Open Access
REVIEW
Circular RNAs in breast cancer diagnosis, treatment and prognosis
1 Department of Breast Oncology Surgery, Affiliated Cancer Hospital & Institute of Guangzhou Medical University, Guangzhou, 510095, China
2 State Key Laboratory of Oncology in South China, Guangdong Provincial Clinical Research Center for Cancer, Sun Yat-sen University Cancer Center, Guangzhou, 510060, China
3 Department of Breast Surgery, The First People’s Hospital of Foshan, Foshan, 528000, China
* Corresponding Authors: LEWEI ZHU. Email: ; HAILIN TANG. Email:
# These authors contributed equally to this work
Oncology Research 2024, 32(2), 241-249. https://doi.org/10.32604/or.2023.046582
Received 08 October 2023; Accepted 06 November 2023; Issue published 28 December 2023
Abstract
Breast cancer has surpassed lung cancer to become the most common malignancy worldwide. The incidence rate and mortality rate of breast cancer continue to rise, which leads to a great burden on public health. Circular RNAs (circRNAs), a new class of noncoding RNAs (ncRNAs), have been recognized as important oncogenes or suppressors in regulating cancer initiation and progression. In breast cancer, circRNAs have significant roles in tumorigenesis, recurrence and multidrug resistance that are mediated by various mechanisms. Therefore, circRNAs may serve as promising targets of therapeutic strategies for breast cancer management. This study reviews the most recent studies about the biosynthesis and characteristics of circRNAs in diagnosis, treatment and prognosis evaluation, as well as the value of circRNAs in clinical applications as biomarkers or therapeutic targets in breast cancer. Understanding the mechanisms by which circRNAs function could help transform basic research into clinical applications and facilitate the development of novel circRNA-based therapeutic strategies for breast cancer treatment.Keywords
Abbreviations
circRNA | Circular RNA |
ncRNA | Noncoding RNA |
ceRNA | Competitive endogenous RNA |
TNBC | Triple-negative breast cancer |
ADM | Adriamycin |
PTX | Paclitaxel |
DDP | Cisplatin |
5-FU | 5-fluorouracil |
PFS | Progression-free survival |
OS | Overall survival |
DSS | Disease-specific survival |
Breast cancer has surpassed lung cancer to become the most common malignancy worldwide, and its burden has continued to increase during the last decade. From 2010–2019, the incidence rate of breast cancer rose annually by 0.5% [1]. In 2020, over 2.3 million breast cancer cases were diagnosed, and approximately 685,000 related deaths occurred. By 2040, it is predicted that over 3 million breast cancer cases will occur every year. In transitioning countries, the incidence rate and mortality rate of breast cancer remain high and increase annually [2].
Noncoding RNAs (ncRNAs) play important roles as oncogenes or tumor suppressors in regulating cancer occurrence and development [3,4]. Many studies have indicated that ncRNAs have significant roles in breast cancer progression; therefore, ncRNAs may serve as promising therapeutics in breast cancer management [5,6]. Circular RNAs (circRNAs), a novel type of ncRNA, are synthesized via the back splicing of protein-coding genes. CircRNAs are involved in multiple biological processes and diseases [7]. CircRNAs are highly stable due to their covalently closed circular structures, which protects them from degradation by exonucleases and makes them promising candidate biomarkers for cancer diagnosis or prognosis evaluation [8].
The expression and function of circRNAs vary in different cancers; they modulate gene expression and cancer progression via a series of mechanisms. The most studied mechanism is the competitive endogenous RNA (ceRNA) mechanism, in which circRNAs act as microRNA (miRNA) sponges to prevent the degradation of targeted mRNAs by miRNAs (Fig. 1A), which are a group of short, conserved RNAs that can regulate the expression of targeted genes by binding to the 3′-untranslated region [9]. For example, circ_0086722 could sponge miR-339-5p to reverse the suppression of STAT5A to promote the progression of prostate cancer [10]. On the other hand, circRNAs can regulate transcription factors to bind to the promoters of targeted genes to modulate the expression of targeted genes (Fig. 1B). Moreover, circRNAs can serve as scaffolds to modulate protein–protein interactions (Fig. 1C) [11]. Some circRNAs even have translational potential [12,13] (Fig. 1D).
Figure 1: The regulatory mechanisms of circRNAs. (A) circRNAs can sponge miRNAs to suppress the activity of miRNAs; (B) circRNAs can bind to transcription factors to regulate gene transcription; (C) circRNAs can serve as protein scaffolds to interact with RNA binding proteins to modulate protein–protein interactions; (D) circRNAs can be translated into proteins.
CircRNA dysregulation has been discussed in various malignancies, including breast cancer [14,15]. CircRNAs take part in the initiation and progression of breast cancer via a variety of mechanisms, acting as either oncogenes or tumor suppressors. The dysregulation of circRNAs in breast cancer is correlated with cell proliferation, apoptosis, autophagy, invasion, migration and treatment resistance. Here, we review the most recent studies on the biosynthesis and characteristics of circRNAs, the roles of circRNA in diagnosis, treatment and prognosis evaluation, and the value of circRNAs in clinical application as biomarkers or therapeutic targets in breast cancer.
