Open Access
ARTICLE
Seed Priming and Foliar Supplementation with β-aminobutyric Acid Alleviates Drought Stress through Mitigation of Oxidative Stress and Enhancement of Antioxidant Defense in Linseed (Linum usitatissimum L.)
1 Department of Agronomy, University of Layyah, Layyah, 31200, Pakistan
2 Department of Agronomy, Bahauddin Zakariya University, Multan, 60000, Pakistan
3 State Key Lab of Crop Stress Biology for Arid Areas, College of Agronomy, Northwest A&F University, Yangling, 712100, China
4 Department of Agronomy, MNS University of Agriculture, Multan, 60000, Pakistan
5 Department of Agronomy, Faculty of Agriculture, University of Poonch Rawalakot, Rawalakot, 10250, Pakistan
6 Independent Researcher, Middlesbrough, North Yorkshire, UK
7 Plant Production Department, College of Food and Agriculture Sciences, King Saud University, P.O. Box 2460, Riyadh, 11451, Saudi Arabia
8 Grassland and Forage Division, National Institute of Animal Science, Rural Development Administration, Cheonan, 31000, Korea
9 Department of Field Crops, Faculty of Agriculture, Siirt University, Siirt, 56100, Turkey
10 Department of Agronomy, Faculty of Agriculture, Kafrelsheikh University, Kafr El-Shaikh, 33516, Egypt
* Corresponding Authors: Allah Wasaya. Email: ; Ayman El Sabagh. Email:
Phyton-International Journal of Experimental Botany 2023, 92(11), 3113-3131. https://doi.org/10.32604/phyton.2023.029502
Received 22 February 2023; Accepted 24 July 2023; Issue published 24 October 2023
Abstract
Drought is one of the critical limitations to agricultural soils and crop plants. Scarcity of water is increasing due to climate change that lead to increasing threats to global food security. Therefore, ecofriendly and cost effective strategies are highly desirable for mitigating drought stress along with sustainable and smart agricultural production. The aim of the study was to mitigate DS using seed priming and exogenous supplementation of β-aminobutyric acid (BABA) in linseed (Linum usitatissimum L.). Different doses (0, 50, 100 and 150 µM) of BABA were used for seed priming agent and foliar spraying under three soil moisture levels viz., 25% (SM25), 45% (SM45) and 65% (SM65). The response variables of both experiments included different agro-botanical traits and oxidative stress indicators such as melondialdehyde content, free proline accumulation, and antioxidant defense in plants. The linseed plants showed water stress at SM25 that reduced plant height, number of branches per plant, time taken to flower initiation and heading, and root and shoot dry weights. Additionally, the number of capsules and seeds per capsule showed a significant decline at SM25, which led to a drastic reduction in 100-seed weight yield in linseed plants in both experiments. However, seed priming and foliar supplementation with of BABA (50–100 µM) significantly improved these morpho-agronomical attributes in linseed plants under DS. The results revealed that the BABA was fully active in linseed plants at SM25. Interestingly, the combination of SM25 with BABA significantly improved the antioxidant enzymes superoxide dismutase (SOD), catalase (CAT), ascorbate peroxidase (APX), and peroxidase (POD) activity, which significantly enhanced DS tolerance in linseed plants. These findings might be useful to oil seed breeders and farmers linseed for breeding program in linseed plants as well as sustainable agricultural production of oil seed crop plants.Keywords
Linseed (Linum usitatissimum L.) is an important oilseed and fiber crop that is generally used fora variety of industrial products [1,2]. Globally, it is cultivated more than 3.2 million hectares as oilseed crop, while 0.26 million hectares cultivated for fibre production [3]. Interestingly, around 20% oil is produced only for edible purposes, linseed oil is used for the manufacturing paints, varnishes, polishes, oilcloth, biodiesel and numerous cosmetic products [4]. Linseed is a rich source of digestible protein, dietary fibres, and an abundance of micro-nutrient, lignin and Omega-3 and Omega-6 fatty acids [5]. Linseed oil contains a variety of important fatty acids with a high amount of linoleic acid (38%–42%), that provide excellent health benefits for both humans and animals [6–9]. Linseed crop is moderately tolerant to abiotic stresses especially drought stress (DS) [2]. However, the crop is susceptible to DS at seedling stage, flower initiation and seed development stage due to its shallow root system [10]. Therefore, suitable strategies are highly demandable for sustainable linseed production under drought stress. Drought is one of the severe constraints that severely limits crop yield by lowering growth and yield traits of many economically important crops [11,12]. Drought stress (DS) disrupts the morphological and physiological processes in crop plants which leads to decrease seed yield and its qualitative traits [13]. DS reduces root proliferation [14], decreases the green pigment in leaves, decline lowers turgidity in leaves, damages cell membrane and slows down the process of cell division in plants [15]. Therefore, DS is key limiting factor of biochemical attributes of oilseed crops [16]. Plant have evolved several defense systems associated with abiotic stress tolerance. Plant accumulates free proline content, and antioxidants such as superoxide dismutase (SOD), catalase (CAT) and peroxidase (POD) under stressful conditions [17]. However, a great variation in these antioxidant concentrations has been observed under different intensities of DS [18]. Antioxidants consist of two types of mechanisms, enzymatic and non-enzymatic. These mechanisms assist the plants to deal effectively with reactive oxygen species (ROS) [19]. Ascorbate, as a non-enzymatic antioxidant protects the plant from the deterioration of H2O2 [20]. A number of antioxidants assist crop plants in deactivating ROS in such a way that CAT converts H2O2 into H2 and O2 and then oxidation of ascorbate gets started by the action of POD [21]. It has been observed that the increasing intensity of drought stress is the result of climate change. Due to the effect of DS, the quantity and quality of crops significantly declined. Thus, eco-friendly and cost effective approaches are imperative for alleviating DS in crops.
