Open Access
REVIEW
Interleukin-1 receptor antagonist: From synthesis to therapeutic applications
1 Department of Pharmacy, The Women University, Multan, 60000, Pakistan
2 Department of Pharmaceutical Chemistry, Government College University, Faisalabad, 38000, Pakistan
3 Research Center for Advanced Materials Science (RCAMS), King Khalid University, Abha, 62413, Saudi Arabia
4 Department of Chemistry, Faculty of Science, King Khalid University, Abha, 62413, Saudi Arabia
* Corresponding Author: MUHAMMAD SAJID HAMID AKASH. Email:
(This article belongs to the Special Issue: Cell-Based Regenerative Therapies)
BIOCELL 2023, 47(4), 809-823. https://doi.org/10.32604/biocell.2023.025850
Received 01 August 2022; Accepted 23 September 2022; Issue published 08 March 2023
Abstract
The cytokine channel’s mechanism for self-regulation involves the application of antagonistic cytokines that are synthesized to connect to the receptors and release soluble cytokine receptors. The very first receptor antagonist of cytokine that was naturally present was interleukin-1 receptor antagonist (IL-1Ra). The IL-1Ra protein forms are disinfected from supernatants of cultured monocytes on stacked IgG. The family of IL-1 consists of IL-1α, IL-1β and IL-1Ra. Human monocytes regulate the production of IL-Ra. IL-Ra takes part in normal physiological functions by using specific antibodies, and acts as an anti-inflammatory agent. IL-Ra is synthesized in the tissues during the period of active disease and can be systematically measured and/or estimated. Maintenance of the levels of IL-Ra and IL-1 is the main factor for host resistance in patients during diseased conditions, as IL-Ra acts as an inherent regulator of various inflammatory responses. In this article, we focuse on how IL-Ra is synthesized and performs its functions once the inflammatory responses are activated.Keywords
The mechanism by which cytokines self-regulate involves the application of antagonistic cytokines synthesized to connect with the receptors and release soluble cytokine receptors. Interleukin-1 receptor antagonist (IL-Ra) is the very first receptor antagonist of naturally occurring cytokine (Arend et al., 1998). Interleukin-1 (IL-1) was first defined in 1972 as the lymphocyte-activating factor involved in the induction of inflammation, increase in body temperature, triggering of the proliferation of T and B cells, regulation of hematopoiesis, and induction of proteins in acute phase. IL-1 is also responsible for the regulation of calcium level in blood, proliferation of different cells, regulation of blood pressure, and sleep modification. IL-1 is one of the main mediators of inflammatory responses that represents the network of pro-inflammatory effect molecules (Vicenová et al., 2009).
The IL-1 family is a group of eleven cytokines that take part in regulating the inflammatory responses. IL-1 is a derived macrophage lymphocyte activating factor. It is a 17-kDa glycoprotein, produced by macrophages and synthesized in multiple organs. IL-Ra binds with different receptors present on different target cells and its production is stimulated inside these cells. IL-1 acts as a mediator to destroy the tissues in human diseases (Khayata et al., 2020).
IL-Ra exists in three forms which are purified from supernatants of cultured monocytes on bound IgG. The synthesis of IL-Ra in humans occurs in monocytes, which depend upon the specific generalization of protein of immune complexes that are adhered to IgG (Orino et al., 1992). The inhibited bioactivity was not examined in the monocytes from the supernatants, which are cultured with soluble immune molecule complexes, especially with red blood cells coated with IgG. Human monocytes regulate the production of IL-Ra. The surface of IL-1β consists of three binding sites that separately interact with the extracellular portion of three Ig domains of the IL-1R (Dinarello, 2018). The contact points for the binding of receptors differ among IL-1Ra, IL-1α, and 1β. In vivo, IL-1 performs its activity on immune and inflammatory cells. IL-1β peptide studies have isolated the molecular regions responsible for mediating the functions. Synthetic nonapeptide constitutes in vivo immunomodulatory properties associated with inflammation during the absence of metabolic changes (Espírito-Santo et al., 2017).
