Open Access
VIEWPOINT
Resveratrol-related compounds: Potential for cancer and beyond
Department of Molecular Medicine, University of Pavia, Pavia, 27100, Italy
* Corresponding Authors: LUCIA ANNA STIVALA. Email: ; VIRGINIE SOTTILE. Email:
BIOCELL 2022, 46(12), 2525-2530. https://doi.org/10.32604/biocell.2022.022924
Received 31 March 2022; Accepted 24 May 2022; Issue published 10 August 2022
Abstract
The nutraceutical resveratrol is associated with a range of biological effects, from antibiotic to anti-inflammatory activities. One major axis of research has sought to harness its anti-tumour potential, with promising preclinical results and early clinical trials. A second strong interest relies on the anti-ageing effects ascribed to the compound and its application to stem cell research. It is becoming clear however that these possible favourable effects are conditioned by a set concentration range not easily controllable in vivo. Here we evoke novel developments in the field that could lead to more reliable conditions for the translational use of resveratrol-based compounds.Keywords
Resveratrol (RV) is a stilbenoid first isolated in 1939 from the traditional medicine plant Veratrum grandiflorum and also present in various foods such as grapes, apples, pistachios, plums and peanuts, earning it a place of choice among nutraceuticals (Zhang et al., 2021). From the two isomeric forms of RV, the trans-version is considered more biologically active (Kuršvietienė et al., 2016). Despite its low natural solubility in water, RV shows relatively high absorption (over 70%) after oral consumption (Vitrac et al., 2003; Walle et al., 2004), however its bioavailability is considered to be below 1% (Walle, 2011). RV is among the most actively studied natural compounds, as underlined by the number of RV-related entries recorded in the international clinical trial database (https://www.clinicaltrials.gov) covering a wide range of applications. This variety of biological effects can be linked to the diverse cellular pathways activated by RV, as the compound interacts with different cellular components and exerts multiple biological activities, including antioxidant (de la Lastra and Villegas, 2007; Xia et al., 2017), anti-inflammatory (Meng et al., 2021; Magrone et al., 2019), anti-microbial (Bostanghadiri et al., 2017), anti-proliferative (Stivala et al., 2001; Savio et al., 2016; Maccario et al., 2012) properties. RV is predominantly associated with antioxidant activity, an effect linked to the presence in its structure of three hydroxyl groups (Kuršvietienė et al., 2016; Stivala et al., 2001), as the molecule acts as scavenger of free radicals by increasing the intracellular concentration of antioxidant enzymes including SOD (superoxide dismutase), catalase, glutathione reductase and glutathione peroxidase (Yen et al., 2003; Ramprasath and Jones, 2010). It has however been proposed that RV could display a pro-oxidant activity, which could be responsible for lipid peroxidation and DNA damage (de la Lastra and Villegas, 2007). RV is also able to modulate several signalling pathways involved in inflammation, including AP-1, COX and NF-κB leading to an anti-inflammatory response (Meng et al., 2021). Crucially, RV is considered a potent activator of the NAD-dependent deacetylase enzyme Sirtuin 1 (SIRT1), a pleiotropic regulator of gene expression and silencing with particular relevance to cancer development (Yi and Luo, 2010; Choupani et al., 2018).
Possible Anti-Cancer Applications
RV has been taken up as anti-cancer molecule following numerous studies demonstrating its anti-tumoral profile, through combined effects on cancer cell proliferation, tumour microenvironment and angiogenesis (reviewed in (Naujokat and McKee, 2020)). RV is considered a chemopreventive agent, acting in the three major stages of carcinogenesis (Fig. 1).
Figure 1: RV anti-cancer effects.