To off-set the adverse effects of DS, various types of signaling molecules such as Beta-aminobutyric acid (BABA) that is environmentally safe and non-proteinogenic amino acid, BABA plays an important role in abiotic stress tolerance in plants [22]. Several reports validated that BABA alleviates DS in maize [23], mitigates cadmium toxicity stress in soybean [24], DS in other plants [13,25], insect-pests attack in soybean and tomato [26,27]. In addition, positive roles BABA in imparting DS tolerance indifferent crops such as chrysanthemum [28], faba bean [29], brassica [23] and sunflower have been reported previously [13]. Seed priming (pre-sowing seed treatment for 6–48 h) with BABA alters seedlings’ growth, physiological, biological and chemical traits under DS [30]. In addition, it enhanced crop stand establishment, photosynthetic efficiency and plant survival [31]. Besides seed priming, exogenous foliar application of signaling molecules remained effective in promoting the growth and yield of French beans [32,33]. Recently, many successful attempts, consisting seed priming and foliar methods have been used to minimize the inhibitory effects of abiotic stresses on different plant species such as in sorghum under salinity stress [34], and in maize and rice under chilling stress [35]. Due to these advancements of BABA, it has not yet been studied whether the BABA alleviates DS in linseed seedlings. However, research gaps are still exist regarding the use of BABA against DS for important industrial oilseed crop like linseed. Therefore, research was designed with the hypothesis that not only the BABA dose but also the application method might influence linseed plants growth, yield and defense mechanisms when subjected to varying levels of DS. In this study, we find out the effect of DS on several physiological and agronomical traits of linseed plants. We also applied the seed priming process and foliar supplementation of different concentrations of BABA for mitigating DS in linseed plants.
2.1 Experimental Site and Planting Material
A study of two parallel pot experiments (seed priming experiment and foliar application experiment) was carried out at the college of Agriculture, Bahauddin Zakariya University, Bahadur Sub-Campus Layyah., Pakistan (30.58°N latitude, 70.57°E longitude) during winter season of 2018 and 2019. Seeds of linseed genotype, i.e., LS-12 were used as study material. For both experiments, clay pots with a dimensions of 26 cm internal diameter and 30 cm height, were used. Ten seeds were sown in each pot initially. After uniform germination, five healthy plants were kept for rest of the study. Each pot was filled with 8 kg of dried soil having sandy clay-loam texture. The soil pH was 7.60, saturation percentage 32.0, electrical conductivity 2.24 dSm−1, organic matter 0.48%, total nitrogen 0.034%, phosphorus 7.40 mg kg−1 and potassium 82 mg kg−1. Plant nutrient requirement was met by applying N, P and K at the rate of 84, 56 and 56 kg ha−1, respectively by applying fertilizers of urea, diammonium phosphate and sulfate of potash. The NPK doze was applied as 8 kg of potted soil, the dosage of 84, 56 and 56 kg ha−1 of NPK works out to 336, 224 and 224 mg NPK/8 kg potted soil or 730, 487 and 448 mg of Urea, DAP and SOP respectively in 8 kg potted soil. P and K fertilizers were applied as full strength, and N fertilizer in half strength, which were mixed and used in pot filling. However, the remaining N was applied at inflorescence development and emergence stage-5 [36]. Sowing was done on 13 November 2018, and harvesting was started in the 3rd week of April 2019. The fungicides and insecticides were used for plant protection.
2.2.1 Experiment 1: Seed Priming With β-Aminobutyric Acid (BABA)
The seed priming of linseed was done with four different doses of BABA (0, 50, 100 and 150 µM). These doses of BABA were selected following the study of Elsherbiny et al. [37]. The linseed seeds were soaked separately into the above mentioned four concentrations of BABA solution and aerated for 24 h. Priming with water (Hydro-priming) was considered as a control treatment. The BABA (β-aminoisobutyric acid) was purchased from Sigma-Aldrich. Soil moisture regimes and different concentrations of BABA were allocated factor wise and replicated three times under a completely randomized statistical design (CRD) factorial arrangement, and treatments were replicated thrice. Three moisture regimes with different field capacity levels, i.e., FC-40% = 25% soil moisture (SM25); FC-60% = 45% soil moisture (SM45) and FC-80% = 65% soil moisture (SM65) were used to impose drought stress. According to the method of Chakma et al. [38], soil moisture content (%) was calculated by using the following equation:
The level of SM65 was considered as the normal irrigation regime, SM45 as medium stress regime, while SM25 as severe stress regime and all these soil moisture levels were calculated by using the Eq. (1). Soil moisture meter was used to estimate soil moisture of different pots and then by adding water into each pot as per desired level according to the treatments.
2.2.2 Experiment 2: Foliar Application of Beta Aminobutyric Acid (BABA)
In this experiment, same concentration of BABA (0, 50, 100 and 150 µM) was sprayed on plant leaves with CRD factorial arrangement with three replications. The BABA doses were sprayed uniformly on the leaves of linseed before sunrise. Note, the BABA was applied 40 days after sowing as the linseed plant has grown in 30–60 DAS [39].
Days of flowering were recorded from the day of seedling emergence to the days of 50%, total ten plants were randomly used for the experiment. The harvesting was terminated while 50% capsules were became brown. At the harvesting stage (145 days after sowing), ten plants from each pot were harvested. The number of branches per plant was counted manually. The plant height was measured using length measuring tape and soil surface to shoot tip was considered for this trait. Root and shoot parts of the linseed were separated, and oven-dried at 70oC. Finally, the shoot and root dry weights were measured by using a digital balance.
During harvesting time, number of total capsules in total five plants were threshed and counted manually. The number of seeds per capsule, and then their average number were recorded. Number of seeds per plant was separated and their weight was recorded using a digital balance for measuring the 100-seeds weight was recorded using an automatic seed counting machine.
2.3.3 Determination of Oxidative Stress Indicators
1 g of fresh leaf sample was extracted in 5% w/v solution of sulfosalicylic acid and centrifuged at 15000 g for about 10 min. Spectrophotometer was used to analyze proline contents at 520 nm in the chemical reaction of acidic ninhydrin. Toluene was used as a blank. Density of proline 0 to 520 μL (20–100 μg/ml) was used as a standard curve described by [40].
Determination of Melondialdehyde (MDA) Content
According to the method of [41],
where A532 represents the highest absorption rate by modified thiobarbituric acid to malondialdehyde (TBA-MDA) assay. A600 representing the correction for nonspecific turbidity and MDA molar extinction coefficient was equal to 155000.
Briefly, 0.5 g of plant tissue was stored in liquid nitrogen until further analysis. At the time of analysis, sample was grounded with liquid nitrogen and centrifuged at 12000 g for 15 min. After that, 0.5 ml supernatant was to the solution containing K2SO4 (10 mM, 7.0 pH) and 1 ml of KI (1M). At 390 nm suspension OD was recorded [42].