CircRNAs in breast cancer diagnosis
Cancer cells can release circRNAs into serum, saliva or urine, and these circRNAs could be detected as diagnostic biomarkers or prognostic indicators [16]. Recently, the functions of circRNAs in breast cancer diagnosis have been investigated in a series of studies [17]. To identify relevant studies, we searched the NCBI PubMed database with the keywords “((circular RNA[Title/Abstract]) OR (circRNA[Title/Abstract])) AND ((plasma[Title/Abstract]) OR (serum[Title/Abstract]) OR (blood[Title/Abstract])) AND (“Breast Neoplasms”[MeSH Terms]) NOT review[Publication Type]”, and we obtained 19 studies, among which 17 studies were included in this review after manual selection. The blood circSMARCA5 level was shown to be decreased in breast cancer patients compared to normal controls, revealing the potential value of circSMARCA5 as a biomarker candidate for breast cancer [18]. Hsa_circ_0104824 was found to be downregulated in breast cancer peripheral blood samples, supporting the diagnostic function of hsa_circ_0104824 for patients with breast cancer [19]. Circ_0000977 is downregulated in triple-negative breast cancer (TNBC) plasma samples, indicating the potential diagnostic value of circ_0000977 in TNBC [20]. Hsa_circ_0008673 is significantly upregulated in breast cancer plasma samples, which represents a novel class of biomarkers with great diagnostic value [21]. CircRASSF2, hsa_circ_0069094, hsa_circ_0079876, hsa_circ_0017650 and hsa_circ_0017526 were all upregulated in breast cancer patients [22,23]. CircBCBM1 is overexpressed in breast cancer plasma samples and could function as a potential diagnostic biomarker [24]. The level of circPRMT5 in serum samples is elevated in breast cancer patients in comparison with healthy volunteers [25]. Circ_0076611 could be released from TNBC cells to exosomes and was detectable in breast cancer patient serum [26]. Circ0088088 and circ00000751 were found to be upregulated in serum exosomes of breast cancer patients compared with those of healthy controls, while circ00005795 was found to be downregulated [27]. Additionally, circHIF1A and circPSMA1 could be released into exosomes and were upregulated in the plasma of breast cancer patients [28,29]. Circ_0000745, circ_0001531 and circ_0001640 were all elevated in whole blood samples of breast cancer patients, revealing great value in the diagnosis of breast cancer [30]. The level of hsa_circ_0001785 in plasma was reported to have better diagnostic accuracy than that of CEA or CA15-3 in breast cancer [31] and may serve as a promising diagnostic biomarker. Overall, circRNAs are promising, stably expressed biomarkers for breast cancer diagnosis or progression prediction [32] (Table 1).
CircRNAs in breast cancer treatment
Breast cancer is a heterogeneous malignancy with various therapeutic approaches based on the particular molecular subtype; treatments include chemotherapy, radiotherapy, endocrine therapy, targeted therapy and immunotherapy [33]. These therapeutic strategies have significantly improved the clinical outcome of breast cancer patients. However, the development of resistance to treatment is a great obstacle that needs further investigation [34]. Moreover, predictive biomarkers for therapy response are important approaches to selecting ideal patient populations and treatment strategies [35]. Recently, ncRNAs have been applied as promising approaches in cancer treatment [36]. RNA-based therapeutics, including circRNA therapies, are currently under evaluation in many clinical trials [37–39]. In breast cancer, circRNAs participate in regulating crucial pathways related to treatment resistance.
Chemotherapy is one of the main therapeutic strategies for breast cancer. Chemoresistance is a great obstacle for breast cancer treatment. Understanding the mechanism of chemoresistance would help develop novel targets and coping strategies. CircRNAs take part in regulating chemoresistance in breast cancer [40] (Table 2). Thus, they may serve as biomarkers as well as therapeutic targets to restore the chemotherapy sensitivity of breast cancer [69,70]. Hsa_circ_0000199 is overexpressed in TNBC, and knockdown of hsa_circ_0000199 could induce chemosensitivity by modulating the levels of miR-206 and miR-613 to inhibit the PI3K/Akt/mTOR pathway [41]. Eighteen circRNAs were dysregulated in adriamycin (ADM)-resistant breast cancer, and hsa_circ_0006528 regulated resistance against ADM through the miR-7-5p-Raf1 axis [42]. Circ_0085495 is overexpressed in ADM-resistant breast cancer, and silencing circ_0085495 repressed ADM resistance by regulating miR-873-5p/integrin β1 signaling [43]. Circ_0044556 is upregulated in breast cancer and could promote cell resistance to ADM treatment by modulating miR‑145/NRAS signaling [44]. Inhibition of circ_0001667 in breast cancer could reduce ADM resistance through the miR-4458/NCOA3 axis [45]. CircATXN7 was found to be highly expressed in breast cancer, and overexpression of circATXN7 led to reduced breast cancer doxorubicin sensitivity by affecting miR-149-5p/HOXA11 signaling [46]. In doxorubicin-resistant TNBC cells, circ-CREIT was downregulated, while overexpression of circ-CREIT restored doxorubicin sensitivity in TNBC. Moreover, exosomes containing circ-CREIT could enhance the sensitivity to doxorubicin in TNBC. Mechanistically, circ-CREIT promoted PKR degradation through polyubiquitylation to activate the RACK1/MTK1 apoptosis pathway. Therefore, circ-CREIT might serve as a promising therapeutic strategy for chemoresistance in TNBC [47]. The level of circKDM4C is decreased in breast cancer, while overexpression of circKDM4C could suppress doxorubicin resistance via the miR-548p/PBLD axis [48]. In paclitaxel (PTX)-resistant breast cancer, circ_0069094 is highly expressed, and inhibiting circ_0069094 could restore sensitivity to PTX in breast cancer with PTX resistance by regulating the miR-136-5p/YWHAZ axis [49]. CircAMOTL1 could enhance breast cancer PTX resistance by regulating AKT signaling [50]. Overexpression of circDUSP1 could restore sensitivity to PTX in TNBC through acting as a decoy of miR-76 and increasing the level of DACT2 in TNBC [51]. Overexpression of circSMARCA5 in breast cancer cells could induce sensitivity to the cytotoxic drugs cisplatin (DDP) or bleomycin. Mechanistically, CircSMARCA5 suppressed SMARCA5 transcription, leading to decreased expression of SMARCA5 and enhanced sensitivity to chemotherapy [18]. CircUBAP2 is elevated in DDP-resistant TNBC and could promote resistance to DDP by regulating miR-300/ASF1B signaling as well as the PI3K/AKT/mTOR axis in TNBC [52]. CircFAT1 induced oxaliplatin resistance in breast cancer by modulating the miR-525-5p/SKA1 axis as well as Notch and Wnt signaling [53]. CircCDR1as is highly expressed in 5-fluorouracil (5-FU)-resistant breast cancer and can regulate the chemosensitivity of 5-FU by modulating the miR-7/CCNE1 pathway [54]. Collectively, the above studies indicated that circRNAs might act as promising clinical biomarkers and treatment targets in breast cancer patients with chemoresistance.
