Open Access
ARTICLE
The Effects of Water and Fertilizer Coupling on Plant and Soil Nitrogen Characteristics and Fruit Growth of Rabbiteye Blueberry Plants in a Semi-Arid Region in China
1 College of Life Sciences, Huizhou University, Huizhou, 516007, China
2 College of Forestry, Guizhou University, Huaxi, 550025, China
3 School of Architecture and Civil Engineering, Huizhou University, Huizhou, 516007, China
4 Provincial Key Laboratory for Agricultural Pest Management of Mountainous Regions, Institute of Entomology, Guizhou University, Guiyang, 550025, China
* Corresponding Author: Delu Wang. Email:
Phyton-International Journal of Experimental Botany 2023, 92(1), 209-223. https://doi.org/10.32604/phyton.2022.023050
Received 07 April 2022; Accepted 26 May 2022; Issue published 06 September 2022
Abstract
To evaluate the effects of nitrogen (N) and irrigation coupling on the soil N distribution, plant N utilization, and fruit yield of rabbiteye blueberries (Vaccinium virgatum), a field experiment was designed using two factors (water and fertilizer application) with four levels of irrigation and three levels of fertilization, and a control. Under the different water and fertilizer combinations, N primarily accumulated in the leaves. Irrigation and N application within appropriate ranges (pure N ≤ 29 g/plant and irrigation volume ≤ 2.5 L/plant) significantly improved the blueberry fruit yield. Increases in water and N within these ranges promoted the effective accumulation of N in various organs and the absorption and utilization of N in the plants, which ultimately promoted blueberry yield. With increased N application rate, the nitrate N content of the 0–20 cm and 20–50 cm soil layers increased. With increased irrigation volume, the nitrate N content of the 0–20 cm soil layer decreased, while the nitrate content in the 20–50 cm soil layer increased. Low N and moderate water treatments resulted in high fruit yields and reduced nitrate N retention in the soil. Under these conditions, the economic input-output ratio was high and the soil N accumulation was low, and thus the economic and ecological benefits were maximized.
Keywords
Abbreviations
BNC | Branch nitrogen content |
WUE | Water use efficiency |
LNAU | Leaf nitrogen absorption and utilization |
LNC | Leaf nitrogen content |
NPP | Nitrogen partial productivity |
NUAU | Nitrogen uptake and utilization |
NUE | Nitrogen use efficiency |
RN | Residual nitrogen |
SD | Standard deviation |
AE | Agronomic utilization rate of N fertilizer |
The blueberry is an important economic fruit crop in China and has played a key role in increasing agricultural income. In 2020, the total blueberry cultivation area in China was 66,400 ha−1, with an output of 347,200 t. The blueberry industry has contributed significantly to poverty alleviation and commercial uplift in China, and the blueberry cultivation area in Guizhou in Southwest China is 15,000 hm2, with an output of 85,000 t [1]. The county of Majiang in Guizhou is an important blueberry production region, but water shortages and low fertilizer utilization rates hamper blueberry production [2]. Therefore, adopting appropriate irrigation and fertilization strategies could enhance water and nutrient use efficiency in blueberry cultivation.
Water shortages are a global issue, and the amount of water allocated for agricultural use is decreasing [3]. These shortages may prevent many fruit crops from obtaining sufficient water to meet their physiological requirements, especially in arid and semi-arid areas. In Southern China, blueberries are mainly cultivated in hilly and mountainous areas that are categorized by poor water availability and retention capacity, and are often threatened by local or intermittent droughts [4]. In addition, blueberries have shallow root systems, no root hairs, and are not tolerant to drought. Under limited summer precipitation, evaporation can cause varying degrees of soil water deficit [5]. Therefore, the efficient utilization of precipitation is key to improving the yield and water use efficiency (WUE) of blueberries in the region.
Fertilizer is another important factor that affects crop yield and nitrogen use efficiency (NUE) [6,7]. The application of nitrogen (N) fertilizer has an important impact on crop yield. Studies have shown that N application can increase crop yields [8]; however, excessive application of N fertilizer may reduce crop yields [9] and pollute the environment. This is because applications of N fertilizer in excess of crop requirements leads to the accumulation of nitrate in the soil. Nitrate accumulation degrades surface and groundwater resources, resulting in eutrophication and the contamination of potable water supplies [10].
In a previous study, it was found that N fertilizer application in non-irrigated ditches during drought years reduced N fertilizer absorption by 50% [11]. Meanwhile, other studies have shown that applied N fertilizer is not always fully absorbed by the crop, resulting in partial leaching into the deep soil and even the groundwater [12,13]. In one study, the accumulation of nitrate N in the soil increased with increasing N fertilizer application [14]. Furthermore, rates of residual fertilizer N in the soil have been found to reach 10 to 35% [15]. In an earlier study, water and N fertilizer were simultaneously transported to the roots of crops through drip irrigation tapes and a clear coupling was observed between water and N. N is a key nutrient in blueberry production [16]; unlike most plants, blueberries prefer ammonium N (NH4+-N) to nitrate N (NO3−-N) [17]. Therefore, it is important to explore the influence of water and N coupling on blueberry plants and their soil environment in order to develop efficient blueberry cultivation systems.
