Open Access
ARTICLE
Arbuscular Mycorrhizal Fungi Alleviates Salt-Alkali Stress Demage on Syneilesis aconitifolia
College of Landscape Architecture, Northeast Forestry University, Harbin, 150040, China
* Corresponding Author: Chunxue Yang. Email:
Phyton-International Journal of Experimental Botany 2023, 92(12), 3195-3209. https://doi.org/10.32604/phyton.2023.043049
Received 20 June 2023; Accepted 07 September 2023; Issue published 28 December 2023
Abstract
Syneilesis aconitifolia is a potential ground cover and decorative material in gardens, which exhibits a strong salt-alkali tolerance, and also has medicinal value. In this study, the arbuscular mycorrhizal (AM) fungi community in the soil surrounding S. aconitifolia roots in the Songnen saline-alkali grassland was used as the inoculation medium for a pot cultivation experiment. After normal culture for 90 days, NaCl and NaHCO3 solutions were applied to subject plants to salt or alkali stress. Solution concentrations of 50, 100, and 200 mmol/L were applied for 10 days, and mycorrhizal colonization, biomass, relative water content (RWC), chlorophyll concentration, malondialdehyde (MDA) concentration, antioxidant system activity, and osmomodulator concentration were determined to identify the effects of AM fungi on root colonization status and salinity tolerance in S. aconitifolia. There were three key results. (1) Compared to the controls, the intensity and rate of colonization decreased under saline-alkali stress, and the adaptability of AM fungi under low concentration alkali stress was higher than that under salt stress. (2) The AM fungi could increase the biomass, RWC, and chlorophyll concentration, and decrease the MDA concentration of S. aconitifolia to some extent. With an increase in the salt or alkali solution concentration, AM fungi not only upregulated the activity of the antioxidant system, but also increased the concentration of osmotic regulatory substances. (3) A multivariate analysis of variance (ANOVA) and radar map analysis showed that the mechanisms of resistance to salt and alkali stress were not the same in S. aconitifolia. In the salt treatment, AM fungi mainly regulated salt stress through osmotic regulatory substances such as soluble sugars, soluble proteins, and proline. In the alkali treatment, AM fungi mainly regulated alkali stress through glutathione (GSH), soluble sugars, and MDA. The results showed that the colonization rate of S. aconitifolia under low concentration alkali stress was higher than that under salt stress, and the inoculation of AM fungi could significantly reduce the MDA concentration of S. aconitifolia plants under salinity and alkali stress, and improve the antioxidant enzyme activity and osmoregulatory substance accumulation, thereby improving the salinity tolerance of S. aconitifolia.Keywords
Supplementary Material
Supplementary Material FileSoil salinization can restrict agricultural development and has become a global environmental problem [1]. As the rapid development of the global economy has intensified the demand for land, increasing attention has been given to saline-alkali soils, which account for 7% of the world’s land area [2]. There are several mechanisms by which saline-alkali soil restricts plant growth. Saline-alkali stress will lead to an increase in soil osmotic pressure, resulting in plant roots being unable to absorb water and mineral elements from the soil, leading to osmotic stress. This will also produce a large amount of reactive oxygen species (ROS) in the plant, causing damage to the plant cell membrane system, and thereby affecting the physiological and biochemical reactions in the cells [3–5]. Alkaline soil can do more damage to plants than a saline soil because of its higher pH, which can affect the ion balance and nutrient absorption [6]. To overcome the harm caused by salinity-alkali stress, plants will also carry out a series of mechanistic adjustments to maintain normal growth, such as the accumulation of osmoregulatory substances, the enhancement of antioxidant systems, and the transport of numerous toxic ions to the vacuoles [7–10].
Arbuscular mycorrhizal (AM) fungi are widely found in a variety of natural environments and farmland ecosystems, including salt-soil habitats [11], alpine grasslands [12], and desert areas [13]. Arbuscular mycorrhizal (AM) fungi are soil’s most important beneficial microorganisms and coexist with most plants worldwide [14,15]. The symbiosis between AM fungi and plants can increase the antioxidant enzyme activity and the content of osmoregulatory substances in host plants, thus enhancing the salt tolerance and drought tolerance of host plants [16,17]. At the same time, AM fungi also play an important role in regulating the nutrient elements of plants, improving the root crown ratio of host plants, and enhancing plant photosynthesis [18,19]. There are also abundant fungal resources in saline-alkali soils [20,21]. It has been reported that a mixed inoculation of AM fungi is more beneficial to host plants than a single inoculation of AM fungi, and local AM fungi have a more significant effect on the growth of host plants [22]. Therefore, in this study, we used AM fungi in the root perimeter soil of S. aconitifolia in Songnen salt-alkali grassland to inoculate S. aconitifolia in a pot experiment and investigate the effect of a local AM fungal inoculation on the host plants.
