Open Access
ARTICLE
Unexpected Diversity in Ecosystem Nutrient Responses to Experimental Drought in Temperate Grasslands
1 School of Life Sciences, Hebei University, Baoding, 071002, China
2 Liaoning Northwest Grassland Ecosystem National Observation and Research Station, Erguna Forest-Steppe Ecotone Research Station, Institute of Applied Ecology, Chinese Academy of Sciences, Shenyang, 110164, China
3 Northwest Institute of Eco-Environment and Resources, Chinese Academy of Sciences, Lanzhou, 730030, China
4 School of Grassland Science, Beijing Forestry University, Beijing, 100083, China
5 College of Forestry, Henan Agricultural University, Zhengzhou, 450002, China
6 State Key Laboratory of Efficient Utilization of Arid and Semi-Arid Arable Land in Northern China, Institute of Agricultural Resources and Regional Planning, Chinese Academy of Agricultural Sciences, Beijing, 100081, China
7 Key Laboratory of Arable Land Quality Monitoring and Evaluation, Ministry of Agriculture and Rural Affairs, Institute of Agricultural Resources and Regional Planning, Chinese Academy of Agricultural Sciences, Beijing, 100081, China
* Corresponding Author: Wentao Luo. Email:
(This article belongs to the Special Issue: Grassland Ecology in China under Global Change)
Phyton-International Journal of Experimental Botany 2024, 93(4), 831-841. https://doi.org/10.32604/phyton.2024.047560
Received 09 November 2023; Accepted 22 March 2024; Issue published 29 April 2024
Abstract
The responses of ecosystem nitrogen (N) and phosphorus (P) to drought are an important component of global change studies. However, previous studies were more often based on site-specific experiments, introducing a significant uncertainty to synthesis and site comparisons. We investigated the responses of vegetation and soil nutrients to drought using a network experiment of temperate grasslands in Northern China. Drought treatment (66% reduction in growing season precipitation) was imposed by erecting rainout shelters, respectively, at the driest, intermediate, and wettest sites. We found that vegetation nutrient concentrations increased but soil nutrient concentrations decreased along the aridity gradient. Differential responses were observed under experimental drought among the three grassland sites. Specifically, the experimental drought did not change vegetation and soil nutrient status at the driest site, while strongly reduced vegetation but increased soil nutrient concentrations at the site with intermediate precipitation. On the contrary, experimental drought increased vegetation N concentrations but did not change vegetation P and soil nutrient concentrations at the wettest site. In general, the differential effects of drought on ecosystem nutrients were observed between manipulative and observational experiments as well as between sites. Our research findings suggest that conducting large-scale, consistent, and controlled network experiments is essential to accurately evaluate the effects of global climate change on terrestrial ecosystem bio-geochemistry.Keywords
The direct and indirect effects of global climate change on Earth’s primary biogeochemical cycles represent a crucial aspect of environmental change research [1]. Nitrogen (N) and phosphorus (P) are the key essential nutrients that play a critical role in regulating plant growth, maintenance, and reproduction within terrestrial ecosystems [2–4]. Plant N is an essential component of many macromolecules, including proteins and nucleic acids [2,5]. Plant P is a vital component of genetic processing, energy storage, and membrane structure [2,6]. Identifying their changes in the ecosystem to climate change therefore has significant implications for the stability of ecosystem structure and functions [5,7–9].
Precipitation is an important driver of biogeochemical cycles in many terrestrial and grassland ecosystems [10–12]. Therefore, the prolonged drought periods projected to intensify and become more severe in numerous regions worldwide by the century’s end, as a result of climate change [13], have significantly reshaped the dynamics of ecosystem nutrients, thereby impacting their processes and functions [5,14–16]. Reduction in soil moisture can depress plant growth, maintenance, and reproduction by reducing nutrient acquisition [6,14]. The acquisition of inorganic nutrients by roots from soils depends on soil nutrient availability, but also on the ability and physiological efficiency of nutrient uptake and translocation systems [6,17]. Drought can decrease microbial activity and the mobilization or immobilization of nutrients, thereby the nutrient availability for vegetation [18,19]. Drought-induced soil moisture reduction can have a detrimental impact on the mass flow or diffusion of nutrients, limiting nutrient transport from roots to shoots and subsequently affecting nutrient uptake [17,20]. A reduction in vegetation nutrient uptake without dependence on soil nutrient mineralization may then result in soil nutrient accumulation [20]. Despite increased soil nutrient availability, plants are unable to absorb these nutrients due to limited water availability [11,21].