In particular, RV is involved in the regulation of phase I and II enzymes and in the scavenging of ROS, blocking the initiation stage of carcinogenesis (Ko et al., 2017). RV has been observed to inhibit cell proliferation, arrest the replication cycle, and induce apoptosis and autophagy (Salehi et al., 2018) in several in vitro cancer models such as HT1080 fibrosarcoma and MCF7 breast adenocarcinoma (Stivala et al., 2001; Savio et al., 2016; Maccario et al., 2012). SIRT1 being a major mediator of RV effects, the ability of RV to upregulate SIRT1 in cancer cells is thought to induce in turn the degradation of β-catenin, supporting anti-cancer activities (Jung et al., 2013; Firestein et al., 2008). The ability of RV to inhibit the growth of cancerous cells is associated with well-documented therapeutic effects on the tumour environment including inhibition of angiogenesis and matrix metalloproteases (MMPs), modulation of epithelial-to-mesenchymal transition (EMT), and inhibition of invasion and metastasis (Belleri et al., 2008; Rauf et al., 2018). The compound was also shown to downregulate the phosphorylation and acetylation of NF-κB, impairing tumour proliferation, invasion and metastasis. In ovarian cancer cell lines, Tino et al. (2016) demonstrated that RV treatment inhibited cell growth by decreasing NF-κB levels and its downstream gene vascular endothelial growth factor (VEGF), which contributes to angiogenesis.
Its direct and indirect anti-tumour properties, combined with the aforementioned antioxidant and anti-inflammatory effects, hold clear potential for the use of RV in the clinic. Although clinical evaluation of the molecule is not without challenges (Ren et al., 2021), some clinical trials have investigated the use of RV in cancer patients, in multiple myeloma, breast and colorectal cancer (Berman et al., 2017). While treatment of colorectal cancer patients with RV or grape powder showed partly reduced tumour proliferation and increased cleaved caspase-3 levels in malignant hepatic metastases (Howells et al., 2011), results to date are not fully consistent across models, as it did yield adverse effects in the multiple myeloma trial (Berman et al., 2017). Preclinical observations have shown steadier benefits using RV, proposed as a chemosensitizer in complement to conventional chemotherapy (Harikumar et al., 2010). This approach trialled by applying RV in combination with doxorubicin (DOX) in breast (Jin et al., 2019), ovarian (Tino et al., 2016) and colorectal (Buhrmann et al., 2016) cancer cell models, was observed to inhibit proliferation, metastasis and chemoresistance. RV could thus potentiate chemotherapy and lower the necessary drug doses, consequently reducing the detrimental effects in patients from oxidative stress, lipid peroxidation, inflammation, apoptosis and autophagy (Yang et al., 2022).
Diverse Effects on Stem Cell Populations
Beside this possible role in cancer, RV have been linked to increased longevity and resistance to age-induced degeneration (Bonkowski and Sinclair, 2016), contributing to its promotion as a multifaceted anti-ageing compound attracting much commercial interest. The effect of RV on stem cell populations, responsible for the long term maintenance of organs and tissue throughout the lifetime, has been investigated in a range of cellular models and linked to its ability to activate Sirtuins (Stefani et al., 2007; Catalgol et al., 2012).
RV was reported to promote self-renewal in undifferentiated embryonic stem cell (ESC) cultures, in both mouse and human models, and reduce apoptotic markers (Li et al., 2017; Safaeinejad et al., 2017, 2018). Consequently, RV is among the additives trialled to facilitate cellular reprogramming for the production of induced pluripotent stem cells (iPSC) (Chen et al., 2011; Ding et al., 2013), presumed to act on the SIRT1-SOX2 axis. Application of RV at various concentrations yielded diverse outcomes on adult stem cell populations, in particular lower concentrations were reported to increase the proliferative ability of mesenchymal progenitors (Yoon et al., 2014; Yuan et al., 2012), with a similar effect on neuroprogenitor also limited to lower doses (Kumar et al., 2016). With regards to differentiation response, low dose RV applied to pancreatic stem cells improved β-cell formation (Xu et al., 2017), while RV added to endothelial progenitors at 20 μM supported their differentiation (Campagnolo et al., 2015). In differentiating mesenchymal stem cell (MSC) cultures, RV was consistently observed to promote osteogenesis (Moon et al., 2020; Dai et al., 2007; Wang et al., 2019; Song et al., 2022) at doses up to 1 μM (reviewed in (Safaeinejad et al., 2018)), warranting its incorporation in tissue engineering products for localised release to promote bone repair (Wei et al., 2021). RV added to mouse mesenchymal progenitors also caused inhibition of the adipogenic response (Zhou et al., 2015; Li et al., 2016) through Sirt1 (Jang et al., 2017), although some conflicting reports have observed the opposite effect in some mouse (Hu et al., 2015) and in human progenitors (Caldarelli et al., 2015). The publication of such discordant results further underlines the likelihood of RV acting in a dose-, time- and species- dependent manner, already evidenced in certain stem cell models (Safaeinejad et al., 2018; Peltz et al., 2012).