IL-1Rs are of two types, type I and type II (Krakauer and Oppenheim, 1998). Type-I receptors transduce the signals while type-II receptors attach to IL-1 but cannot transduce the signals. Epithelial cells and keratinocytes produce variant forms of IL-Ra. The mRNA of the IL-Ra protein encodes the developed portion similar to IL-Ra monocyte but, varies in the structure the of leader peptide. The monocyte contains 25 amino acids-long signals sequence while the IL-Ra keratinocyte contains a truncated sequence of 7-amino acid. The first four amino acids of the N-terminal are the same as those of the IL-Ra leader peptide monocyte while the remaining residues are different (Fields et al., 2019). It took nearly 350 million years to evolve IL-1α and IL-1β as a peptide but only IL-Ra evolved as a signal peptide. Early members of IL-1 have a molecular mass of 31 kDa and are precursor proteins for the IL-1 family when first generated. The pro and mature IL-1α are biologically active. Many other growth factors of cytokines like neurotropic factors are active in the form of precursor. Compared to these, IL-1β is inactive and also needs cleavage of 17 kDa peptide to maintain the biological activity. The binding of IL-Ra is also explained by the ability to bind the IL-1 with a receptor on the cell surface without inducing any intra-cellular agonist effect. In vitro, the agonist effect is absent, but is observed in multiple cells i.e., human fibroblasts, murine thymocytes, human monocytes, rabbit chondrocytes, human endothelial cells, neutrophils, and macrophages.
Different strategies are being used to encompass the half-life of IL-Ra (Wu and Huang, 2018). The drugs that contain protein from the flow are quickly removed by degradation via enzymes and through biochemical and biophysical clearance. IL-Ra has a low molecular weight and is quickly eliminated from the kidneys through biochemical and biophysical clearance (Lan et al., 1995). The clearance of IL-Ra from the body occurs through different mechanisms, including quick distribution in organs, metabolism in the liver, and quick removal from the kidney, thus leading to changes in the healing dosage of therapeutic agents (Kim et al., 1995).
IL-Ra takes part in normal physiological functions by using specific antibodies and acts as an anti-inflammatory agent in several models of diseases. In humans, endogenous IL-Ra is expressed in diseases and is also described as the most important part of host defense (Evans, 2018).
Biochemistry of interleukin-1 receptor antagonist
IL-Ra protein is purified from the supernatants of cultured monocytes on adherent IgG (Arend and Leung, 1994). A combination of reverse phase chromatography, anion exchange and gel filtration in the purification scheme results in over 10,000-fold purification and a 3% yield. IL-Ra is incorporated with S-methionine in cultured monocytes on adherent IgG. Thus, the process of purification is observed by bioactivity assays. The IL-Ra derived from monocytes consists of three purified forms analyzed by the N-terminal sequence; the size of two forms is 22 kDa and that of the third form is 17 kDa (Perrier et al., 2002). N-terminal analysis of the two forms was successful and revealed amino acids identical through 20 residues and the third form of IL-Ra exhibited a blocked N-terminus. Peptides obtained after digestion of the three forms of IL-Ra with endo-proteinases were then sequenced that revealed an assembly after composition analysis. Absorption with N-glycanase indicated that the 22 kDa form of IL-Ra is glycosylated, while the digested molecules constitute the size of 17 kDa. After N-glycanase digestion, the size of the 17 kDa form remains unchanged. In summary, the IgG-stimulated monocyte supernatants possess three IL-Ra peptide forms: two glycosylated 22 kDa forms and a non-glycosylated 17 kDa form. The presence of N-linked carbohydrate is not necessary to maintain organic activity as the three forms showed the same level of activity in the two bioassays, i.e., production of IL-1 induced PGE2 by fibroblasts and nitrogen induced proliferation of IL-1 augmented murine thymocytes. Multiple IL-Ra forms exist in the 22–25 kDa size range and reflect varying degrees of glycosylated peptide (Arend, 1990).
Based on the analysis of protein sequence, cDNA for IL-Ra is cloned successfully (Howard et al., 1998). From the cultured monocytes, RNA is harvested on IgG for 17 h and is used in πg10 for the preparation of cDNA library. This library is secreted using two synthesized probes of oligonucleotide and a single clone is identified which is hybridized with both probes. Digestion of EcoRI releases a DNA of 1.8 kb insert and hybridized to a total of 5 probes. CDNA is cloned into the vector of expression in M13wp19 which is a sequence of nucleotide.