Most available research on the interaction between water and N has focused on wheat [18], tomatoes [19], red dates [20], apples [21], and other plants. Meanwhile, research on the coupling of water and fertilizer in blueberries has primarily concentrated on yield and quality. However, there are no reports available on the effect of water and N application on the soil N distribution, plant N utilization, and fruit yield of blueberries. Thus, in the present study, the soil N distribution, plant N distribution, and the fruit yield of rabbiteye “Brightwell” blueberries (Vaccinium virgatum) were analyzed under different water and N coupling treatments. The aim was to identify the most appropriate water and N input parameters under the local ecological conditions. The findings provide a scientific basis and practical guidance for using appropriate water and N management to obtain high yields of blueberries in this region. The results also provide practical and technical guidance for minimizing environmental degradation and improving farmers’ incomes.
The research site was located in Xuanwei Town, Majiang County, Guizhou Province, China (26° 21′–26° 31′ N and 107° 33′–107° 47′ E; Fig. 1). The site was located in a subtropical monsoon humid zone with a warm and humid climate throughout the year. The annual average temperature, annual average rainfall, annual sunshine hours, and frost-free period were 15.7°C, 1266 mm, 1200 h, and 293 days, respectively. The base soil was acid yellow soil, which is suitable for the cultivation of rabbiteye blueberries in Guizhou. Some important soil parameters of the study site included a pH of 4.35–5.50, an organic matter content of 23.9 g/kg, a total N content of 0.78 g/kg, a total phosphorous (P) content of 0.19 g/kg, and a total potassium (K) content of 2.8 g/kg. Upon evaluation of the literature, it was discovered that the pH, annual average temperature, annual average rainfall, annual sunshine hours, frost-free period, and organic matter content values for blueberry production were within the natural ranges of the study site (4.35–5.50, 15.0–16.5°C, 1203–1329 mm, 1140–1260 h, 278–310 days, and 22.7–25.1 g/kg, respectively), and thus the test area was representative of the natural conditions [22,23].
Figure 1: Schematic diagram of the geographical location of the experimental area
Five-year-old plants of the rabbiteye blueberry cultivar “Brightwell” were used as the test materials under field conditions. Water (W) and fertilizer (F) were set as the two factors. The fertilizers used were chemically pure and included ammonium sulfate (N content of 21.2%), superphosphate (P2O5 content of 60.6%), and potassium sulfate (K2O content of 63.2%). There were 13 water and fertilizer treatment combinations, with three replicates per treatment and three plants per replicate, which resulted in a total of 117 plants. Among these 13 combinations, one combination consisted of the no fertilization and no irrigation control (CK).
Fertilizer was applied four times a year: in early March (before flowering), early May (before fruit production), late August to early September (post fruit production and in the flower bud differentiation period), and in early December (reduced fertilizer). Upon fertilization, a 50 cm × 20 cm × 20 cm trench was dug on the left and right sides of the outer periphery of the canopy projection. The fertilizer was dissolved in water (or after fully mixing it with water) according to the design plan (Table 1) and applied to the trench, which was then covered with soil. The other cultivation management practices were based on daily blueberry cultivation management methods [24]. The design scheme is shown in Table 1 and was created in accordance with the literature [25]. Preliminary tests confirmed that there were large differences in the growth of blueberries among the treatments.
Samples were obtained on the 10th day after each treatment, and sampling that would have taken place on rainy days was postponed until the weather cleared. Soil samples were collected with soil augers, using the depth of the water and fertilizer application (20 cm) as the origin. Soil was collected from depths of 20–50 cm to assess the blueberry root distribution and the fertilizer utilization efficiency. Specifically, four soil samples were obtained vertically from the fertilization trench (at a depth of 20 cm) every 10 cm, corresponding to soil layer depths of 20, 30, 40, and 50 cm. Three replicate soil samples were collected for each treatment (Fig. 2). The soil samples were numbered and placed immediately into a Ziplock bag in an ice box. The samples were then brought back to the laboratory in the shortest possible time and placed in a refrigerator at −20°C for storage. To reduce the impact of time on the measurements, N was measured within one week. Similarly, according to the principle of symmetry, the soil on both sides of the fertilization point was collected and brought back to the laboratory for determination of the total N in the soil at the fertilization point. Mature, healthy, and pest-free leaves and annual branches were also collected from around the canopy, placed in a numbered Ziplock bag in an ice box, and transported back to the laboratory for processing.