Syneilesis aconitifolia is a perennial herb of the Compositae family. It is widely used as a Chinese herbal medicine because it contains various active ingredients such as alkaloids and terpenoids, and the whole plant is considered to have medicinal properties [23–25]. It does not have a high medicinal value but is a commonly cultivated plant in urban landscaping because of its peculiar plant type and lush flowering ability. During the investigation of the saline-alkali grassland in Songnen, it was found that S. aconitifolia was unevenly distributed in the area, with dense vegetation and good growth state, which inferred that S. aconitifolia had a certain degree of salt tolerance. It was found that there were abundant AM fungi resources in the soil surrounding the root of S. aconitifolia in Songnen saline grassland, and they could form a good symbiotic relationship with S. aconitifolia [26]. However, there have been no studies of the growth status and physiological characteristics of S. aconitifolia under salt-alkali stress, and the symbiotic relationship between AM fungi and S. aconitifolia and the response of the AM fungus-S. aconitifolia symbiosis system to salt-alkali stress has not been determined. Therefore, this study took S. aconitifolia as the research object to study the effects of inoculation of AM fungi under different salt-alkali stress on the growth and physiological characteristics of S. aconitifolia, which provided data support for AM-S. aconitifolia symbiosis under salt-alkali stress, and provided a certain theoretical basis for the rational utilization of salt-alkali land resources.
Experiments were conducted to investigate salt (NaCl) stress, alkali (NaHCO3) stress, and AM fungal inoculation treatments. Based on the growth of S. aconitifolia plants in a preliminary experiment, the NaCl and NaHCO3 concentrations in the stress treatments were set to 0, 50, 100, and 200 mmol/L, with the 0 mmol/L treatment being just distilled water. Under salt and alkali stress conditions, colonized and non-colonized treatments were established, respectively. The colonization of AM fungus was achieved by applying a 30 g inoculation on the soil surface of each pot, which was mixed evenly with the surface soil to ensure full contact with the S. aconitifolia root system. For the treatments without colonization, 30 mL of inoculation filtrate was applied to each pot. Inoculation filtrate was obtained by mixing the inoculating agent and water in a ratio of 1:2, and then passing the mixture through a 400-mesh sieve [27]. There were 16 treatments with three replicates per treatment for a total of 140 seedlings. Ninety days after seedling migration, the plants were subjected to salt and alkali stress at the set concentrations, with 50 mL of NaCl and NaHCO3 solution applied to each potted plant every day for 10 days (six groups of NaCl and NaHCO3 solutions with different concentrations were set up, which were 50, 100 and 200 mmol/L, respectively, and the control group was treated with distilled water). S. aconitifolia growth indicators were measured after the stress treatment was completed.
The S. aconitifolia plants used in the experiment were obtained from sowing and their seeds were purchased from Shuikong Pinglinmu Seed Department Store in Jiaxian County, Pingdingshan City, Henan Province, China. Seeds were disinfected before sowing. The treated seeds were placed in a glass Petri dish lined with double moistened filter paper. The Petri dish was placed into a constant temperature incubator to induce seed germination, with the temperature set to 18°C, humidity of 50%, and a 16:8 h light dark photoperiod. When the root system grew to approximately 1 cm after germination, the seeds were transferred to a pot with an AM fungal agent, with four seedlings in each pot. The AM fungal inoculation used for colonization was the peri-soil of S. aconitifolia roots collected from Songnen salt-alkali grassland, and it included AM fungal hypha and spores. The spore density was about 30/g. At the same time, we used morphological methods to identify the spores in the inoculant and found that the Glomus accounted for the largest number, and Septoglomus deserticola was the dominant species [26]. The collected soil surrounding the S. aconitifolia root was sifted for 20-mesh sieve, and then the sifted peri-soil of S. aconitifolia roots was used as the AM fungal inoculation, applied 30 g of inoculation to the surface of each pot of soil, and evenly mixed the fungus with the surface soil to make full contact between the fungus and the root system of S. aconitifolia. The size of the cultivated pot was 14 cm in diameter and 15 cm in height. The cultivation substrate was mixed according to a volume ratio of grass charcoal soil and vermiculite of 1:1 and sterilized at 121°C for 2 h before use. After the seedlings were moved, the plants were placed in the flower experimental nursery of Northeast Forestry University for cultivation.
2.3 Determination of Plant Root Colonization
After the salt and alkali stress treatments, the plants were separated from the soil, with care taken to avoid the root system during the separation process. After the root system was separated, rinsed with tap water, and the surface moisture was absorbed, the root segments were cut to about 1 cm with scissors, soaked in FAA fixative solution, and labeled and stored at 4°C. According to Phillips’ method [28], the root system was boiled and stained. After staining, 30 root segments were randomly selected from each pot, placed on a glass slide dripped with glycerol lactate, covered with a coverslip, and placed under an optical microscope (Axio Scope A1, Zeiss, Jena, Germany) to observe the hypha, arbuscular, and vesicles. Photographs were also taken. The mycorrhizal colonization (%) and intensity of structural colonization (%) were determined using MYCOCALC software. The mycorrhizal colonization (%) indicated the proportion of roots containing mycorrhizal structures to the entire root system. Intensity of structural colonization (%) indicated the intensity of AM fungal formation throughout the root system.