An active reading frame that encodes for a peptide of 177 amino acids and a 3-untranslated long stretch with 5-untranslated stretch of 14 nucleotides was formed by the cDNA for the inhibition of interleukin-1 receptors. The residues of 25 N-terminal of a protein powerfully suggest a sequence of leader peptides. The established peptide consists of 152 amino acids and is created by immediate cleavage after the 25th residue exhibits the sequence of N-terminal which is identified to native disinfected IL-1Ra. Its isoelectric point is 5.2 which is identical to natural 17 kDa IL-1Ra. This cDNA is seen in Escherichia coli having successful 17 kDa recombinant protein production which inhibited the bioactivity of IL-1 (Arend, 1990). IL-Ra-derived monocyte has a unique structure and is similar to IL-1. Amino acid sequence comparison revealed the homology of 18% of IL-1α and 30% of IL-1β. At the level of amino acid, human IL-1β and IL-1α are homologous (26%) to each other. Many of the homologies sequenced between IL-Ra and IL-1β are located in the C-terminal short segment of the molecule (Rivers-Auty et al., 2018).
An identical protein is purified from the cell line of human myelomonocytic supernatants after stimulation with GM-CSF and differentiation in phorbol myristate acetate. The protein is sequenced partially, and a cDNA is cloned, expressed, and reveals an identical sequence of nucleotide to monocyte-derived cDNA. Equal forms of IL-Ra proteins are cleansed from the human urine and THP-1cells of PMA supernatants (Silva et al., 2019).
The size of the non-glycosylated IL-Ra form is reportedly larger or between 17–21 kDa (Hamilton et al., 2008). Even yet, this molecule's predicted MW is 115, 17 kDa, and it has been sequenced. The genomic DNA structure of IL-Ra proves that the molecule is an IL-1 family member. The human IL-Ra gene possesses 3 introns and 4 exons that are similar to the gene structure for IL-1β and IL-1α. The difference occurs in the first axon in which the IL-Ra gene encodes for a peptide that is not present in IL-1. Gene family is originated by the duplication of genes from the primordial precursor. IL-1α, IL-1β and IL-Ra genes are situated on the human chromosome (Arend, 1990).
The production of IL-Ra occurs through the monocytes of humans, depending on the specific generalization of protein through the culture placed on the substrate of immune complexes which are adherent or sometimes adherent IgG. The inhibited bioactivity was not evaluated in the monocytes which belong to the supernatants and were cultured with complexes of soluble immune molecules, especially with red blood cells that are coated with IgG (Arend, 1990).
Monocytes of human blood express the gene for IL-Ra on stimulation (Ruiz de Souza et al., 1995). IL-Ra protein is visualized during the first 4–6 h. In cells, the primary transcript is IL-Ra which has no peptide and diffused stains are present in cytosol although, the remaining is present intracellularly. The production of IL-Ra occurs in keratinocytes while the attachment of an IL-Ra to the nuclear DNA is blocked by epithelial cells (Espat et al., 1994).
Synthesis of IL-1Ra in monocytes
Human monocytes regulate the production of IL-Ra that is produced at the mRNA level (Tron et al., 1988). Adherent human monocytes produce equal amounts of IL-Ra proteins for 24 hours in a culture media. The half-life of mRNA is the same as that of IL-1β and IL-Ra in the cells which are induced with LPS (Dinarello, 2018). Monocytes which are induced with IgG have a high and sustained rate of transcription in IL-Ra and have a significant prolongation in the stability of mRNA. LPS is responsible for the synthesis of IL-Ra in human monocytes. Numerous other findings have demonstrated the various mechanisms by which IL-Ra production takes place. The mononuclear cells of the blood in peripheries were placed in the suspended culture at the lower end of polypropylene tubes. Production of IL-Ra was increased by adding 1% human AB serum to the suspended culture over 24 h (Granowitz et al., 1992). The soluble IgG also increased the synthesis of IL-1Ra. IgG has low induction of IL-1Ra. The production of IL-Ra was persuaded by growth factors, especially after a very short time of serum starvation (Raja et al., 2018). The IgG mediates effects on the production of IL-Ra in human monocytes by the receptors on Fc portion of IgG (Arend, 1993).