Outstanding Issues & Perspectives
The basis of this apparent discrepancy in the differentiation response to RV has been linked to a biphasic dose-response profile (Borriello et al., 2013; reviewed in (Calabrese et al., 2010)), which might hinder general comparisons across models and studies. The fact that RV’s beneficial effects may depend on reaching a specific in vivo concentration, points to the need for a better controlled pharmacological profile to achieve the desired effects (Scott et al., 2012; Cai et al., 2015). Better RV bioavailability is under active investigation (Chimento et al., 2019), including via alternative formulations (Howells et al., 2011; Tripathi et al., 2018), nano-encapsulation to assist the delivery and release (Santos et al., 2019) and chemical analogues including hydroxylated (Piotrowska et al., 2012) or methylated (Kapetanovic et al., 2011) derivatives such as pterostilbene showing superior bioavailability in vivo. One derivative in particular, trans-4,4′-dihydroxystilbene or DHS has shown promising biological characteristics. Produced as a synthetic new compound, its chemical structure (Fig. 2) was originally optimised to replicate the trans bicyclic/phenolic stilbene backbone of RV, based on the correlation previously observed between structure and activity (Stivala et al., 2001). DHS was subsequently also identified as a natural derivative (Torres et al., 2003), and found to be a potent antioxidant and anti-proliferative stilbene, superior to RV in vitro (Maccario et al., 2012; Savio et al., 2009).
Figure 2: Chemical structure of RV (trans-3,5,4'-trihydroxystilbene) and DHS (trans-4,4’-dihydroxystilbene). Image created using the design tool from https://chem-space.com.
DHS is active at a lower concentration compared to RV, and shows a different mechanism of action. In particular, DHS modified the cell cycle in the G1 phase, whereas RV induces a block at the beginning of the S phase, involving different DNA polymerases (Savio et al., 2009). Strictly related to its structure, DHS is more potent than RV in inhibiting neoplastic transformation of murine fibroblasts, as well as the proliferation and invasion of breast cancer cells (Maccario et al., 2012). In vivo, a murine lung cancer model was used to show that tumour volume and cell proliferation were significantly inhibited by DHS, and this is related to the reduction of angiogenesis (Savio et al., 2016). In addition, liver metastatic lesions were significantly reduced by DHS treatment, an observation that was confirmed in a zebrafish tumour model (Savio et al., 2016) and in a separate colon cancer model (Kimura et al., 2020). These early results underline the potential of DHS as an alternative RV derivative for treatment of cancer and metastasis. More work is underway to explore its biological effects on other cell types and broader therapeutic applications.
Acknowledgement: We thank colleagues from the Department of Molecular Medicine for their support.
Availability of Data and Materials: Not applicable.
Author Contribution: The authors confirm contribution to the paper as follows: Manuscript preparation: M. Savio, V. Minoia, V. Sottile; Critical review of the manuscript: P. Fulghieri, LA. Stivala. All authors reviewed and approved the final version of the manuscript.
Ethics Approval: Not applicable.
Funding Statement:This work is supported by a Grant from the Italian Ministry of Education, University and Research (MUR) to the Department of Molecular Medicine of the University of Pavia under the Initiative ‘Dipartimenti di Eccellenza (2018–2022)’.
Conflicts of Interest:The authors declare that they have no conflicts of interest to report regarding the present study.