Radiotherapy is a standard treatment for breast cancer patients. However, radiation resistance could lead to relapse after treatment. Investigating the underlying mechanisms of breast cancer radiation resistance may help improve the outcome of patients [71]. The capacities of circRNAs to modulate breast cancer radiosensitivity have been discussed in a few studies. Overexpression of circNCOR1 reduced breast cancer radiosensitivity to promote breast cancer growth by acting as a decoy of hsa-miR-638 to regulate the level of CDK2 [55]. Circ-ABCC1 is upregulated in radio-resistant breast cancer and could induce radioresistance through acting as a decoy of miR-627-5p to increase the level of ABCC1 [56]. Circ-ADAM9 is upregulated in breast cancer, and inhibition of circ-ADAM9 could induce breast cancer radiosensitivity and apoptosis by acting as a decoy of miR-383-5p to regulate the level of PFN2 [57]. The above studies indicated that circRNAs have important functions in radiation resistance in breast cancer. However, further explorations are necessary to determine the mechanism and potential clinical value of circRNAs in breast cancer radiotherapy.
Endocrine therapy is a basic therapeutic strategy for ERα-positive breast cancer. However, resistance to endocrine therapy is a great obstacle in breast cancer treatment, as approximately 20%–50% of patients develop resistance to endocrine therapy [72]. Increasing studies have claimed that circRNAs have important functions in endocrine resistance and that they are considered predictive biomarkers to monitor the response to endocrine therapy. Moreover, they have great potential as therapeutic targets to overcome resistance to endocrine therapy [73]. CircPVT1 is elevated in ERα-positive breast cancer and could promote resistance to endocrine therapy by sponging miR-181a-2-3p to stabilize the expression of ESR1 and promote the activity of estrogen/ERα target genes, while targeting circPVT1 could resensitize breast cancer cells to tamoxifen treatment [58]. Circ-SFMBT2 is a crucial regulator of the ERα pathway, and overexpression of circ-SFMBT2 can induce tamoxifen resistance by enhancing ERα stability. Mechanistically, circ-SFMBT2 promotes the recruitment of RNF181 to ERα to suppress ERα ubiquitination, leading to enhanced ERα stability and elevated expression of ERα target genes. Inhibiting circ-SFMBT2 might be a potential therapeutic strategy in ER-positive breast cancer [59]. CircTRIM28 is overexpressed in breast cancer. Silencing circTRIM28 could enhance breast cancer tamoxifen sensitivity by acting as a decoy of miR-409-3p to increase the level of HMGA2 [60]. CircCDK1 is upregulated in tamoxifen-resistant breast cancer. Knockdown of circCDK1 could restore breast cancer sensitivity to tamoxifen by modulating miR-489-3p/CDK1 signaling [61]. Circ_UBE2D2 is upregulated in tamoxifen-resistant breast cancer, and circ_UBE2D2 could induce breast cancer tamoxifen resistance by regulating miR-200a-3p [62]. Hsa_circ_0025202 levels are decreased in breast cancer vs. control samples, and overexpression of hsa_circ_0025202 could enhance breast cancer tamoxifen sensitivity by modulating miR-182-5p/FOXO3a signaling [63]. Taken together, the above results indicate that circRNAs are crucial players in endocrine therapy of breast cancer and could serve as promising biomarkers as well as targets for ERα-positive breast cancer.
Great developments in targeted therapies have occurred in recent decades, and the number of treatment options for breast cancer patients has increased significantly [74,75]. For HER2-positive breast cancer, trastuzumab is the main molecularly targeted agent. However, approximately 25% of patients develop resistance to trastuzumab, indicated by rapid recurrence. The clinical efficacy could be improved by overcoming drug resistance. CircRNAs have been reported to be correlated with breast cancer targeted therapy resistance. CircCDYL2 is a promising biomarker for trastuzumab resistance and has vital functions in resistance against trastuzumab, and patients with elevated levels of circCDYL2 exhibit rapid recurrence. Mechanistically, circCDYL2 prevents GRB7 degradation to promote GRB7 interaction with FAK and sustains AKT and ERK1/2 activities to sustain the HER2 pathway [64]. Circ-BGN is overexpressed in breast cancer and contributes to trastuzumab resistance by suppressing cell ferroptosis through inducing deubiquitination of SLC7A11 mediated by OTUB1, leading to poor OS. Inhibiting circ-BGN could restore sensitivity against trastuzumab in breast cancer cells [65]. Circ_0001598 levels were elevated in breast cancer and modulated trastuzumab resistance in HER2-positive breast cancer by regulating the miR-1184/PD-L1 axis [66]. Circ-MMP11 is upregulated in lapatinib-resistant breast cancer. Overexpression of circ-MMP11 could induce resistance against lapatinib in breast cancer through acting as a decoy of miR-153-3p and regulating the level of ANLN [67]. The above findings showed that circRNAs play key roles in breast cancer resistance to targeted therapy, and further exploration is warranted to transform these findings to clinical application for breast cancer.
Recently, increasing attention has been given to breast cancer immunotherapy, which could help improve the response of breast cancer to other therapies [76,77]. However, not every patient can benefit from immunotherapy. Therefore, predictive biomarkers are needed to better select patients who may respond to immunotherapy [78]. CircRNAs have great capacities in immune evasion of a series of cancers, including breast cancer. However, the potential mechanisms of circRNAs in the immunotherapy resistance of breast cancer are mostly unclear and need to be further explored. CircFGFR4 is overexpressed in TNBC. CircFGFR4 acts as a decoy of miR-185-5p and increases the expression of CXCR4, so overexpression of circFGFR4 led to reduced infiltration of CD8+ T cells and enhanced resistance against anti-PD-1 immunotherapy in TNBC [68]. The circHIPK3 level is elevated and associated with a lower immunotherapy response in breast cancer [79]. The above findings indicate that circRNAs have great potential as predictive biomarkers for immunotherapy response as well as immunotherapeutic targets in breast cancer.