Measurement of Catalase (CAT), Superoxide Dismutase (SOD), Peroxidase (POD) and Ascorbate Peroxidase (APX)
The CAT, SOD, POX and APX extraction was conducted at low temperature ranging from 0°C to 4°C. Plant tissue was grinded with liquid nitrogen. Briefly, 5 g of tissue was homogenized with a extracting buffering solution (K2SO4 50 mM, 7.8 pH). This homogenized mixture was centrifuged at 12000 g for 15 min. Chilling temperature (4°C) was maintained during expration process. The CAT activity was determined using the method used previously [43]. For this purpose we add 50 ml of enzyme extract (EE) in 2.5 ml of PO4 buffer of (50 mM, 7.4 pH) along with 0.1 ml of H2O2 (1%). Spectrophotometer was used and the absorbence of the solution extract was read at 240 nm. A procedure was being followed for identifying the activity of SOD [44]. A reaction homogenate was prepared containing K2PO4 (50 mM, 7.0 pH), EDTA (0.1 mM), APX mixture (0.5 mM), H2O2 (1.54 mM) EE (50 ml) by following the procedure of [45]. Activity of APX was checked by using spectrophotometer at 290 nm for 3 min due to the reduction in absorption.
Two-way analysis of variance (ANOVA) was used for analysing experimental data. The software Statistix 8.1. was applied for this analysis. Furthermore, least significant difference test (LSD) test was considered where the signicant level was considered at p < 0.05 among the treatment means. Moreover, radar and bar graphs for the mean and interactive study of the present analyses were created by using program OriginProLabTM Analysis version 8.5.1.
The recorded results revealed that individual effects of irrigation regime and various levels of BABA had significant influence at p < 0.05 on growth parameters (days to flowering, days to harvesting, branches on each plant, plant height, shoot and root dry weight), yield attributes (capsules per plant, weight of 100-seeds and seed yield) and oxidative stress indicators and antioxidants such as proline, MDA, H202, CAT, SOD, POD and APX. However, the average number of seeds in each linseed capsule was insignificant at p < 0.05 (Table 1). The interaction effect of irrigation regime and BABA were non-significant in both experiments. However, the number of branches and seed yield produced by each plant were significantly higher in seed priming experiment. In addition, the antioxidants activity was varied significantly in both experiments (Table 1). Severe stress regimes (SM25) and medium stress regime (SM45) considerably decreased the growth and yield traits while increase the antioxidant level of linseed compared to normal irrigation regime (SM65). However, the use of seed priming technique and foliar method of BABA in different concentrations enhanced these attributes by mitigating drought stress.
3.1 Seed Priming with BABA Improved Plant Growth and under Drought Condition
In Experiment 1 (seed priming), the individual effect of irrigation regimes especially SM25 and SM45 decreased the days to flowering and harvesting by 8.53%, 5.78% and 3.69%, 1.84%, respectively, as compared to SM65. Application of BABA (50, 100 and 150 mM) decreased the effect of DS by increasing the days to flowering by 2.62%, 1.02%, 0% and days to harvesting by 1.82%, 0.16%, 0.16%, respectively, as compared to the control treatment (Table 2).
The results showed that SM25 and SM45 reduced number of branches per plant as well as average height of plants by 13.8%, 5.5% and 15.1%, 4.1%, respectively, as compared to SM65. However, 50 mM of BABA application increased number of branches per plant by 3.7%, while 100 and 150 mM concentrations of BABA decreased their numbers by 5.7% and 11.7%, respectively in comparison to corresponding values recorded in control conditions. The improvement of plant height (2.76% and 0.25%) was recorded by the application of BABA (50 and 100 mM), while 150 mM of BABA decreased the plant height by 1.51% as compared to the control treatment (Table 2). The individual effect of SM25 and SM45 reduced the shoot dry weight and root dry weight by 39.8%, 18.3% and 39.9%, 18.5%, respectively, as compared to SM65. Application of BABA (50, 100 and 150 mM) minimized the adverse effects of DS by enhancing the shoot and root dry weights by 32.0%, 14.7%, 1.8% and 32.2%, 15.4%, 2.1%, respectively, as compared to the control (Table 3).
Similarly, number of capsules and seeds per plant and per capsules were decreased by moisture stress at SM25 and SM45 as compared to SM65. However, 50 mM of BABA application had a growth triggering effect, while BABA application at 100, 150 mM had a decreasing effect on capsules per plant by 13.2% and 3.8%, 5.8%, respectively, as compared to the control condition. Similarly, 50 and 100 mM of BABA application increased the number of seeds per capsule by 5.7% and 5.7%, while 150 mM of BABA application decreased this trait by 1.4% as compared to the control conditions (Table 4). The individual effect of SM25 and SM45 decreased the weight of 100-seeds and also seed yield by 32.2%, 11.9% and 51.9%, 25.8%, respectively, as compared to SM65. But, application of BABA (50, 100 and 150 mM) mitigated the effect of DS by improving 100-seed weight and seed yield per plant by 15.2%, 10.9%, 10.9% and 37.5%, 9.2%, 3.2%, respectively, as compared to the control conditions (Table 4).
3.2 Foliar Application of BABA Enhanced Drought Atress Tolerance in Linseeds
The results pertaining to the individual effect of irrigation regimes (SM25 and SM45) revealed that these remained effective in decreasing the days to flowering and harvesting by 8.23%, 4.53% and 3.73%, 2.04%, respectively, in comparison to the SM65. The application of BABA (50, 100 and 150 mM) ameliorated the adverse effects of DS by increasing the days to flowering by 1.01%, 2.89% and 0% as compared to the control conditions. However, application of BABA (50 and 100 mM) also increased the days to harvesting by 0.72% and 1.44%, while 150 mM of BABA had 0.43% negative effect on days to harvesting as compared to the control conditions (Table 2).
The moisture regimes of SM25 and SM45 reduced the number of branches per plant and plant height by 18.3%, 7.6% and 15.6%, 5.1%, respectively, as compared to SM65. Foliar application of BABA (50, 100 and 150 mM) produced taller plants by 1.25%, 4.38% and 0.38%, respectively compared to the control treatment (Table 2). In terms of individual effect, SM25 and SM45 reduced the shoot dry weight and root dry weight by 31.2%, 18.0% and 42.5%, 24.0%, respectively, as compared to SM65. The application of BABA (50, 100 and 150 mM) triggered the shoot dry weight and root dry weight by 13.7%, 31.7%, 4.7% and 14.2%, 32.1%, 4.7%, respectively, as compared to the control conditions (Table 3). The number of capsules per plant and seeds per capsule were reduced by 26.1%, 11.1% and 7.9%, 4.9% in response to SM25 and SM45 compared to SM65. Only 100 mM of BABA application increased the number of capsules per plant by 12.4%, while 50 and 150 mM concentrations of BABA decreased the number of capsules per plant by 6.0% and 5.2%, respectively compared to the SM65. Similarly, the number of seeds per capsules were increased by 2.7% and 5.5% with 50 and 100 mM of BABA application, while 150 mM of BABA application decreased the number of seeds per capsule (1.5%) as compared to control treatment (Table 4).