2.4 Determination of Biomass and Water Content
Complete plants with uniform growth under the different NaCl and NaHCO3 treatments were selected and separated from the soil for the determination of biomass and water status. They were also used for the determination of other physiological indicators after cutting away the aboveground parts and removing surface impurities. Each indicator was measured three times. The fresh weight (Wf), dry weight (Wd), and saturated weight (Wt) of the plants were accurately weighed by an electronic balance. The relative water content (RWC) and water saturation deficit (WSD) of the plants were calculated according to the method proposed by Liu et al. [29]: RWC = [(WF − WD)/(Wt − WD)] × 100%, WSD = 1 − RWC. The physiological and biochemical indicators of S. aconitifolia leaves under stress were determined by the method of Wang et al. [30].
2.5 Determination of Chlorophyll and Osmoregulatory Substance Concentration
After stress treatments, samples were taken from 16 treated plants, and the leaves of the same plants were removed with sterilised scissors, 0.5 g of leaves for each repeated stress treatment, where fresh leaves were determined for the concentration of osmomodulators (proline, soluble sugars, and soluble proteins), chlorophyll and MDA. The remaining samples are wrapped in tin foil and stored at ultra-low temperature (−80°C). The concentration of chlorophyll and osmoregulatory substances (soluble sugar, soluble protein and proline) were determined according to the method described by Wang et al. [30]. The chlorophyll a (Chl a) and chlorophyll b (Chl b) concentrations in fresh leaves were assessed spectrophotometrically. The concentration of proline, soluble protein, and soluble sugar were determined by the acid ninhydrin method, Coomassie brilliant blue G–250 staining, and anthrone colorimetry method.
2.6 Determination of Malondialdehyde Concentration and Antioxidant Enzymes Activity
Plant leaves treated with different salt and alkali stress were clipped with scissors, and 0.5 g of leaves were repeatedly used for each stress treatment. The concentration of malondialdehyde was determined according to the method described by Wang et al. [30], and antioxidant enzymes activity of the plants were calculated according to the method proposed by Liu et al. [29]. The MDA concentration was determined by the thiobarbituric acid (TBA) method. The glutathione (GSH) concentration was reduced by 2,3,4,6-tetradeuterio-5-nitrobenzoic acid (TDNB), the superoxide dismutase (SOD) activity was determined by the NBT method, the activity of peroxidase (POD) was determined by the guaiacol method and the activity of catalase (CAT) was determined by the permanganate titration.
A one-way analysis of variance (ANOVA) was conducted to analyze all results using the SPSS 25.0 statistical analysis package. All data were presented as the mean ± standard deviation (SD) of the three independent replications, and Duncan’s multiple range test was applied with the level of significance fixed at p < 0.05. Under the conditions of saline-alkali stress and AM fungus inoculation, we used a two-way ANOVA to analyze the physiological indexes of S. aconitifolia, and to confirm under which treatment the optimal growth performance was achieved. Excel was used for all calculations, and the results were expressed as the mean ± standard error (SE). Results were presented in histograms using SigmaPlot 14.0 and a radar chart was constructed using gene cloud drawing.
3.1 Colonization Characteristics
After 10 days of stress, colonized structures such as hyphae, vesicles, and arbuscules were observed in the root system of S. aconitifolia (Supplementary Fig. S1). With an increase in the salt or alkali stressor concentration, the colonization rate showed different degrees of decline, with the lowest colonization rate under the 200 mmol/L treatment. There was no significant difference in the colonization rate between the salt and alkali treatments (p > 0.05). The intensity and rate of colonization were the same under alkali stress but displayed a trend of first decreasing and then increasing under salt stress, with the lowest colonization rate under the 100 mmol/L treatment (Fig. 1). Under salt stress, the intensity and rate of colonization had a strong correlation (p < 0.05) with the Wf and Wd, and the concentration of chlorophyll a and total chlorophyll of S. aconitifolia (Table 1). Compared with salt stress, the intensity and rate of colonization were strongly correlated with the Wf, Wd, RWC, WSD, the concentration of chlorophyll a, chlorophyll b, and total chlorophyll of S. aconitifolia under alkali stress (p < 0.01).
Figure 1: Effects of AM fungi on the colonization rate and colonization intensity of S. aconitifolia root under salt-alkali stress
Note: Values in the figure are mean ± standard error, and different lowercase letters indicate significant differences among different treatment groups (p < 0.05). +AM indicates inoculation of AM fungi, and −AM indicates non-inoculation of AM fungi.
3.2 Growth Characteristics of S. aconitifolia
Under the same salt or alkali stress conditions, the Wf and Wd of inoculated S. aconitifolia plants were higher than those of the corresponding non-inoculated plants (Fig. 2). With an increase in the stress concentration, the Wf and Wd of inoculated and non-inoculated plants initially decreased. The Wd and Wf of non-inoculated and inoculated plants decreased by 64.8%, 34.2%, 57.5%, and 43.3%, respectively, compared with the control under 200 mmol/L salt stress, and decreased by 62.5%, 42.3%, 60.2%, and 51.3%, respectively, compared with the control under the corresponding alkali stress. Compared with the non-inoculated plants, the RWC of inoculated plants was significantly increased under all concentrations in the salt and alkali treatments. The RWC of inoculated and non-inoculated plants decreased with an increase in the salt concentration, with the maximum value at 50 mmol/L. With an increase in the alkali concentration, the RWC of S. aconitifolia plants decreased gradually for inoculated and non-inoculated plants. The minimum value was observed at 200 mmol/L (Table 2).