CircRNAs in breast cancer prognosis
CircRNAs have been identified as promising prognostic biomarkers in breast cancer [80,81]. The increased circCDYL level in breast cancer serum and tissue is associated with shorter survival, indicating the possibility of circCDYL as a predictive biomarker for breast cancer prognosis [82]. Hsa_circ_0067842 is elevated in breast cancer and is related to worse breast cancer outcomes [83]. The hsa_circ_0001785 level in plasma is closely associated with breast cancer histological grade, TNM stage and distant metastasis, indicating the potential diagnostic and predictive capacity of hsa_circ_0001785 in breast cancer plasma [31]. CircCNOT2 is detectable in plasma and is correlated with poor progression-free survival (PFS) in advanced breast cancer patients with aromatase inhibitor (AI) therapy [84]. Plasma hsa_circ_0008673 levels are related to shorter overall survival (OS) and disease-specific survival (DSS) in breast cancer, indicating that it acts as a promising predicator for breast cancer prognosis evaluation [21]. The serum circRASSF2 level is associated with worse OS and PFS in breast cancer patients [22]. A high level of circBCBM1 in breast cancer is related to poorer brain metastasis-free survival, so circBCBM1 could act as a promising prognostic biomarker in breast cancer [24]. Collectively, the above studies indicated that circRNAs may act as promising prognostic biomarkers for breast cancer patients (Table 3).
Recently, circRNA has become a hot topic in cancer research. CircRNA dysregulation has been discovered in a variety of malignancies, and circRNAs have important functions in tumorigenesis as well as cancer progression through diverse molecular mechanisms [85,86]. Recent studies have claimed that various circRNAs are related to breast cancer tumorigenesis, recurrence and multidrug resistance through various mechanisms [87–89]. In addition, circRNAs are crucial players in treatment resistance and are correlated with the clinicopathological characteristics of breast cancer patients. Here, we summarized recent studies on the characteristics and roles of circRNAs in breast cancer.
An increasing number of studies have indicated that circRNAs could act as promising diagnostic and prognostic biomarkers. Indeed, tissue-specific expression and resistance to exonucleases make circRNAs an attractive new class of noninvasive biomarkers for clinical application [90]. However, these studies have some limitations, such as inconsistency and nonreproducibility. Most of the studies published are based on breast cancer cell lines or tissue samples from rather small populations, and most lack validation in larger independent cohorts. Therefore, a larger number of samples are needed to confirm the results. Due to sample heterogeneity, differences in detection methods and a lack of proper validation techniques, most assessed circRNAs are not sensitive or specific enough to be used as clinical biomarkers. Currently, the prospective value of circRNAs as biomarkers is under investigation in clinical trials in a series of human malignancies, including breast cancer. For instance, serum hsa_circ_0001785 and hsa_circ_100219 have been investigated as promising diagnostic or prognostic biomarkers in breast cancer (NCT05771337).
In spite of the advances in breast cancer treatment strategies, development of resistance to these therapeutics is still an obstacle in clinical setting [91,92]. The roles and underlying mechanisms of circRNAs in predicting breast cancer treatment responsiveness have been investigated [93]. However, most of the studies have focused on chemotherapy and endocrine therapy, and there are still not enough studies covering the roles and possible mechanisms of circRNAs in radiotherapy, immunotherapy or targeted therapy in breast cancer, which is worth further exploration. In addition, there is still no circRNA drug that can be used in clinical settings due to low specificity and instability. More investigations are needed to promote the application of circRNAs as targetable molecules in future clinical treatment.
In conclusion, circRNAs have important functions in the initiation and progression of breast cancer. Understanding the mechanisms by which circRNAs function would aid in the development of novel circRNA-based therapeutic strategies for breast cancer treatment. Further explorations, especially high-quality animal studies and large-scale clinical trials, are needed to transform basic research into clinical applications.
Acknowledgement: Not applicable.
Funding Statement: This work was supported by the Basic and Applied Basic Research Foundation of Guangdong Province (2022A1515220184).
Author Contributions: The authors confirm contribution to the paper as follows: study conception and design: L. Zhu, H. Tang; data collection: C. Song, X. Huang; analysis and interpretation of results: J. Zhang, X. Huang; draft manuscript preparation: X. Huang. All authors reviewed the results and approved the final version of the manuscript.
Availability of Data and Materials: Data will be made available on request.
Ethics Approval: Not applicable.
Conflicts of Interest: The authors declare that they have no conflicts of interest to report regarding the present study.
References
1. Giaquinto, A. N., Sung, H., Miller, K. D., Kramer, J. L., Newman, L. A. et al. (2022). Breast cancer statistics, 2022. CA: A Cancer Journal for Clinicians, 72(6), 524–541. [Google Scholar] [PubMed]
