorption capacity of a biosorbent depends on processing factors such as pH, contact time, absorbent dosage and pollutant initial concentration [92,93]. For example, the charge on the adsorption sites of the biosorbents changes depending on the pH. Arivoli et al. [143] studied activated carbon prepared from Aloe vera leaf skin for removal of toxic dyes such as congo red, malachite green, rhodamine B and rose Bengal. The research revealed that the pH could be used to select for the removal of positively (malachite green and rose Bengal) or negatively (congo red and rhodamine B) charged dye pollutants [143]. The pHpzc (point zero charge) determines the interaction between adsorption sites of the biosorbents and pollutant. If the pH > pHpzc, that there are more negatively charged adsorption sites available on the biosorbents and thus there is more interaction with positively charged pollutant. For example, an activated carbon based on Aloe vera leaf skin based biosorbents was able to remove up to 22.98 mg/g methylene blue at pH 12. Khaniabadi et al. [144] suggested that the activated carbon Aloe vera leaf skin had more negatively charged adsorption sites at pH 12 (pHpzc = 11.3) thereby resulting in higher removal of the positively charged methylene blue. Khaniabadi et al. [145] also investigated the adsorption of aniline and methyl orange by sulfuric acid treated activated carbon derived from Aloe vera leaf skin. The study found that biosorbents were able to remove a maximum of aniline and methyl orange of 14.47 and 46.31 mg/g at pH 3. Since the biosorbents have a pHpzc of 5.8, it is likely that a pH < pHpzc resulted in more interaction between the positively charged adsorption sites and the negatively charged aniline and methyl orange [145].
Various studies have focused on the use of Aloe vera leaf for the lab-scale batch adsorption. Gupta et al. [146] investigated the use of Aloe vera leaf powder as a biosorbent for the removal of nickel (II) from aqueous solutions batch mode. This biosorbent achieved a nickel (II) adsorption of 42.2% under optimal conditions of pH 7, contact time of 180 min, absorbent dose of 1 g, initial absorbate concentration of 100 mg/L and temperature at 303 K [146]. Another study by Gupta et al. [147] focused on the use of Na2CO3-treated Aloe vera leaf powder as a biosorbent for the removal of nickel (II) from aqueous solutions in batch mode. The Na2CO3-modified biosorbent achieved maximum adsorption of 28.98 mg/g nickel (II) under the optimum conditions of pH 7, contact time of 90 min, absorbent dose of 0.6 g, initial absorbate concentration of 20–200 mg/L and temperature at 303 K [147]. The switch from lab-scale batch to large-scale continuous processes should also be considered for the treatment of large volumes of wastewater. Therefore, Gupta et al. [148] investigated Na2CO3-treated Aloe vera leaf powder as a biosorbent for the removal of nickel (II) from aqueous solutions in a continuous fixed bed column. Thus, the biosorbent was able to achieve a maximum adsorption of 16.28 mg/g at operating conditions of bed depth 6 cm, flow rate of 10 ml/min, initial absorbate concentration 20 mg/L and temperature at 303 K [148]. This study has shown that Na2CO3-treated Aloe vera leaf powder can be applied for the removal of nickel (II) through a continuous process.
Bioethanol is a renewable bio-based resource that can be produced through first and second generational production. The first-generation bioethanol is produced from food-based feedstock such as corn and sugar cane [149]. However, food-based resources for bioethanol production causes a competition between food, feed, and fuel industry for land use [149,150]. The competition between food, feed and fuel can be prevented through the second-generation bioethanol production which uses non-food feedstock from agricultural waste such as rice straw, wheat straw and corn straw [151,152]. The process to produce bioethanol through lignocellulosic feedstock consists out of 1) delignification pretreatment, 2) enzymatic saccharification, 4) fermentation and 5) distillation and evaporation [152].
Lignocellulosic biomass needs to be pretreated before the bioethanol production because of its recalcitrant lignin structure. Lignocellulosic biomass consists out of the interwoven biopolymers, cellulose, hemicellulose, and lignin. Aloe vera leaf skin is a lignocellulosic biomass that is composed out of 57.72% α-cellulose, 16.39% hemicellulose and 13.73% lignin [57]. Lignin must be solubilized and separated to make the cellulose and hemicellulose accessible for bioethanol production. Researchers have investigated Aloe vera leaf skin for bioethanol production by looking into various pretreatment techniques such as acid-hydrolysis [95], laccase mediated delignification [96,98], mild acid microwave treatment [97]. Sathya et al. [95] investigated various lignocellulosic feedstocks pretreated via acid-hydrolysis for bioethanol production. The study found that 5 g of Aloe vera leaf skin pretreated with 2.5% sulfuric acid for two days resulted in the highest sugar concentration of 1.92 mg/L among the analyzed lignocellulosic feedstocks. The sugar fermentation by the yeast Saccharomyces cerevisiae MTCC 4779 took 144 h at 25°C to produce 9.60 mg/L bioethanol. Rajeswari et al. [97] investigated microwave acid pretreatment for delignification of Aloe vera leaf skin followed by enzymatic saccharification for bioethanol production. A maximum delignification of 66.00% was achieved upon treatment with 0.5% sulfuric acid at microwave irradiation of 480W. Enzymatic saccharification of the delignified Aloe vera leaf skin (63.60%) was higher in comparison to the untreated Aloe vera leaf skin (27.70%). The microwave acid pretreatment followed by enzymatic saccharification yielded a sugar concentration of 298.90 mg/g. On the other hand, untreated Aloe vera leaf skin yielded a lower sugar concentration of 129.68 mg/g sugars. A later study by Rajeswari et al. [96] found that laccase-mediated pretreatment of Aloe vera leaf skin led to a maximum delignification of 76.67% at optimum conditions of 1:3.7 (Aloe vera leaf skin-laccase ratio), 50°C and 6 h incubation. The study also found that laccase-mediated delignified Aloe vera leaf skin (44.34%) showed higher enzymatic saccharification in comparison to raw Aloe vera leaf skin (24.26%). The subsequent hydrolysis of the laccase-mediated delignified Aloe vera leaf resulted in a sugar concentration of 207 mg/g. Laccase-mediated delignification of Aloe vera leaf skin is described as a greener option in comparison to the acid-hydrolysis pretreatment [96]. Rajeswari et al. [98] also investigated the potential optimization of enzymatic saccharification of laccase-mediated delignified Aloe vera leaf skin followed by fermentation for bioethanol production. The enzymatic saccharification was optimized by using cellulase produced from Aspergillus sp. under optimal conditions of 1:17 (Aloe vera leaf skin-laccase ratio), 53°C and 8.5 h incubation. At optimal conditions the enzymatic saccharification reached 63.00% and yielded 306 mg/g sugars. Three methods were investigated for the fermentation using Saccharomyces cerevisiae: free cell suspension, immobilized yeast suspension and immobilized yeast in a packed bed reactor. The immobilized cells in the packed bed reactor showed the highest ethanol yield and productivity of 16.50 g/L and 2.75 g L−1 h−1, respectively. The immobilized cells in the packed bed reactor also showed the highest bioethanol production per sugar consumed. Additional process advantages of doing a fermentation with immobilized cells include ease of product separation, low risk of contamination, low cost of separation and reusability [98].
The MixAlcoTM process is used to convert lignocellulosic biomass into mixed alcohols fuels and can be summarized in 1) pretreatment, 2) fermentation, 3) concentration, 4) thermal conversion, 5) hydrogenation and 6) oligomerization [153–155]. The pretreatment process depends on the lignin content of the lignocellulosic biomass [154]. Granda et al. [154] reported that biomass containing less than 10% lignin does not need to be pretreated. The fermentation step requires the use of a mixed culture of microorganisms to convert lignocellulosic biomass into carboxylic acids via primary fermentation. Forrest et al. [99] investigated Aloe vera leaf skin as a potential substrate for MixAlcoTM process by focusing on the anaerobic batch fermentation using a mixed culture of marine organisms. Aloe vera leaf skin (80 wt%) was used to produce acetic acid with chicken manure (20 wt%) as a co-substrate. The Aloe vera leaf skin produced 25.5 g/L total carboxylic acid, which consists out of acetic acid (19.6 g/L), butyric acid (4.3 g/L), propionic acid (1.4 g/L) and valeric acid (0.3 g/L). Among all the potential substrates tested by Forrest et al. [99], Aloe vera leaf skin was among the highest total acid producers with high conversion (59%) and selectivity (0.64 g total acids/g volatile solids (VS) digested). The carboxylic acids produced can be thermochemically converted to ketones via ketonization and hydrogenated to mixed alcohol fuels [155]. Further research on the feasibility of producing mixed alcohol fuels via the MixAlcoTM process from Aloe vera skin should be considered.
Biogas and digestate can be produced through anaerobic digestion of lignocellulosic biomass such as Aloe vera leaf skin using fermentative microorganisms. Biogas is used to generate heat, electricity, and natural gas, while the digestate is used as fertilizer. The anaerobic digestion of organic material consists out of 1) sugars converted to monosaccharides through hydrolysis, 2) monosaccharides converted to volatile fatty acids via acidogenesis, 3) volatile fatty acids converted to acetic acid, CO2, and H2 through acetogenesis and 4) acetates converted to methane via methanogenesis [156].
Huang et al. [100] investigated the anaerobic digestion of Aloe vera leaf skin, dairy manure, and Aloe vera skin codigested with dairy manure for biogas production. The anaerobic digestion of Aloe vera leaf skin generated biogas yield of 235.6 mL/g VS, while the dairy manure generated a biogas yield of 265.6 mL/g VS. The study also revealed that anaerobic co-digestion of 3:1 (mass ratio) Aloe vera leaf skin with dairy manure (303.0 mL/g VS) generated a higher biogas yield in comparison to digestion solely with Aloe vera leaf skin or dairy manure. The low biogas yield of Aloe vera leaf skin was associated to its high carbon-nitrogen ratio (C/N 34.52), high lignin content (14.1%) and low buffering capacity. The co-digestion with dairy manure reduced the C/N ratio to 23.3 and enhanced the buffering capacity that likely led to the improvement of the biogas yield [100]. Incorporating 0.3 wt% vermiculite to the anaerobic digestion described by Huang et al. [100], led to a higher biogas yield (354.0 mL/g VS) [104]. Researchers have further improved the biogas yield of anaerobic digestion of Aloe vera leaf skin with dairy manure by incorporating various strategies such as microbial electrolysis cells, magnetic fields, and accelerants such as bio-based carbons, fly ash and titanium-based nanoparticles (Table 6). Aloe vera leaf skin has also been used as a bio-based carbon accelerant for the improvement of anaerobic digestion of acorn slag waste with diary manure [101]. Wang et al. [101] revealed that addition of the Aloe vera leaf skin bio-based carbon as an accelerant improved the biogas yield (580.9 mL/g VS) compared to the control (409.2 mL/g VS). In another study, a cobalt and Aloe vera leaf skin bio-based carbon composite was developed to investigate its impact on the anaerobic digestion of sewage sludge with dairy manure [102]. The study by Chen et al. [102] revealed that the Aloe derived carbon-based composites increased the biogas yield (577.3–585 mL/g VS) in comparison to the control (435.8 mL/g VS).