The individual effect of irrigation regimes SM25 and SM45 decreased 100-seed weight and seed yield per plant by 32.3%, 12.9% and 52.1%, 24.9%, respectively, as compared to the SM65. The application of BABA (50, 100 and 150 mM) mitigated DS by enhancing 100-seed weight and seed yield per plant by 12.5%, 14.6%, 10.4% and 8.1%, 36.3%, 3.8%, respectively, as compared to the control condition (Table 4).
3.3 Comparison the Effect of Seed Priming and Foliar Application of BABA
The results of comparative study indicated that foliar application of BABA showed better performances for all the growth, yield and antioxidants parameters except for days to flowering, H2O2 and CAT content compared to the seed priming with BABA application (Table 5; Figs. 1 and 2). The maximum days to flowering in response to seed priming (73.9) and foliar spray 2 (73.47) were found for BABA concentration of 50 and 100 mM, respectively under SM65 (Table 5). The maximum number of branches and the seed yield per plant (4.00 cm and 5.29 g, respectively) were recorded following the application of 50 mM of BABA under SM65 (Table 5).
Figure 1: Interactive study of irrigation regime (FC, feld capacity) and β-aminobutyric acid (BABA) on the Proline (μM g−1 FW), Melondialdehyde (MDA; μM g−1 FW) and Hydrogen Peroxide (H2O2; μM g−1 FW) of linseed in Experiment 1 (seed priming) and Experiment 2 (foliar application) at p < 0.05. SM25, FC-40% = 25% soil moisture; SM45, FC-60% = 45% soil moisture; SM65, FC-80% = 65% soil moisture
Figure 2: Interactive study of irrigation regime (FC, field capacity) and β-aminobutyric acid (BABA) on the catalase (CAT; μM g−1 FW), superoxide dismutase (SOD; μM g−1 FW), Peroxidase (POD; μM g−1 FW) and ascorbate peroxidase (APX; μM g−1 FW) of linseed in Experiment 1 (seed priming) and Experiment 2 (foliar application) at p < 0.05. SM25, FC-40% = 25% soil moisture; SM45, FC-60% = 45%
For seed priming, the interaction effect of FC and BABA showed that of the maximum content of proline (145.98%), MDA (195.36%) and H2O2 (114.17%) were recorded especially by BABA (0 mM) under SM25 as compared to the SM65 (Fig. 1).
Contrastingly, the maximum content of CAT, SOD and POD were produced by BABA (150 mM) at SM25 which were 165.55%, 125.60% and 126.57%, respectively, than non-primed linseed seeds under SM65%. Contrastingly, seed priming with BABA (50 mM) under DS significantly increased the activity of APX (70.63%) in comparison to non-primed seeds under SM65 (Fig. 2). Likewise, foliar spray also showed significant enhancement of antioxidant activity in linseed crop under DS condition. The interactive impact of FC and BABA showed that the maximum proline (131.00%), MDA (114.24%) and H2O2 (51.29%) was identificed in seed sample that primed with BABA (0 mM) under FC-40% as compared to the SM65 (Fig. 1). In contrast, the increment in the activity of CAT, SOD, POD and APX was also observed for the 150 mM concentration of BABA in the presence of SM25 which was 105.80%, 114.58%, 125.43% and 55.67%, respectively as compared to 0 mM dose of BABA applied under SM65 (Fig. 2).
Drought stress (DS) is one of the most serious challenges to the agricultural crop cultivation including linseed crops. Growth and yield are critical morphological parameters of linseed plants which are seriously reduced by DS [46]. The incidence of DS at critical growth stages (seedling, flowering, grain development, etc.) significantly reduces the seed yield of linseed [47,48]. The negative impacts of DS on the vegetative growth characteristics of linseed also hinder the progression of its reproductive growth life cycle [49]. However, this current study explored that the application of 50–100 mM of BABA as seed priming agent and exogenous foliar application on linseed improved the days to flowering (Table 5). Our results matched with the study of Ragia [28] whereby foliar application of BABA increased the growth and flower parameters and minimized the adverse effect of water deficit. Under DS condition, there was a significant decrease in the time to flowering (days to anthesis) while promoting flower appearance compared to the normal irrigation regime. The present study also revealed a gradual reduction in plant height and the number of branches per plant in linseed under water deficit conditions compared to normal irrigation. The diminished growth of plants under drought stress can be attributed to the inhibition of photosynthesis and a decrease in photosynthetic efficiency, ultimately disrupting the balance between source and sink relationship [50]. Moreover this study implies that the application of 50–100 mM of BABA mitigated the adverse effect of low moisture stress on linseed and these findings are consistent with Quéro et al. [51].
Shoot and root dry weights were pronouncedly influenced by DS as per results of our study, which might be attributed to limited supply of carbohydrates to sinks from leaves owing to disruption of photosynthetic process [52,53]. Reduction of water availability due to drought is obvious that leads to inhibition of plant root growth, resulting the transportation of water and minerals in several parts of plants, plant reclines of stomatal functioning and transpiration. This adverse effect can be least by the action of stress hormones abscisic acid (ABA) [54]. Likewise, application of BABA in different concentrations enhanced the ABA concentration in plant [55]. Water deficit negatively affected the crop production (Table 5) but the foliar application of BABA mitigated the detrimental effects [56]. However, our current study implies that the performance of physiological parameters were improved due to supplementation of BABA in linseed plants. This implies that root volume and nutrient uptake known to decrease with water deficit improved with BABA application. Further, BABA leads to accumulate several metabolites in plants and protects plants from stress signals, and this is useful when a plant is experiencing water deficit. The metabolites recover the gradient important for water uptake and thus protect the plant from drought stress [51].