Figure 2: Sampling diagram
2.4 Determination of Plant Physiological Indexes
2.4.1 Determination of Aboveground Biomass and Total N Content of the Plants
Leaves and branches were collected in May (before fruit harvest) and September (after fruit harvest) from three plants in each treatment. Mature leaves and annual branches with normal growth and no pests and diseases were collected from the periphery of the canopy and packed in Ziplock bags. The leaves and branches were weighed and placed in a drying cabinet to dry the tissues at 105°C for 0.5 h. The tissues were then were dried at a temperature of 75°C until constant mass was reached. The dry matter was weighed using an electronic balance. All plant samples were dried, crushed, and then passed through a 0.5 mm sieve. The samples were kept sealed in a dry environment. To determine the total N content, plant samples (leaves and branches) were digested with concentrated H2SO4-H2O2 and were subjected to a Kjeldahl nitrogen analyzer [26].
2.4.2 Determination of Fruit Yield and the Utilization Rate of Water and Fertilizer
A one-hundredth decimal electronic balance was used to weigh the mature fruits, and the sum of the yield of plants from each treatment was recorded as the treatment yield. The average individual fruit weight was calculated and converted to g/plant. Various indexes were measured as follows:
where Y is the fruit yield (g/plant) and Wi represents the irrigation amount per unit area (L/plant);
2.4.3 Determination of Soil Inorganic N
The fresh soil samples from each measuring point were air-dried, mixed, and passed through a 2 mm sieve. Weigh 5 g of soil sample, extract it with 50 mL of KCl solution with a concentration of 2 mol·L−1, shake it for 0.5 h, centrifuge, stand and filter, and take the supernatant for determination [30].
In this study, 12 water-fertilizer coupling treatments were compared taking no application of water and fertilizer as control; thus making 13 treatments in total (Table 1). Each treatment had 3 replications (3 × 13 = 39) and comprehensive evaluation with membership function by transforming the data related to physiological and growth responses in following Eqs. (1) and (2).
In the above equations, X and x represent the coded and the average calculated value of each treatment, respectively; xmin and xmax represent the minimum and maximum value, respectively, obtained from each parameter from different treatments. The membership function values and average value were accumulated and calculated. The larger average value represented the optimal treatment group [31].
Excel 2010, SPSS 25 (IBM Corp., Armonk, NY, USA), and Graphpad (Prism x86) were used for data processing. One-way analysis of variance, two-way analysis of variance (ANOVA) and Tukey’s significance test were performed on the experimental data.
3.1 Effect of Water and N Coupling on the N Content of the Blueberry Plants
As shown in Table 2, the N content of the blueberry leaves and branches differed significantly (P < 0.05) under different water and N treatments in May and September. In all treatments, the N content of the shoots was significantly lower than that of the leaves. This indicated that the N demand of the leaves was higher than that of the shoots. Irrigation had a highly significant effect on leaf N content in May (before fruit production). At both low and high N application rates, the leaf N content decreased first and then increased with irrigation. However, under moderate N application, the leaf N content increased with irrigation. The leaf N absorption and utilization were highest in the F1W1, F1W3, and F1W4 treatments, and lowest in the F3W3 treatment. In May (before fruit production), the effect of water and N on the N content of the branches was highly significant; the branch N content was highest in F2W2 and F1W2 and lowest in F3W1 and F3W2. The interaction of water and N application on leaf N content was also significant in September (after fruit production); leaf N absorption and utilization was highest in F1W1 and F2W2, and lowest in F1W4. N application also had a significant impact on the N content of the branches in September. Under insufficient water conditions, the N content of the leaves decreased with increased fertilization. When water was sufficient, the N content increased first and then decreased with increasing fertilization, with higher leaf N content in F1W2 and lower in F3W3. These results show that the low N and low water, low N and moderate water, and moderate N and moderate water treatments were beneficial for the absorption and utilization of N in the leaves and branches of the blueberry plants.
3.2 Effect of Water and N Coupling on Blueberry Yield and the Utilization of Water and N
The effects of water and N on blueberry fruit yield were significant. F1W2 and F2W2 were associated with higher yields, and F1W4, F2W3, F3W4, and CK were associated with lower yields. This indicated that moderate amounts of water were beneficial for fruit yield (Table 3). The agronomic efficiency of N fertilizer decreased with increasing fertilization. F1W2, F1W3, and F2W2 plants displayed higher agronomic efficiencies, and F2W1 and F3W1 plants displayed lower efficiencies. Among these, the agronomic efficiency of N fertilizer was highest in the F1W2 and lowest in the F3W4 treatment. This indicated that the combination of high fertilization and high water reduced the NUE. The WUE of the leaves differed significantly among the treatments, and the irrigation treatment had a particularly significant effect on WUE. The irrigation efficiency decreased with increased irrigation. F1W1 and F3W1 displayed the highest irrigation efficiencies and F1W4 displayed the lowest. The soil residual N was the lowest under F1W2 and F1W3. These results indicate that optimal coupling of water and N can improve WUE and NUE, and consequently increase fruit yield.