Experimental precipitation manipulations have been identified as a way to explore the responses and adaptation of ecosystems to drought [5,14]. The growing number of these field manipulative experiments has significantly enhanced our understanding of the plastic responses of ecosystem nutrient cycles to short-term precipitation changes [6,22–24]. Regrettably, manipulative drought experiments are typically conducted at a single location within limited time and space constraints. It remains uncertain whether the observed responses can be generalized to broader temporal and spatial scales [4,25,26]. Currently, there is a significant gap in our understanding of how local mechanisms influencing ecological responses to climate change can be extrapolated to a regional level [5]. This is one of many challenges ecologists face when moving beyond the scale on which most climate change experiments are conducted (i.e., the plot scale) [6]. Natural precipitation gradient provides the opportunity to study adjustments and adaptations of ecosystem nutrient cycles to long-term drought trends [23,27]; however, these experiments offer limited insights into the underlying mechanisms and may be influenced by additional covariate variables [22–24]. Combining manipulative control experiments with gradient observation studies would be valuable in identifying the key responses to water availability reduction and understanding how processes transition from short-term to long-term scales [6,28–30].
To examine the in situ responses of ecosystem nutrient dynamics to experimental drought, we have carried out coordinated and comparative drought experiments, respectively, at the driest (170 mm per year, close to desert and shrubland ecosystem), intermediate (334 mm per year), and wettest (400 mm per year, closed to forest ecosystem) grassland sites in Northern China. The wettest site received over twice the amount of precipitation as the driest site. We hypothesized that i) vegetation and soil nutrient concentrations would reduce with increased drought in the manipulative experiment and would be lower in the drier site than the wetter site in the gradient experiment, but ii) the magnitude of the response would differ between the two approaches [29,31] and among the three grassland sites in the manipulative experiment [26,32].
We, respectively, selected the driest, intermediate, and wettest sites in the temperate grasslands of Northern China to conduct the manipulative drought experiment (Fig. S1). This temperate grassland is a significant community within the Eurasian steppe region, known as the most extensive grassland area globally [33]. While the region features a continental climate throughout, there is a precipitation gradient that increases from west to east, varying from around 150 mm in the western desert steppe to approximately 400 mm in the eastern meadow steppe, as evidenced by long-term data collection [5,21]. Productivity reaches its annual peak in the middle of August due to the high availability of soil water. All three sites feature calcic-orthic aridisols as per the US soil taxonomy classification and are categorized as chestnut soils in the Chinese soil classification system.
The driest site, close to the desert and shrublands, was located on the western edge of the temperate grassland distribution. This particular ecosystem represents the driest habitat within our surveyed temperate grasslands, receiving an annual precipitation of around 170 mm, with a significant portion occurring during the growing season. The experimental plots were situated in an area where grazing was restricted. The plant composition was predominantly characterized by the perennial bunchgrass Stipa breviflora, along with Allium mongolicum and Peganum harmala, collectively contributing to over 90% of the total canopy cover in this habitat. The medium precipitation site was located in the middle of our studied temperature grasslands, where the mean annual precipitation is 334 mm per year, with 90% of the precipitation distributed from May to October. The research site has been enclosed with fencing to prohibit all livestock grazing activities since 1983. The prevailing climatic conditions have fostered a plant community primarily characterized by the dominant perennial rhizome grass Leymus chinensis, alongside several perennial bunchgrasses such as S. grandis, Achnatherum sibiricum, and Potentilla tanacetifolia, collectively contributing to over 85% of the total plant canopy cover in this habitat. The wettest site, close to the forest ecosystem, was located on the eastern edge of the temperate grassland distribution. This ecosystem is considered the wettest habitat in our studied temperate grasslands and receives approximately 400 mm of precipitation annually, much of which falls during the growing season. The temperate ecosystem at this station was historically heavily grazed by sheep and cattle. However, for the past 30 years, livestock grazing has been prohibited at the site. The landscape is now characterized by a dominance of perennial rhizome grass L. chinensis, along with Pulsatilla turczaninovii, Iris ventricosa, and Artemisia laciniata, collectively contributing to over 70% of the total plant canopy cover in this habitat.