Yield and yield-related parameters of different linseed genotypes were influenced differently by varying levels of DS as adverse effects of DS depend on plant species, time of incidence, severity and duration of DS, etc. [48]. With water deficit conditions, the number of capsules per plant, number of seeds, 1000-seeds weight, plant height, the number of branches per plant and seed yield declined as compared to normal irrigation [46]. Imposing low moisture stress prior to the anthesis stage was found to have a detrimental effect on physiological and yield characteristics. One of the main factors contributing to reduced seed weight is the shortened time to flowering that caused by drought stress. There is typically a negative correlation between seed yield per plant and the duration of flowering and maturity. Additionally, the number of capsules per plant is often associated with the total number of branches, while growth traits directly or indirectly influence yield traits in the production of linseed [49]. The DS was reported to involve serious reduction of grains number which is a valuable attribute at reproductive growth stages of crop plants [55]. The application of BABA promotes abscisic acid (ABA) accumulation in plants and improves the plant defense mechanism and yield parameters [55]. Foliar applied BABA enhanced 45% grain yield compared with the control (no application). Water stress and foliar application of BABA had a prominent effect on yield traits, but the interactive study showed non-significant results. Low moisture stress at the vegetative stage can affect photosynthetic pigments in plants and decline crop yields [13]. Higher accumulation of osmolytes such as proline, soluble sugar lead to enhance plant adaptation under [55,57]. Similarly, in this study 50 mM BABA as seed primed and 100 mM of foliar-applied BABA promoted the yield and yield-related parameters of linseed and minimized the adverse effect of DS (Tables 5). This observation is in agreement with previous findings, where a higher concentration of BABA was found to effectively preserve green pigments and support the nutrient cycle in plants, in contrast to lower doses of BABA [55,57].
Several osmolites such as proline, soluble sugar, glycine betaine, which help to plant adaptation under stressful conditions [58–60]. Proline is of the significant osmolites that level found to be induced even in drought stress and other oxidative that enhance plant fitness and adaptation [61]. The exogenous application of neurotransmitters alleviates oxidative stress-induced antioxidant enzyme activity in plants [62,63]. In this study, the improvement of plants attributes through exogenous supplementation BABA is newly reported in linseed. Additionally, BABA decreased the MDA content in linseed plants exposed to varying levels of DS This decrease in MDA level protect from membrane disruption [64]. Increase level of MDA and H2O2 was found in salt stressed plants [65,66]. The salt, drought and other abiotic stresses involved in inducing H2O2 accumulation as well as lipid peroxidation, which leads to enhance MDA concentration. As a consequence of these stress indicators several cellular difficulties occurs like membrane injury and reduction of membrane stability index [65]. The interactive study of BABA and low moisture stress showing the significant results of MDA and H2O2. In short MDA and H2O2 enhanced under low moisture stress condition [56]. However, our current study implies that the application of BABA lowers the MDA concentration [65] as well as H2O2 in linseed plant (Fig. 1).
The concentrations of MDA, H2O2, CAT, SOD and POD in lentil were enhanced under DS compared to well-watered conditions [67–69]. It is highly documented that elevation of antioxidant enzymes under stress significantly help to enhance stress tolerance in plants [70,71]. In this study, the enhancement of CAT, SOD and POD enzymes activity revealed that BABA is fully active at SM25 in linseed, which were also reported to be effective against ROS and helped plants in minimizing oxidative damage under DS conditions [72,73]. Application of BABA treatments on improved biosynthesis of CAT, SOD and POD antioxidants and decreased levels of H2O2 served as vital factor in protecting the membrane structure and ultimately plants survival was increased under severe DS conditions. Moreover, the BABA application on plants aided in scavenging ROS which might be attributed for improved number of seeds per capsule and higher 100-seeds weight of linseed [70,71,74].
From these findings it is confirmed that both methods (seed priming and foliar application) of BABA application are efficient in improving growth and production of linseed, hence the variations in environmental conditions, and stress levels may account for the divergent application methods and concentrations of BABA for different methods of application for achieving maximum potential.
This study implies that BABA mediating seed priming and foliar application significantly mitigates DS in linseed. The BABA significantly improved DS by regulating morpho-agrological, and physiological attributes, which enhanced plant adaptation in linseed under DS. This study also disclosed that exogenously applied BABA was fully active to enhance the antioxidant enzymes activity under moisture stress conditions which enabled crop plants to off-set the adverse effects of DS. These findings suggested that BABA was active at SM25 in linseed that further supported to mitigate oxidative stress indicators (MDA and H2O2). Moreover, the DS efficiency was dose dependent manner, seed priming and foliar application of BABA (50–100 µM) showed better performance under SM25, while high dose of BABA (150 µM) significantly inhibited plant growth, adaptation and yield performance in linseed. These findings might be useful to linseed growers and farmers for linseed breeding as well as mitigating the adverse effects of DS in linseed and other oil seed crops.
Acknowledgement: The authors extend their appreciation to the Researchers Supporting Project Number (RSP2023R298), King Saud University, Riyadh, Saudi Arabia.
Funding Statement: The authors extend their appreciation to the Researchers Supporting Project Number (RSP2023R298), King Saud University, Riyadh, Saudi Arabia.
Author Contributions: TAY, AW and MH: designing of experiment. MA, NS, MA, KM and MA: data collection and experiment management. TAY, MA: analysis and interpretation of data. MA, TAY and AW: manuscript preparation. MA and MAI: preparation of figures and tables. CCO, IAA, MAR and AES: reviewing and editing of manuscript.
Availability of Data and Materials: All obtained data is enclosed with this manuscript.
Ethics Approval: Not Applicable.
Conflicts of Interest: The authors declare that they have no conflicts of interest to report regarding the present study.