3.3 Effect of Water and N Coupling on Blueberry Soil N Distribution
3.3.1 Effect of Water and N Coupling on Soil Ammonium N
As indicated in Fig. 3, ammonium N was mainly absorbed in the 0–20 cm soil layer. With the exception of CK, the 0–20 cm soil layer had the lowest ammonium N content in the F1W3 treatment in May, and in the F1W4 treatment in September. In the 20–50 cm soil layer, the F1W1, F1W2, F1W3, and F1W4 treatments resulted in lowest ammonium N contents in the two months. This indicated that the plants in these treatments were better at absorbing soil ammonium N. In the 0–20 cm soil layer, the ammonium N content showed an increasing trend with the season in the various treatments (Fig. 3a). The ammonium N content of the 0–20 cm soil layer in the two months ranged from 0.15 to 57.9 g/kg, while that of the 20–50 cm soil layer ranged from 0.29 to 34.9 g/kg. In September, the ammonium N in the soil layers showed an accumulating trend, with maxima of 57.9 g/kg in the 0–20 cm layer and 34.9 g/kg in the 20–50 cm layer. During the two months, under the same irrigation conditions, increased nitrogen application resulted in an overall increasing trend in the soil ammonium N content of the soil layers. Under the same N application conditions, increasing the irrigation led to a decrease in the ammonium N content of the soil layers in May; in September, the ammonium N content of the soil layers increased first and then decreased. These results demonstrated that the soil ammonium N was easily absorbed by the plants, and that the range of migration with water was small.
Figure 3: Bar plot showing the effect of water and nitrogen coupling on blueberry soil ammonium nitrogen. a is the effect of water-nitrogen coupling on 0–20 cm soil ammonium nitrogen, b is the effect of water-nitrogen coupling on 20–50 cm soil ammonium nitrogen. The error bars show the standard deviation (SD)
3.3.2 Effect of Water and N Coupling on Soil Nitrate N
As shown in Fig. 4, the F1W3 treatment was associated with the lowest nitrate N content in the 0–20 cm soil layer in the two seasons. However, in the 20–50 cm soil layer in September, the F2W2 treatment was associated with the lowest soil nitrate N content. This suggests that there is little threat of soil nitrate N pollution under these treatment conditions. The soil nitrate N content of the different treatments in the 0–20 cm layer (Fig. 4a) showed an upward trend with the season, while that of the 20–50 cm layer (Fig. 4b) showed a downward trend with the season. The nitrate N content ranged from 0.11 to 97.5 g/kg in the 0–20 cm soil layer and from 2.55 to 79.7 g/kg in the 20–50 cm soil layer. Maximum nitrate N content values were reached in September. During the two months, under the same irrigation conditions, increased N application led to an overall increasing trend in the soil nitrate N content in the soil layers. Meanwhile, under the same N application conditions, increased irrigation led to a decrease in the total soil nitrate N content in the 0–20 cm soil layer and to an increase in total soil nitrate N in the 20–50 cm soil layer. Thus, the reduction of the soil nitrate N content in the 0–20 cm soil layer was related to both the nitrate content of the plants and the downward movement of soil nitrate N. This indicated that with increased irrigation and N application, there was significant nitrate N leaching in the soil.
Figure 4: Bar plot showing the effects of water and nitrogen coupling on nitrate nitrogen on the soil. a is the effect of water and nitrogen coupling on 0–20 cm soil nitrate nitrogen, b is the effect of water and nitrogen coupling on 20–50 cm soil nitrate nitrogen. The error bars show the standard deviation (SD)
3.3.3 Membership Function Analysis
As indicated in Tables 4 and 5, the average membership functions of each treatment in both seasons were higher than that of CK. This indicated that the indexes of N absorption and utilization of the blueberries in all water and N treatments were better than in CK. The average membership functions of the moderate N and water treatments (F1W2, F1W1, and F2W2) were significantly higher than those of the other treatments. This indicated that low N and moderate water (F1W2) was the optimal combination for producing high yields of blueberries with minimal environmental impact. The next best combinations were low N and low water (F1W1) and moderate N and moderate water (F2W2).