A significant reduction in the precipitation during the growing season (−66% of the ambient) occurred in 2015 and 2016. This is about 50% reduction of total annual precipitation.
At each site, we established replicate 6 m × 6 m experimental plots (n = 6 control and drought treatment) in a relatively homogeneous area. At each site, all experimental plots were positioned more than 2 m apart, with a separation of 1 m, and enclosed by a 6 mil plastic barrier to isolate them hydrologically from neighboring plots. The 6 m × 6 m plot size included an external buffer of 1 m to minimize the edge effects.
We passively manipulated the precipitation reaching the plots via a version of rainout shelters of transparent polyethylene [34]. The rain shelters had small effects on the light environment and permitted >90% of photosynthetically active radiation [6,26,34].
The steel frame comprised four corner posts securely anchored in the soil to a depth of 1 m. The roof was sloped at a 15° angle towards the plot’s edge, forming a central peak 6 m long, flanked by two lower edges of the same length equipped with gutters to channel rainwater away from the plots. The rainout shelters were elevated 2 m above the ground surface and the peaked roof allowed for quick drainage of the ambient precipitation. The rain shelters were not closed to the ground to reduce unwanted influences of the experiments on wind and temperature. The soil moisture content was significantly decreased due to the imposed drought (Fig. S2).
In August 2016, we placed four subquadrats (50 cm × 50 cm) within a primary quadrat (1 m × 1 m) in each plot. We randomly sampled the plant foliar of the dominant species as well as the topsoil (0–10 cm) within two diagonally arranged subquadrats.
In the manipulative experiment, the foliar samples were pooled by species and plot. Plant and soil samples are ground to a fine powder (Retsch MM 400, Retsch GmbH and Co KG, Haan, Germany). We determined plant and soil N concentrations using an elemental analyzer. The plant and soil samples underwent acid digestion using a mixture of acids in a microwave oven. This process allowed the sample to dissolve into a clear solution. Plant and soil (available) P concentrations were then colorimetrically analyzed using the ammonium molybdate-vanadate reagent.
The nutrient concentrations of vegetation were determined by calculating the mean values of all the dominant species selected in each plot. We employed linear mixed models to examine the impact of drought on vegetation and soil nutrient levels. The models incorporated drought treatment (control, drought), site, and their interaction as fixed factors, with species nested within a block considered as a random effect. We conducted pairwise comparisons between control and drought treatments within each site. Here, we used drought treatment as a fixed factor and species nested within a block as a random factor. We conducted comparisons of vegetation and soil nutrient concentrations across the three sites using one-way ANOVAs, followed by Duncan’s Test for post-hoc analysis of multiple comparisons.
We tested all data for homogeneity of variance and normality of distribution. Since all data met model assumptions, untransformed data were utilized for statistical analysis. We performed all the statistical analyses in the nlme package of R 3.2.4 (R Foundation for Statistical Computing, Vienna, Austria).
The manipulative experiment showed that, when all data were combined in three sites, vegetation N concentration did not change and vegetation P concentration was significantly reduced, while soil total N and available P concentrations did not show any variations under drought treatment (p < 0.001, Fig. 1). Differently, the gradient experiment showed that the vegetation N and P concentrations were higher and soil total N and available P concentrations were lower at the drier versus wetter site (p < 0.05, Figs. 2 and 3).