Thermochemical processes use heat to convert lignocellulosic biomass into fuels and chemicals via combustion, pyrolysis, gasification, and liquefaction. Gasification is the conversion of lignocellulosic biomass into fuels through partial oxidation by a gasification agent at high temperature (> 700°C). The gasification agent consists out of air, oxygen, steam, or supercritical water. Supercritical water is water that has reached the critical temperature of >373°C and critical pressure >22.1 MPa. Aloe vera leaf skin was among several food wastes that was investigated by Nanda et al. [108] through catalytic and non-catalytic supercritical water gasification (SCWG) for hydrogen rich syngas production. The highest H2 yield, and selectivity were achieved under the optimal conditions of 1:5 biomass to water ratio, 600°C for 45 min at 23–25 MPa. The non-catalytic SCWG of the Aloe vera leaf skin resulted in a high H2 yield (1.68 mmol/g,) but the lowest H2 selectivity (29.6%) among the food wastes under optimum conditions. For the catalytic SCWG, incorporating an alkali-based homogenous catalyst (2 wt% K2CO3) improved the H2 yield (3.59 mmol/g) and selectivity (36%) of Aloe vera the leaf skin. The low H2 selectivity exhibited by the leaf skin was due to the higher yield of CO2 and CH4. Aloe vera leaf skin was among the best producers of total gas (CO, CO2 and CH4) for the non-catalytic and catalytic SCGW. Among the other food wastes, coconut peel performed better regarding the H2 yield (3.59 mmol/g) and selectivity (36%) for the non-catalytic and the catalytic SCWG.
Researchers have mostly investigated Aloe vera gel and whole plant extracts to synthesize natural polymers-derived biomaterials for medicinal purposes such as wound dressing [157], drug delivery [46,158] and tissue engineering [159,160]. However, Cheng et al. [57] investigated whether cellulosic nanofibers could be isolated from Aloe vera leaf skin for future application into polymer scaffolds. The study revealed that the Aloe vera leaf skin cellulosic nanofibers (AVRNF) were weaker than nanofibers from wood, rice straw and potato tuber. In addition, the AVRNF was weaker in comparison to the nanofibers isolated from wood. The authors argue that the weakness of the AVRNF might be due to the isolation treatment or the characteristics of the leaf skin. Therefore, Cheng et al. [109] investigated the impact of three treatments to determine the reason for the weakness and brittleness of the AVRNF. The first treatment involved reducing the bleaching time from 4 h to 1.5 h at 70°C–80°C. The second treatment involved using 2% acidified sodium chlorite solution for bleaching instead of 4% sodium hydroxide. Both chemical treatments did not show any significant improvements in the mechanical performance, which means that there was no improvement in the tensile strength or young’s modulus. During the third treatment, the characteristic of the Aloe vera leaf skin was analyzed. The leaf skin contains a layer called the cuticle, which was removed by boiling the leaf skin for 2–3 h. The AVRNF without cuticle showed a significantly higher tensile strength (170 MPa) and young’s modulus (11 GPa) in comparison with the AVRNF with the cuticle (110 MPa and 10 GPa). Analysis of the cuticles revealed that its tensile strength (28.08 MPa) and young’s modulus (3.79 GPa) was very low and likely contributed to the weakness of the AVRNF. In a later study, Kakroodi et al. [110] investigated the mechanical performance of polyvinyl alcohol (PVA) reinforced with AVRNF without cuticle biocomposite using solvent casting. The control showed a tensile strength and young’s modulus of 71 MPa and 4.3 GPA, respectively. The study revealed that 2% AVRNF without cuticle was sufficient to increase the tensile strength and young’s modulus of the biocomposite to 116 MPa and 5.6 GPa. Reinforcing the PVA with 10% AVRNF without cuticle further increased the tensile strength and young’s modulus to 161 MPa and 8.0 GPa.
In later studies, researchers investigated polylactic acid (PLA) reinforced with Aloe vera rind fibers (AVRF) biocomposite using the extrusion-injection molding process [111,112]. The AVRF was extracted through a retting process from Aloe vera leaf skin waste produced by a textile company in India. Chaitanya et al. [111] reported that the mechanical performance of the PLA improved when reinforced with 30% AVRF. In addition, further improvements in the mechanical performance could be achieved by treating the AVRF with 5% NaOH. The 5 h NaOH treatment improved the tensile strength and young’s modulus of the reinforced PLA to 54.7 MPa and 7.1 GPa, respectively. The NaOH treatment removes non-cellulosic materials from the fiber surface to achieve better interfacial adhesion between fibers and PLA matrix [111]. In a later study, Chaitanya et al. [112] investigated NaHCO3 for AVRF treatment, due to the environmental hazard associated with NaOH. The AVRF was treated with 10% NaHCO3 for 24–168 h to determine optimum time for improved mechanical performance. PLA reinforced with 30% AVRF treated with 10% NaHCO3 for 72 h achieved the highest tensile strength and young’s modulus of 52.4 MPa and 7.3 GPa, respectively. Treatment for 72 h likely led to maximum removal of non-cellulosic material and improved the interfacial adhesion between fiber and matrix. However, the mechanical performance of the PLA reinforced with AVRF was lower than the composites previously described Kakroodi et al. [110]. There are various potential explanations for the lower mechanical performance, which are the difference in isolation treatment (chemical vs. retting), biocomposite preparation (solvent casting vs. extrusion-injection molding) and leaf skin characteristics (with vs. without cuticle).
Lignin is a natural polymer that can be extracted from plant material and used for medicinal applications. Therefore, Jeyaraj et al. [115] explored the use of lignin isolated from Aloe vera for application as a cancer drug delivery system. Lignin was isolated from Aloe vera through a microwave extracted and then atom transfer radical polymerization was used to synthesize the lignin grafted methacrylate (LIG-g-MA) polymer. The LIG-g-MA polymer was analyzed for its drug delivery potential of 5-fluorouracil (5-FU), which is an anti-cancer agent. The in vitro study revealed that the LIG-g-MA polymer loaded with 5-FU exhibited cytotoxicity and growth inhibition of the breast cancer cell line, MCF-7 cells. The drug loaded polymer showed higher cytotoxicity against the MCF-cells in comparison free 5-FU, because the polymer delivers the drug directly to the target cancer cells. Future research could use Aloe vera waste as source of lignin for application as drug delivery systems.
4.8 Bioactive Compounds (Future Considerations)
Aloe vera waste contains various bioactive compounds, such as anthraquinones, chromones, flavonoids and phenolic acids. Aloe vera waste is a potential valuable feedstock for the extraction of these bioactive components. Aloe vera waste can be promoted to various industries due to the nature of these bioactive compounds as opposed to artificial ingredients. For example, anthraquinones that can be applied as antioxidant, antimicrobial, antifungal, antiviral and anticancer agent [161,162]. Anthraquinones have various applications estimated to be worth $2.2 billion by 2025 on the global market [163].
Aloe-emodin (Fig. 4) is an anthraquinone derivative that has various pharmaceutical and food applications. Various researchers have reported that aloe-emodin has the ability to inhibit proliferation and induce apoptosis in various cancer cell lines, e.g., lung squamous, breast, hepatoma and colon [164,165]. Aloe-emodin is also a valuable compound due to its inhibitory capacity on advanced glycation end-products (AGEs) such as fructosamine, glyoxal, methylglyoxal [161]. These AGEs are produced during the food heating process and are associated with diabetes and age-related diseases such as Alzheimer’s disease [166,167]. Researchers have investigated various extraction methods to isolate aloe-emodin from plants via ultrasound-assisted, microwave-assisted and soxhlet extraction has been reported [116,168,169]. Wang et al. [116] reported that a higher aloe-emodin yield could be extracted from Aloe vera leaf skin through microwave-assisted extraction optimized with 80% ethanol (V/V) at microwave irradiation of 340 W at 3 min. The microwave-assisted extraction led to faster extractions, less solvent consumption and higher extraction yields as opposed to ultrasound-assisted and soxhlet extraction.
Aloin is an anthrone glycoside that is a mixture of the two diastereoisomers (see Fig. 1), aloin A and aloin B [170]. A previous study has shown that aloin could be used as a potential safer non-steroidal anti-inflammatory (NSAID) drug for the treatment of inflammation [171]. Aloin provides protection to liver injury in mice induced by alcohol through inhibition of lipid accumulation, oxidative stress, and inflammation [172,173]. Aloin has also shown to be a promising drug for the treatment of heart hypertrophy and fibrosis induced by continuous administration of the β-adrenergic agonist isoproterenol [162]. Furthermore, researchers have reported the use of aloin as an effective antiviral agent against severe acute respiratory syndrome coronavirus 2 (SARS-CoV-2) infection, which is a strain of coronavirus that caused the worldwide Coronavirus Disease 2019 (COVID-19) outbreak [174]. Besides aloin, researchers have reported that the rutin and β-sitosterol, found in the Aloe vera leaf skin and flower, can be applied in combating the SARS-CoV-2 infection [175,176]. For example, Abouelela et al. [175] screened 237 natural products of Aloe genus for their antiviral capacity against SARS-CoV-2 infection. The study showed that rutin has the capacity to inhibit the main protease and spike glycoprotein involved in the SARS-CoV-2 infection [175]. β-Sitosterol is another compound found in the Aloe vera leaf skin that can bind to the spike protein of the SARS-CoV-2 and restrict viral invasion [176].