Synthesis of IL-Ra by other cells
Many other cells are involved in the synthesis of IL-1Ra. As compared to the monocytes; an in vitro culture of macrophages led to an increase in the level of production of IL-Ra. The in vitro macrophages revealed the production of IL-Ra without any stimulation, but GM-CSF increased the level of synthesis without any secretion. To produce IL-Ra proteins by macrophages that are aligned by the mRNA level of IL-Ra in which transcription is involved which is the major process (Gabay et al., 1997). The level of synthesis in macrophages is not affected by LPS or culture on the adherent IgG. The synthesized IL-Ra is 22 to 25 kDa glycosylated species while the lowest molecular weight is found in the cell.
IL-Ra is also synthesized by alveolar macrophages in large amounts. The alveolar macrophages are acquired from the fluid of Broncho-alveolar in patients with intestinal lung disease and produce inhibitory activity of IL-1 during in vitro culture. The binding of IL-1α with thymoma cells of EL4-6-1 murine is affected by the inhibitory activity or with murine thymocytes (Bird et al., 1987). During in vitro culture, IL-Ra mRNA is produced by non-stimulate alveolar macrophages of humans. The synthesis of IL-Ra through alveolar macrophages is increased by the in vitro culture of IL-1 or serum (Arend, 1993).
The synthesis of IL-Ra also occurs from fluid or tissues of synovial macrophages (Mehta et al., 2019). Fluid from synovial macrophage is acquired from patients afflicted with inflammatory arthritis which releases the inhibitory bioactivity of IL-1 during in vitro culture.
In rheumatoid arthritis patients, IL-Ra is also present in the synovial tissues of macrophages from the synovium of rheumatoid arthritis and osteoarthritis patients. Chondrocytes in the culture from the articular cartilage produced IL-Ra (Jayasuriya et al., 2012). IL-Ra is derived from neutrophils and macrophages in synovial fluid and the fibroblasts in the synovial tissues (Dinarello, 1994).
Peritoneal macrophages, hepatocytes and cells of uterine stromal
Macrophages are refined through the peritoneal fluid of females who have undergone laparoscopy for the evaluation of infertility. With the aid of PCR primers, the secreted IL-Ra transcript is isolated from the lining of hepatoma cells as well as from liver RNA in 30% of females who have no disease, no inflammatory adhesions, and neither stage 1 nor stage 2 endometriosis that exhibits elevated amounts of IL-Ra. IL-Ra is also present in ovarian cells (Syrop and Halme, 1986). These are derived from cells of granulosa in ovaries and also play a major role of self-regulation in the ovary.
Fibroblasts are also responsible for the synthesis of IL-1Ra from the skin biopsies of human adults (Kanangat et al., 2006). Amplificative PCR of reverse-transcribed mRNA showed that IL-1Ra mRNA occurs in fibroblast cells with an increased level in the culture. In PMA-stimulated fibroblast cells, a low level of IL-1Ra mRNA is shown (Chan et al., 1992). The detection of IL-1Ra occurs in non-stimulated as well as PMA-induced cells and they can be detected through ELISA. The IL-1Ra is the major form of synthesized protein through dermal fibroblasts (Chan et al., 1992). Therefore, the synovial fibroblasts can produce IL-1Ra protein (Arend, 1993).
The surface of IL-1 consists of three binding sites that separately interact with the extracellular portion having three Ig domains of the IL-1 receptor (Krumm et al., 2014). Contact points for the binding of receptors are different among IL-1Ra, IL-1α, and IL-1β. IL-1 performs functions in vivo on immune and inflammatory cells. IL-1β peptide studies separate the molecular regions that are responsible for mediating the functions. Synthetic nona-peptide exhibits in vivo immunomodulatory properties associated with inflammation during the absence of metabolic changes. A monoclonal antibody in a region of IL-1β inhibits in vivo immuno-stimulation effect in mice without changing the pyrogenic activity. Peptides contained in human pro-IL-1ß residues 208 to 240 mediate the inflammatory effects and pyrogenicity of the molecule (Borthwick, 2016). Inducing inflammatory events, IL-1β regions mediate the action on immune cells by binding to IL-1RI and IL-1RII.
The structural feature of IL-1Ra binding depicts the absence of an agonist effect. In mature IL-1β, mutation at position 11 in arginine to glycine reduces the bioactivity of the molecule towards T-cells (100-fold) and reduces the binding of receptors by 25%. Without any change in receptor binding affinity, in mature IL-1β the mutation occurs at position 145 from aspartate to lysine and leads to a reduction of the biological activity (90%) towards T-cells (Dinarello, 1996). Instead of indicating the presence or absence of active sites, mutations affect IL-1 activities by changing the conformation. IL-1β and IL-1α contain large loops and have β-pleated sheets in an anti-parallel manner. IL-1Ra and IL-1β have similar secondary and tertiary structures, but different regions in the primary sequence contain β-strands. IL-1Ra and IL-1β exhibit a difference in the location of the charged side chain which is important in the binding of receptors and activation (Dinarello and Thompson, 1991).