2. Arnold, M., Morgan, E., Rumgay, H., Mafra, A., Singh, D. et al. (2022). Current and future burden of breast cancer: Global statistics for 2020 and 2040. Breast, 66, 15–23. [Google Scholar] [PubMed]
3. Yan, H., Bu, P. (2021). Non-coding RNA in cancer. Essays in Biochemistry, 65(4), 625–639. [Google Scholar] [PubMed]
4. Zhao, M., Wang, Y., Tan, F., Liu, L., Hou, X. et al. (2022). Circular RNA circCCNB1 inhibits the migration and invasion of nasopharyngeal carcinoma through binding and stabilizing TJP1 mRNA. Science China-Life Sciences, 65(11), 2233–2247. [Google Scholar] [PubMed]
5. Huang, X., Tan, W., Liu, Z., Fu, X., Li, Z. et al. (2023). EIF4A3-induced circZFAND6 promotes breast cancer proliferation and metastasis through the miR-647/FASN axis. Life Sciences, 324, 121745. [Google Scholar] [PubMed]
6. Huang, X., Wu, J., Wang, Y., Xian, Z., Li, J. et al. (2023). FOXQ1 inhibits breast cancer ferroptosis and progression via the circ_0000643/miR-153/SLC7A11 axis. Experimental Cell Research, 431(1), 113737. [Google Scholar] [PubMed]
7. Zhou, Q., Boeckel, J. N., Yao, J., Zhao, J., Bai, Y. et al. (2023). Diagnosis of acute myocardial infarction using a combination of circulating circular RNA cZNF292 and clinical information based on machine learning. MedComm, 4(3), e299. [Google Scholar] [PubMed]
8. Wu, S., Wu, Y., Deng, S., Lei, X., Yang, X. (2023). Emerging roles of noncoding RNAs in human cancers. Discover Oncology, 14(1), 128. [Google Scholar] [PubMed]
9. Gao, Z., Jiang, J., Hou, L., Zhang, B. (2022). Dysregulation of MiR-144-5p/RNF187 axis contributes to the progression of colorectal cancer. Journal of Translational Internal Medicine, 10(1), 65–75. [Google Scholar] [PubMed]
10. Deng, W., Zhou, X., Zhu, K., Chen, R., Liu, X. et al. (2022). Novel circular RNA circ_0086722 drives tumor progression by regulating the miR-339-5p/STAT5A axis in prostate cancer. Cancer Letters, 533, 215606. [Google Scholar] [PubMed]
11. Wang, L., Yu, P., Wang, J., Xu, G., Wang, T. et al. (2022). Downregulation of circ-ZNF609 promotes heart repair by modulating RNA N(6)-methyladenosine-modified yap expression. Research, 2022, 9825916. [Google Scholar] [PubMed]
12. Li, J., Sun, D., Pu, W., Wang, J., Peng, Y. (2022). Circular RNAs in cancer: Biogenesis, function, and clinical significance. Trends Cancer, 6(4), 319–336, 2020. [Google Scholar]
13. Wen, S. Y., Qadir, J., Yang, B. B. (2022). Circular RNA translation: Novel protein isoforms and clinical significance. Trends in Molecular Medicine, 28(5), 405–420. [Google Scholar] [PubMed]
14. Zeng, Y., Zou, Y., Gao, G., Zheng, S., Wu, S. et al. (2021). The biogenesis, function and clinical significance of circular RNAs in breast cancer. Cancer Biology & Medicine, 19(1), 14–29. [Google Scholar]
15. Kong, Y., Yang, L., Wei, W., Lyu, N., Zou, Y. et al. (2019). CircPLK1 sponges miR-296-5p to facilitate triple-negative breast cancer progression. Epigenomics, 11(10), 1163–1176. [Google Scholar] [PubMed]
16. Lei, B., Tian, Z., Fan, W., Ni, B. (2019). Circular RNA: A novel biomarker and therapeutic target for human cancers. International Journal of Medical Sciences, 16(2), 292–301. [Google Scholar] [PubMed]
17. Yi, Y., Wu, M., Zeng, H., Hu, W., Zhao, C. et al. (2021). Tumor-derived exosomal non-coding RNAs: The emerging mechanisms and potential clinical applications in breast cancer. Frontiers in Oncology, 11, 738945. [Google Scholar] [PubMed]
18. Xu, X., Zhang, J., Tian, Y., Gao, Y., Dong, X. et al. (2020). CircRNA inhibits DNA damage repair by interacting with host gene. Molecular Cancer, 19(1), 128. [Google Scholar] [PubMed]
19. Li, X., Ma, F., Wu, L., Zhang, X., Tian, J. et al. (2020). Identification of hsa_circ_0104824 as a potential biomarkers for breast cancer. Technology in Cancer Research & Treatment, 19. [Google Scholar]
20. Darbeheshti, F., Mansoori, Y., Azizi-Tabesh, G., Zolfaghari, F., Kadkhoda, S. et al. (2023). Evaluation of circ_0000977-mediated regulatory network in breast cancer: A potential discriminative biomarker for triple-negative tumors. Biochemical Genetics, 61(4), 1487–1508. [Google Scholar] [PubMed]
21. Hu, Y., Song, Q., Zhao, J., Ruan, J., He, F. et al. (2020). Identification of plasma hsa_circ_0008673 expression as a potential biomarker and tumor regulator of breast cancer. Journal of Clinical Laboratory Analysis, 34(9), e23393. [Google Scholar] [PubMed]
22. Zhong, W., Bao, L., Yuan, Y., Meng, Y. (2021). CircRASSF2 acts as a prognostic factor and promotes breast cancer progression by modulating miR-1205/HOXA1 axis. Bioengineered, 12(1), 3014–3028. [Google Scholar] [PubMed]
23. Li, Z., Chen, Z., Hu, G., Zhang, Y., Feng, Y. et al. (2020). Profiling and integrated analysis of differentially expressed circRNAs as novel biomarkers for breast cancer. Journal of Cellular Physiology, 235(11), 7945–7959. [Google Scholar] [PubMed]
24. Fu, B., Liu, W., Zhu, C., Li, P., Wang, L. et al. (2021). Circular RNA circBCBM1 promotes breast cancer brain metastasis by modulating miR-125a/BRD4 axis. International Journal of Biological Sciences, 17(12), 3104–3117. [Google Scholar] [PubMed]
25. Li, X., Zhang, D., Feng, Z., Xu, X., Zhang, J. et al. (2022). Circular RNA circPRMT5 is upregulated in breast cancer and is required for cell proliferation and migration. Turkish Journal of Medical Sciences, 52(2), 303–312. [Google Scholar] [PubMed]
26. Turco, C., Esposito, G., Iaiza, A., Goeman, F., Benedetti, A. et al. (2022). MALAT1-dependent hsa_circ_0076611 regulates translation rate in triple-negative breast cancer. Communications Biology, 5(1), 598. [Google Scholar] [PubMed]