Aloesin (Fig. 4) is an 8-C-glycoside that is found in the Aloe vera leaf skin. Extraction of aloesin from Aloe vera leaf skin has already been investigated by Añibarro-Ortega et al. [63], which reported that the highest extraction yield of 63 mg/L aloesin was achieved using a solvent-water system containing 51.5% propylene glycol. Researchers have observed that aloesin has inhibitory activity towards tyrosinase and beta-secretase [177–179]. Tyrosinase is an enzyme responsible for the conversion of tyrosine into melanin. Researchers have reported that aloesin is able to inhibit the tyrosinase in order to reduce hyperpigmentation caused by ultraviolet radiation [177,178]. Aloesin has also shown promise into inhibiting the enzymatic activity of beta-secretase [179], which is an enzyme involved in the neurodegenerative process that leads to Alzheimer’s disease [180,181].
Aloe vera leaf waste is a potential feedstock to produce high value products for the application into food, animal feed, biosorbents, biofuel and natural polymers. Researchers have found that Aloe vera waste are rich in vitamins and bioactive components (anthraquinone, phenolic acids, flavonoids, chromones) that have antioxidant properties. The Aloe vera waste are considered high value due to the potential for extraction of bioactive compounds. Researchers have also found Aloe vera waste can be valorized through environmental application as animal feed and biosorbents. Application of the leaf skin to animal feed decreased the carbon footprint of milk in lactating cows without causing adverse effects on the rumen health. Aloe vera leaf skin and active carbon derived thereof have also shown its application as a biosorbent to remove heavy metals, dyes, and oil-based pollutants from wastewater. However, the preparation method and processing parameters are important for optimal adsorption. Valorization of Aloe vera leaf skin to bioenergy was also highlighted by several researchers involving bioethanol, biogas, and syngas production. Several attempts have been made to optimize the conversion of Aloe vera leaf skin without compromising the yield of biogas and bioethanol. The MixAlcoTM process was found to be a promising technique to produce biofuels, because of its high conversion and selectivity. Aloe vera waste can also be used to isolate and synthesize natural polymers that can be applied for medicinal purposes such as drug delivery system for anticancer agents. There are various valorization approaches that could be considered and integrated in an Aloe vera circular economy that would provide benefits for society and the economy.
Acknowledgement: The authors would like to thank the SISSTEM team at the University of Aruba, KU Leuven and the European Union (FED/2019/406–549).
Funding Statement: This review was funded by the European Union (FED/2019/406–549).
Conflicts of Interest: The authors declare that they have no conflicts of interest to report regarding the present study.
References
1. WFO (2022). Aloe. http://www.worldfloraonline.org/taxon/wfo-4000001341. [Google Scholar]
2. Grace, O. M., Buerki, S., Symonds, M. R. E., Forest, F., van Wyk, A. E. et al. (2015). Evolutionary history and leaf succulence as explanations for medicinal use in aloes and the global popularity of Aloe vera. BMC Evolutionary Biology, 15(1), 1–12. DOI 10.1186/s12862-015-0291-7. [Google Scholar] [CrossRef]
3. Grace, O. M. (2011). Current perspectives on the economic botany of the genus Aloe L. (Xanthorrhoeaceae). South African Journal of Botany, 77(4), 980–987. DOI 10.1016/j.sajb.2011.07.002. [Google Scholar] [CrossRef]
4. Femenia, A., Sánchez, E. S., Simal, S., Rosselló, C. (1999). Compositional features of polysaccharides from Aloe vera (Aloe barbadensis Miller.) plant tissues. Carbohydrate Polymers, 39(2), 109–117. DOI 10.1016/S0144-8617(98)00163-5. [Google Scholar] [CrossRef]
5. Maan, A. A., Nazir, A., Khan, M. K. I., Ahmad, T., Zia, R. et al. (2018). The therapeutic properties and applications of Aloe vera: A review. Journal of Herbal Medicine, 12, 1–10. DOI 10.1016/j.hermed.2018.01.002. [Google Scholar] [CrossRef]
6. Hamman, J. H. (2008). Composition and applications of Aloe vera leaf gel. Molecules, 13(8), 1599–1616. DOI 10.3390/molecules13081599. [Google Scholar] [CrossRef]
7. Sierra-García, G. D., Castro-Ríos, R., González-Horta, A., Lara-Arias, J., Chávez-Montes, A. (2014). Acemannan, an extracted polysaccharide from Aloe vera: A literature review. Natural Product Communications, 9(8), 1217–1221. DOI 10.1177/1934578x1400900836. [Google Scholar] [CrossRef]
8. Puia, A., Puia, C., Moiş, E., Graur, F., Fetti, A. et al. (2021). The phytochemical constituents and therapeutic uses of genus Aloe: A review. Notulae Botanicae Horti Agrobotanici Cluj-Napoca, 49(2), 12332. DOI 10.15835/nbha49212332. [Google Scholar] [CrossRef]
9. Nalimu, F., Oloro, J., Kahwa, I., Ogwang, P. E. (2021). Review on the phytochemistry and toxicological profiles of Aloe vera and Aloe ferox. Future Journal of Pharmaceutical Sciences, 7(1), 1–21. DOI 10.1186/s43094-021-00296-2. [Google Scholar] [CrossRef]
10. Delatorre-Herrera, J., Delfino, I., Salinas, C., Silva, H., Cardemil, L. (2010). Irrigation restriction effects on water use efficiency and osmotic adjustment in Aloe vera plants (Aloe barbadensis Miller.). Agricultural Water Management, 97(10), 1564–1570. DOI 10.1016/j.agwat.2010.05.008. [Google Scholar] [CrossRef]
11. Hazrati, S., Tahmasebi-Sarvestani, Z., Mokhtassi-Bidgoli, A., Modarres-Sanavy, S. A. M., Mohammadi, H. et al. (2017). Effects of zeolite and water stress on growth, yield and chemical compositions of Aloe vera L. Agricultural Water Management, 181, 66–72. DOI 10.1016/j.agwat.2016.11.026. [Google Scholar] [CrossRef]
12. Hazrati, S., Tahmasebi-Sarvestani, Z., Nicola, S., Kashkooli, A. B., Habibzadeh, F. et al. (2020). Effect of light and water deficiency on growth and concentration of various primary and secondary metabolites of Aloe vera L. Journal of Agricultural Science and Technology, 22(5), 1343–1358. [Google Scholar]
13. Lucini, L., Pellizzoni, M., Molinari, G. P. (2013). Anthraquinones and β-polysaccharides content and distribution in Aloe plants grown under different light intensities. Biochemical Systematics and Ecology, 51, 264–268. DOI 10.1016/j.bse.2013.09.007. [Google Scholar] [CrossRef]
14. Khajeeyan, R., Salehi, A., Dehnavi, M. M., Farajee, H., Kohanmoo, M. A. (2019). Physiological and yield responses of Aloe vera plant to biofertilizers under different irrigation regimes. Agricultural Water Management, 225, 105768. DOI 10.1016/j.agwat.2019.105768. [Google Scholar] [CrossRef]
15. Khajeeyan, R., Salehi, A., Movahhedi Dehnavi, M., Farajee, H., Kohanmoo, M. A. (2021). Growth parameters, water productivity and aloin content of Aloe vera affected by mycorrhiza and PGPR application under different irrigation regimes. South African Journal of Botany, 147, 1188–1198. DOI 10.1016/j.sajb.2021.02.026. [Google Scholar] [CrossRef]
16. Prisa, D., Gobbino, M. (2021). Microbic and algae biofertilizers in Aloe Barbadensis Miller. Open Access Research Journal of Biology and Pharmacy, 1(2), 1–9. DOI 10.53022/oarjbp.2021.1.2.0019. [Google Scholar] [CrossRef]
17. Wielgosiński, G., Targaszewska, A. (2014). The impact of waste incineration on human beings and the environment. Ecological Chemistry and Engineering S, 21(2), 353–363. DOI 10.2478/eces-2014-0027. [Google Scholar] [CrossRef]
18. Vaverková, M. D. (2019). Landfill impacts on the environment—Review. Geosciences, 9(10), 431. DOI 10.3390/geosciences9100431. [Google Scholar] [CrossRef]
19. de Titto, E., Savino, A. (2019). Environmental and health risks related to waste incineration. Waste Management and Research, 37(10), 976–986. DOI 10.1177/0734242X19859700. [Google Scholar] [CrossRef]
20. Tait, P. W., Brew, J., Che, A., Costanzo, A., Danyluk, A. et al. (2020). The health impacts of waste incineration: A systematic review. Australian and New Zealand Journal of Public Health, 44(1), 1753–6405. DOI 10.1111/1753-6405.12939. [Google Scholar] [CrossRef]
21. Ahlawat, K. S., Khatkar, B. S. (2011). Processing, food applications and safety of Aloe vera products: A review. Journal of Food Science and Technology, 48(5), 525–533. DOI 10.1007/s13197-011-0229-z. [Google Scholar] [CrossRef]
22. Xia, Q., Yin, J. J., Fu, P. P., Boudreau, M. D. (2007). Photo-irradiation of Aloe vera by UVA-formation of free radicals, singlet oxygen, superoxide, and induction of lipid peroxidation. Toxicology Letters, 168(2), 165–175. DOI 10.1016/j.toxlet.2006.11.015. [Google Scholar] [CrossRef]
23. Waller, T. A., Pelley, R. P., Strickland, F. M. (2004). Industrial processing and quality control of Aloe barbadensis (Aloe vera) gel. In: Aloes: The genus Aloe, 38, 157–224. [Google Scholar]