Figure 2: Effects of AM fungi on dry and fresh weight of S. aconitifolia
Note: Values in the figure are mean ± standard error, and different lowercase letters indicate significant differences among different treatment groups (p < 0.05). +AM indicates the inoculation of AM fungi, and −AM indicates non-inoculation of AM fungi.
Compared with the non-inoculated treatment, the concentration of chlorophyll a and total chlorophyll of S. aconitifolia were higher in the inoculated treatment, but the concentration of chlorophyll b was decreased under alkali stress. With an increase of stress concentration, the concentration of chlorophyll a, chlorophyll b, and total chlorophyll displayed a trend of constant decline. The concentration of chlorophyll a and total chlorophyll in the leaves of inoculated plants were significantly higher than those in the control, while the concentration of chlorophyll b was not significantly different from the control. The two-way ANOVA showed that salt stress had significant effects on the concentration of chlorophyll a, chlorophyll b, and total chlorophyll of S. aconitifolia (p < 0.01), while alkali stress had a significant effect on the concentration of chlorophyll a and total chlorophyll (p < 0.01) (Table 3).
3.3 Effects of AM Fungi on the Osmotic Regulator and Cell Membrane System of S. aconitifolia under Saline-Alkali Stress
Inoculation with AM fungi increased the soluble sugar concentration in the leaves of S. aconitifolia compared with the non-inoculated treatment (Table 4). With the increase in the stress concentration, the soluble protein concentration of S. aconitifolia leaves in the non-inoculated treatment displayed a trend of first increasing and then decreasing. The changes in the proline concentration differed from those of the soluble sugar and soluble protein concentrations. When the concentration was 0 mmol/L, the proline concentration in non-inoculated plants was 3.42 times that of inoculated plants. Under the same salt or alkali stress conditions (Fig. 3), the MDA concentration of inoculated S. aconitifolia plants was lower than that of the corresponding non-inoculated plants. With an increase in the salt or alkali concentrations, the MDA concentration in inoculated and non-inoculated plants continuously increased. However, the MDA concentration of inoculated and non-inoculated plants increased less than that under alkali stress. Table 3 shows that the interaction between AM fungi and alkali had a significant effect on MDA concentration (p < 0.01), while the interaction between AM fungus and salt had no significant effect on MDA concentration (p > 0.05).
Figure 3: Effects of AM fungi on MDA of S. aconitifolia
Note: Values in the figure are mean ± standard error, and different lowercase letters indicate significant differences among different treatment groups (p < 0.05). +AM indicates inoculation of AM fungi, and −AM indicates the non-inoculation of AM fungi.
With an increase in the NaHCO3 concentration, the POD, SOD, and CAT activities in leaves first increased and then decreased regardless of whether they were inoculated, while GSH activity continuously increased (Table 5). The POD, SOD, and CAT activities reached a maximum at 100 mmol/L. Among them, POD, SOD and CAT were the maximum at 100 mM, and the activities of POD, SOD, and CAT of the inoculated plants increased by 0.15 times, 1.37 times and 0.88 times compared with the control group. GSH was maximized at 200 mM, and the GSH activity of the inoculated plants increased by 0.24 times compared with the control group. When the NaCl concentration increased, the changes in POD, SOD, CAT, and GSH activities differed, but the POD and CAT activities of inoculated plants were higher than those of non-inoculated plants. The POD activity reached a maximum under the 200 mmol/L treatment, with an increase of 0.13 times compared with the control group. The CAT activity reached a maximum under the 100 mM treatment, with an increase of 4.64 times compared with the control group.
Soil salinization not only inhibited plant growth but also affected AM fungal germination and colonization [31]. With an increase in the salt or alkali concentration, the intensity and rate of colonization of AM fungi displayed a decreasing trend, because the excessive Na+ and Cl− concentration in the soil affected the growth of plant roots, hindered the uptake of water and nutrients by the roots, reduced the germination of AM fungal spores and other propagules, inhibited the elongation of germinated hyphae in the soil, and reduced the chance of AM fungi contacting plant roots [32]. Previous studies showed that inoculation with saline-alkali tolerant AM fungi was more effective than inoculation with non-saline-alkaline tolerant AM fungi [33]. This was confirmed in this study. With an increase in the salt or alkali concentration, the rate and intensity of S. aconitifolia colonization displayed a downward but not significant trend. We also found that AM fungi isolated from the S. aconitifolia root enclosure were more suitable for low-concentration alkali stress than salt stress, which differed from the results of previous studies [34]. This could be because the symbionts formed by AM fungi isolated from the S. aconitifolia root enclosure were better able to adapt to alkali stress.
The seedling period is one of the most important and sensitive stages in the entire life history of plants [35], and it is important to assess salt or alkali stress at this stage to clarify the response of plant physiological states and identify the mechanism of saline-alkali stress tolerance [36]. Excessive NaCl and NaHCO3 can affect the Wf and Wd of plants, and the high pH of NaHCO3 is particularly harmful to plants. Therefore, the Wf and Wd of plants under alkali stress was lower than under salt stress [37,38]. With an increase in the salt or alkali concentration, the Wf and Wd of S. aconitifolia decreased to varying degrees, but when the concentration was less than 200 mmol/L, the plant weight in the alkali treatment group was higher than that in the salt treatment group. The weight of the inoculated plants was higher than that of the non-inoculated plants, indicating that the inoculated S. aconitifolia had an improved salinity tolerance, which agreed with the results of previous studies [39]. However, under alkali stress, the inoculated group was also able to significantly increase the Wf and Wd of S. aconitifolia, which differed from previous studies [40]. This could be because the S. aconitifolia was more adaptable to alkali stress than salt stress, and AM fungi were more likely to establish symbionts with the S. aconitifolia to repair the damage caused by adversity in a low alkali concentration environment.