References
1. Shivaraj, S. M., Dhakate, P., Sonah, H., Vuong, T., Nguyen, H. T. et al. (2019). Progress toward development of climate-smart flax: A perspective on omics-assisted breeding. In: Genomic designing of climate-smart oilseed crops, pp. 239–274. Cham: Springer. [Google Scholar]
2. Singh, S. B., Marker, S. (2006). Linseed: A plant with many uses. Agrobios Newsletter, 5(2), 13. [Google Scholar]
3. Praczyk, M., Wielgusz, K. (2021). Agronomic assessment of fibrous flax and linseed advanced breeding lines as potential new varieties. Agronomy, 11(10), 1917. [Google Scholar]
4. Shim, Y. Y., Gui, B., Wang, Y., Reaney, M. J. (2015). Flaxseed (Linum usitatissimum L.) oil processing and selected products. Trends in Food Science & Technology, 43(2), 162–177. [Google Scholar]
5. Ganvit, J. B., Sharma, S., Surve, V. H., Ganvit, V. C. (2019). Effect of sowing dates and crop spacing on growth, yield and quality of linseed under south Gujarat condition. Journal of Pharmacognosy and Phytochemistry, 8(1), 388–392. [Google Scholar]
6. El-Beltagi, H. S., Salama, Z. A., El-Hariri, D. M. (2007). Evaluation of fatty acids profile and the content of some secondary metabolites in seeds of different flax cultivars (Linum usitatissimum L.). General and Applied Plant Physiology, 33(3–4), 187–202. [Google Scholar]
7. David, L. A., Toms, B. (2006). Plant for a future: Linseed. Agrobios Newsletter, 4(12), 38–39. [Google Scholar]
8. Chauhan, M. P., Singh, S., Singh, A. K. (2009). Post harvest uses of linseed. Journal of Human Ecology, 28(3), 217–219. [Google Scholar]
9. Al-Okbi, S. Y., Mohamed, D. A., Kandil, E., Abo-Zeid, M. A., Mohammed, S. E. et al. (2017). Anti-inflammatory activity of two varieties of pumpkin seed oil in an adjuvant arthritis model in rats. Grasas y Aceites, 68(1), e180. [Google Scholar]
10. Ansari, A., Razmjoo, J., Karimmojeni, H. (2016). Mycorrhizal colonization and seed treatment with salicylic acid to improve physiological traits and tolerance of flaxseed (Linum usitatissimum L.) plants grown under drought stress. Acta Physiologiae Plantarum, 38, 1–10. [Google Scholar]
11. Sehgal, A., Sita, K., Siddique, K. H., Kumar, R., Bhogireddy, S. et al. (2018). Drought or/and heat-stress effects on seed filling in food crops: Impacts on functional biochemistry, seed yields, and nutritional quality. Frontiers in Plant Science, 9, 1705. [Google Scholar] [PubMed]
12. Wasaya, A., Rehman, I., Mohi Ud Din, A., Hayder Bin Khalid, M., Ahmad, Y. T. et al. (2023). Foliar application of putrescine alleviates terminal drought stress by modulating water status, membrane stability, and yield- related traits in wheat (Triticum aestivum L.). Frontiers in Plant Science, 13, 1000877. https://doi.org/10.3389/fpls.2022.1000877 [Google Scholar] [PubMed] [CrossRef]
13. Wang, J. Y., Turner, N. C., Liu, Y. X., Siddique, K. H., Xiong, Y. C. (2016). Effects of drought stress on morphological, physiological and biochemical characteristics of wheat species differing in ploidy level. Functional Plant Biology, 44(2), 219–234. [Google Scholar]
14. Ahmad, Z., Anjum, S., Skalicky, M., Waraich, E. A., Muhammad Sabir Tariq, R. et al. (2021). Selenium alleviates the adverse effect of drought in oilseed crops camelina (Camelina sativa L.) and canola (Brassica napus L.). Molecules, 26(6), 1699. [Google Scholar] [PubMed]
15. Latif, M., Akram, N. A., Ashraf, M. (2016). Regulation of some biochemical attributes in drought-stressed cauliflower (Brassica oleracea L.) by seed pre-treatment with ascorbic acid. The Journal of Horticultural Science and Biotechnology, 91(2), 129–137. [Google Scholar]
16. La, V. H., Lee, B. R., Zhang, Q., Park, S. H., Islam, M. T. et al. (2019). Salicylic acid improves drought-stress tolerance by regulating the redox status and proline metabolism in Brassica rapa. Horticulture, Environment, and Biotechnology, 60, 31–40. [Google Scholar]
17. Sadak, M. S., Bakry, B. A. (2020). Alleviation of drought stress by melatonin foliar treatment on two flax varieties under sandy soil. Physiology and Molecular Biology of Plants, 26(5), 907–919. [Google Scholar] [PubMed]
18. Nemat Alla, M. M., Abogadallah, G. M., Badran, E. G., Nada, R. M. (2014). Differential tolerance of two wheat cultivars to NaCl is related to antioxidant potentialities. Brazilian Journal of Botany, 37(3), 207–215. [Google Scholar]
19. Foyer, C. H., Theodoulou, F. L., Delrot, S. (2001). The functions of inter-and intracellular glutathione transport systems in plants. Trends in Plant Science, 6(10), 486–492. [Google Scholar] [PubMed]
20. Nemat Alla, M. M., Hassan, N. M., El-Bastawisy, Z. M. (2008). Changes in antioxidants and kinetics of glutathione-S-transferase of maize in response to isoproturon treatment. Plant Biosystems, 142(1), 5–16. [Google Scholar]
21. Hassan, N., Ebeed, H., Aljaarany, A. (2020). Exogenous application of spermine and putrescine mitigate adversities of drought stress in wheat by protecting membranes and chloroplast ultra-structure. Physiology and Molecular Biology of Plants, 26(2), 233–245. [Google Scholar] [PubMed]
22. Ma, X. H., Xu, J. Y., Han, D., Huang, W. X., Dang, B. J. et al. (2020). Combination of β-aminobutyric acid and Ca2+ alleviates chilling stress in tobacco (Nicotiana tabacum L.). Frontiers in Plant Science, 11, 556. [Google Scholar] [PubMed]
23. Shaw, A. K., Bhardwaj, P. K., Ghosh, S., Roy, S., Saha, S. et al. (2016). β-aminobutyric acid mediated drought stress alleviation in maize (Zea mays L.). Environmental Science and Pollution Research, 23, 2437–2453. [Google Scholar] [PubMed]
24. Hossain, Z., Makino, T., Komatsu, S. (2012). Proteomic study of β-aminobutyric acid-mediated cadmium stress alleviation in soybean. Journal of Proteomics, 75(13), 4151–4164. [Google Scholar] [PubMed]
25. Yin, X., Liu, S., Qin, Y., Xing, R., Li, K. et al. (2021). Metabonomics analysis of drought resistance of wheat seedlings induced by β-aminobutyric acid-modified chitooligosaccharide derivative. Carbohydrate Polymers, 272, 118437. [Google Scholar] [PubMed]