24. He, Q., Liu, C. H., Kojo, E., Tian, Z. (2005). Quality and safety assurance in the processing of Aloe vera gel juice. Food Control, 16(2), 95–104. DOI 10.1016/j.foodcont.2003.12.001. [Google Scholar] [CrossRef]
25. Ramachandra, C. T., Rao, P. S. (2008). Processing of Aloe vera leaf gel: A review. American Journal of Agricultural and Biological Sciences, 3(2), 502–510. DOI 10.3844/ajabssp.2008.502.510. [Google Scholar] [CrossRef]
26. Upton, R. (2012). Aloe vera leaf, Aloe vera leaf juice, Aloe vera inner leaf juice, Aloe vera (L.) burm. F: Standards of Identity, Analysis & Quality Control. American Herbal Pharmacopoeia. [Google Scholar]
27. Chandegara, V., Kumar Varshney, A., Chandegara, V. K., Varshney, A. K. (2013). Aloe vera L. processing and products : A review Aloe vera L. processing and products: A review. International Journal of Medicinal and Aromatic Plants, 3(4), 492–506. [Google Scholar]
28. Manvitha, K., Bidya, B., Karkala Manvitha, C. (2014). Aloe vera: A wonder plant its history, cultivation and medicinal uses. Journal of Pharmacognosy and Phytochemistry, 2(5), 85–88. [Google Scholar]
29. Wang, Y. T. (2007). Severity of leaf harvest, supplemental nutrients, and sulfur application on long-term leaf production of Aloe barbadensis miller. HortScience, 42(7), 1584–1588. DOI 10.21273/HORTSCI.42.7.1584. [Google Scholar] [CrossRef]
30. Cristiano, G., Murillo-Amador, B., de Lucia, B. (2016). Propagation techniques and agronomic requirements for the cultivation of Barbados aloe (Aloe vera (L.) Burm. F.)—A review. Frontiers in Plant Science, 7, 1410. DOI 10.3389/fpls.2016.01410. [Google Scholar] [CrossRef]
31. Martínez-Burgos, W. J., Serra, J. L., MarsigliaF, R. M., Montoya, P., Sarmiento-Vásquez, Z. et al. (2022). Aloe vera: From ancient knowledge to the patent and innovation landscape–A review. South African Journal of Botany, 147, 993–1006. DOI 10.1016/j.sajb.2022.02.034. [Google Scholar] [CrossRef]
32. Zapata, P. J., Navarro, D., Guillén, F., Castillo, S., Martínez-Romero, D. et al. (2013). Characterisation of gels from different Aloe spp. as antifungal treatment: Potential crops for industrial applications. Industrial Crops and Products, 42(1), 223–230. DOI 10.1016/j.indcrop.2012.06.002. [Google Scholar] [CrossRef]
33. Flores-López, M. L., Romaní, A., Cerqueira, M. A., Rodríguez-García, R., Jasso de Rodríguez, D. et al. (2016). Compositional features and bioactive properties of whole fraction from Aloe vera processing. Industrial Crops and Products, 91, 179–185. DOI 10.1016/j.indcrop.2016.07.011. [Google Scholar] [CrossRef]
34. Dinesha, D. T., Ramachandra, C. T., Uday, K. N., Sharanagouda, H., Prakash, K. (2019). Performance evaluation of roller type Aloe vera gel extraction machine. Journal of Pharmacognosy and Phytochemistry, 8(4), 1987–1992. [Google Scholar]
35. Chandegara, V. K., Varshney, A. K. (2014). Design and development of leaf splitting unit for Aloe vera gel expulsion machine. Journal of Food Process Engineering, 37(4), 427–437. DOI 10.1111/jfpe.12098. [Google Scholar] [CrossRef]
36. Gajbhiye, A., Gupta, S. K., Alam, S., Sharma, S. R., Mittal, T. C. (2017). Design, development, and evaluation of Aloe vera leaf gel expulsion machine. Journal of Food Process Engineering, 40(5), e12543. DOI 10.1111/jfpe.12543. [Google Scholar] [CrossRef]
37. Naik, R., Annamalai, S. J. K. (2020). Development of continuous feed equipment for Aloe vera whole gel extraction. Journal of Scientific and Industrial Research, 79(11), 971–979. [Google Scholar]
38. Coats, B. C. (1979). Hypoallergenic stabilized Aloe vera gel. US05/883749. [Google Scholar]
39. Chandegara, V. K., Varshney, A. K. (2013). Effect of centrifuge speed on Gel extraction from Aloe vera leaves. Journal of Food Processing & Technology, 5(1), 1–6. DOI 10.4172/2157-7110.1000295. [Google Scholar] [CrossRef]
40. Martinez Burgos, W. J. (2020). Optimización del proceso de eliminación de aloína del gel fileteado mecánico de Aloe vera concentrado 10X. Cumbres, 3(2), 181–192. DOI 10.48190/cumbres.v3n2a16. [Google Scholar] [CrossRef]
41. Boudreau, M. D., Beland, F. A., Nichols, J. A., Pogribna, M. (2013). Toxicology and carcinogenesis studies of a nondecolorized whole leaf extract of Aloe barbadensis miller (Aloe vera) in F344/N rats and B6C3F1 mice (drinking water study). National Toxicology Program Technical Report Series, 1(577), 1–266. [Google Scholar]
42. Eshun, K., He, Q. (2004). Aloe vera: A valuable ingredient for the food, pharmaceutical and cosmetic industries–A review. Critical Reviews in Food Science and Nutrition, 44(2), 91–96. DOI 10.1080/10408690490424694. [Google Scholar] [CrossRef]
43. Saberian, H., Hamidi-Esfahani, Z., Abbasi, S. (2013). Effect of pasteurization and storage on bioactive components of Aloe vera gel. Nutrition and Food Science, 43(2), 175–183. DOI 10.1108/00346651311313553. [Google Scholar] [CrossRef]
44. García-Torres, R., Ponagandla, N. R., Rouseff, R. L., Goodrich-Schneider, R. M., Reyes-De-Corcuera, J. I. (2009). Effects of dissolved oxygen in fruit juices and methods of removal. Comprehensive Reviews in Food Science and Food Safety, 8(4), 409–423. DOI 10.1111/j.1541-4337.2009.00090.x. [Google Scholar] [CrossRef]
45. Rodríguez, E. R., Martín, J. D., Romero, C. D. (2010). Aloe vera as a functional ingredient in foods. Critical Reviews in Food Science and Nutrition, 50(4), 305–326. DOI 10.1080/10408390802544454. [Google Scholar] [CrossRef]
46. Gao, Y., Kuok, K. I., Jin, Y., Wang, R. (2019). Biomedical applications of Aloe vera. Critical Reviews in Food Science and Nutrition, 59(sup1), S244–S256. DOI 10.1080/10408398.2018.1496320. [Google Scholar] [CrossRef]
47. Jiao, P., Jia, Q., Randel, G., Diehl, B., Weaver, S. et al. (2010). Quantitative 1H-NMR spectrometry method for quality control of Aloe vera products. Journal of AOAC International, 93(3), 842–848. [Google Scholar]
48. Miranda, M., Maureira, H., Rodríguez, K., Vega-Gálvez, A. (2009). Influence of temperature on the drying kinetics, physicochemical properties, and antioxidant capacity of Aloe vera (Aloe barbadensis Miller.) gel. Journal of Food Engineering, 91(2), 297–304. DOI 10.1016/j.jfoodeng.2008.09.007. [Google Scholar] [CrossRef]
49. Femenia, A., García-Pascual, P., Simal, S., Rosselló, C. (2003). Effects of heat treatment and dehydration on bioactive polysaccharide acemannan and cell wall polymers from Aloe barbadensis miller. Carbohydrate Polymers, 51(4), 397–405. DOI 10.1016/S0144-8617(02)00209-6. [Google Scholar] [CrossRef]
50. Minjares-Fuentes, R., Rodríguez-González, V. M., González-Laredo, R. F., Eim, V., González-Centeno, M. R. et al. (2017). Effect of different drying procedures on the bioactive polysaccharide acemannan from Aloe vera (Aloe barbadensis Miller.). Carbohydrate Polymers, 168, 327–336. DOI 10.1016/j.carbpol.2017.03.087. [Google Scholar] [CrossRef]
51. Gulia, A., Sharma, H. K., Sarkar, B. C., Upadhyay, A., Shitandi, A. (2010). Changes in physico-chemical and functional properties during convective drying of Aloe vera (Aloe barbadensis) leaves. Food and Bioproducts Processing, 88(2–3), 161–164. DOI 10.1016/j.fbp.2009.09.001. [Google Scholar] [CrossRef]
52. Canche-Escamilla, G., Colli-Acevedo, P., Borges-Argaez, R., Quintana-Owen, P., May-Crespo, J. F. et al. (2019). Extraction of phenolic components from an Aloe vera (Aloe barbadensis Miller.) crop and their potential as antimicrobials and textile dyes. Sustainable Chemistry and Pharmacy, 14, 100168. DOI 10.1016/j.scp.2019.100168. [Google Scholar] [CrossRef]
53. Oladejo, J., Shi, K., Chen, Y., Luo, X., Gang, Y. et al. (2020). Closing the active carbon cycle: Regeneration of spent activated carbon from a wastewater treatment facility for resource optimization. Chemical Engineering and Processing-Process Intensification, 150, 107878. DOI 10.1016/j.cep.2020.107878. [Google Scholar] [CrossRef]
54. El Gamal, M., Mousa, H. A., El-Naas, M. H., Zacharia, R., Judd, S. (2018). Bio-regeneration of activated carbon: A comprehensive review. Separation and Purification Technology, 197, 345–359. DOI 10.1016/j.seppur.2018.01.015. [Google Scholar] [CrossRef]
55. Yeow, P. K., Wong, S. W., Hadibarata, T. (2021). Removal of azo and anthraquinone dye by plant biomass as adsorbent–A review. Biointerface Research in Applied Chemistry, 11(1), 8218–8232. DOI 10.33263/BRIAC111.82188232. [Google Scholar] [CrossRef]
56. Nazario Patricia, Q. (2019). Ahorro y uso eficiente del agua y electricidad en la industrilization del gel de sábila. [Google Scholar]
57. Cheng, S., Panthapulakkal, S., Sain, M., Asiri, A. (2014). Aloe vera rind cellulose nanofibers-reinforced films. Journal of Applied Polymer Science, 131(15), 40592. [Google Scholar]