At position 11 of IL-1β, arginine to glycine induces gene expression earlier in fibroblasts rather than stimulating the late gene expression. There is an equal binding of IL-1Ra to IL-1RI but it triggers at the level of cellular response. The occurrence of a late process of transcription involves the interaction between the ligand and receptor but is absent in IL-1β or IL-1Ra. Reduction in biological activities occurs due to mutation in arginine to glycine at position 11 of IL-1β and amino acid substitution at the same site does not produce similar changes in the function. The analysis of molecular modeling indicates that mutation in arginine to glycine results in the disruption of the structural integrity of 1/12 pair of anti-parallel β-strand. The β-strand 1 collapses into the hydrated space between strands 4, 2, and 1, and structurally alters the cleft in IL-1β which contains conserved amino acid clusters. Aspartate 145 amino acid shows delayed biological activity of IL-1. An initial trigger for biological activities of IL-1 resides in the β-bulge domain that is located between IL-1β 4 and 5 strands and IL-1β 11 mutein. These structural features that are not involved in mediating the activities of IL-1β are present in IL-1Ra. In IL-1Ra, mutation at position 145 in lysine to aspartate led to a reduction of the agonist activities towards both the fibroblasts and T-cells (Arend, 1993).
The binding of epitope receptor for IL-1Ra and IL-1β are formed by rim’s charged surface of the band structure of β-pleated and hydrophobic core which is represented by two side chains of phenylalanine of mature IL-1β at positions 150 and 46 (Evans et al., 1995). Two structural features that mediate IL-1β biological activities are absent in IL-1Ra; a region around residue 145 of aspartic acid and β-bulge between strands 5 and 4. As IL-1α and IL-1β do not possess similar structures, activation and binding of the receptor by IL-1α involves distinct structural determinants (Dinarello and Thompson, 1991).
Balance between the production of IL-1Ra and IL-1
IL-1Ra is produced in response to various inflammatory or contagious diseases in humans and septic animals (Arend, 2002). IL-1 secretion, amount, and receptor antagonist are balanced in most diseases. Protein synthesis and gene expression of IL-1Ra and IL-1 are differentially regulated. For example, before IL-1Ra, IL-1β is synthesized and transcribed in cells. Cozzolino and coworkers, and Rambaldi, argued that dysregulation in agonist-antagonist synthesis occurs in different disorders when they studied the gene expression of IL-1Ra and IL-1β in fresh cells from individuals suffering from acute myelogenous leukemia (Rambaldi et al., 1993). The cells of leukemia from one of the 11 patients expressed IL-1Ra after the stimulation of IL-1β. Large amounts of circulating IL-1Ra are found in systemic rheumatoid arthritis during sepsis in humans and experimental endotoxemia (Schulte et al., 2013). Circulating IL-1β levels exceed 500 pgml-1 after 3 to 4 h, which then rapidly fall; whereas in the same individuals, peak IL-1Ra level occurs after 4 h, reaches 100-fold concentration of IL-1β and is retained for 12 h. The peak level of IL-1Ra in baboons occurs after 8–10 h during an E. coli sepsis. Thus, the huge and small amounts of IL-1Ra and IL-1β production respectively show a real response in most clinical situations. Patients’ synovial fluid with a disease of rheumatoid arthritis is measured by comparing the levels of IL-1β and IL-1Ra (Dinarello and Thompson, 1991). IL-1Ra produced endogenously contributes to limiting disease severity but is inadequate in overwhelming an acute inflammation or infection (Dinarello et al., 2012a). Exogenous IL-1Ra provides beneficial effects in animal models.
IL-1Ra is secreted into an extracellular compartment and consists of the classical signal peptide, although less than 10% IL-1α and 50% IL-1β are secreted in cell culture (Iyer and Cheng, 2012). 50% IL-1Ra is associated with the cells in adherent monocytes. IL-1Ra intracellular form is described in keratinocytes without a signal peptide and suggests that in these cells, intracellular IL-1Ra encounters the biological activity of IL-1α that remains in the keratinocyte’s cytosolic compartment. Although it does not happen in human monocytes exposed to endotoxin, intracellular IL-1 is 10% less than the amount of IL-1Ra that is still present in the cells. For normal physiological functions in both extracellular and intracellular components, a balance between IL-1Ra and IL-1 is important.