References
Belleri M, Ribatti D, Savio M, Stivala LA, Forti L et al. (2008). αvβ3 integrin-dependent antiangiogenic activity of resveratrol stereoisomers. Molecular Cancer Therapeutics 7: 3761–3770. DOI 10.1158/1535-7163.MCT-07-2351. [Google Scholar] [CrossRef]
Berman AY, Motechin RA, Wiesenfeld MY, Holz MK (2017). The therapeutic potential of resveratrol: A review of clinical trials. npj Precision Oncology 1: 35. DOI 10.1038/s41698-017-0038-6. [Google Scholar] [CrossRef]
Bonkowski MS, Sinclair DA (2016). Slowing ageing by design: The rise of NAD+ and sirtuin-activating compounds. Nature Reviews Molecular Cell Biology 17: 679–690. DOI 10.1038/nrm.2016.93. [Google Scholar] [CrossRef]
Borriello A, Bencivenga D, Caldarelli I, Tramontano A, Borgia A et al. (2013). Resveratrol and cancer treatment: Is hormesis a yet unsolved matter? Current Pharmaceutical Design 19: 5384–5393. DOI 10.2174/1381612811319300007. [Google Scholar] [CrossRef]
Bostanghadiri N, Pormohammad A, Chirani AS, Pouriran R, Erfanimanesh S et al. (2017). Comprehensive review on the antimicrobial potency of the plant polyphenol resveratrol. Biomedicine & Pharmacotherapy 95: 1588–1595. DOI 10.1016/j.biopha.2017.09.084. [Google Scholar] [CrossRef]
Buhrmann C, Shayan P, Popper B, Goel A, Shakibaei M (2016). Sirt1 is required for resveratrol-mediated chemopreventive effects in colorectal cancer cells. Nutrients 8: 145. DOI 10.3390/nu8030145. [Google Scholar] [CrossRef]
Cai H, Scott E, Kholghi A, Andreadi C, Rufini A et al. (2015). Cancer chemoprevention: Evidence of a nonlinear dose response for the protective effects of resveratrol in humans and mice. Science Traslational Medicine 7: 298ra117. DOI 10.1126/scitranslmed.aaa7619. [Google Scholar] [CrossRef]
Calabrese EJ, Mattson MP, Calabrese V (2010). Resveratrol commonly displays hormesis: Occurrence and biomedical significance. Human & Experimental Toxicology 29: 980–1015. DOI 10.1177/0960327110383625. [Google Scholar] [CrossRef]
Caldarelli I, Speranza MC, Bencivenga D, Tramontano A, Borgia A et al. (2015). Resveratrol mimics insulin activity in the adipogenic commitment of human bone marrow mesenchymal stromal cells. The International Journal of Biochemistry & Cell Biology 60: 60–72. DOI 10.1016/j.biocel.2014.12.011. [Google Scholar] [CrossRef]
Campagnolo P, Hong X, Di Bernardini E, Smyrnias I, Hu Y et al. (2015). Resveratrol-induced vascular progenitor differentiation towards endothelial lineage via MiR-21/Akt/β-catenin is protective in vessel graft models. PLoS One 10: e0125122. DOI 10.1371/journal.pone.0125122. [Google Scholar] [CrossRef]
Catalgol B, Saime B, Taga Y, Ozer NK (2012). Resveratrol: French paradox revisited. Frontiers in Pharmacology 3: 141. DOI 10.3389/fphar.2012.00141. [Google Scholar] [CrossRef]
Chen T, She L, Yu J, Wan H, Guo A et al. (2011). Rapamycin and other longevity-promoting compounds enhance the generation of mouse induced pluripotent stem cells: Longevity-promoting agents enhance reprogramming. Aging Cell 10: 908–911. DOI 10.1111/j.1474-9726.2011.00722.x. [Google Scholar] [CrossRef]
Chimento A, De Amicis F, Sirianni R, Sinicropi MS, Puoci F et al. (2019). Progress to improve oral bioavailability and beneficial effects of resveratrol. International Journal of Molecular Sciences 20: 1381. DOI 10.3390/ijms20061381. [Google Scholar] [CrossRef]