58. Lucini, L., Pellizzoni, M., Pellegrino, R., Molinari, G. P., Colla, G. (2015). Phytochemical constituents and in vitro radical scavenging activity of different aloe species. Food Chemistry, 170, 501–507. DOI 10.1016/j.foodchem.2014.08.034. [Google Scholar] [CrossRef]
59. Quezada, M. P., Salinas, C., Gotteland, M., Cardemil, L. (2017). Acemannan and fructans from Aloe vera (Aloe barbadensis Miller.) plants as novel prebiotics. Journal of Agricultural and Food Chemistry, 65(46), 10029–10039. DOI 10.1021/acs.jafc.7b04100. [Google Scholar] [CrossRef]
60. Shi, X. D., Nie, S. P., Yin, J. Y., Que, Z. Q., Zhang, L. J. et al. (2017). Polysaccharide from leaf skin of Aloe barbadensis miller: Part I. Extraction, fractionation, physicochemical properties and structural characterization. Food Hydrocolloids, 73, 176–183. DOI 10.1016/j.foodhyd.2017.06.039. [Google Scholar] [CrossRef]
61. Debnath, T., Ghosh, M., Lee, Y. M., Nath, N. C. D., Lee, K. G. et al. (2018). Identification of phenolic constituents and antioxidant activity of Aloe barbadensis flower extracts. Food and Agricultural Immunology, 29(1), 27–38. DOI 10.1080/09540105.2017.1358254. [Google Scholar] [CrossRef]
62. López-Cervantes, J., Sánchez-Machado, D. I., Cruz-Flores, P., Mariscal-Domínguez, M. F., Servín de la Mora-López, G. et al. (2018). Antioxidant capacity, proximate composition, and lipid constituents of Aloe vera flowers. Journal of Applied Research on Medicinal and Aromatic Plants, 10, 93–98. DOI 10.1016/j.jarmap.2018.02.004. [Google Scholar] [CrossRef]
63. Añibarro-Ortega, M., Pinela, J., Barros, L., Ćirić, A., Silva, S. P. et al. (2019). Compositional features and bioactive properties of Aloe vera leaf (Fillet, mucilage, and rind) and flower. Antioxidants, 8(10), 444. DOI 10.3390/antiox8100444. [Google Scholar] [CrossRef]
64. Faid, S. F. (2019). Phytochemical constituents from Aloe vera leaves and garden grass seeds and their utilization as anticancer activity. International Journal of Engineering Research & Technology, 8(11), 527–536. DOI 10.17577/IJERTV8IS110240. [Google Scholar] [CrossRef]
65. Shi, X. D., Yin, J. Y., Zhang, L. J., Li, O. Y., Huang, X. J. et al. (2019). Studies on polysaccharides from leaf skin of Aloe barbadensis miller: Part II. Structural characteristics and molecular properties of two lower molecular weight fractions. Food Hydrocolloids, 86, 50–61. DOI 10.1016/j.foodhyd.2018.01.038. [Google Scholar] [CrossRef]
66. Martínez-Sánchez, A., López-Cañavate, M. E., Guirao-Martínez, J., Roca, M. J., Aguayo, E. (2020). Aloe vera flowers, a byproduct with great potential and wide application, depending on maturity stage. Foods, 9(11), 1542. DOI 10.3390/foods9111542. [Google Scholar] [CrossRef]
67. Reynolds, T. (1985). The compounds in Aloë leaf exudates: A review. Botanical Journal of the Linnean Society, 90(3), 157–177. DOI 10.1111/j.1095-8339.1985.tb00377.x. [Google Scholar] [CrossRef]
68. Añibarro-Ortega, M., Pinela, J., Ćirić, A., Lopes, E., Molina, A. K. et al. (2021). Extraction of aloesin from Aloe vera rind using alternative green solvents: Process optimization and biological activity assessment. Biology, 10(10), 951. DOI 10.3390/biology10100951. [Google Scholar] [CrossRef]
69. Mandrioli, R., Mercolini, L., Ferranti, A., Fanali, S., Raggi, M. A. (2011). Determination of aloe emodin in Aloe vera extracts and commercial formulations by HPLC with tandem UV absorption and fluorescence detection. Food Chemistry, 126(1), 387–393. DOI 10.1016/j.foodchem.2010.10.112. [Google Scholar] [CrossRef]
70. López, Z., Núñez-Jinez, G., Avalos-Navarro, G., Rivera, G., Salazar-Flores, J. et al. (2017). Antioxidant and cytotoxicological effects of Aloe vera food supplements. Journal of Food Quality, 2017, 1–10. DOI 10.1155/2017/7636237. [Google Scholar] [CrossRef]
71. Majumder, R., Das, C. K., Mandal, M. (2019). Lead bioactive compounds of Aloe vera as potential anticancer agent. Pharmacological Research, 148, 104416. DOI 10.1016/j.phrs.2019.104416. [Google Scholar] [CrossRef]
72. Borges-Argáez, R., Chan-Balan, R., Cetina-Montejo, L., Ayora-Talavera, G., Sansores-Peraza, P. et al. (2019). In vitro evaluation of anthraquinones from Aloe vera (Aloe barbadensis Miller.) roots and several derivatives against strains of influenza virus. Industrial Crops and Products, 132, 468–475. DOI 10.1016/j.indcrop.2019.02.056. [Google Scholar] [CrossRef]
73. Saleem, R., Faizi, S., Deeba, F., Siddiqui, B. S., Qazi, M. H. (1997). A new bisbenzopyran from aloe barbadensis roots. Planta Medica, 63(5), 454–456. DOI 10.1055/s-2006-957732. [Google Scholar] [CrossRef]
74. Reynolds, T. (2004). Aloes: The genus Aloe. Boca Raton: CRC Press. [Google Scholar]
75. Tojeira, A., Pereira, R., Bártolo, P., Mendes, A., Vaz, D. et al. (2010). Monitoring the modifications of Aloe vera by high performance liquid chromatography. V Congresso Internacional de BiomaterialesHabana, Cuba. [Google Scholar]
76. Keyhanian, S., Stahl-Biskup, E. (2007). Phenolic constituents in dried flowers of Aloe vera (Aloe barbadensis) and their in vitro antioxidative capacity. Planta Medica, 73(6), 599–602. DOI 10.1055/s-2007-967202. [Google Scholar] [CrossRef]
77. Sotelo, A., López-García, S., Basurto-Peña, F. (2007). Content of nutrient and antinutrient in edible flowers of wild plants in Mexico. Plant Foods for Human Nutrition, 62(3), 133–138. DOI 10.1007/s11130-007-0053-9. [Google Scholar] [CrossRef]
78. Chang, X. L., Chen, B. Y., Feng, Y. M. (2011). Water-soluble polysaccharides isolated from skin juice, gel juice and flower of Aloe vera miller. Journal of the Taiwan Institute of Chemical Engineers, 42(2), 197–203. DOI 10.1016/j.jtice.2010.07.007. [Google Scholar] [CrossRef]
79. Nejatzadeh-Barandozi, F., Enferadi, S. (2012). FT-IR study of the polysaccharides isolated from the skin juice, gel juice, and flower of Aloe vera tissues affected by fertilizer treatment. Organic and Medicinal Chemistry Letters, 2(1), 1–9. DOI 10.1186/2191-2858-2-33. [Google Scholar] [CrossRef]
80. Tan, Z. J., Li, F. F., Xu, X. L. (2013). Extraction and purification of anthraquinones derivatives from Aloe vera L. using alcohol/salt aqueous two-phase system. Bioprocess and Biosystems Engineering, 36(8), 1105–1113. DOI 10.1007/s00449-012-0864-4. [Google Scholar] [CrossRef]
81. López, A., de Tangil, M. S., Vega-Orellana, O., Ramírez, A. S., Rico, M. (2013). Phenolic constituents, antioxidant and preliminary antimycoplasmic activities of leaf skin and flowers of Aloe vera (L.) burm. f. (syn. A. barbadensis mill.) from the canary islands (Spain). Molecules, 18(5), 4942–4954. DOI 10.3390/molecules18054942. [Google Scholar] [CrossRef]
82. Geng, L., Zhou, W., Qu, X., Chen, W., Li, Y. et al. (2014). Optimization of the preparation of pectin from Aloe using a Box-Behnken design. Carbohydrate Polymers, 105(1), 193–199. DOI 10.1016/j.carbpol.2014.01.069. [Google Scholar] [CrossRef]
83. Ma, L. K., Chen, K., Tian, X. F., Gao, Y. H., Zhang, S. A. et al. (2020). Effect of particle size on the physicochemical property of the peel powder from Aloe barbadensis. IOP Conference Series: Earth and Environmental Science, 612(1), 012005. DOI 10.1088/1755-1315/612/1/012005. [Google Scholar] [CrossRef]
84. Vidic, D., Tarić, E., Alagić J., Maksimović, M. (2014). Determination of total phenolic content and antioxidant activity of ethanol extracts from Aloe spp. Bulletin of the Chemists and Technologists of Bosnia and Herzegovina, 42, 5–10. [Google Scholar]
85. Sánchez-Machado, D. I., López-Cervantes, J., Mariscal-Domínguez, M. F., Cruz-Flores, P., Campas-Baypoli, O. N. et al. (2017). An HPLC procedure for the quantification of aloin in latex and gel from Aloe barbadensis leaves. Journal of Chromatographic Science, 55(3), 251–257. DOI 10.1093/chromsci/bmw179. [Google Scholar] [CrossRef]
86. Lotfizadeh, V., Mollaei, S., Hazrati, S. (2021). Biological activities of aloin-rich extracts obtained from Aloe vera L. Journal of Medicinal Plants and by-Product, 1–7. DOI 10.22092/JMPB.2021.355897.1395. [Google Scholar] [CrossRef]
87. Nakiguli, C. K., Kosgei, V. J., Cherutoi, J. K., Odda, J. (2022). Phytochemical composition and antioxidant activities of phosphate buffered saline and aqueous extracts of Aloe barbadensis miller leaf latex and gel from three counties of Kenya. Asian Journal of Applied Chemistry Research, 11(1), 17–32. DOI 10.9734/ajacr/2022/v11i130244. [Google Scholar] [CrossRef]
88. Haq, A., Ali, Q., Rashid, M. S., Waheed, F., Hayat, S. et al. (2020). Antibacterial and antifungal activity of Aloe vera plant. Life Science Journal, 17(7), 76–82. [Google Scholar]
89. Abdissa, N., Induli, M., Fitzpatrick, P., Alao, J. P., Sunnerhagen, P. et al. (2014). Cytotoxic quinones from the roots of Aloe dawei. Molecules, 19(3), 3264–3273. DOI 10.3390/molecules19033264. [Google Scholar] [CrossRef]