27. Yang, S. J., Wang, D. D., Zhou, S. Y., Zhang, Q., Wang, J. Y. et al. (2020). Identification of circRNA-miRNA networks for exploring an underlying prognosis strategy for breast cancer. Epigenomics, 12(2), 101–125. [Google Scholar] [PubMed]
28. Chen, T., Wang, X., Li, C., Zhang, H., Liu, Y. et al. (2021). CircHIF1A regulated by FUS accelerates triple-negative breast cancer progression by modulating NFIB expression and translocation. Oncogene, 40(15), 2756–2771. [Google Scholar] [PubMed]
29. Yang, S. J., Wang, D. D., Zhong, S. L., Chen, W. Q., Wang, F. L. et al. (2021). Tumor-derived exosomal circPSMA1 facilitates the tumorigenesis, metastasis, and migration in triple-negative breast cancer (TNBC) through miR-637/Akt1/β-catenin (cyclin D1) axis. Cell Death and Disease, 12(5), 420. [Google Scholar] [PubMed]
30. Wang, Y. W., Xu, Y., Wang, Y. Y., Zhu, J., Gao, H. D. et al. (2021). Elevated circRNAs circ_0000745, circ_0001531 and circ_0001640 in human whole blood: Potential novel diagnostic biomarkers for breast cancer. Experimental and Molecular Pathology, 121, 104661. [Google Scholar] [PubMed]
31. Yin, W. B., Yan, M. G., Fang, X., Guo, J. J., Xiong, W. et al. (2018). Circulating circular RNA hsa_circ_0001785 acts as a diagnostic biomarker for breast cancer detection. Clinica Chimica Acta, 487, 363–368. [Google Scholar]
32. Li, Z., Chen, Z., Hu, G., Jiang, Y. (2019). Roles of circular RNA in breast cancer: Present and future. American Journal of Translational Research, 11(7), 3945–3954. [Google Scholar] [PubMed]
33. Sarhangi, N., Hajjari, S., Heydari, S. F., Ganjizadeh, M., Rouhollah, F. et al. (2022). Breast cancer in the era of precision medicine. Molecular Biology Reports, 49(10), 10023–10037. [Google Scholar] [PubMed]
34. Dou, L., Lu, E., Tian, D., Li, F., Deng, L. et al. (2023). Adrenomedullin induces cisplatin chemoresistance in ovarian cancer through reprogramming of glucose metabolism. Journal of Translational Internal Medicine, 11(2), 169–177. [Google Scholar] [PubMed]
35. Rodrigues-Ferreira, S., Nahmias, C. (2022). Predictive biomarkers for personalized medicine in breast cancer. Cancer Letters, 545, 215828. [Google Scholar] [PubMed]
36. Liu, J., Zhao, G., Liu, X. L., Zhang, G., Zhao, S. Q. et al. (2021). Progress of non-coding RNAs in triple-negative breast cancer. Life Sciences, 272, 119238. [Google Scholar] [PubMed]
37. Zhang, C., Zhang, B. (2023). RNA therapeutics: Updates and future potential. Science China-Life Sciences, 66(1), 12–30. [Google Scholar] [PubMed]
38. Su, P., Zhang, L., Zhou, F., Zhang, L. (2022). Circular RNA vaccine, a novel mRNA vaccine design strategy for SARS-CoV-2 and variants. MedComm, 3(3), e153. [Google Scholar] [PubMed]
39. Li, M., Wang, Y., Wu, P., Zhang, S., Gong, Z. et al. (2023). Application prospect of circular RNA-based neoantigen vaccine in tumor immunotherapy. Cancer Letters, 563, 216190. [Google Scholar] [PubMed]
40. Ghazimoradi, M. H., Babashah, S. (2022). The role of circRNA/miRNA/mRNA axis in breast cancer drug resistance. Frontiers in Oncology, 12, 966083. [Google Scholar] [PubMed]
41. Li, H., Xu, W., Xia, Z., Liu, W., Pan, G. et al. (2021). Hsa_circ_0000199 facilitates chemo-tolerance of triple-negative breast cancer by interfering with miR-206/613-led PI3K/Akt/mTOR signaling. Aging, 13(3), 4522–4551. [Google Scholar] [PubMed]
42. Gao, D., Zhang, X., Liu, B., Meng, D., Fang, K. et al. (2017). Screening circular RNA related to chemotherapeutic resistance in breast cancer. Epigenomics, 9(9), 1175–1188. [Google Scholar] [PubMed]
43. Xie, H., Zheng, R. (2022). Circ_0085495 knockdown reduces adriamycin resistance in breast cancer through miR-873-5p/integrin β1 axis. Anticancer Drugs, 33(1), e166–e177. [Google Scholar] [PubMed]
44. Chen, J., Shi, P., Zhang, J., Li, Y., Ma, J. et al. (2022). CircRNA_0044556 diminishes the sensitivity of triple‐negative breast cancer cells to adriamycin by sponging miR‐145 and regulating NRAS. Molecular Medicine Reports, 25(2), 51. [Google Scholar] [PubMed]
45. Cui, Y., Fan, J., Shi, W., Zhou, Z. (2022). Circ_0001667 knockdown blocks cancer progression and attenuates adriamycin resistance by depleting NCOA3 via releasing miR-4458 in breast cancer. Drug Development Research, 83(1), 75–87. [Google Scholar] [PubMed]
46. Wang, H., Shan, S., Wang, H., Wang, X. (2022). CircATXN7 contributes to the progression and doxorubicin resistance of breast cancer via modulating miR-149-5p/HOXA11 pathway. Anticancer Drugs, 33(1), e700–e710. [Google Scholar] [PubMed]
47. Wang, X., Chen, T., Li, C., Li, W., Zhou, X. et al. (2022). CircRNA-CREIT inhibits stress granule assembly and overcomes doxorubicin resistance in TNBC by destabilizing PKR. Journal of Hematology & Oncology, 15(1), 122. [Google Scholar]
48. Liang, Y., Song, X., Li, Y., Su, P., Han, D. et al. (2019). circKDM4C suppresses tumor progression and attenuates doxorubicin resistance by regulating miR-548p/PBLD axis in breast cancer. Oncogene, 38(42), 6850–6866. [Google Scholar] [PubMed]
49. Kong, Z., Han, Q., Zhu, B., Wan, L., Feng, E. (2023). Circ_0069094 regulates malignant phenotype and paclitaxel resistance in breast cancer cells via targeting the miR-136-5p/YWHAZ axis. Thoracic Cancer, 14(19), 1831–1842. [Google Scholar] [PubMed]