26. Yao, L., Zhong, Y., Wang, B., Yan, J., Wu, T. (2020). BABA application improves soybean resistance to aphid through activation of phenylpropanoid metabolism and callose deposition. Pest Management Science, 76(1), 384–394. [Google Scholar] [PubMed]
27. Jafarbeigi, F., Samih, M. A., Alaei, H., Shirani, H. (2020). Induced tomato resistance against Bemisia tabaci triggered by salicylic acid, β-aminobutyric acid, and Trichoderma. Neotropical Entomology, 49, 456–467. [Google Scholar] [PubMed]
28. Ragia, M. M. (2019). Application of β-aminobutyric acid and spermidine raise drought tolerance and alleviate oxidative stress in potted chrysanthemum. Alexandria Journal of Agricultural Sciences, 64(3), 155–164. [Google Scholar]
29. Abid, G., Ouertani, R. N., Jebara, S. H., Boubakri, H., Muhovski, Y. et al. (2020). Alleviation of drought stress in faba bean (Vicia faba L.) by exogenous application of β-aminobutyric acid (BABA). Physiology and Molecular Biology of Plants, 26, 1173–1186. [Google Scholar] [PubMed]
30. Jisha, K. C., Puthur, J. T. (2016). Seed priming with beta-amino butyric acid improves abiotic stress tolerance in rice seedlings. Rice Science, 23(5), 242–254. [Google Scholar]
31. Farooq, M., Usman, M., Nadeem, F., ur Rehman, H., Wahid, A. et al. (2019). Seed priming in field crops: Potential benefits, adoption and challenges. Crop and Pasture Science, 70(9), 731–771. [Google Scholar]
32. Abdel-Aziz, H. M. M., Hasaneen, M. N. A., Omer, A. M. (2019). Impact of engineered nanomaterials either alone or loaded with NPK on growth and productivity of French bean plants: Seed priming vs foliar application. South African Journal of Botany, 125, 102–108. [Google Scholar]
33. Sharifi, R., Mohammadi, K., Rokhzadi, A. (2016). Effect of seed priming and foliar application with micronutrients on quality of forage corn (Zea mays). Environmental & Experimental Biology, 14(4), 151–156. [Google Scholar]
34. Maswada, H. F., Djanaguiraman, M., Prasad, P. V. V. (2018). Seed treatment with nano-iron (III) oxide enhances germination, seeding growth and salinity tolerance of sorghum. Journal of Agronomy and Crop Science, 204(6), 577–587. [Google Scholar]
35. He, F., Sheng, M., Tang, M. (2017). Effects of Rhizophagus irregularis on photosynthesis and antioxidative enzymatic system in Robinia pseudoacacia L. under drought stress. Frontiers in Plant Science, 8, 183. [Google Scholar] [PubMed]
36. Smith, J. M., Froment, M. A. (1998). A growth stage key for winter linseed (Linum usitatissimum). Annals of Applied Biology, 133(2), 297–306. [Google Scholar]
37. Mahmud, J. A., Hasanuzzaman, M., Khan, M. I. R., Nahar, K., Fujita, M. (2020). β-Aminobutyric acid pretreatment confers salt stress tolerance in Brassica napus L. by modulating reactive oxygen species metabolism and methylglyoxal detoxification. Plants, 9(2), 241. [Google Scholar] [PubMed]
38. Chakma, R., Biswas, A., Saekong, P., Ullah, H., Datta, A. (2021). Foliar application and seed priming of salicylic acid affect growth, fruit yield, and quality of grape tomato under drought stress. Scientia Horticulturae, 280, 109904. [Google Scholar]
39. Rokade, B. S., Kamble, P. S., Jadhav, J. D., Madane, K. T. (2015). Crop growth rate, leaf area index as affected by sowing dates in linseed (Linum usitatissimum L.). Advance Research Journal of Crop Improvement, 6(1), 39–42. [Google Scholar]
40. Fallah, M., Hadi, H., Amirnia, R., Hassanzadeh-Ghorttapeh, A., Zuan, A. T. K. et al. (2021). Eco-friendly soil amendments improve growth, antioxidant activities, and root colonization in lingrain (Linum Usitatissimum L.) under drought conditions. PLoS One, 16(12), e0261225. [Google Scholar] [PubMed]
41. Heath, R. L., Packer, L. (1968). Photoperoxidation in isolated chloroplasts: I. Kinetics and stoichiometry of fatty acid peroxidation. Archives of Biochemistry and Biophysics, 125(1), 189–198. [Google Scholar] [PubMed]
42. Velikova, V., Yordanov, I., Edreva, A. J. P. S. (2000). Oxidative stress and some antioxidant systems in acid rain-treated bean plants: Protective role of exogenous polyamines. Plant Science, 151(1), 59–66. [Google Scholar]
43. Maehly, A., Chance, B. (1954). Catalases and peroxidases. Methods of Biochemical Analysis, 1, 357–424. [Google Scholar] [PubMed]
44. Dhindsa, R. S., Plumb-Dhindsa, P. A. M. E. L. A., Thorpe, T. A. (1981). Leaf senescence: Correlated with increased levels of membrane permeability and lipid peroxidation, and decreased levels of superoxide dismutase and catalase. Journal of Experimental Botany, 32(1), 93–101. [Google Scholar]
45. Chen, G. X., Asada, K. (1989). Ascorbate peroxidase in tea leaves: Occurrence of two isozymes and the differences in their enzymatic and molecular properties. Plant and Cell Physiology, 30(7), 987–998. [Google Scholar]
46. Aghdam, M. T. B., Mohammadi, H., Ghorbanpour, M. (2016). Effects of nanoparticulate anatase titanium dioxide on physiological and biochemical performance of Linum usitatissimum (Linaceae) under well-watered and drought stress conditions. Brazilian Journal of Botany, 39, 139–146. [Google Scholar]
47. Gaju, O., Reynolds, M. P., Sparkes, D. L., Foulkes, M. J. (2009). Relationships between large-spike phenotype, grain number, and yield potential in spring wheat. Crop Science, 49(3), 961–973. [Google Scholar]
48. Abdel-Moneam, M. A., Sultan, M. S., Seadh, S. E., El-Metwally, L. I. (2021). Combining ability and heterosis for earliness, growth and biological yield of some flax genotypes at normal-irrigation and water-shortage environments. Journal of Plant Production, 12(8), 899–907. [Google Scholar]
49. Adugna, W., Labuschagne, M. T. (2003). Association of linseed characters and its variability in different environments. The Journal of Agricultural Science, 140(3), 285–296. [Google Scholar]
50. Ripoll, J., Urban, L., Bertin, N. (2016). The potential of the MAGIC TOM parental accessions to explore the genetic variability in tomato acclimation to repeated cycles of water deficit and recovery. Frontiers in Plant Science, 6, 1172. [Google Scholar] [PubMed]
51. Quéro, A., Fliniaux, O., Elboutachfaiti, R., Petit, E., Guillot, X. et al. (2015). β-Aminobutyric acid increases drought tolerance and reorganizes solute content and water homeostasis in flax (Linum usitatissimum). Metabolomics, 11, 1363–1375. [Google Scholar]