There are numerous types of IL-1 receptors as described in Table 1. But there are two major types, i.e., type I and type II. Type I receptors transduce the signals while type II receptors attach to IL-1 but cannot transduce the signals (Krakauer and Oppenheim, 1998). Fusion and secretion of IL-1Ra occur in the cell (Khayata et al., 2020).
Secretion of IL-1Ra in epithelial cells
Epithelial cells along with keratinocytes also produce IL-1Ra variant forms. For the IL-1Ra protein, mRNA encodes the developed portion that is the same as the IL-1Ra monocyte, but it varies in the structure of the leader peptide. Monocyte IL-1Ra holds signals sequence 25 amino acid; IL-1Ra keratinocyte contains a truncated sequence of 7-amino acid. The N-terminal consist of the very first 4 amino acids same as with the IL-1Ra leader peptide monocyte and the remaining residues are different. IL-1Ra keratinocyte lacks the sequence of a full signal which is not secreted and remains in the cell. IL-1Ra is found in the skin in large quantities which are limited to the stratum corneum. Production of IL-1Ra increases with an in vivo differentiation of keratinocytes and IL-1Ra forms in the assay of murine thymocytes exhibited an equivalent biological potency (Lee et al., 2018).
The biological relevance of IL-1Ra intracellular variants in epithelial cells has been explored. IL-1Ra serves a role of intracellular signaling which is shown by the finding of an anti-sense IL-1α oligo-deoxy nucleotide when transferred into an endothelial cell that prevents senescence and expands the cell’s proliferative life span. The IL-1Ra molecule participates with an IL-1 for the intracellular responses and controls the effects of IL-1 in differentiation and the growth of the cell (Arend, 1990).
Structural and functional relationship of the IL-1 family
The family of IL-1 consists of IL-1α, IL-1β, and IL-1Ra. From previous studies, it is known that it took 350 million years to evolve IL-1α and IL-1β as a peptide, but only IL-1Ra evolved as a signal peptide. Early members have a molecular mass of 31 kDa when they are first formed and are precursor proteins. The pro and mature IL-1α are both biologically active. Many other growth factors of cytokines like neurotropic factors that have low leading sequences are active in the form of precursors. As compared to these, IL-1β is inactive and also needs cleavage to 17 kDa peptide to maintain biological activity. The precursor of IL-1Ra contains a leader sequence and is cleaved in the mature form and secreted in form of proteins. Another form of IL-1Ra is secreted in the cells i.e., intracellularly. The intracellular secretion of IL-1Ra is the result of replacing the first exon coding on the signal peptide. Both forms are identical. IL-1Ra blocks the cell surface (Symons et al., 1995). IL-1Ra appears as an intracellular protein in the cells of the epithelial layer where it works to block intracellular IL-1Ra. In keratinocytes of the skin, the terminal differentiation occurs by balancing between IL-1Ra and IL-1α (Zheng et al., 2019). In human beings, IL-1α, IL-1β, and IL-1Ra are present at the arm of chromosome 2. Polymorphism of IL-1 gene in human diseases is linked with autoimmune diseases (Dinarello, 1994).
Thymocytes proliferation of augmented IL-1, synthesis of chondrocyte collagenase, endothelial cell adhesiveness induced IL-1 for neutrophils, and synthesis of PGE2 by IL-1 that induces synovial cell is blocked by recombinant IL-1Ra (Chow and Chin, 2020). The protein kinase activation ability of IL-1 in fibroblasts is also blocked by IL-1Ra that follows IL-1 binding to cells. 10-1000-fold IL-1Ra inhibit 50% IL-1 induced in vitro responses; due to the presence of IL-1 receptor cells in excess as compared to the number required to trigger the IL-1 biological response. In IL-6 from human monocytes, TNF and IL-1 production is blocked by IL-1Ra and at equimolar ratios, inhibition of 50% is observed (Jang et al., 2006), although total inhibition of IL-1Ra to the IL-1 is observed at 10-fold in molar excess. This indicates that the effect of ‘spare receptor’ in cells that express the receptor of type I is not important in cells that express the type II receptor.