Figure 1: Responses of vegetation and soil nutrients (foliar N and P concentrations and soil total N and available P concentrations) to experimental drought in grasslands. The error bars represent the standard errors of the mean. The significant p-value (p < 0.05) is shown
Figure 2: The mixed model analysis of variance results for vegetation nitrogen (N) and phosphorus (P) concentrations at each grassland site in Northern China. Lowercase letters denote significant differences among the sites. The error bars represent the standard errors of the mean. A significant p-value (p < 0.05) is shown
Figure 3: The mixed model analysis of variance results for soil total N and available P concentrations for each grassland site with different precipitation levels in temperate grasslands of Northern China. Lowercase letters signify significant differences among the respective sites. The bars indicate the standard errors of the mean. The significant p-value (p < 0.05) is shown
Significant interactive effects of drought treatment and site on vegetation nitrogen (N) and phosphorus (P) concentrations were observed (p < 0.05), indicating that the effects of experimental drought on vegetation N and P concentrations varied between sites (Table 1). Experimental drought did not change the N and P concentrations of vegetation in the driest site and significantly reduced the N and P concentrations in the site with intermediate precipitation (Fig. 2). Experimental drought increased the vegetation N concentration from 16 to 21 mg g−1 (p < 0.001) but did not influence the P concentration at the wettest site (Fig. 2).
The interactive effects of drought treatment and site on soil total N and available P concentrations were significant (p < 0.05), indicating that the responses of soil total N and available P concentrations to experimental drought varied among sites (Table 1). Soil total N and available P concentrations did not significantly change during drought at the driest and wettest sites, while the total N and available P concentrations significantly increased (p < 0.05) under drought treatment at the site with intermediate precipitation (Fig. 3).
We assessed the responses of vegetation and soil nutrient status to a crossed design of a natural aridity gradient and an experimental drought treatment. In the observational experiment, we observed an increase in vegetation nitrogen (N) and phosphorus (P) contents, whereas soil N and P contents decreased as aridity levels increased from the wettest to the driest grassland sites. In contrast, in the manipulative experiment, we noted a decrease in vegetation N and P, while soil N and P contents increased with drought conditions at the grassland site with intermediate aridity. This suggests that the impacts of drought on ecosystem nutrient cycles were different between manipulative and observational experiments, which are consistent with our hypotheses and some previous studies [6,24,29]. These differences between long-term (gradient observation) and short-term (manipulative experiments) studies can result from the changes in the relative contributions of variations within vs. among species on ecosystem nutrient composition in the context of climatic drought [35]. In observational studies along the natural gradient, changes in ecosystem biogeochemistry reflect processes linked to the effect of resilience and marked responses to long-term drought changes. Over an extended period, the ecosystem has undergone evolution, leading to the introduction of new species and the decline of resident species across various sites along the aridity gradient [21,24]. In this phase, shifts in ecosystem nutrient composition provide information on what organisms must change in organisms to optimize their structure and functions to live under contrasting precipitation conditions. However, nutrient variations within species play a more marked role in the resistance and stability of ecosystem nutrient dynamics to short-term drought changes in experimental studies [24,29]. The ecosystem community evolves over a short period, with significant changes in the abundance of resident species, but little in species composition. In this phase, changes in ecosystem nutrient composition provide evidence of how ecosystems cope with drought when they are displaced from their optimum.