Choupani J, Derakhshan SM, Bayat S, Alivand MR, Khaniani MS (2018). Narrower insight to SIRT1 role in cancer: A potential therapeutic target to control epithelial-mesenchymal transition in cancer cells. Journal of Cellular Physiology 233: 4443–4457. DOI 10.1002/jcp.26302. [Google Scholar] [CrossRef]
Dai Z, Li Y, Quarles LD, Song T, Pan W et al. (2007). Resveratrol enhances proliferation and osteoblastic differentiation in human mesenchymal stem cells via ER-dependent ERK1/2 activation. Phytomedicine 14: 806–814. DOI 10.1016/j.phymed.2007.04.003. [Google Scholar] [CrossRef]
de la Lastra C, Villegas I (2007). Resveratrol as an antioxidant and pro-oxidant agent: Mechanisms and clinical implications. Biochemical Society Transactions 35: 1156–1160. DOI 10.1042/BST0351156. [Google Scholar] [CrossRef]
Ding DF, Li X, Xu H, Wang Z, Liang Q et al. (2013). Mechanism of resveratrol on the promotion of induced pluripotent stem cells. Journal of Integrative Medicine 11: 389–396. DOI 10.3736/jintegrmed2013039. [Google Scholar] [CrossRef]
Firestein R, Blander G, Michan S, Oberdoerffer P, Ogino S et al. (2008). The SIRT1 deacetylase suppresses intestinal tumorigenesis and colon cancer growth. PLoS One 3: e2020. [Google Scholar]
Harikumar KB, Kunnumakkara AB, Sethi G, Diagaradjane P, Anand P et al. (2010). Resveratrol, a multitargeted agent, can enhance antitumor activity of gemcitabine in vitro and in orthotopic mouse model of human pancreatic cancer. International Journal of Cancer 127: 257–268. [Google Scholar]
Howells LM, Berry DP, Elliott PJ, Jacobson EW, Hoffmann E et al. (2011). Phase I randomized, double-blind pilot study of micronized resveratrol (SRT501) in patients with hepatic metastases—safety, pharmacokinetics, and pharmacodynamics. Cancer Prevention Research 4: 1419–1425. DOI 10.1158/1940-6207.CAPR-11-0148. [Google Scholar] [CrossRef]
Hu P, Zhao L, Chen J (2015). Physiologically achievable doses of resveratrol enhance 3T3-L1 adipocyte differentiation. European Journal of Nutrition 54: 569–579. DOI 10.1007/s00394-014-0738-4. [Google Scholar] [CrossRef]
Jang MJ, Park UH, Kim JW, Choi H, Um SJ et al. (2017). CACUL1 reciprocally regulates SIRT1 and LSD1 to repress PPARγ and inhibit adipogenesis. Cell Death & Disease 8: 3201. DOI 10.1038/s41419-017-0070-z. [Google Scholar] [CrossRef]
Jin X, Wei Y, Liu Y, Lu X, Ding F et al. (2019). Resveratrol promotes sensitization to doxorubicin by inhibiting epithelial-mesenchymal transition and modulating SIRT1/B-catenin signaling pathway in breast cancer. Cancer Medicine 8: 1246–1257. DOI 10.1002/cam4.1993. [Google Scholar] [CrossRef]
Jung W, Hong KD, Jung WY, Lee E, Shin BK et al. (2013). SIRT1 expression is associated with good prognosis in colorectal cancer. Korean Journal of Pathology 47: 332. DOI 10.4132/KoreanJPathol.2013.47.4.332. [Google Scholar] [CrossRef]
Kapetanovic IM, Muzzio M, Huang Z, Thompson TN, McCormick DL (2011). Pharmacokinetics, oral bioavailability, and metabolic profile of resveratrol and its dimethylether analog, pterostilbene, in rats. Cancer Chemother Pharmacol 68: 593–601. DOI 10.1007/s00280-010-1525-4. [Google Scholar] [CrossRef]
Kimura Y, Sumiyoshi M, Kiyoi T, Baba K (2020). Dihydroxystilbenes prevent azoxymethane/dextran sulfate sodium-induced colon cancer by inhibiting colon cytokines, a chemokine, and programmed cell death-1 in C57BL/6J mice. European Journal of Pharmacology 886: 173445. DOI 10.1016/j.ejphar.2020.173445. [Google Scholar] [CrossRef]
Ko JH, Sethi G, Um JY, Shanmugam MK, Arfuso F et al. (2017). The role of resveratrol in cancer therapy. International Journal of Molecular Sciences 18: 2589. DOI 10.3390/ijms18122589. [Google Scholar] [CrossRef]