50. Ma, J., Fang, L., Yang, Q., Hibberd, S., Du, W. W. et al. (2019). Posttranscriptional regulation of AKT by circular RNA angiomotin-like 1 mediates chemoresistance against paclitaxel in breast cancer cells. Aging, 11(23), 11369–11381. [Google Scholar] [PubMed]
51. Huang, S., Xie, J., Lei, S., Fan, P., Zhang, C. et al. (2023). CircDUSP1 regulates tumor growth, metastasis, and paclitaxel sensitivity in triple-negative breast cancer by targeting miR-761/DACT2 signaling axis. Molecular Carcinogenesis, 62(4), 450–463. [Google Scholar] [PubMed]
52. Wang, L., Yang, X., Zhou, F., Sun, X., Li, S. (2022). Circular RNA UBAP2 facilitates the cisplatin resistance of triple-negative breast cancer via microRNA-300/anti-silencing function 1B histone chaperone/PI3K/AKT/mTOR axis. Bioengineered, 13(3), 7197–7208. [Google Scholar] [PubMed]
53. Yao, Y., Li, X., Cheng, L., Wu, X., Wu, B. (2021). Circular RNA FAT atypical cadherin 1 (circFAT1)/microRNA-525-5p/spindle and kinetochore-associated complex subunit 1 (SKA1) axis regulates oxaliplatin resistance in breast cancer by activating the notch and Wnt signaling pathway. Bioengineered, 12(1), 4032–4043. [Google Scholar] [PubMed]
54. Yang, W., Gu, J., Wang, X., Wang, Y., Feng, M. et al. (2019). Inhibition of circular RNA CDR1as increases chemosensitivity of 5-FU-resistant BC cells through up-regulating miR-7. Journal of Cellular And Molecular Medicine, 23(5), 3166–3177. [Google Scholar] [PubMed]
55. He, Z. Y., Zhuo, R. G., Yang, S. P., Zhou, P., Xu, J. Y. et al. (2023). CircNCOR1 regulates breast cancer radiotherapy efficacy by regulating CDK2 via hsa-miR-638 binding. Cellular Signalling, 109, 110787. [Google Scholar] [PubMed]
56. Zhang, C., Wang, J., Wang, H., Li, J. (2022). Circ-ABCC1 enhances radioresistance of breast cancer cells via miR-627-5p/ABCC1 axis. Cellular and Molecular Biology, 68(10), 187–192. [Google Scholar] [PubMed]
57. Song, P., Wu, J., Chen, J., Wang, F., Chen, J. et al. (2023). Knockdown of circ-ADAM9 inhibits malignant phenotype and enhances radiosensitivity in breast cancer cells via acting as a sponge for miR-383-5p. Strahlentherapie und Onkologie, 199(1), 78–89. [Google Scholar] [PubMed]
58. Yi, J., Wang, L., Hu, G. S., Zhang, Y. Y., Du, J. et al. (2023). CircPVT1 promotes ER-positive breast tumorigenesis and drug resistance by targeting ESR1 and MAVS. The EMBO Journal, 42(10), e112408. [Google Scholar] [PubMed]
59. Li, Z., Li, Y., Han, D., Wang, X., Li, C. et al. (2023). circRNA-SFMBT2 orchestrates ERα activation to drive tamoxifen resistance in breast cancer cells. Cell Death and Disease, 14(7), 482. [Google Scholar] [PubMed]
60. Yang, S., Zou, C., Li, Y., Yang, X., Liu, W. et al. (2022). Knockdown circTRIM28 enhances tamoxifen sensitivity via the miR-409-3p/HMGA2 axis in breast cancer. Reproductive Biology and Endocrinology, 20(1), 146. [Google Scholar] [PubMed]
61. Liu, D., Zhou, Z., Guo, Y., Du, Q., Li, L. (2022). CircCDK1 knockdown reduces CDK1 expression by targeting miR-489-3p to suppress the development of breast cancer and strengthen the sensitivity of Tamoxifen. Anticancer Drugs, 33(3), 286–299. [Google Scholar] [PubMed]
62. Hu, K., Liu, X., Li, Y., Li, Q., Xu, Y. et al. (2020). Exosomes mediated transfer of circ_UBE2D2 enhances the resistance of breast cancer to tamoxifen by binding to MiR-200a-3p. Medical Science Monitor, 26, e922253. [Google Scholar] [PubMed]
63. Sang, Y., Chen, B., Song, X., Li, Y., Liang, Y. et al. (2019). circRNA_0025202 regulates tamoxifen sensitivity and tumor progression via regulating the miR-182-5p/FOXO3a axis in breast cancer. Molecular Therapy, 27(9), 1638–1652. [Google Scholar] [PubMed]
64. Ling, Y., Liang, G., Lin, Q., Fang, X., Luo, Q. et al. (2022). circCDYL2 promotes trastuzumab resistance via sustaining HER2 downstream signaling in breast cancer. Molecular Cancer, 21(1), 8. [Google Scholar] [PubMed]
65. Wang, S., Wang, Y., Li, Q., Li, X., Feng, X. (2022). A novel circular RNA confers trastuzumab resistance in human epidermal growth factor receptor 2-positive breast cancer through regulating ferroptosis. Environmental Toxicology, 37(7), 1597–1607. [Google Scholar] [PubMed]
66. Huang, L., Ma, J., Cui, M. (2021). Circular RNA hsa_circ_0001598 promotes programmed death-ligand-1-mediated immune escape and trastuzumab resistance via sponging miR-1184 in breast cancer cells. Immunologic Research, 69(6), 558–567. [Google Scholar] [PubMed]
67. Wu, X., Ren, Y., Yao, R., Zhou, L., Fan, R. (2021). Circular RNA circ-MMP11 contributes to lapatinib resistance of breast cancer cells by regulating the miR-153-3p/ANLN axis. Frontiers in Oncology, 11, 639961. [Google Scholar] [PubMed]
68. Wang, F., Lu, Q., Yu, H., Zhang, X. M. (2023). The circular RNA circFGFR4 facilitates resistance to anti-PD-1 of triple-negative breast cancer by targeting the miR-185-5p/CXCR4 axis. Cancer Management and Research, 15, 825–835. [Google Scholar] [PubMed]
69. Wang, H., Shan, X., Peng, Y., Zhou, W. (2023). Circular RNAs in the chemoresistance of triple-negative breast cancer: A systematic review. Drug Development Research, 84(5), 805–814. [Google Scholar] [PubMed]
70. Zhu, Z., Jiang, H., Xie, J., Jin, X., Li, B. et al. (2022). Current evidence on circRNAs as potential theranostic markers for detecting chemoresistance in breast cancer: A systematic review and meta‐analysis. Scientific Reports, 12(1), 22016. [Google Scholar] [PubMed]
71. Inalegwu, A., Cuypers, B., Claesen, J., Janssen, A., Coolkens, A. et al. (2022). Fractionated irradiation of MCF7 breast cancer cells rewires a gene regulatory circuit towards a treatment-resistant stemness phenotype. Molecular Oncology, 16(19), 3410–3435. [Google Scholar] [PubMed]