90. Singh, P., Hundal, J. S., Patra, A. K., Wadhwa, M., Sharma, A. (2021). Sustainable utilization of Aloe vera waste in the diet of lactating cows for improvement of milk production performance and reduction of carbon footprint. Journal of Cleaner Production, 288, 125118. DOI 10.1016/j.jclepro.2020.125118. [Google Scholar] [CrossRef]
91. Quirós-Pozo, R., Ventura-Castellano, A., Ramírez-Bolaños, S., Roo-Filgueira, J., Robaina, L. (2021). Evaluation of Aloe vera by-product against cereals in feeds for golden mullet (Liza aurata). Aquaculture Reports, 20, 100659. DOI 10.1016/j.aqrep.2021.100659. [Google Scholar] [CrossRef]
92. Giannakoudakis, D. A., Hosseini-Bandegharaei, A., Tsafrakidou, P., Triantafyllidis, K. S., Kornaros, M. et al. (2018). Aloe vera waste biomass-based adsorbents for the removal of aquatic pollutants: A review. Journal of Environmental Management, 227, 354–364. DOI 10.1016/j.jenvman.2018.08.064. [Google Scholar] [CrossRef]
93. Katubi, K. M., Amari, A., Harharah, H. N., Eldirderi, M. M., Tahoon, M. A. et al. (2021). Aloe vera as promising material for water treatment: A review. Processes, 9(5), 782. DOI 10.3390/pr9050782. [Google Scholar] [CrossRef]
94. Meez, E., Hosseini-Bandegharaei, A., Rahdar, A., Thysiadou, A., Matis, K. A. et al. (2021). Synthetic oil-spills decontamination by using sawdust and activated carbon from Aloe vera as absorbents. Biointerface Research in Applied Chemistry, 11(4), 11778–11796. DOI 10.33263/BRIAC114.1177811796. [Google Scholar] [CrossRef]
95. Hellen Sathya, D. J., Turakhia, A. M., Kumar, M. A., Balaji, N., Selvanaveen, S. et al. (2017). Bioethanol from saccharificed lignocellulosic rich Aloe vera rinds using saccharomyces cerevisiae MTCC 4779. Energy Sources, Part A: Recovery, Utilization and Environmental Effects, 39(13), 1347–1352. DOI 10.1080/15567036.2017.1328004. [Google Scholar] [CrossRef]
96. Rajeswari, G., Jacob, S. (2020). Deciphering the Aloe vera leaf rind as potent feedstock for bioethanol through enzymatic delignification and its enhanced saccharification. Industrial Crops and Products, 143, 111876. DOI 10.1016/j.indcrop.2019.111876. [Google Scholar] [CrossRef]
97. Rajeswari, G., Arutselvy, B., Jacob, S. (2020). Delignification of Aloe vera rind by mild acid associated microwave pretreatment to persuade enhanced enzymatic saccharification. Waste and Biomass Valorization, 11(11), 5965–5975. DOI 10.1007/s12649-019-00830-7. [Google Scholar] [CrossRef]
98. Rajeswari, G., Jacob, S. (2021). Saccharolysis of laccase delignified Aloe vera leaf rind and fermentation through free and immobilized yeast for ethanol production. Journal of Food Process Engineering, 44(2), e13514. DOI 10.1111/jfpe.13514. [Google Scholar] [CrossRef]
99. Forrest, A. K., Sierra, R., Holtzapple, M. T. (2010). Suitability of pineapple, Aloe vera, molasses, glycerol, and office paper as substrates in the MixAlco processTM. Biomass and Bioenergy, 34(8), 1195–1200. DOI 10.1016/j.biombioe.2010.03.013. [Google Scholar] [CrossRef]
100. Huang, X., Yun, S., Zhu, J., Du, T., Zhang, C. et al. (2016). Mesophilic anaerobic co-digestion of aloe peel waste with dairy manure in the batch digester: Focusing on mixing ratios and digestate stability. Bioresource Technology, 218, 62–68. DOI 10.1016/j.biortech.2016.06.070. [Google Scholar] [CrossRef]
101. Wang, Z., Yun, S., Xu, H., Wang, C., Zhang, Y. et al. (2019). Mesophilic anaerobic co-digestion of acorn slag waste with dairy manure in a batch digester: Focusing on mixing ratios and bio-based carbon accelerants. Bioresource Technology, 286, 121394. DOI 10.1016/j.biortech.2019.121394. [Google Scholar] [CrossRef]
102. Chen, J., Yun, S., Shi, J., Wang, Z., Abbas, Y. et al. (2020). Role of biomass-derived carbon-based composite accelerants in enhanced anaerobic digestion: Focusing on biogas yield, fertilizer utilization, and density functional theory calculations. Bioresource Technology, 307, 123204. DOI 10.1016/j.biortech.2020.123204. [Google Scholar] [CrossRef]
103. Jia, B., Yun, S., Shi, J., Han, F., Wang, Z. et al. (2020). Enhanced anaerobic mono-and co-digestion under mesophilic condition: Focusing on the magnetic field and Ti-sphere core–shell structured additives. Bioresource Technology, 310, 123450. DOI 10.1016/j.biortech.2020.123450. [Google Scholar] [CrossRef]
104. Xu, H., Yun, S., Wang, C., Wang, Z., Han, F. et al. (2020). Improving performance and phosphorus content of anaerobic co-digestion of dairy manure with aloe peel waste using vermiculite. Bioresource Technology, 301, 122753. DOI 10.1016/j.biortech.2020.122753. [Google Scholar] [CrossRef]
105. Abbas, Y., Yun, S., Wang, K., Ali Shah, F., Xing, T. et al. (2021). Static-magnetic-field coupled with fly-ash accelerant: A powerful strategy to significantly enhance the mesophilic anaerobic-co-digestion. Bioresource Technology, 327, 124793. DOI 10.1016/j.biortech.2021.124793. [Google Scholar] [CrossRef]
106. Ke, T., Yun, S., Wang, K., An, J., Liu, L. et al. (2021). Enhanced anaerobic co-digestion performance by using surface-annealed titanium spheres at different atmospheres. Bioresource Technology, 347, 126341. DOI 10.1016/j.biortech.2021.126341. [Google Scholar] [CrossRef]
107. Xing, T., Yun, S., Li, B., Wang, K., Chen, J. et al. (2021). Coconut-shell-derived bio-based carbon enhanced microbial electrolysis cells for upgrading anaerobic co-digestion of cow manure and aloe peel waste. Bioresource Technology, 338, 125520. DOI 10.1016/j.biortech.2021.125520. [Google Scholar] [CrossRef]
108. Nanda, S., Isen, J., Dalai, A. K., Kozinski, J. A. (2016). Gasification of fruit wastes and agro-food residues in supercritical water. Energy Conversion and Management, 110, 296–306. DOI 10.1016/j.enconman.2015.11.060. [Google Scholar] [CrossRef]
109. Cheng, S., Panthapulakkal, S., Ramezani, N., Asiri, A. M., Sain, M. (2014). Aloe vera rind nanofibers: Effect of isolation process on the tensile properties of nanofibre films. BioResources, 9(4), 7653–7665. DOI 10.15376/biores.9.4.7653-7665. [Google Scholar] [CrossRef]
110. Kakroodi, A. R., Cheng, S., Sain, M., Asiri, A. (2014). Mechanical, thermal, and morphological properties of nanocomposites based on polyvinyl alcohol and cellulose nanofiber from Aloe vera rind. Journal of Nanomaterials, 2014, 1–7. DOI 10.1155/2014/903498. [Google Scholar] [CrossRef]
111. Chaitanya, S., Singh, I. (2016). Novel Aloe vera fiber reinforced biodegradable composites-development and characterization. Journal of Reinforced Plastics and Composites, 35(19), 1411–1423. DOI 10.1177/0731684416652739. [Google Scholar] [CrossRef]
112. Chaitanya, S., Singh, I. (2018). Ecofriendly treatment of Aloe vera fibers for PLA based green composites. International Journal of Precision Engineering and Manufacturing-Green Technology, 5(1), 143–150. DOI 10.1007/s40684-018-0015-8. [Google Scholar] [CrossRef]
113. Ramesh, P., Prasad, B. D., Narayana, K. L. (2020). Effect of fiber hybridization and montmorillonite clay on properties of treated kenaf/Aloe vera fiber reinforced PLA hybrid nanobiocomposite. Cellulose, 27(12), 6977–6993. DOI 10.1007/s10570-020-03268-6. [Google Scholar] [CrossRef]
114. Dehouche, N., Idres, C., Kaci, M., Zembouai, I., Bruzaud, S. (2020). Effects of various surface treatments on Aloe vera fibers used as reinforcement in poly(3-hydroxybutyrate-co-3-hydroxyhexanoate) (PHBHHx) biocomposites. Polymer Degradation and Stability, 175, 109131. DOI 10.1016/j.polymdegradstab.2020.109131. [Google Scholar] [CrossRef]
115. Jeyaraj, M., Praphakar, R. A., Rajendran, C., Ponnamma, D., Sadasivuni, K. K. et al. (2016). Surface functionalization of natural lignin isolated from: Aloe barbadensis Miller biomass by atom transfer radical polymerization for enhanced anticancer efficacy. RSC Advances, 6(56), 51310–51319. DOI 10.1039/c6ra01866a. [Google Scholar] [CrossRef]
116. Wang, G., Su, P., Zhang, F., Hou, X., Yang, Y. et al. (2011). Comparison of microwave-assisted extraction of aloe-emodin in aloe with Soxhlet extraction and ultrasound-assisted extraction. Science China Chemistry, 54(1), 231–236. DOI 10.1007/s11426-010-4017-9. [Google Scholar] [CrossRef]
117. Gao, J., Zhang, G., Dai, R., Bi, K. (2004). Isolation of aloinoside B and metabolism by rat intestinal bacteria. Pharmaceutical Biology, 42(8), 581–587. DOI 10.1080/13880200490902446. [Google Scholar] [CrossRef]
118. Ajila, C. M., Brar, S. K., Verma, M., Tyagi, R. D., Godbout, S. et al. (2012). Bio-processing of agro-byproducts to animal feed. Critical Reviews in Biotechnology, 32(4), 382–400. DOI 10.3109/07388551.2012.659172. [Google Scholar] [CrossRef]
119. Sirohi, S. K., Pandey, N., Goel, N., Singh, B., Mohini, M. et al. (2009). Microbial activity and ruminal methanogenesis as affected by plant secondary metabolites in different plant extracts. Environmental Engineering, 1(1), 52–58. [Google Scholar]