Kumar V, Pandey A, Jahan S, Shukla RK, Kumar D et al. (2016). Differential responses of trans-resveratrol on proliferation of neural progenitor cells and aged rat hippocampal neurogenesis. Scientific Reports 6: 28142. DOI 10.1038/srep28142. [Google Scholar] [CrossRef]
Kuršvietienė L, Stanevičienė I, Mongirdienė A, Bernatonienė J (2016). Multiplicity of effects and health benefits of resveratrol. Medicina 52: 148–155. DOI 10.1016/j.medici.2016.03.003. [Google Scholar] [CrossRef]
Li N, Du Z, Shen Q, Lei Q, Zhang Y et al. (2017). Resveratrol enhances self-renewal of mouse embryonic stem cells. Journal of Cellular Biochemistry 118: 1928–1935. DOI 10.1002/jcb.25942. [Google Scholar] [CrossRef]
Li S, Bouzar C, Cottet-Rousselle C, Zagotta I, Lamarche F et al. (2016). Resveratrol inhibits lipogenesis of 3T3-L1 and SGBS cells by inhibition of insulin signaling and mitochondrial mass increase. Biochimica et Biophysica Acta (BBA)-Bioenergetics 1857: 643–652. DOI 10.1016/j.bbabio.2016.03.009. [Google Scholar] [CrossRef]
Maccario C, Savio M, Ferraro D, Bianchi L, Pizzala R et al. (2012). The resveratrol analog 4,4′-dihydroxy-trans-stilbene suppresses transformation in normal mouse fibroblasts and inhibits proliferation and invasion of human breast cancer cells. Carcinogenesis 33: 2172–2180. DOI 10.1093/carcin/bgs244. [Google Scholar] [CrossRef]
Magrone T, Magrone M, Russo MA, Jirillo E (2019). Recent advances on the anti-inflammatory and antioxidant properties of red grape polyphenols: In vitro and in vivo studies. Antioxidants 9: 35. DOI 10.3390/antiox9010035. [Google Scholar] [CrossRef]
Meng T, Xiao D, Muhammed A, Deng J, Chen L et al. (2021). Anti-inflammatory action and mechanisms of resveratrol. Molecules 26: 229. DOI 10.3390/molecules26010229. [Google Scholar] [CrossRef]
Moon DK, Kim BG, Lee AR, Choe YI, Khan I et al. (2020). Resveratrol can enhance osteogenic differentiation and mitochondrial biogenesis from human periosteum-derived mesenchymal stem cells. Journal of Orthopaedic Surgery and Research 15: 203. DOI 10.1186/s13018-020-01684-9. [Google Scholar] [CrossRef]
Naujokat C, McKee DL (2020). The big five phytochemicals targeting cancer stem cells: Curcumin, EGCG, sulforaphane, resveratrol and genistei. Current Medicinal Chemistry 22: 4321–4342. [Google Scholar]
Peltz L, Gomez J, Marquez M, Alencastro F, Atashpanjeh N et al. (2012). Resveratrol exerts dosage and duration dependent effect on human mesenchymal stem cell development. PLoS One 7: e37162. DOI 10.1371/journal.pone.0037162. [Google Scholar] [CrossRef]
Piotrowska H, Kucinska M, Murias M (2012). Biological activity of piceatannol: Leaving the shadow of resveratrol. Mutation Research 750: 60–82. DOI 10.1016/j.mrrev.2011.11.001. [Google Scholar] [CrossRef]
Ramprasath VR, Jones PJH (2010). Anti-atherogenic effects of resveratrol. European Journal of Clinical Nutrition 64: 660–668. DOI 10.1038/ejcn.2010.77. [Google Scholar] [CrossRef]
Rauf A, Imran M, Butt MS, Nadeem M, Peters DG et al. (2018). Resveratrol as an anti-cancer agent: A review. Critical Reviews in Food Science and Nutrition 58: 1428–1447. DOI 10.1080/10408398.2016.1263597. [Google Scholar] [CrossRef]
Ren B, Kwah MXY, Liu C, Ma Z, Shanmugam MK et al. (2021). Resveratrol for cancer therapy: Challenges and future perspectives. Cancer Letters 515: 63–72. DOI 10.1016/j.canlet.2021.05.001. [Google Scholar] [CrossRef]