4.1 Blueberry Fruit Yield and Soil N Distribution under Water and N Coupling
Yield formation in the reproductive organs of plants is closely linked to nutrient accumulation and distribution in the soil. In the present study, it was shown that in May (before fruit production) and September (after fruit production), N accumulation was significantly higher in the leaves than in the branches. This is consistent with the results found for oil sunflower by Wang et al. [32]. These results imply that the N in the leaves may be transported to the fruit, thus promoting yield formation. The effects of different water and N applications on N accumulation, distribution, absorption, and utilization in the blueberry leaves and branches differed significantly. Under low and moderate irrigation conditions, the application of additional N fertilizer within a certain range (pure N ≤ 29 g/plant) could promote fruit yield and increase Nitrogen levels. These results corroborate the findings of Pang et al. [33] for blueberry plants. However, the results are inconsistent with the findings of Xing et al. [34] for tomato plants. Thus, it can be deduced that appropriate amounts of N fertilizer are beneficial for N absorption by fruit crops and promote increased fruit yield, but different plants have different optimal N fertilization demands. Under low and moderate N fertilization conditions, increasing irrigation within a certain range (irrigation ≤ 2.5 L/plant) supported the accumulation of N in the blueberry leaves and branches and promoted yield. The variation in N accumulation and absorption trends of the blueberry leaves and branches reflect the biological impacts of the different water and fertilizer combinations.
The WUE results of the present study are consistent with those of Ertek et al. [35] and Zotarelli et al. [36]: under deficient irrigation, WUE is typically high, and a high irrigation volume often leads to low WUE. The findings of this study indicate that under higher levels of N application (F3), the agronomic utilization rate and partial productivity of N fertilizer decreased. This result is similar to that of the study of maize by Guo et al. [37]. Excessive N application thus reduced the agronomic utilization rate of N fertilizer. It was also found that the low N and moderate water (F1W2 and F1W3) treatments resulted in high fruit yields but also less nitrate N retention in the soil. Under these conditions, the economic input-output ratio was high and the soil N accumulation was low, and thus the economic and ecological benefits were maximized.
The main types of inorganic N absorbed and used by plants are ammonium N and nitrate N [38,39]. In the present study, it was found that the amount of ammonium N and nitrate N in the soil decreased with reduced N application and elevated irrigation over the two months. This is because nitrate N is highly mobile and thus easily lost with water. These results are consistent with the results of related studies in amaranth and spinach [40], and in a cucumber-tomato rotation system [41]. Controlling the irrigation and N application significantly slowed the migration of nitrate N in the soil. This indicates that water and N management can regulate the distribution of nitrate N and ammonium N in the soil and can also effectively reduce soil N, which has ecological and economic benefits. Studies have shown that different N application rates mainly influence the soil ammonium N content in the 0–20 cm soil layer [42]. These results demonstrate that soil particles and colloids have a strong adsorption effect on ammonium N. Moreover, due to the exchange reaction in the soil and the organification, nitrification, and denitrification of inorganic N, it is difficult for soil NH4+-N to migrate deeper. Ammonium N fertilizer provides a substrate for nitrification to ensure an effective N supply at a later period and avoids excessive ammonium N accumulation in the short term. Therefore, it is not surprising that in this study, there was no significant correlation between soil ammonium N content and N application rate. As the N application rate was increased, the nitrate N content of the 0–20 cm and 20–50 cm soil layers showed an increasing trend. With an increase in irrigation volume, the nitrate N content of the 0–20 cm soil layer decreased, and the nitrate content in the 20–50 cm soil layer increased. This increase in nitrate N content suggests that the reduction in soil nitrate N content in the 0–20 cm layer was not only related to the consumption of soil nitrate N by plants, but also to the downward movement of soil nitrate N. This result is similar to the conclusions of Zhang et al. [43] in their study of oats and of Li et al. [41] in their study of a cucumber-tomato rotation system. In these studies, increased irrigation and N application resulted in significant leaching of soil nitrate N.
4.2 Selection of a High-Yield Water and N Coupling Strategy for Blueberry Cultivation
The coupling of water and N significantly impacted the yield of rabbiteye blueberry fruit. As indicated in Tables 4 and 5, the top three treatments in terms of yield included the low N and moderate water (F1W2 and F1W3), and the low N and low water (F1W1) treatments. Thus, in the Guizhou blueberry production area, it is recommended that farmers use a combination of low N and low water, or low N and moderate water for rabbiteye blueberry cultivation and management in order to maximize fruit yield.
Improving the yield and quality of the fruits is an important goal in blueberry cultivation. Bryla et al. showed that fertigation is a key factor affecting blueberry fruit yield, with a tight balance between ensuring sufficient water supply in the dry season and reducing N leaching [16]. Under sufficient irrigation, the fruit yield of the W2 treatments was still significantly higher than that of the W4 treatments. A comparison of the nitrate N content of the 0–20 cm and 20–50 cm soil layers in the W4 and W2 treatments in May indicated that less nitrate was leached into the soil below 50 cm when water was sufficient (as in W2). Therefore, without considering fruit yield or environmental friendliness, W2 irrigation conditions are the best choice. In terms of soil nutrients, the N application level of the F1 treatment was optimal throughout the growing season. Considering plant water requirements, the W2 irrigation parameters should be applied from May to September to ensure plant growth and development. As nitrate N accumulated in the 0–50 cm soil layer in September, the monitoring of ammonium N and nitrate N in the root zone below 50 cm should be strengthened.