120. Calabrò, S., Musco, N., Tudisco, R., Grossi, M., Calabrò, V. et al. (2013). Effect of Aloe arborescens on in vitro rumen fermentations. Journal of Nutritional Ecology and Food Research, 1(2), 117–123. DOI 10.1166/jnef.2013.1014. [Google Scholar] [CrossRef]
121. Bani, P., Grossi, P., Lucini, L., Pellizzoni, M., Minuti, A. et al. (2016). Administration of Aloe arborescens homogenate to cattle: Interaction with rumen fermentation and gut absorption of aloin. Italian Journal of Animal Science, 15(2), 233–240. DOI 10.1080/1828051X.2016.1157007. [Google Scholar] [CrossRef]
122. Bhati, M., Dhuria, R. K., Sharma, T., Meel, M. S., Saini, S. K. (2017). Effect of Aloe vera as herbal feed additive on digestibility of nutrients and rumen fermentation in rathi calves. Veterinary Practitioner, 18(2), 282–283. [Google Scholar]
123. Saini, R., Chhikara, S. K., Sahu, S., Yadav, D. C., Kumar, P. (2021). Aloe vera (Aloe barbadensis) as feed additive for buffalo calves: Effects on Gut parasites and cost of production. Journal of Animal Research, 11(4), 675–679. DOI 10.30954/2277-940X.04.2021.15. [Google Scholar] [CrossRef]
124. Banakar, P. S., Kumar, S., Vinay, V. V., Dixit, S., Tyagi, N. et al. (2021). Supplementation of Aloe vera extract in lactating goats’ diet: Effects on rumen fermentation efficiency, nutrient utilization, lactation performance, and antioxidant status. Tropical Animal Health and Production, 53(5), 1–10. DOI 10.1007/s11250-021-02894-x. [Google Scholar] [CrossRef]
125. Bush, J., van den Boom, R., Franklin, S. (2018). Comparison of Aloe vera and omeprazole in the treatment of equine gastric ulcer syndrome. Equine Veterinary Journal, 50(1), 30–40. DOI 10.1111/evj.12706. [Google Scholar] [CrossRef]
126. Babak, D., Nahashon, S. N. (2014). A review on effects of Aloe vera as a feed additive in broiler chicken diets. Annals of Animal Science, 14(3), 491–500. DOI 10.2478/aoas-2014-0026. [Google Scholar] [CrossRef]
127. Ahmad, Z., Hafeez, A., Ullah, Q., Naz, S., Khan, R. U. (2020). Protective effect of Aloe vera on growth performance, leucocyte count and intestinal injury in broiler chicken infected with coccidiosis. Journal of Applied Animal Research, 48(1), 252–256. DOI 10.1080/09712119.2020.1773473. [Google Scholar] [CrossRef]
128. Akram, M. Z., Salman, M., Jalal, H., Asghar, M. U., Ali, Z. et al. (2019). Evaluation of dietary supplementation of Aloe vera as an alternative to antibiotic growth promoters in broiler production. Turkish Journal of Veterinary Research, 3(1), 21–26. [Google Scholar]
129. Qiao, J., Li, H. H., Zheng, C. J., Feng, Z. Y., Wang, W. (2013). Dietary supplementation with Aloe vera polysaccharide enhances the growth performance and immune function of weaned piglets. Journal of Animal and Feed Sciences, 22(4), 329–334. DOI 10.22358/jafs/65921/2013. [Google Scholar] [CrossRef]
130. Heidarieh, M., Mirvaghefi, A. R., Sepahi, A., Sheikhzadeh, N., Shahbazfar, A. A. et al. (2013). Effects of dietary Aloe vera on growth performance, skin and gastrointestine morphology in rainbow trout (Oncorhynchus mykiss). Turkish Journal of Fisheries and Aquatic Sciences, 13(2), 367–373. DOI 10.4194/1303-2712-v13_2_20. [Google Scholar] [CrossRef]
131. Sharif Rohani, M., Haghighi, M., Bazari Moghaddam, S. (2017). Study on nanoparticles of Aloe vera extract on growth performance, survival rate and body composition in Siberian sturgeon (Acipenser baerii). Iranian Journal of Fisheries Sciences, 16(2), 457–468. [Google Scholar]
132. Gabriel, N. N., Qiang, J., He, J., Ma, X. Y., Kpundeh, M. D. et al. (2015). Dietary Aloe vera supplementation on growth performance, some haemato-biochemical parameters and disease resistance against streptococcus iniae in tilapia (GIFT). Fish and Shellfish Immunology, 44(2), 504–514. DOI 10.1016/j.fsi.2015.03.002. [Google Scholar] [CrossRef]
133. Ajibola, S. I., Obasa, S. O., Akintokun, A. K., Abdulraheem, I. (2021). Body weight changes, nutrient utilization and intestinal microflora of African catfish (Clarias gariepinus) fed Aloe barbadensis leaves. Nigerian Journal of Animal Production, 43(2), 385–398. DOI 10.51791/njap.v43i2.725. [Google Scholar] [CrossRef]
134. Ibidunni, A. S., Olubodun, O. S., Ikililu, A. (2018). Growth performance, haematology and histopathology of African catfish (Clarias gariepinus) fed varying levels of Aloe barbadensis leaves. Journal of Fisheries, 6(1), 553–562. DOI 10.17017/jfish.v6i1.2018.245. [Google Scholar] [CrossRef]
135. Gabriel, N. N., Wilhelm, M. R., Habte-Tsion, H. M., Chimwamurombe, P., Omoregie, E. et al. (2019). Effect of dietary Aloe vera polysaccharides supplementation on growth performance, feed utilization, hemato-biochemical parameters, and survival at low pH in African catfish (Clarias gariepinus) fingerlings. International Aquatic Research, 11(1), 57–72. DOI 10.1007/s40071-019-0219-8. [Google Scholar] [CrossRef]
136. Khanal, M., Lamichhane, S., Bhattarai, A., Kayastha, B. L., Labh, S. N. (2021). Extract of Aloe vera (Aloe barbadensis Miller.) enhances the growth, protein contents, and gastrosomatic index (GaSI) of common carp cyprinus carpio. Journal of Nutrition and Metabolism, 2021, 8029413. DOI 10.1155/2021/8029413. [Google Scholar] [CrossRef]
137. Syed, R., Masood, Z., Ul Hassan, H., Khan, W., Mushtaq, S. et al. (2022). Growth performance, haematological assessment and chemical composition of Nile tilapia, oreochromis niloticus (Linnaeus, 1758) fed different levels of Aloe vera extract as feed additives in a closed aquaculture system. Saudi Journal of Biological Sciences, 29(1), 296–303. DOI 10.1016/j.sjbs.2021.08.098. [Google Scholar] [CrossRef]
138. Mahdavi, M., Hajimoradloo, A., Ghorbani, R. (2013). Effect of Aloe vera extract on growth parameters of common carp (Cyprinus carpio). World Journal of Medical Sciences, 9(1), 55–60. DOI 10.5829/idosi.wjms.2013.9.1.75128. [Google Scholar] [CrossRef]
139. Kumar, M., Rakesh, S., Nagpal, R., Hemalatha, R., Ramakrishna, A. et al. (2013). Probiotic lactobacillus rhamnosus GG and Aloe vera gel improve lipid profiles in hypercholesterolemic rats. Nutrition, 29(3), 574–579. DOI 10.1016/j.nut.2012.09.006. [Google Scholar] [CrossRef]
140. Ghasemi-Sadabadi, M., Veldkamp, T., van Krimpen, M., Ebrahimnezhad, Y., Ghalehkandi, J. G. et al. (2020). Determining tolerance of Japanese quail to different dietary fat peroxidation values by supplementation with rosemary and Aloe vera on performance and meat quality. Animal Feed Science and Technology, 267, 114574. DOI 10.1016/j.anifeedsci.2020.114574. [Google Scholar] [CrossRef]
141. Malik, R., Lata, S., Singhal, S. (2015). Removal of heavy metal from waste water by the use of modified Aloe vera leaf powder. International Journal of Basic and Applied Chemical Sciences, 5(2), 6–17. [Google Scholar]
142. Noli, F., Kapashi, E., Kapnisti, M. (2019). Biosorption of uranium and cadmium using sorbents based on Aloe vera wastes. Journal of Environmental Chemical Engineering, 7(2), 102985. DOI 10.1016/j.jece.2019.102985. [Google Scholar] [CrossRef]
143. Arivoli, S., Sundaravadivelu, M., Elango, K. P. (2008). Removal of basic and acidic dyes from aqueous solution by adsorption on a low cost activated carbon: Kinetic and thermodynamic study. Indian Journal of Chemical Technology, 15(2), 130–139. [Google Scholar]
144. Khaniabadi, Y. O., Basiri, H., Jafari, A., Saeedi, S., Goudarzi, G. et al. (2016). Removal of methylene blue from aqueous solution by activated carbon from Aloe vera wastes. Jundishapur Journal of Health Sciences, 10(4), e38242. DOI 10.17795/jjhs-38242. [Google Scholar] [CrossRef]
145. Khaniabadi, Y. O., Heydari, R., Nourmoradi, H., Basiri, H., Basiri, H. (2016). Low-cost sorbent for the removal of aniline and methyl orange from liquid-phase: Aloe vera leaves wastes. Journal of the Taiwan Institute of Chemical Engineers, 68, 90–98. DOI 10.1016/j.jtice.2016.09.025. [Google Scholar] [CrossRef]
146. Gupta, S., Kumar, A. (2019). Removal of nickel (II) from aqueous solution by biosorption on A. barbadensis Miller waste leaves powder. Applied Water Science, 9(4), 1–11. DOI 10.1007/s13201-019-0973-1. [Google Scholar] [CrossRef]
147. Gupta, S., Sharma, S. K., Kumar, A. (2019). Biosorption of Ni (II) ions from aqueous solution using modified Aloe barbadensis miller leaf powder. Water Science and Engineering, 12(1), 27–36. DOI 10.1016/j.wse.2019.04.003. [Google Scholar] [CrossRef]
148. Gupta, S., Kumar A. (2021). Biosorption of Ni(II) from aqueous solutions and real industrial wastewater using modified A. barbadensis miller leaves residue powder in a lab scale continuous fixed bed column. Cleaner Engineering and Technology, 5, 100349. DOI 10.1016/j.clet.2021.100349. [Google Scholar] [CrossRef]
149. Haji Esmaeili, S. A., Szmerekovsky, J., Sobhani, A., Dybing, A., Peterson, T. O. (2020). Sustainable biomass supply chain network design with biomass switching incentives for first-generation bioethanol producers. Energy Policy, 138, 111222. DOI 10.1016/j.enpol.2019.111222. [Google Scholar] [CrossRef]