Safaeinejad Z, Kazeminasab F, Kiani-Esfahani A, Ghaedi K, Nasr-Esfahani MH (2018). Multi-effects of resveratrol on stem cell characteristics: Effective dose, time, cell culture conditions and cell type-specific responses of stem cells to resveratrol. European Journal of Medicinal Chemistry 155: 651–657. DOI 10.1016/j.ejmech.2018.06.037. [Google Scholar] [CrossRef]
Safaeinejad Z, Nabiuni M, Peymani M, Ghaedi K, Nasr-Esfahani MH et al. (2017). Resveratrol promotes human embryonic stem cells self-renewal by targeting SIRT1-ERK signaling pathway. European Journal of Cell Biology 96: 665–672. DOI 10.1016/j.ejcb.2017.08.002. [Google Scholar] [CrossRef]
Salehi B, Mishra A, Nigam M, Sener B, Kilic M et al. (2018). Resveratrol: A double-edged sword in health benefits. Biomedicines 6: 91. DOI 10.3390/biomedicines6030091. [Google Scholar] [CrossRef]
Santos AC, Pereira I, Pereira-Silva M, Ferreira L, Caldas M et al. (2019). Nanotechnology-based formulations for resveratrol delivery: Effects on resveratrol in vivo bioavailability and bioactivity. Colloids and Surfaces B: Biointerfaces 180: 127–140. DOI 10.1016/j.colsurfb.2019.04.030. [Google Scholar] [CrossRef]
Savio M, Coppa T, Bianchi L, Vannini V, Maga G et al. (2009). The resveratrol analogue 4,4′-Dihydroxy-trans-stilbene inhibits cell proliferation with higher efficiency but different mechanism from resveratrol. The International Journal of Biochemistry & Cell Biology 41: 2493–2502. DOI 10.1016/j.biocel.2009.08.005. [Google Scholar] [CrossRef]
Savio M, Ferraro D, Maccario C, Vaccarone R, Jensen LD et al. (2016). Resveratrol analogue 4,4′-dihydroxy-trans-stilbene potently inhibits cancer invasion and metastasis. Scientific Reports 6: 19973. DOI 10.1038/srep19973. [Google Scholar] [CrossRef]
Scott E, Steward WP, Gescher AJ, Brown K (2012). Resveratrol in human cancer chemoprevention choosing the right dose. Moecular Nutrition & Food Research 56: 7–13. DOI 10.1002/mnfr.201100400. [Google Scholar] [CrossRef]
Song CY, Guo Y, Chen FY, Liu WG (2022). Resveratrol promotes osteogenic differentiation of bone marrow-derived mesenchymal stem cells through MiR-193a/SIRT7 axis. Calcified Tissue International 110: 117–130. DOI 10.1007/s00223-021-00892-7. [Google Scholar] [CrossRef]
Stefani M, Markus MA, Lin RCY, Pinese M, Dawes IW et al. (2007). The effect of resveratrol on a cell model of human aging. Annals of the New York Academy of Sciences 1114: 407–418. DOI 10.1196/annals.1396.001. [Google Scholar] [CrossRef]
Stivala LA, Savio M, Carafoli F, Perucca P, Bianchi L et al. (2001). Specific structural determinants are responsible for the antioxidant activity and the cell cycle effects of resveratrol. Journal of Biological Chemistry 276: 22586–22594. DOI 10.1074/jbc.M101846200. [Google Scholar] [CrossRef]
Tino AB, Chitcholtan K, Sykes PH, Garrill A (2016). Resveratrol and acetyl-resveratrol modulate activity of VEGF and IL-8 in ovarian cancer cell aggregates via attenuation of the NF-κB protein. Journal of Ovarian Research 9: 84. DOI 10.1186/s13048-016-0293-0. [Google Scholar] [CrossRef]
Torres P, Avila JG, Romo de Vivar A, Garcı́a AM, Marı́n JC et al. (2003). Antioxidant and insect growth regulatory activities of stilbenes and extracts from yucca periculosa. Phytochemistry 64: 463–473. DOI 10.1016/S0031-9422(03)00348-0. [Google Scholar] [CrossRef]