The present analysis shows that the water-N ratio is of great significance for the precise cultivation of Guizhou rabbiteye blueberries and for the conservation of the soil. In particular, determining the best water-N ratios for the fruit farmers is necessary for improving fruit yield and quality, and for reducing environmental pollution, which have obvious economic and ecological benefits. In the present study, only the N and water requirements of rabbiteye blueberries in the Guizhou production area were evaluated. Other blueberry varieties, such as Blue Rain, North Jersey, and Jersey, have different ecological characteristics, and thus future research should focus on determining the optimal water and fertilization requirements for these varieties in Guizhou. The present results provide a theoretical and technical foundation for the establishment of a precise blueberry cultivation system in Guizhou.
In this study, N accumulation mainly occurred in the leaves of the blueberry plants, and was significantly higher in the leaves than in the branches in both seasons (May and September). Increasing water and N application within appropriate ranges (pure N ≤ 29 g/plant and irrigation ≤ 2.5 L/plant) could promote the effective accumulation of N in the blueberry leaves and branches, promote the absorption and utilization of N by the blueberries, and increase the fruit yield. For the same irrigation conditions, the inorganic N content of the soil increased with the increase in N application. With increased irrigation, the soil ammonium N content decreased, while the soil nitrate N content decreased in the 0–20 cm soil layer but increased in the 20–50 cm soil layer. With increased irrigation and N application, nitrate N began to leach significantly into the soil. Under the study conditions, the low N and low water, and low N and moderate water treatments improved rabbiteye blueberry production and supported the ecological soil environment. The findings of this study can be used to improve not only Guizhou rabbit-eye blueberry planting precision and effect, but also improve the soil environment and the revenue of local fruit growers.
Authorship: Xiaolan Guo: Writing-original draft preparation and methodology; Di Zhao and Jinbin Hu: Formal analysis and investigation; Muhammad Shakeel and Jianbin Wang: Writing-review and editing; Delu Wang: Supervision.
Permission for Plant Material Collection: We have obtained permission from Wuyang Maritt Blueberry Base Institute for sample collection. The study was carried out with relevant institutional, national, and international guidelines and legislation.
Funding Statement: This study was funded by the National Natural Science Foundation of China (NSFC 31260192) and the Major Projects in Guizhou Province (SY [2015] 6032-2).
Conflicts of Interest:The authors declare that they have no conflict of interest report regarding the present study.
References
1. Li, Y. D., Pei, J. B., Chen, L. (2020). Annual report on China’s blueberry industry. Journal of Jilin Agricultural University, 43(1), 1–8. [Google Scholar]
2. Zhang, Y. C., Wang, X., Liu, B. G., Liu, Q., Zheng, H. et al. (2020). Comparative study of individual and co-application of biochar and wood vinegar on blueberry fruit yield and nutritional quality. Chemosphere, 246, 125699. DOI 10.1016/j.chemosphere.2019.125699. [Google Scholar] [CrossRef]
3. Yan, N. N., Wu, B. F., Zhu, W. W. (2020). Assessment of agricultural water productivity in arid China. Water, 12(4), 1161. DOI 10.3390/w12041161. [Google Scholar] [CrossRef]
4. Xu, G. L., Liu, X. M., Xu, X. B., Li, Q. Q., Zhang, H. et al. (2016). Effects of four arbuscular mycorrhizal fungi on drought resistance of Nangao blueberry. Journal of Zhejiang University, 42(4), 427–434. [Google Scholar]
5. Paltineanu, C., Coman, M., Nicolae, S., Ancu, I., Calinescu, M. et al. (2017). Root system distribution of highbush blueberry crops of various ages in medium-textured soils. Erwerbs-Obstbau, 60(3), 187–193. DOI 10.1007/s10341-017-0357-3. [Google Scholar] [CrossRef]
6. Hernández, M., Echarte, L., Della Maggiora, A., Cambareri, M., Barbieri, P. et al. (2015). Maize water use efficiency and evapotranspiration response to N supply under contrasting soil water availability. Field Crops Research, 178, 8–15. DOI 10.1016/j.fcr.2015.03.017. [Google Scholar] [CrossRef]