150. Muscat, A., de Olde, E. M., de Boer, I. J. M., Ripoll-Bosch, R. (2020). The battle for biomass: A systematic review of food-feed-fuel competition. Global Food Security, 25, 100330. DOI 10.1016/j.gfs.2019.100330. [Google Scholar] [CrossRef]
151. Sarkar, N., Ghosh, S. K., Bannerjee, S., Aikat, K. (2012). Bioethanol production from agricultural wastes: An overview. Renewable Energy, 37(1), 19–27. DOI 10.1016/j.renene.2011.06.045. [Google Scholar] [CrossRef]
152. Gupta, A., Verma, J. P. (2015). Sustainable bio-ethanol production from agro-residues: A review. Renewable and Sustainable Energy Reviews, 41, 550–567. DOI 10.1016/j.rser.2014.08.032. [Google Scholar] [CrossRef]
153. Holtzapple, M. T., Granda, C. B. (2009). Carboxylate platform: The MixAlco process. Part 1: Comparison of three biomass conversion platforms. Applied Biochemistry and Biotechnology, 156(1–3), 95–106. DOI 10.1007/s12010-008-8466-y. [Google Scholar] [CrossRef]
154. Granda, C. B., Holtzapple, M. T., Luce, G., Searcy, K., Mamrosh, D. L. (2009). Carboxylate platform: The MixAlco process. Part 2: Process economics. Applied Biochemistry and Biotechnology, 156(1–3), 107–124. DOI 10.1007/s12010-008-8481-z. [Google Scholar] [CrossRef]
155. Pham, V., Holtzapple, M., El-Halwagi, M. (2010). Techno-economic analysis of biomass to fuel conversion via the MixAlco process. Journal of Industrial Microbiology and Biotechnology, 37(11), 1157–1168. DOI 10.1007/s10295-010-0763-0. [Google Scholar] [CrossRef]
156. Xu, N., Liu, S., Xin, F., Zhou, J., Jia, H. et al. (2019). Biomethane production from lignocellulose: Biomass recalcitrance and its impacts on anaerobic digestion. Frontiers in Bioengineering and Biotechnology, 7(191), 1–12. DOI 10.3389/fbioe.2019.00191. [Google Scholar] [CrossRef]
157. Alven, S., Khwaza, V., Oyedeji, O. O., Aderibigbe, B. A. (2021). Polymer-based scaffolds loaded with Aloe vera extract for the treatment of wounds. Pharmaceutics, 13(7), 961. DOI 10.3390/pharmaceutics13070961. [Google Scholar] [CrossRef]
158. Balaji, A., Vellayappan, M. V., John, A. A., Subramanian, A. P., Jaganathan, S. K. et al. (2015). Biomaterials based nano-applications of Aloe vera and its perspective: A review. RSC Advances, 5(105), 86199–86213. DOI 10.1039/c5ra13282g. [Google Scholar] [CrossRef]
159. Rahman, S., Carter, P., Bhattarai, N. (2017). Aloe vera for tissue engineering applications. Journal of Functional Biomaterials, 8(1), 6. DOI 10.3390/jfb8010006. [Google Scholar] [CrossRef]
160. Darzi, S., Paul, K., Leitan, S., Werkmeister, J. A., Mukherjee, S. (2021). Immunobiology and application of Aloe vera-based scaffolds in tissue engineering. International Journal of Molecular Sciences, 22(4), 1708. DOI 10.3390/ijms22041708. [Google Scholar] [CrossRef]
161. Kang, S., Zhao, X., Yue, L., Liu, L. (2017). Main anthraquinone components in Aloe vera and their inhibitory effects on the formation of advanced glycation end-products. Journal of Food Processing and Preservation, 41(5), e13160. DOI 10.1111/jfpp.13160. [Google Scholar] [CrossRef]
162. Syed, A. M., Kundu, S., Ram, C., Kulhari, U., Kumar, A. et al. (2022). Aloin alleviates pathological cardiac hypertrophy via modulation of the oxidative and fibrotic response. Life Sciences, 288, 120159. DOI 10.1016/j.lfs.2021.120159. [Google Scholar] [CrossRef]
163. Globe Newswire (2019). Anthraquinone market worth US$2.2 billion by 2025: Acumen research and consulting. https://www.globenewswire.com/news-release/2019/01/11/1690490/0/en/Anthraquinone-Market-Worth-US-2-2-Billion-By-2025-Acumen-Research-and-Consulting.html. [Google Scholar]
164. Sanders, B., Ray, A. M., Goldberg, S., Clark, T., McDaniel, H. R. et al. (2017). Anti-cancer effects of aloe-emodin: A systematic review. Journal of Clinical and Translational Research, 3(3), 283–296. DOI 10.18053/jctres.03.201703.001. [Google Scholar] [CrossRef]
165. Al Kazman, B. S. M., Prieto, J. M. (2021). Friends or foes? Cytotoxicity, HPTLC and NMR analyses of some important naturally occurring hydroxyanthraquinones. Nutraceuticals, 1(1), 13–30. DOI 10.3390/nutraceuticals1010004. [Google Scholar] [CrossRef]
166. Reddy, V. P., Beyaz, A. (2006). Inhibitors of the maillard reaction and AGE breakers as therapeutics for multiple diseases. Drug Discovery Today, 11(13–14), 646–654. DOI 10.1016/j.drudis.2006.05.016. [Google Scholar] [CrossRef]
167. Reddy, V. P., Zhu, X., Perry, G., Smith, M. A. (2009). Oxidative stress in diabetes and Alzheimer’s disease. Journal of Alzheimer’s Disease, 16(4), 763–774. DOI 10.3233/JAD-2009-1013. [Google Scholar] [CrossRef]
168. Xu, Y., Yu, X., Gui, J., Wan, Y., Chen, J. et al. (2022). Ultrasonic solvent extraction followed by dispersive solid phase extraction (d-SPE) cleanup for the simultaneous determination of five anthraquinones in Polygonum multiflorum by UHPLC-PDA. Foods, 11(3), 386. DOI 10.3390/foods11030386. [Google Scholar] [CrossRef]
169. Alam, P., Noman, O. M., Herqash, R. N., Almarfadi, O. M., Akhtar, A. et al. (2022). Response surface methodology (RSM)-Based optimization of ultrasound-assisted extraction of sennoside A, sennoside B, aloe-emodin, emodin, and chrysophanol from Senna alexandrina (aerial partsHPLC-UV and antioxidant analysis. Molecules, 27(1), 298. DOI 10.3390/molecules27010298. [Google Scholar] [CrossRef]
170. Wu, X., Zhong, J., Xie, Z., Ding, W., Wan, J. (2013). Isolation and purification of aloin, isoaloeresin d and aloenin-2′-p-coumaroyl ester from Aloe barbadensis mill by high-speed counter-current chromatography. Journal of Liquid Chromatography and Related Technologies, 36(18), 2589–2600. DOI 10.1080/10826076.2012.723094. [Google Scholar] [CrossRef]
171. Rauwald, H. W., Maucher, R., Dannhardt, G., Kuchta, K. (2021). Dihydroisocoumarins, naphthalenes, and further polyketides from Aloe vera and A. plicatilis: Isolation, identification and their 5-LOX/COX-1 inhibiting potency. Molecules, 26(14), 4223. DOI 10.3390/molecules26144223. [Google Scholar] [CrossRef]
172. Cui, Y., Ye, Q., Wang, H., Li, Y., Xia, X. et al. (2014). Aloin protects against chronic alcoholic liver injury via attenuating lipid accumulation, oxidative stress and inflammation in mice. Archives of Pharmacal Research, 37(12), 1624–1633. DOI 10.1007/s12272-014-0370-0. [Google Scholar] [CrossRef]
173. Yang, S., Lee, W., Lee, B. S., Lee, C., Park, E. K. et al. (2019). Aloin reduces HMGB1-mediated septic responses and improves survival in septic mice by activation of the SIRT1 and PI3K/Nrf2/HO-1 signaling axis. American Journal of Chinese Medicine, 47(3), 613–633. DOI 10.1142/S0192415X19500320. [Google Scholar] [CrossRef]
174. Rubio-Martínez, J., Jiménez-Alesanco, A., Ceballos-Laita, L., Ortega-Alarcón, D., Vega, S. et al. (2021). Discovery of diverse natural products as inhibitors of SARS-CoV-2 MproProtease through virtual screening. Journal of Chemical Information and Modeling, 61(12), 6094–6106. DOI 10.1021/acs.jcim.1c00951. [Google Scholar] [CrossRef]
175. Abouelela, M. E., Assaf, H. K., Abdelhamid, R. A., Elkhyat, E. S., Sayed, A. M. et al. (2021). Identification of potential SARS-CoV-2 main protease and spike protein inhibitors from the genus Aloe: An in silico study for drug development. Molecules, 26(6), 1767. DOI 10.3390/molecules26061767. [Google Scholar] [CrossRef]
176. Khan, S. L., Siddiqui, F. A. (2020). Beta-sitosterol: As immunostimulant, antioxidant and inhibitor of SARS-CoV-2 spike glycoprotein. Archives of Pharmacology and Therapeutics, 2(1), 12–16. [Google Scholar]
177. Choi, S., Park, Y. I., Lee, S. K., Kim, J. E., Chung, M. H. (2002). Aloesin inhibits hyperpigmentation induced by UV radiation. Clinical and Experimental Dermatology, 27(6), 513–515. DOI 10.1046/j.1365-2230.2002.01120.x. [Google Scholar] [CrossRef]
178. Mikayoulou, M., Mayr, F., Temml, V., Pandian, A., Vermaak, I. et al. (2021). Anti-tyrosinase activity of South African Aloe species and isolated compounds plicataloside and aloesin. Fitoterapia, 150, 104828. DOI 10.1016/j.fitote.2021.104828. [Google Scholar] [CrossRef]
179. Lv, L., Yang, Q. Y., Zhao, Y., Yao, C. S., Sun, Y. et al. (2008). BACE1 (β-secretase) inhibitory chromone glycosides from Aloe vera and aloe nobilis. Planta Medica, 74(5), 540–545. DOI 10.1055/s-2008-1074496. [Google Scholar] [CrossRef]
180. Das, B., Yan, R. (2017). Role of BACE1 in Alzheimer’s synaptic function. Translational Neurodegeneration, 6(1), 1–8. DOI 10.1186/s40035-017-0093-5. [Google Scholar] [CrossRef]
181. Hampel, H., Vassar, R., de Strooper, B., Hardy, J., Willem, M. et al. (2021). The β-secretase BACE1 in Alzheimer’s disease. Biological Psychiatry, 89(8), 745–756. DOI 10.1016/j.biopsych.2020.02.001. [Google Scholar] [CrossRef]
Cite This Article
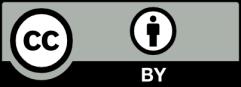