Tripathi V, Chhabria S, Jadhav V, Bhartiya D, Tripathi A (2018). Stem cells and progenitors in human peripheral blood get activated by extremely active resveratrol (XARTM). Stem Cell Reviews and Reports 14: 213–222. DOI 10.1007/s12015-017-9784-7. [Google Scholar] [CrossRef]
Vitrac X, Desmoulière A, Brouillaud B, Krisa S, Deffieux G et al. (2003). Distribution of [14C]-trans-resveratrol, a cancer chemopreventive polyphenol, in mouse tissues after oral administration. Life Sciences 72: 2219–2233. DOI 10.1016/S0024-3205(03)00096-1. [Google Scholar] [CrossRef]
Walle T (2011). Bioavailability of resveratrol: Resveratrol bioavailability. Annals of the New York Academy of Sciences 1215: 9–15. DOI 10.1111/j.1749-6632.2010.05842.x. [Google Scholar] [CrossRef]
Walle T, Hsieh F, DeLegge MH, Oatis JE, Walle UK (2004). High absorption but very low bioavailability of oral resveratrol in humans. Drug Metabolism and Disposition 32: 1377–1382. DOI 10.1124/dmd.104.000885. [Google Scholar] [CrossRef]
Wang H, Hu Z, Wu J, Mei Y, Zhang Q et al. (2019). Sirt1 promotes osteogenic differentiation and increases alveolar bone mass via Bmi1 activation in mice. Journal of Bone and Mineral Research 34: 1169–1181. [Google Scholar]
Wei B, Wang W, Liu X, Xu C, Wang Y et al. (2021). Gelatin methacrylate hydrogel scaffold carrying resveratrol-loaded solid lipid nanoparticles for enhancement of osteogenic differentiation of BMSCs and effective bone regeneration. Regenerative Biomaterials 8: rbab044. [Google Scholar]
Xia N, Daiber A, Förstermann U, Li H (2017). Antioxidant effects of resveratrol in the cardiovascular system. British Journal of Pharmacology 174: 1633–1646. [Google Scholar]
Xu S, Sun F, Ren L, Yang H, Tian N et al. (2017). Resveratrol controlled the fate of porcine pancreatic stem cells through the Wnt/β-Catenin signaling pathway mediated by Sirt1. PLoS One 12: e0187159. [Google Scholar]
Yang R, Dong H, Jia S, Yang Z (2022). Resveratrol as a modulatory of apoptosis and autophagy in cancer therapy. Clinical and Translational Oncology 24: 1219–1230. DOI 10.1007/s12094-021-02770-y. [Google Scholar] [CrossRef]
Yen GC, Duh PD, Lin CW (2003). Effects of resveratrol and 4-hexylresorcinol on hydrogen peroxide-induced oxidative DNA damage in human lymphocytes. Free Radical Research 37: 509–514. [Google Scholar]
Yi J, Luo J (2010). SIRT1 and P53, effect on cancer, senescence and beyond. Biochimica et Biophysica Acta (BBA)-Proteins and Proteomics 1804: 1684–1689. DOI 10.1016/j.bbapap.2010.05.002. [Google Scholar] [CrossRef]
Yoon DS, Choi Y, Jang Y, Lee M, Choi WJ et al. (2014). SIRT1 directly regulates SOX2 to maintain self-renewal and multipotency in bone marrow-derived mesenchymal stem cells. Stem Cells 32: 3219–3231. DOI 10.1002/stem.1811. [Google Scholar] [CrossRef]
Yuan HF, Zhai C, Yan XL, Zhao DD, Wang JX et al. (2012). SIRT1 is required for long-term growth of human mesenchymal stem cells. Journal of Molecular Medicine 90: 389–400. DOI 10.1007/s00109-011-0825-4. [Google Scholar] [CrossRef]
Zhang LX, Li CX, Kakar MU, Khan MS, Wu PF et al. (2021). Resveratrol (RVA pharmacological review and call for further research. Biomedicine & Pharmacotherapy 143: 112164. DOI 10.1016/j.biopha.2021.112164. [Google Scholar] [CrossRef]
Zhou Y, Zhou Z, Zhang W, Hu X, Wei H et al. (2015). SIRT1 inhibits adipogenesis and promotes myogenic differentiation in C3H10T1/2 pluripotent cells by regulating wnt signaling. Cell & Bioscience 5: 61. DOI 10.1186/s13578-015-0055-5. [Google Scholar] [CrossRef]
Cite This Article
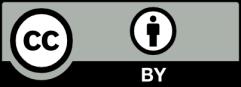