Under salt or alkali stress, plants respond to osmotic stress by adjusting their own water content [41]. In this study, the RWC of S. aconitifolia was affected by salt and alkali, with alkaline conditions having the greater impact (Fig. 4). Under the alkali treatment, the absorption of water by S. aconitifolia was reduced and the synthesis of other osmotic regulatory substances, such as soluble sugars, soluble proteins, and proline, was promoted to resist stress. The study also found that inoculation with AM fungus significantly increased the RWC of S. aconitifolia, which indicated that AM fungi could form a mycorrhizal network around the root system and enhance the absorption of water by roots. The concentration of Chlorophyll is one of the most sensitive physiological indicators of abiotic stress in plants because it has an important role in photosynthesis [42,43]. The chlorophyll concentration continuously decreases with an increase in chlorophyll degrading enzyme activity, which is a normal physiological stress response when plants are subjected to salt or alkali stress [44]. In this study, inoculation with AM fungi increased the concentration of chlorophyll a, chlorophyll b, and total chlorophyll in S. aconitifolia, indicating that the symbiosis of AM fungi and S. aconitifolia increased the uptake of Mg2+ by roots and also regulated the formation of hormones in plants to promote the synthesis of chlorophyll.
Figure 4: Radar map of S. aconitifolia response to different stresses
Note: The blue line indicates salt treatment, the red is alkali treatment, the green line indicates salt + AM fungal treatment, and the purple line indicates alkali + AM fungal treatment. MDA: malondialdehyde, SOD: superoxide dismutase, POD: peroxidase, CAT: catalase, GSH: reduced glutathione, RWC: relative water content, WSD: water saturation deficit.
Many ROS substances are produced in plants under salt-alkali stress, leading to membrane peroxidation [45–47]. Malondialdehyde is a product of lipid peroxidation, and the MDA concentration of S. aconitifolia could indicate the degree of damage to the cell membrane. The MDA concentration under the same concentration treatments was higher under alkali stress than salt stress, which indicated that the high pH was also damaging to the membrane system due to osmotic stress. Inoculation with AM fungi significantly reduced the MDA concentration and alleviated the damage to the saline base of the plant cell membrane system, which was consistent with previous research [45]. Plants enhance their salt and alkali tolerance by regulating their antioxidant and osmotic regulation systems [30,48]. The antioxidant system eliminates ROS in plants by improving the SOD, CAT, and POD activities and reducing the GSH activity [30,49,50]. The osmotic regulation system maintains the intracellular osmotic pressure and water content by synthesizing proline, soluble sugars, and soluble proteins [51,52]. In this study, S. aconitifolia depended more on proline and soluble protein for osmotic regulation under alkali stress and more on SOD and GSH for maintaining an antioxidant system under salt stress. This indicated that the stress resistance mechanism of S. aconitifolia differed under different types of stress. Inoculation with AM fungi increased the contribution of proline, soluble sugar, and soluble protein in the osmotic regulation system of S. aconitifolia under salt stress, and increased the contribution of GSH in the antioxidant system of S. aconitifolia under alkali stress. This indicated that AM fungi and S. aconitifolia were symbionts that were involved in the synthesis of organic matter. The regulatory effect of AM fungi differed under the different types of stress.
In summary, this study proved that the physiological functions of S. aconitifolia are reduced to varying degrees under salt or alkali stress, and inoculation with local AM fungi could improve osmotic regulation, the concentration of chlorophyll, and the antioxidant system of S. aconitifolia, ultimately improving the salinity tolerance. S. aconitifolia was found to regulate itself differently in response to different types of stress. Under salt stress, the S. aconitifolia upregulated the antioxidant system to reduce the role of osmotic regulatory substances. Under alkali stress, S. aconitifolia upregulated the effect of osmotic regulatory substances and reduced the effect of the ascorbic acid-GSH circulatory system. The results provided the corresponding theoretical basis for the symbiotic system formed between the local AM fungi community and host plants to tolerate saline-alkaline conditions. However, due to experimental limitations, this could not be fully verified. In future studies, the response mechanism of S. aconitifolia to saline-alkali stress should be explored at the microscopic level through isotope labeling and molecular biology techniques.
Acknowledgement: We thank the Biotechnology Company (Qingke Biotechnology Co., Ltd., Beijing, China) for the technical support, and we are sincerely thankful for the help from Yajie Liu.
Funding Statement: The research was funded by the National Natural Science Foundation of China with Grant Number 31601986 and the Heilongjiang Postdoctoral Scientific Research Developmental Fund (LBH-Q16005).