72. Miranda, F., Prazeres, H., Mendes, F., Martins, D., Schmitt, F. (2022). Resistance to endocrine therapy in HR + and/or HER2 + breast cancer: The most promising predictive biomarkers. Molecular Biology Reports, 49(1), 717–733. [Google Scholar] [PubMed]
73. Treeck, O., Haerteis, S., Ortmann, O. (2023). Non-coding RNAs modulating estrogen signaling and response to endocrine therapy in breast cancer. Cancers, 15(6), 1632. [Google Scholar] [PubMed]
74. Jacobs, A. T., Martinez Castaneda-Cruz, D., Rose, M. M., Connelly, L. (2022). Targeted therapy for breast cancer: An overview of drug classes and outcomes. Biochemical Pharmacology, 204, 115209. [Google Scholar] [PubMed]
75. Lau, K. H., Tan, A. M., Shi, Y. (2022). New and emerging targeted therapies for advanced breast cancer. International Journal of Molecular Sciences, 23(4), 2288. [Google Scholar] [PubMed]
76. Cortes, J., Cescon, D. W., Rugo, H. S., Nowecki, Z., Im, S. A. et al. (2020). Pembrolizumab plus chemotherapy versus placebo plus chemotherapy for previously untreated locally recurrent inoperable or metastatic triple-negative breast cancer (KEYNOTE-355A randomised, placebo-controlled, double-blind, phase 3 clinical trial. Lancet, 396(10265), 1817–1828. [Google Scholar] [PubMed]
77. Schmid, P., Rugo, H. S., Adams, S., Schneeweiss, A., Barrios, C. H. et al. (2020). Atezolizumab plus nab-paclitaxel as first-line treatment for unresectable, locally advanced or metastatic triple-negative breast cancer (IMpassion130Updated efficacy results from a randomised, double-blind, placebo-controlled, phase 3 trial. Lancet Oncology, 21(1), 44–59. [Google Scholar] [PubMed]
78. Yin, Y., Yan, Y., Fan, B., Huang, W., Zhang, J. et al. (2023). Novel combination therapy for triple-negative breast cancer based on an intelligent hollow carbon sphere. Research, 6, 0098. [Google Scholar] [PubMed]
79. Lian, Q. X., Song, Y., Han, L., Wang, Z., Song, Y. (2023). Development of a circHIPK3-based ceRNA network and identification of mRNA signature in breast cancer patients harboring BRCA mutation. PeerJ, 11, e15572. [Google Scholar] [PubMed]
80. Chen, B., Wei, W., Huang, X., Xie, X., Kong, Y. et al. (2018). circEPSTI1 as a prognostic marker and mediator of triple-negative breast cancer progression. Theranostics, 8(14), 4003–4015. [Google Scholar] [PubMed]
81. Tang, H., Huang, X., Wang, J., Yang, L., Kong, Y. et al. (2019). circKIF4A acts as a prognostic factor and mediator to regulate the progression of triple-negative breast cancer. Molecular Cancer, 18(1), 23. [Google Scholar] [PubMed]
82. Liang, G., Ling, Y., Mehrpour, M., Saw, P. E., Liu, Z. et al. (2020). Autophagy-associated circRNA circCDYL augments autophagy and promotes breast cancer progression. Molecular Cancer, 19(1), 65. [Google Scholar] [PubMed]
83. Li, J., Dong, X., Kong, X., Wang, Y., Li, Y. et al. (2023). Circular RNA hsa_circ_0067842 facilitates tumor metastasis and immune escape in breast cancer through HuR/CMTM6/PD-L1 axis. Biology Direct, 18(1), 48. [Google Scholar] [PubMed]
84. Smid, M., Wilting, S. M., Uhr, K., Rodriguez-Gonzalez, F. G., de Weerd, V. et al. (2019). The circular RNome of primary breast cancer. Genome Research, 29(3), 356–366. [Google Scholar] [PubMed]
85. Xiao, W., Zheng, S., Zou, Y., Yang, A., Xie, X. et al. (2019). CircAHNAK1 inhibits proliferation and metastasis of triple-negative breast cancer by modulating miR-421 and RASA1. Aging, 11(24), 12043–12056. [Google Scholar] [PubMed]
86. Zou, Y., Zheng, S., Xiao, W., Xie, X., Yang, A. et al. (2019). circRAD18 sponges miR-208a/3164 to promote triple-negative breast cancer progression through regulating IGF1 and FGF2 expression. Carcinogenesis, 40(12), 1469–1479. [Google Scholar] [PubMed]
87. Wang, Z., Yang, L., Wu, P., Li, X., Tang, Y. et al. (2022). The circROBO1/KLF5/FUS feedback loop regulates the liver metastasis of breast cancer by inhibiting the selective autophagy of afadin. Molecular Cancer, 21(1), 29. [Google Scholar] [PubMed]
88. Liu, P., Wang, Z., Ou, X., Wu, P., Zhang, Y. et al. (2022). The FUS/circEZH2/KLF5/feedback loop contributes to CXCR4-induced liver metastasis of breast cancer by enhancing epithelial-mesenchymal transition. Molecular Cancer, 21(1), 198. [Google Scholar] [PubMed]
89. Zeng, Y., Du, W., Huang, Z., Wu, S., Ou, X. et al. (2023). Hsa_circ_0060467 promotes breast cancer liver metastasis by complexing with eIF4A3 and sponging miR-1205. Cell Death Discovery, 9(1), 153. [Google Scholar] [PubMed]
90. Jahani, S., Nazeri, E., Majidzadeh, A. K., Jahani, M., Esmaeili, R. (2020). Circular RNA; a new biomarker for breast cancer: A systematic review. Journal of Cellular Physiology, 235(7–8), 5501–5510. [Google Scholar] [PubMed]
91. Bick, G., Zhang, J., Lower, E. E., Zhang, X. (2022). Transcriptional coactivator MED1 in the interface of anti-estrogen and anti-HER2 therapeutic resistance. Cancer Drug Resistance, 5(2), 498–510. [Google Scholar] [PubMed]
92. Apollonova, V., Plevako, D., Garanin, A., Sidina, E., Zabegina, L. et al. (2023). Resistance of breast cancer cells to paclitaxel is associated with low expressions of miRNA-186 and miRNA-7. Cancer Drug Resistance, 6(3), 596–610. [Google Scholar] [PubMed]
93. Wang, X., Fang, L. (2018). Advances in circular RNAs and their roles in breast Cancer. Journal of Experimental & Clinical Cancer Research, 37(1), 206. [Google Scholar]
Cite This Article
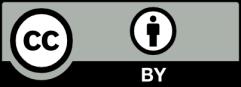