In line with our hypotheses, differential directions and magnitudes of the nutritional responses of the ecosystem to experimental drought were observed among temperate grasslands in Northern China [5]. The concentrations of nutrients in the soil and vegetation exhibited remarkable stability even under the conditions of experimental drought at the driest site, implying that the ecosystem had developed adaptive mechanisms to cope with prolonged dry spells, thereby maintaining a delicate balance in nutrient levels within the ecosystem (Figs. 2 and 3). The greater resistance to drought at the driest site can be explained by the ‘previous exposure’ hypothesis [36], which suggests that The ecosystem’s resilience to climate change is influenced by its past climatic history and ecosystems frequently exposed to climate extremes may exhibit greater resistance to similar future extremes [16,37,38]. In this region, the ecosystem has a long history of drought because periodic drought occurs regularly during the growing season. Therefore, its species may be better adapted to cope with drought than other wetter habitats [18,39,40]. The enhanced resilience to drought in the arid ecosystem can also be attributed to the ‘functional composition’ hypothesis, which suggests that stress-tolerant traits of organisms play a crucial role in shaping their responses to climate change and other environmental stressors [18,36,41]. Plant species serve as an example; to adapt to water stress, they have evolved sets of functional traits such as slow growth rates, high nutrient concentrations, long tissue lifespans, and high root-to-shoot ratios in this drought-susceptible habitat [42,43].
Vegetation and soil nutrient concentrations changed the most with experimental drought at the site with intermediate precipitation, suggesting the least resistance to drought. Consistent with this result, Paruelo et al. demonstrated that grasslands with moderate precipitation levels exhibited the highest sensitivity to changes in precipitation [44]. There are two potential reasons for this interesting pattern. First, the habitat with intermediate precipitation neither has abundant soil water for organism growth as the wettest habitat nor has stress-tolerant organisms capable of withstanding strong environmental variation as the driest habitat [15]. Second, drought reduced microbial and vegetational nutrient uptake more strongly than microbial activity and litter decomposition rate, leading to an increase in a higher nutrient pool in soils at this site [11,15]. The elevated soil nutrient accumulation observed in this context could heighten the risk of nutrient loss through leaching or erosion, leading to short- to medium-term nutrient depletion [20].
Our findings carry several significant implications that must be taken into account for predicting the extent of ecological responses to anticipated climate disturbances. Firstly, both experimental and gradient methods yielded varying responses of ecosystem nutrients to heightened aridity. Experimental studies offer valuable insights into the immediate and medium-term responses of ecosystem nutrients to environmental shifts. In contrast, gradient observational studies unveil the anticipated long-term responses in ecosystem biogeochemistry. Secondly, there is considerable variation in the nutritional reactions of ecosystems to drought across sites experiencing differing water conditions within the biome. The inconsistency among sites highlighted that site-specific mechanisms that elucidate ecological responses to climate change may not directly translate at a regional level. This underscores the need for coordinated multisite studies with controlled driver magnitudes. Finally, the intricate nutritional responses of ecosystems to projected climate shifts underline the necessity for future experiments to enhance our comprehension of ecosystem dynamics within the grassland biome.
Acknowledgement: We thank everyone who assisted in carrying out the field experiment.
Funding Statement: This study was financially supported by the National Key Research and Development Program of China (2019YFE0117000), the National Natural Science Foundation of China (32171549 and 31971465) and the Youth Innovation Promotion Association CAS (2020199).
Author Contributions: W.L. initiated the research project; N.T., L.S., Y.S., C.Q., X.Z., Q.Y., J.Q., and Z.W. conducted the experiments; B.Q. and W.L. analyzed the data and prepared the initial manuscript draft, with input from H.W. for subsequent revisions of the text.
Availability of Data and Materials: The datasets produced and analyzed during the present study can be obtained from the corresponding author upon request.
Ethics Approval: Not applicable.
Conflicts of Interest: The authors have no relevant financial or non-financial interests to disclose.