Author Contributions: Study conception and design: Chunxue Yang; data collection: Jiamei Xu; analysis and interpretation of results: Linlin Fang; draft manuscript preparation: Linlin Fang. All authors reviewed the results and approved the final version of the manuscript.
Availability of Data and Materials: Not applicable.
Ethics Approval: Not applicable.
Conflicts of Interest: The authors declared that they have no conflicts of interest to report regarding the present study.
Supplementary Materials: The supplementary material is available online at https://doi.org/10.32604/phyton.2023.043049.
References
1. Mukhopadhyay, R., Binoy, S., Hanuman-Sahay, J., Parbodh-Chander, S., Nanthi-S, B. (2021). Soil salinity under climate change: Challenges for sustainable agriculture and food security. Journal of Environmental Management, 280, 111736. [Google Scholar] [PubMed]
2. Kopittke, P. M., Menzies, N. W., Wang, P., McKenna, B. A., Lombi, E. (2019). Soil and the intensification of agriculture for global food security. Environment International, 132, 105078. [Google Scholar] [PubMed]
3. Ali, S., Rizwan, M., Qayyum, M. F., Ok, Y. S., Ibrahim, M. et al. (2017). Biochar soil amendment on alleviation of drought and salt stress in plants: A critical review. Environmental Science and Pollution Research, 24, 12700–12712. [Google Scholar] [PubMed]
4. Manoj, P., Amitava, R., Kiran, R., Rajendra, P. M., Dinesh, C. J. (2020). A consortium of arbuscular mycorrizal fungi improves nutrient uptake, biochemical response, nodulation and growth of the pea (Pisum sativum L.) under salt stress. Rhizosphere, 15, 100235. [Google Scholar]
5. Li, Y., Cai, Y. N., Ren, A. Q., Wei, L. X., Zhang, R. X. et al. (2022). Effects of saline-alkali stress on growth and physiological characteristics of Acer truncatum seedlings. Journal of Northeast Forestry University, 50(8), 5–14+21. [Google Scholar]
6. Yang, J. Y., Zheng, W., Tian, Y., Wu, Y., Zhou, D. W. (2011). Effects of various mixed salt-alkaline stresses on growth, photosynthesis, and photosynthetic pigment concentrations of Medicago ruthenica seedlings. Photosynthetica, 49, 275–284. [Google Scholar]
7. Abeer, H., Abdulaziz, A. A., Ramalingam, R., Al-Bandari-Fahad, A., Horiah-Abdulaziz, A. et al. (2018). Arbuscular mycorrhizal fungi regulate the oxidative system, hormones and ionic equilibrium to trigger salt stress tolerance in Cucumis sativus L. Saudi Journal of Biological Sciences, 25, 1102–1114. [Google Scholar]
8. Xu, J. M., Guo, J. Y., Wu, Y., Yang, C. X. (2021). Effect of AM fungi on the salt tolerance of Mentha spicata and Dianthus plumarius. Acta Botanica Boreali-Occidentalia Sinica, 41, 2104–2112. [Google Scholar]
9. Ahmad, P., Sharma, S. (2010). Physio-biochemical attributes in two cultivars of mulberry (Morus alba L.) under NaHCO3 stress. International Journal of Plant Production, 4, 79–86. [Google Scholar]
10. Khan, M. J., Asgher, M., Khan, N. A. (2014). Alleviation of salt-induced photosynthesis and growth inhibition by salicylic acid involves glycinebetaine and ethylene in mungbean (Vigna radiata L.). Plant Physiology and Biochemistry, 80, 67–74. [Google Scholar] [PubMed]
11. Zhao, F., Liu, C., Zhu, C. L., Zhang, H. S. (2020). Effects of functional microorganisms and biochar on the growth of seashore mallow and its rhizosphere soil fertility in coastal saline soil. Soil and Fertilizer Sciences in China, 289(5), 161–168. [Google Scholar]
12. Li, C. C., Zhou, Y. X., Gu, Q. (2021). The species diversity and community assembly of arbuscular mycorrhizal fungi in typical alpine grassland in Sanjiangyuan region. Acta Prataculturae Sinica, 30(1), 46–58. [Google Scholar]
13. Wang, W. B., He, X. L., Zhao, L. L., Wang, C. X., Zeng, X. D. et al. (2020). Spatial distribution characteristics of arbuscular mycorrhizal fungi of Artemisia ordosica in Desert Areas of Northwest China. Journal of Fungal Research, 18(1), 20–30. [Google Scholar]
14. Yang, F., Cao, H. M., Chen, Y. Y., Wang, J. L. (2019). Research progress on structure and identification method of arbusular mycorrhizal fungi. Modern Agricultural Science and Technology, 750(16), 152–157. [Google Scholar]
15. Wei, L. L., Lu, C. Y., Ding, J., Yu, S. (2016). Functional relationships between arbuscular mycorrhizal symbionts and nutrient dynamics in plant-soil-microbe system. Acta Ecologica Sinica, 36(14), 4233–4243. [Google Scholar]