7. Li, S. X., Wang, Z. H., Li, S. Q., Gao, Y. J. (2015). Effect of nitrogen fertilization under plastic mulched and non-plastic mulched conditions on water use by maize plants in dryland areas of China. Agriculture Water Management, 162(11), 15–32. DOI 10.1016/j.agwat.2015.08.004. [Google Scholar] [CrossRef]
8. Miao, Y. F., Wang, Z. H., Li, S. X. (2015). Relation of nitrate N accumulation in dryland soil with wheat response to N fertilizer. Field Crops, 170, 119–130. DOI 10.1016/j.fcr.2014.09.016. [Google Scholar] [CrossRef]
9. Li, T., Liu, J., Wang, S., Zhang, Y., Zhan, A. et al. (2018). Maize yield response to nitrogen rate and plant density under film mulching. Agronomy Journal, 110(3), 996–1007. DOI 10.2134/agronj2017.09.0547. [Google Scholar] [CrossRef]
10. Liu, C. A., Zhou, L. M., Jia, J. J., Wang, L. J., Si, J. T. et al. (2014). Maize yield and water balance is affected by nitrogen application in a film-mulching ridge-furrow system in a semiarid region of China. European Journal of Agronomy, 52(1), 103–111. DOI 10.1016/j.eja.2013.10.001. [Google Scholar] [CrossRef]
11. Benjamin, J. G., Porter, L. K., Duke, H. R., Ahuja, L. R. (1997). Corn growth and nitrogen uptake with furrow irrigation and fertilizer bands. Agronomy Journal, 89(4), 609–612. [Google Scholar]
12. Jolley, V. D., Pierre, W. H. (1977). Profile accumulation of fertilizer-derived nitrate and total nitrogen recovery in two long-term nitrogen-rate experiments with corn. Soil Science Society of America Journal, 41(2), 373–378. [Google Scholar]
13. Spalding, R. F., Exner, M. E. (1993). Occurrence of nitrate in groundwater—A review. Journal of Environmental Quality, 22(3), 392–402. [Google Scholar]
14. Wang, X. N., Wang, Z. H., Li, S. X. (2007). The effect of nitrogen fertilizer rate on summer maize yield and soil water-nitrogen dynamics. Journal of Ecology, 27(1), 197–204. [Google Scholar]
15. Kumar, K., Goh, K. M. (2002). Recovery of 15N-labelled fertilizer applied to winter wheat and perennial ryegrass crops and residual 15N recovery by succeeding wheat crops under different crop residue management practices. Nutrient Cycling in Agroecosystems, 62(2), 123–130. [Google Scholar]
16. Bryla, D. R., Strik, B. C. (2015). Nutrient requirements, leaf tissue standards, and new options for fertigation of northern highbush blueberry. HortTechnology, 25(4), 464–470. [Google Scholar]
17. Poonnachit, U., Darnell, R. (2004). Effect of ammonium and nitrate on ferric chelate reductase and nitrate reductase in Vaccinium species. Annals of Botany, 93(4), 399–405. [Google Scholar]
18. Sui, J., Wang, J., Gong, S., Xu, D., Zhang, Y. (2015). Effect of nitrogen and irrigation application on water movement and nitrogen transport for a wheat crop under drip irrigation in the North China Plain. Water, 7(11), 6651–6672. [Google Scholar]
19. Harmanto, Salokhe, V. M., Babel, M. S., Tantau, H. J. (2005). Water requirement of drip irrigated tomatoes grown in greenhouse in tropical environment. Agriculture Water Management, 71(3), 225–242. [Google Scholar]
20. Dai, Z., Fei, L., Huang, D., Zeng, J., Chen, L. et al. (2019). Coupling effects of irrigation and nitrogen levels on yield, water and nitrogen use efficiency of surge-root irrigated jujube in a semiarid region. Agriculture Water Management, 213, 146–154. [Google Scholar]
21. Zhou, H., Niu, X., Yan, H., Zhao, N., Zhang, F. et al. (2019). Interactive effects of water and fertilizer on yield, soil water and nitrate dynamics of young apple tree in semiarid region of Northwest China. Agronomy, 9(7), 360. DOI 10.3390/agronomy9070360. [Google Scholar] [CrossRef]
22. Yang, L., Nie, F., Zhou, H. Y., Fang, P. W., He, J. et al. (2007). The introduction cultivation of blueberry and its utilization evaluation in Guizhou. Guizhou Agricultural Science, 35(5), 48–52. [Google Scholar]
23. Nie, F., Wang, Y., Liao, Y. J., Wen, G. Q. (2010). Studies on high-efficiency cultivation and matching management technologies of rabbiteye blueberry. Anhui Agricultural Sciences, 38(28), 15525–15527. [Google Scholar]
24. Wang, Y., Zhang, Q. Y., Li, Y. X., Wen, G. Q., Liao, Y. J. et al. (2014). High-efficiency and high-yielding cultivation techniques of Lanshou in hilly and mountainous areas of Guizhou. Southern Agriculture, 8(18), 26–27. [Google Scholar]
25. Zhu, M. L. (2017). Anhui Wuwei open field blueberry water and fertilizer management technology. Soil Fertility and Water Management, 5, 22–24. [Google Scholar]