References
1. Delgado-Baquerizo M, Maestre FT, Gallardol A, Bowker MA, Wallenstein MD, Luis Quero J, et al. Decoupling of soil nutrient cycles as a function of aridity in global drylands. Nat. 2013;502(7473):672–6. [Google Scholar]
2. Wright IJ, Westoby M. Leaves at low versus high rainfall: coordination of structure, lifespan, and physiology. New Phytol. 2002;155(3):403–16. [Google Scholar] [PubMed]
3. Elser JJ, Fagan WF, Kerkhoff AJ, Swenson NG, Enquist BJ. Biological stoichiometry of plant production: metabolism, scaling and ecological response to global change. New Phytol. 2010;186(3):593–608. [Google Scholar] [PubMed]
4. Luo W, Zuo X, Griffin-Nolan RJ, Xu C, Sardans J, Yu Q, et al. Chronic and intense droughts differentially influence grassland carbon-nutrient dynamics along a natural aridity gradient. Plant Soil. 2022;473(1):137–48. [Google Scholar]
5. Luo W, Muraina TO, Griffin-Nolan RJ, Ma W, Song L, Fu W, et al. Responses of a semiarid grassland to recurrent drought are linked to community functional composition. Ecol. 2023;104(2):e3920. [Google Scholar]
6. Luo W, Zuo X, Ma W, Xu C, Li A, Yu Q, et al. Differential responses of canopy nutrients to experimental drought along a natural aridity gradient. Ecol. 2018;99(7):2230–9. [Google Scholar]
7. Wright IJ, Reich PB, Westoby M, Ackerly DD, Baruch Z, Bongers F, et al. The worldwide leaf economics spectrum. Nat. 2004;428(6985):821–7. [Google Scholar]
8. Walter J, Grant K, Beierkuhnlein C, Kreyling J, Weber M, Jentsch A. Increased rainfall variability reduces biomass and forage quality of temperate grassland largely independent of mowing frequency. Agr Ecosyst Environ. 2012;148(12):1–10. [Google Scholar]
9. Sardans J, Grau O, Chen HYH, Janssens IA, Ciais P, Piao S, et al. Changes in nutrient concentrations of leaves and roots in response to global change factors. Glocal Change Biol. 2017;23(9):3849–56. [Google Scholar]
10. Engler R, Randin CF, Thuiller W, Dullinger S, Zimmermann NE, Araujo MB, et al. 21st century climate change threatens mountain flora unequally across Europe. Global Change Biol. 2011;17(7):2330–41. [Google Scholar]
11. Luo W, Xu C, Ma W, Yue X, Liang X, Zuo X, et al. Effects of extreme drought on plant nutrient uptake and resorption in rhizomatous vs bunchgrass-dominated grasslands. Oecologia. 2018;188(2):633–43. [Google Scholar] [PubMed]
12. Carroll CJW, Slette IJ, Griffin-Nolan RJ, Baur LE, Hoffman AM, Denton EM, et al. Is a drought a drought in grasslands? Productivity responses to different types of drought. Oecologia. 2021;197(4):1017–26. doi:10.1007/s00442-020-04793-8. [Google Scholar] [PubMed] [CrossRef]
13. Dai AG. Drought under global warming: a review. Wires Clim Change. 2011;2(1):45–65. doi:10.1002/wcc.81. [Google Scholar] [CrossRef]
14. He M, Dijkstra FA. Drought effect on plant nitrogen and phosphorus: a meta-analysis. New Phytol. 2014;204(4):924–31. doi:10.1111/nph.12952. [Google Scholar] [PubMed] [CrossRef]
15. Knapp AK, Condon KV, Folks CC, Sturchio MA, Griffin-Nolan RJ, Kannenberg SA, et al. Field experiments have enhanced our understanding of drought impacts on terrestrial ecosystems-but where do we go from here? Funct Ecol. 2023;38(1):76–97. doi:10.1111/1365-2435.14460. [Google Scholar] [CrossRef]
16. Vilonen L, Ross M. Smith MDWhat happens after drought ends: synthesizing terms and definitions. New Phytol. 2022;235(2):420–31. doi:10.1111/nph.18137. [Google Scholar] [CrossRef]