52. Bulgari, R., Cocetta, G., Trivellini, A., Vernieri, P. A. O. L. O., Ferrante, A. (2015). Biostimulants and crop responses: A review. Biological Agriculture & Horticulture, 31(1), 1–17. [Google Scholar]
53. Movahhedi-Dehnavi, M., Behzadi, Y., Niknam, N., Mohtashami, R. (2019). Salicylic acid mitigates the effects of drought and salinity on nutrient and dry matter accumulation of Linseed. Journal of Plant Process and Function, 8(31), 31–44. [Google Scholar]
54. Wilkinson, S., Davies, W. J. (2002). ABA-based chemical signalling: The co-ordination of responses to stress in plants. Plant, Cell & Environment, 25(2), 195–210. [Google Scholar]
55. Du, Y. L., Wang, Z. Y., Fan, J. W., Turner, N. C., Wang, T. et al. (2012). β-Aminobutyric acid increases abscisic acid accumulation and desiccation tolerance and decreases water use but fails to improve grain yield in two spring wheat cultivars under soil drying. Journal of Experimental Botany, 63(13), 4849–4860. [Google Scholar] [PubMed]
56. Javadi, T., Rohollahi, D., Ghaderi, N., Nazari, F. (2017). Mitigating the adverse effects of drought stress on the morpho-physiological traits and anti-oxidative enzyme activities of Prunus avium through β-amino butyric acid drenching. Scientia Horticulturae, 218, 156–163. [Google Scholar]
57. Hussain, H. A., Men, S., Hussain, S., Chen, Y., Ali, S. et al. (2019). Interactive effects of drought and heat stresses on morpho-physiological attributes, yield, nutrient uptake and oxidative status in maize hybrids. Scientific Reports, 9(1), 3890. [Google Scholar] [PubMed]
58. Harinasut, P., Srisunak, S., Pitukchaisopol, S., Charoensataporn, R. (2000). Mechanisms of adaptation to increasing salinity of mulberry: Proline content and ascorbate peroxidase activity in leaves of multiple shoots. ScienceAsia, 26, 207–211. [Google Scholar]
59. Rahman, M. A., Yong-Goo, K., Iftekhar, A., Liu, G. S., Hyoshin, L. et al. (2016). Proteome analysis of alfalfa roots in response to water deficit stress. Journal of Integrative Agriculture, 15(6), 1275–1285. [Google Scholar]
60. Rahman, M. A., Alam, I., Kim, Y. G., Ahn, N. Y., Heo, S. H. et al. (2015). Screening for salt-responsive proteins in two contrasting alfalfa cultivars using a comparative proteome approach. Plant Physiology and Biochemistry, 89(12), 112–122. [Google Scholar] [PubMed]
61. Bakry, B. A., El-Hariri, D. M., Sadak, M. S., El-Bassiouny, H. M. S. (2012). Drought stress mitigation by foliar application of salicylic acid in two linseed varieties grown under newly reclaimed sandy soil. Journal of Applied Sciences Research, 8(7), 3503–3514. [Google Scholar]
62. Raza, A., Charagh, S., García-Caparrós, P., Rahman, M. A., Ogwugwa, V. H. et al. (2022). Melatonin-mediated temperature stress tolerance in plants. GM Crops & Food, 13(1), 196–217. [Google Scholar]
63. Raza, A., Salehi, H., Rahman, M. A., Zahid, Z., Madadkar Haghjou, M. et al. (2022). Plant hormones and neurotransmitter interactions mediate antioxidant defenses under induced oxidative stress in plants. Frontiers in Plant Science, 13, 961872. [Google Scholar] [PubMed]
64. Rahman, M. A., Kabir, A. H., Mandal, A., Roy, S. K., Song, Y. et al. (2020). Glutathione restores Hg-induced morpho-physiological retardations by inducing phytochelatin and oxidative defense in alfalfa. Biology, 9(11), 364. [Google Scholar] [PubMed]
65. Ali, E. F., Hassan, F. A. S. (2018). β-Aminobutyric acid raises salt tolerance and reorganises some physiological characters in Calendula officinalis L. plant. Annual Research & Review in Biology, 30(5), 1–16. [Google Scholar]
66. Rahman, M. A., Alam, I., Sharmin, S. A., Kabir, A. H., Kim, Y. G. et al. (2021). Physiological and proteomic analyses reveal the protective roles of exogenous hydrogen peroxide in alleviating drought stress in soybean plants. Plant Biotechnology Reports, 15(6), 805–818. [Google Scholar]
67. Yasir, T. A., Khan, A., Skalicky, M., Wasaya, A., Rehmani, M. I. A. et al. (2021). Exogenous sodium nitroprusside mitigates salt stress in lentil (Lens culinaris Medik.) by affecting the growth, yield, and biochemical properties. Molecules, 26(9), 2576. [Google Scholar] [PubMed]
68. Rahman, M. A., Woo, J. H., Lee, S. H., Park, H. S., Kabir, A. H. et al. (2022). Regulation of Na+/H+ exchangers, Na+/K+ transporters, and lignin biosynthesis genes, along with lignin accumulation, sodium extrusion, and antioxidant defense, confers salt tolerance in alfalfa. Frontiers in Plant Science, 13, 1041764. [Google Scholar] [PubMed]
69. Singh, D., Sharma, N. L., Singh, D., Siddiqui, M. H., Taunk, J. et al. (2023). Exogenous hydrogen sulfide alleviates chromium toxicity by modulating chromium, nutrients and reactive oxygen species accumulation, and antioxidant defence system in mungbean (Vigna radiata L.) seedlings. Plant Physiology and Biochemistry, 200, 107767. [Google Scholar] [PubMed]
70. Li, S., Tan, H. Y., Wang, N., Zhang, Z. J., Lao, L. et al. (2015). The role of oxidative stress and antioxidants in liver diseases. International Journal of Molecular Sciences, 16(11), 26087–26124. [Google Scholar] [PubMed]
71. Foyer, C. H., Fletcher, J. M. (2001). Plant antioxidants: Colour me healthy. Biologist, 48(3), 115–120. [Google Scholar] [PubMed]
72. Ju, Y. H., Roy, S. K., Roy Choudhury, A., Kwon, S. J., Choi, J. Y. et al. (2021). Proteome changes reveal the protective roles of exogenous citric acid in alleviating Cu toxicity in Brassica napus L. International Journal of Molecular Sciences, 22(11), 5879. [Google Scholar] [PubMed]
73. Wasaya, A., Affan, M., Ahmad Yasir, T., Mubeen, K., Rehman, H. U. et al. (2021). Foliar potassium sulfate application improved photosynthetic characteristics, water relations and seedling growth of drought-stressed maize. Atmosphere, 12(6), 663. [Google Scholar]
74. Batool, A., Cheng, Z. G., Akram, N. A., Lv, G. C., Xiong, J. L. et al. (2019). Partial and full root-zone drought stresses account for differentiate root-sourced signal and yield formation in primitive wheat. Plant Methods, 15(1), 1–14. [Google Scholar]
Cite This Article
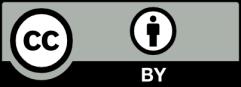