26. Shidan, B. (2005). Soil agrochemical analysis, 3rd edition. Beijing: China Agricultural Press. [Google Scholar]
27. Badr, M. A., Abou-Hussein, S. D., El-Tohamy, W. A. (2016). Tomato yield, nitrogen uptake and water use efficiency as affected by planting geometry and level of nitrogen in an arid land. Agricultural Water Management, 169, 90–97. [Google Scholar]
28. Wang, H. D., Li, J., Cheng, M. H., Zhang, F. C., Wang, X. K. et al. (2019). Optimal drip fertigation management improves yield, quality, water and nitrogen use efficiency of greenhouse cucumber. Scientia Horticulturae, 243, 357–366. [Google Scholar]
29. Li, Z., Li, G. L., Sun, Y. Q., Su, W. B., Fan, F. Y. et al. (2019). Effects of water and nitrogen supply on nitrogen assimilation and utilization of sugar beet under drip irrigation under mulch. Journal of Plant Physiology, 55(6), 803–813. [Google Scholar]
30. Du, S., Gao, X. Z. (2006). Second edition of soil analysis technical specifications. Beijing: China Agriculture Press. [Google Scholar]
31. Roy, R., Mostofa, M. G., Wang, J. X., Fornara, D., Sarker, T. et al. (2021). Revegetation intervention of drought-prone coal-mined spoils using Caragana korshinskii under variable water and nitrogen-phosphorus resources. Agriculture Water Management, 246, 106712. [Google Scholar]
32. Wang, Z. H., Quan, L. S., Zheng, X. R., Pei, L. (2016). Effects of water-nitrogen coupling on nitrogen uptake and nitrate accumulation in soil of oil sunflower in drip-irrigated multiple cropping system. Scientia Agricultura Sinica, 47(10), 91–100. [Google Scholar]
33. Pang, W., Hou, Z. X., Li, G. L., Liu, Y., Liu, S. H. et al. (2012). The effect of N application on the growth and fruit quality of blueberry. Scientia Agricultura Sinica, 28(13), 225–229. [Google Scholar]
34. Xing, Y. Y., Zhang, F. C., Zhang, Y., Li, J., Qiang, S. C. et al. (2015). Effect of irrigation and fertilizer coupling on greenhouse tomato yield, quality, water and nitrogen utilization under fertigation. Scientia Agricultura Sinica, 48(4), 713–726. [Google Scholar]
35. Ertek, A., Şensoy, S., Küçükyumuk, C., Gedik, I. (2004). Irrigation frequency and amount affect yield components of summer squash (Cucurbita pepo L.). Agriculture Water Management, 67(1), 63–76. [Google Scholar]
36. Zotarelli, L., Dukes, M. D., Scholberg, J. M., Hanselman, T., Le Femminella, K. et al. (2008). Nitrogen and water use efficiency of zucchini squash for a plastic mulch bed system on a sandy soil. Scientia Horticulture, 116(1), 8–16. [Google Scholar]
37. Guo, B. Y., Gao, H., Tang, C., Liu, T., Chu, G. X. (2015). Response of water coupling with N supply on maize nitrogen uptake, water and N use efficiency, and yield in drip irrigation condition. Journal of Applied Ecology, 26(12), 3679–3686. [Google Scholar]
38. Miller, A., Cramer, M. (2005). Root nitrogen acquisition and assimilation. In: Lambers, H., Colmer, T. D. (Eds.Root physiology: From gene to function, pp. 1–36. Dordrecht: Springer. [Google Scholar]
39. Song, S., Yi, L., Liu, H., Sun, G., Chen, R. (2011). Effect of ammonium and nitrate ratios on growth and yield of flowering Chinese cabbage. In: Jin, D., Lin, S. (Eds.Advances in multimedia, software engineering and computing, pp. 227–232. Berlin, Heidelberg: Springer. [Google Scholar]
40. Yu, H. M., Li, Z. H., Gong, Y. S. (2005). Leached nitrate in vegetable field under different water and nitrogen fertilizer management practices. Scientia Agricultura Sinica, 38(9), 1849–1855. [Google Scholar]
41. Li, R. N., Zhang, Y. C., Huang, S. W., Tang, J. W., Wang, L. Y. et al. (2013). Effects of combined application of organic manure and chemical fertilizers on soil nitrogen availability and movement under water and fertilizer saving management in cucumber-tomato double cropping system. Plant Nutrition and Fertilizers, 19(3), 677–688. [Google Scholar]
42. Ma, X. H., Yu, Z. W., Liang, X. F., Yan, H., Shi, G. P. (2006). Effects of nitrogen application rate and bottom application rate on the spatial and temporal changes of soil nitrate nitrogen and ammonium nitrogen content. Journal of Applied Ecology, 17(4), 630–634. [Google Scholar]
43. Zhang, P., Jiang, J., Ma, J. J., Yang, Z. P., Wang, Y. L. (2018). Effects of different water and nitrogen treatments on changes of water, nitrogen and salt in saline soil and oat yield. Journal of Irrigation and Drainage, 37(5), 1–12. [Google Scholar]
Cite This Article
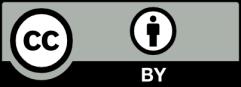