17. Gessler A, Schaub M, McDowell NG. The role of nutrients in drought-induced tree mortality and recovery. New Phytol. 2017;214(2):513–20. doi:10.1111/nph.14340. [Google Scholar] [PubMed] [CrossRef]
18. Jensen KD, Beier C, Michelsen A, Emmett BA. Effects of experimental drought on microbial processes in two temperate heathlands at contrasting water conditions. APPL Soil Ecol. 2003;24(2):165–76. doi:10.1016/S0929-1393(03)00091-X. [Google Scholar] [CrossRef]
19. Sanaullah M, Rumpel C, Charrier X, Chabbi A. How does drought stress influence the decomposition of plant litter with contrasting quality in a grassland ecosystem? Plant Soil. 2012;352(1):277–88. doi:10.1007/s11104-011-0995-4. [Google Scholar] [CrossRef]
20. Sardans J, Peñuelas J, Prieto P, Estiarte M. Drought and warming induced changes in P and K concentration and accumulation in plant biomass and soil in a Mediterranean shrubland. Plant Soil. 2008;306(1):261–71. doi:10.1007/s11104-008-9583-7. [Google Scholar] [CrossRef]
21. Luo W, Elser J, Lü XT, Wang Z, Bai E, Yan C, et al. Plant nutrients do not covary with soil nutrients under changing climatic conditions. Glocal Biogeochem Cy. 2015;29(8):1298–1308. doi:10.1002/2015GB005089. [Google Scholar] [CrossRef]
22. Luo YQ, Melillo J, Niu SL, Beier C, Clark JS, Classen AT, et al. Coordinated approaches to quantify long-term ecosystem dynamics in response to global change. Glocal Change Biol. 2011;17(2):843–54. doi:10.1111/j.1365-2486.2010.02265.x. [Google Scholar] [CrossRef]
23. Elmendorf SC, Henry GHR, Hollister RD, Fosaa AM, Gould WA, Hermanutz L, et al. Experiment, monitoring, and gradient methods used to infer climate change effects on plant communities yield consistent patterns. Proc Natl Acad Sci USA. 2015;112(2):448–52. [Google Scholar] [PubMed]
24. Yuan ZY, Jiao F, Shi XR, Sardans J, Maestre FT, Delgadobaquerizo M. Experimental and observational studies find contrasting responses of soil nutrients to climate change. eLife. 2017;6(6):e23255. [Google Scholar] [PubMed]
25. Peñuelas J, Gordon C, Llorens L, Nielsen T, Tietema A, Beier C, et al. Nonintrusive field experiments show different plant responses to warming and drought among sites, seasons, and species in a north-south European gradient. Ecosystems. 2004;7(6):598–612. [Google Scholar]
26. Cherwin K, Knapp A. Unexpected patterns of sensitivity to drought in three semi-arid grasslands. Oecologia. 2012;169(3):845–52. [Google Scholar] [PubMed]
27. Hewitt JE, Thrush SF, Dayton PK, Bonsdorff E. The effect of spatial and temporal heterogeneity on the design and analysis of empirical studies of scale-dependent systems. Am Nat. 2007;169(3):398–408. [Google Scholar] [PubMed]
28. Dunne JA, Saleska SR, Fischer ML, Harte J. Integrating experimental and gradient methods in ecological climate change research. Ecol. 2004;85(4):904–16. [Google Scholar]
29. Sandel B, Goldstein LJ, Kraft NJ, Okie JG, Shuldman MI, Ackerly DD, et al. Contrasting trait responses in plant communities to experimental and geographic variation in precipitation. New Phytol. 2010;188(2):565–75. [Google Scholar] [PubMed]
30. Martin-StPaul NK, Limousin JM, Vogt-Schilb H, Rodríguez-Calcerrada J, Rambal S, Longepierre D, et al. The temporal response to drought in a Mediterranean evergreen tree: comparing a regional precipitation gradient and a throughfall exclusion experiment. Glob Change Biol. 2013;19(8):2413–26. doi:10.1111/gcb.12215. [Google Scholar] [PubMed] [CrossRef]
31. Smith MD, Knapp AK, Collins SL. A framework for assessing ecosystem dynamics in response to chronic resource alterations induced by global change. Ecol. 2009;90(12):3279–89. doi:10.1890/08-1815.1. [Google Scholar] [PubMed] [CrossRef]