16. Xu, M. L., Yang, J. H., Xie, H. X., Li, M. (2021). Effects of AM fungus on defense enzymes and endogenous hormones in leaves of watermelon seedlings under salt stress. Journal of Qingdao Agricultural University (Natural Science), 38(4), 235–240. [Google Scholar]
17. Qi, X. C., Yi, G. T., Fu, X. X., Wang, H. L., Wang, J. L. et al. (2021). Effects of arbuscular mycorrhizal fungi on physiological indexes of Potentilla anserina under drought-stress. Acta Agrestia Sinica, 29(11), 2407–2412. [Google Scholar]
18. Zhou, S. L., Guo, L. D. (2021). Research progress of arbuscular mycorrhizal fungi associated with plants in desert areas. Mycosystema, 40(10), 2523–2536. [Google Scholar]
19. Sun, H., Zheng, Y. L., Lin, Y. L., Chen, C., Yang, F. Y. (2021). Effects of biochar, phosphorus addition and AMF inoculation on switchgrass growth and soil properties under Cd stress. Acta Prataculturae Sinica, 30(12), 71–80. [Google Scholar]
20. Zhang, X. H., Han, C. Z., Gao, H. M., Cao, Y. P. (2019). Comparative transcriptome analysis of the garden Asparagus (Asparagus offic-inalis L.) reveals the molecular mechanism for growth with arbuscular mycorrhizal fungi under salinity stress. Plant Physiology and Biochemistry, 141, 20–29. [Google Scholar] [PubMed]
21. Zhou, Y. H., Liu, Y. J., Wang, Y. D., Yang, C. X. (2022). Claroideoglomus etunicatum improved the growth and saline–alkaline tolerance of Potentilla anserina by altering physiological and biochemical properties. BIOCELL, 46(8), 1967–1978. https://doi.org/10.32604/biocell.2022.019304 [Google Scholar] [CrossRef]
22. Liu, Y. J., Fang, L. L., Zhao, W. N., Yang, C. X. (2023). Effects of different arbuscular mycorrhizal fungi on physiology of Viola prionantha under salt stress. Phyton-International Journal of Experimental Botany, 92, 55–69. https://doi.org/10.32604/phyton.2022.022159 [Google Scholar] [CrossRef]
23. Wu, S. Z., Li, J. L., Zhu, X. Z. (2010). Experimental study on the antitumor activity of alcohol extract from Syneilesis aconitifolia. Chinese Journal of Hospital Pharmacy, 31(2), 102–104. [Google Scholar]
24. Li, J., Wang, F. Q., Ding, N., Zhao, M., Wang, J. L. et al. (2018). Chemical constituents from Syneilesis aconitifolia. Chinese Traditional and Herbal Drugs, 49(16), 3742–3746. [Google Scholar]
25. Wang, F. Q., Sun, L. Q., Wang, J. L., Zhao, M., Li, J. et al. (2020). Chemical constituents from Syneilesis aconitifolia (II). Chinese Traditional and Herbal Drugs, 51(11), 2878–2885. [Google Scholar]
26. Xu, J. M., Fang, L. L., Yang, C. X. (2023). Infect and species diversity of AM fungi in the rhizosphere of Syneilesis aconitifolia in Songnen saline-alkali grassland. Journal of Northwest Forestry University, 38(2), 173–179. [Google Scholar]
27. Wang, Y. N., Tao, S., Hua, X. Y., Yu, X. Y., Yan, X. F. et al. (2018). Effects of arbuscular mycorrhizal fungi on the growth and physiological metabolism of Leymus chinensis under salt-alkali stress. Acta Ecologica Sinica, 38(6), 2187–2194. [Google Scholar]
28. Phillips, J. M., Hayman, D. S. (1970). Improved producers for clearing roots and staining parasitic and vesicular arbuscular mycorrhizal fungi for rapid assessment of infection. Transactions of the British Mycological Society, 55(1), 158–161. [Google Scholar]
29. Liu, P., Li, M. J. (2016). Plant physiology experiments: Determination of enzyme activity and growth indicators. 3rd editionBeijing, China: Science Press. [Google Scholar]
30. Wang, X. K., Huang, J. L. (2015). Determination of physiological and biochemical indexes. 2nd editionBeijing, China: Higher Education Press. [Google Scholar]
31. Rabie, G. H., Almadini, A. M. (2015). Role of bioinoculants in development of salt-tolerance of Vicia faba plants under salinity stress. African Journal of Biotechnology, 4, 210–222. [Google Scholar]
32. Santander, C., Aroca, R., Ruiz-lozano, J. M., Olave, J. L. (2017). Arbuscular mycorrhiza effects on plant performance under osmotic stress. Mycorrhiza, 27(7), 639–657. [Google Scholar] [PubMed]
33. Jahangir, A. M., AbdulAziz, A., Basharat, A. D., Abeer, H., Thobayet, S. et al. (2022). Arbuscular mycorrhizal fungi isolated from highly saline “sabkha habitat” soil alleviated the NaCl-induced stress and improved Lasiurus scindicus Henr. Growth. Agriculture, 12(3), 337. [Google Scholar]
34. Alexios, A., Anna, A., Panagiotis, P., Maria, B., Nikolina, V. et al. (2023). Hedypnois cretica L. and Urospermum picroides L. plant growth, nutrient status and quality characteristics under salinity stress. Horticulturae, 9, 65. [Google Scholar]
35. Jones, H. G., Flowers, T. J., Jones, M. B. (1989). Physiology and ecology and their application to plant improvement. New York: Biochemistry. [Google Scholar]
36. Mayer, A. M., Poljakoff-Mayber, A. (1963). The germination of seeds. Oxford: Pergamon Press. [Google Scholar]
37. Yang, C. X., Chong, J. N., Li, C. Y., Kim, C. M., Shi, D. C. et al. (2017). Osmotic adjustment and ion balance traits of an alkali resistant halophyte Kochia sieversiana during adaptation to salt and alkali conditions. Plant and Soil, 294, 263–276. [Google Scholar]
38. Hameed, A., Egamberdieva, D., Abd-Allah, E. F., Hashem, A., Kumar, A. et al. (2014). Salinity stress and arbuscular mycorrhizal symbiosis in plants. In: Use of microbes for the alleviation of soil stresses. New York: Springer. [Google Scholar]
39. Ghazi-N, A. K. (2006). Nursery inoculation of tomato with arbuscular mycorrhizal fungi and subsequent performance under irrigation with saline water. Scientia Horticulturae, 109(1), 1–7. [Google Scholar]
40. Lin, J. X., Wang, Y. N., Sun, S. N., Mu, C. S., Yan, X. F. (2017). Effects of arbuscular mycorrhizal fungi on the growth, photosynthesis and photosynthetic pigments of Leymus chinensis seedlings under salt-alkali stress and nitrogen deposition. Science of the Total Environment, 576, 234–241. [Google Scholar] [PubMed]
41. Yang, Y. R., Han, X. Z., Liang, Y., Ghosh, A., Chen, J. et al. (2015). The combined effects of arbuscular mycorrhizal fungi (AMF) and lead (Pb) stress on Pb accumulation, plant growth parameters, photosynthesis, and antioxidant enzymes in Robinia pseudoacacia L. PLos One, 10(2), e145726. [Google Scholar]
42. Toshiyuki, T., Motohiko, K., Masahiro, Y., Toshio, Y. A. (2010). Quantitative trait locus for chlorophyll content and its association with leaf photosynthesis in rice. Rice, 3, 172–180. [Google Scholar]
43. Taibi, K., Taibi, F., Abderrahim, L. A., Ennajah, A., Belkhodja, M. et al. (2016). Effect of salt stress on growth, chlorophyll content, lipid peroxidation and antioxidant defence systems in Phaseolus vulgaris L. South African Journal of Botany, 105, 306–312. [Google Scholar]
44. Guo, R., Zhou, J., Hao, W., Gu, F., Liu, Q. et al. (2014). Germination, growth, chlorophyll fluorescence and ionic balance in linseed seedlings subjected to saline and alkaline stresses. Plant Production Science, 17, 20–31. [Google Scholar]
45. Ruiz-sanchez, M., Aroca, R., Munoz, Y., Polon, R., Ruiz-lozano, J. M. (2010). The arbuscular mycorrhizal symbiosis enhances the pho-tosynthetic efficiency and the antioxidative response of rice plants subjected to drought stress. Journal of Plant Physiology, 167(11), 862–869. [Google Scholar] [PubMed]
46. Hu, F. J., Zhai, X. M., Deng, M., Yang, H. B., Sheng, Z. L. (2022). Effect of soil acidity and aluminum stress on reactive oxygen metabolism in inoculating AMF tea seedlings. South China Agriculture, 16(9), 17–21+26. [Google Scholar]
47. Yang, F., Guo, J. T., Tang, Y. L. (2020). Effects of arbuscular mycorrhizal fungi (AMF) on growth and development of grafted cucumber seedlings with double-broken roots. China Cucurbits and Vegetables, 33(12), 61–65. [Google Scholar]
48. Sun, S. M., Chang, W., Song, F. Q. (2020). Mechanism of arbuscular mycorrhizal fungi improve the oxidative stress to the host plants under salt stress: A review. Chinese Journal of Applied Ecology, 31(10), 3589–3596. [Google Scholar] [PubMed]
49. Na, W., Zhen, L., Fei, W., Ming, T. (2016). Comparative photochemistry activity and antioxidant responses in male and female Populus cathayana cuttings inoculated with arbuscular mycorrhizal fungi under salt. Scientific Reports, 6, 37663. [Google Scholar]
50. Ana-María, V. B., Sergey, S., Milena, C., Oxana, D., Igor, P. (2012). Salt-sensitive and salt-tolerant barley varieties differ in the extent of potentiation of the ROS-induced K+ efflux by polyamines. Plant Physiology and Biochemistry, 61, 18–23. [Google Scholar]
51. Cao, Y. P., Dai, P., Dai, S. Y. (2017). Effects of arbuscular mycorrhiza fungi (AMF) on osmoregulation substances and antioxidant enzyme activities of asparagus plant under salt stress. Journal of Southwest University (Natural Science Edition), 39(5), 43–48. [Google Scholar]
52. Chen, X. N., Aili, Y. N., Gao, W. L., Ma, X. D. (2022). Effect of arbuscular mycorrhizal fungi on the growth and physiology of Alhagi sparsifolia seedling under salt stress. Pratacultural Science, 39(9), 1763–1772. [Google Scholar]
Cite This Article
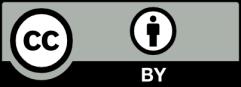