32. Knapp AK, Carroll CJW, Denton EM, La Pierre KJ, Collins SL, Smith MD. Differential sensitivity to regional-scale drought in six central US grasslands. Oecologia. 2015;177(4):949–57. doi:10.1007/s00442-015-3233-6. [Google Scholar] [PubMed] [CrossRef]
33. Bai YF, Han XG, Wu JG, Chen Z, Li LH. Ecosystem stability and compensatory effects in the inner mongolia grassland. Nat. 2004;431(7005):181–4. doi:10.1038/nature02850. [Google Scholar] [PubMed] [CrossRef]
34. Yahdjian L, Sala OE. A rainout shelter design for intercepting different amounts of rainfall. Oecologia. 2002;133(2):95–101. [Google Scholar] [PubMed]
35. Auger S, Shipley B. Inter-specific and intra-specific trait variation along short environmental gradients in an old-growth temperate forest. J Veg Sci. 2013;24(3):419–28. [Google Scholar]
36. Grime JP, Brown VK, Thompson K, Masters GJ, Hillier SH, Clarke IP, et al. The response of two contrasting limestone grasslands to simulated climate change. Science. 2000;289(5480):762–5. [Google Scholar] [PubMed]
37. Bertiller MB, Sain CL, Carrera AL, Vargas DN. Patterns of nitrogen and phosphorus conservation in dominant perennial grasses and shrubs across an aridity gradient in Patagonia. Argentina J Arid Environ. 2005;62(2):209–23. [Google Scholar]
38. Cernusak LA, Winter K, Turner BL. Leaf nitrogen to phosphorus ratios of tropical trees: experimental assessment of physiological and environmental controls. New Phtol. 2010;185(3):770–9. [Google Scholar] [PubMed]
39. Wang Z, Silva LCR, Sun G, Luo P, Mou C, Horwath WR. Quantifying the impact of drought on soil-plant interactions: a seasonal analysis of biotic and abiotic controls of carbon and nutrient dynamics in high-altitudinal grasslands. Plant Soil. 2015;389(1):59–71. [Google Scholar]
40. Wellstein C, Poschlod P, Gohlke A, Chelli S, Campetella G, Rosbakh S, et al. Effects of extreme drought on specific leaf area of grassland species: a meta-analysis of experimental studies in temperate and sub-Mediterranean systems. Glocal Change Biol. 2017;23(7):2473–81. [Google Scholar]
41. Grime JP, Fridley JD, Askew AP, Thompson K, Hodgson JG, Bennett CR. Long-term resistance to simulated climate change in an infertile grassland. Proc Natl Acad Sci USA. 2008;105(29):10028–10032. [Google Scholar] [PubMed]
42. Fernandez-Going BM, Anacker BL, Harrison SP. Temporal variability in California grasslands: soil type and species functional traits mediate response to precipitation. Ecol. 2012;93(22):2104–14. [Google Scholar]
43. Kang L, Han X, Zhang Z, Sun OJ. Grassland ecosystems in China: review of current knowledge and research advancement. Philos T R Soc B. 2007;362(1482):997–1008. [Google Scholar]
44. Paruelo JM, Lauenroth WK, Burke IC, Sala OE. Grassland precipitation-use efficiency varies across a resource gradient. Ecosystems. 1999;2(1):64–8. [Google Scholar]
Supplementary Materials
Figure S1: (A) Large rainout shelters were established at three grassland sites in Northern China. During the growing season (May–Aug.) 66% of incoming precipitation is passively reduced by these shelters. The drought treatment reduced aboveground net primary production across six sites, including wettest (B), intermediate (C), and driest steppe (D)
Figure S2: Seasonal dynamics of soil moisture at 10-cm depth in control and drought plots at three sites along an aridity gradient in Northern China
Cite This Article
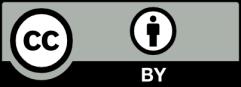