IL-1 activity injected into animals is also blocked by the recombinant IL-1Ra but, the biological effect of cytokine is blocked in vitro by an excess of IL-1Ra as shown in Table 2. For example, human IL-1ß having potency (100 ng kg−1) for intravenous injection into the rabbits induces a high-grade fever. By injecting 10 µg kg−1 of IL-1Ra, 50% response is blocked, and complete blockage is observed with further injected IL-1Ra (100 µg kg−1). In rabbits, hypotension is induced by IL-1 due to the same dose relationship. In mice, IL-1Ra blocks the induced inflammation of IL-1. When IL-1 is to be injected into a peritoneum, the local accumulation of neutrophils is blocked by IL-1Ra. Circulating IL-6 levels by induction of IL-1 is also blocked by IL-1Ra and IL-1-induced neutrophilia is blocked by IL-1Ra (Dinarello and Thompson, 1991).
IL-1Ra has the ability to bind the IL-1 with the receptor’s cell surface without inducing any intracellular agonist effect (Volarevic et al., 2010). The agonist effect is absent in vitro and is observed in multiple cells, i.e., human fibroblasts, murine thymocytes, human monocytes, rabbit chondrocytes, human endothelial cells, neutrophils and macrophages (Dumas et al., 2012). The presence of hormone-like molecules or a naturally occurring cytokine which is a receptor antagonist is unique in biology. IL-1Ra shows a slight agonist effect at high concentrations in different assays (Dinarello and Thompson, 1991).
IL-1Ra binds to IL-1RI which is 80 kDa and is present in endothelial cells, T-cells, chondrocytes, and fibroblasts (Dripps et al., 1991). The binding of human IL-1Ra with IL-1RI on synovial fibroblast cells of cultured rheumatoid has similar kinetics as those observed with the T-cells. IL-1Ra binds to the cells with Ki of 134 pM and Kd of 213 pM in an adherent culture and these values are similar to the binding of IL-1Ra. These two ligands bind on two different regions of IL-1RI’s extracellular portion and sterically inhibit the binding with each other (Wei et al., 2014).
IL-1Ra in humans binds with recombinant murine IL-1RIIs in a manner similar to IL-1α, which indicates that the receptors are not sterically available in the intact cell (Peters et al., 2013). In comparison to IL-1RIs, human IL-1RIIs bind to IL-1ß with an affinity that is ten times greater than that of IL-1 and IL-1Ra, which exhibit comparable binding to one another (Dinarello, 1998). Binding among IL-1α or an IL-1ß to the IL-1RIIs is competitively inhibited by the IL-1Ra on human neutrophils and associates with the IL-1RIs than IL-1RIIs. This contrast is biologically similar even if IL-1RI can transduce an intracellular signal. IL-1RIIs exhibit a small cytoplasmic domain and are functionally inert (Fields et al., 2019).
IL-1Ra blocks the mediated effect of IL-1r on human monocytes, thus inhibiting the production and induction of TNF-a, IL-6, and IL-1 in cells (Dinarello, 2018). IL-1ß is chemically cross-linked with 68-kDa IL-1RII monocytes, so the biological response of IL-1 is mediated by higher affinity IL-1RIs. IL-1Ra addition into the monocytes for about 8 h after stimulation of IL-1ß results in inhibiting the responses (Granowitz et al., 1992). The results show that IL-1 receptors are recycled on cells or cell’s subpopulation shows IL-1 receptors are newly acquired during the culture.
Binding of IL-1Ra soluble IL-1R
Adult human IL-1RI is a polypeptide that contains 552 amino acids with cytoplasmic, transmembrane and extracellular domains of 213, 20, and 319 residues, respectively. In comparison, an adult human IL-1RII is a protein containing 386 amino-acids with transmembrane, extracellular, and cytoplasmic domains of 26, 332, and 29 residues, respectively. These IL-1R types are Ig superfamily members that possess three Ig domains in extracellular portions (Krakauer and Oppenheim, 1998). Instead of all three IL-1 ligands that bind to both types of IL-1R, an extracellular portion of two receptors exhibits a 28% homogeneity in the sequence of amino acids. The greatest conversation areas in the extracellular portion of IL-1R are nearer to domains 2 and 3 of the C and N terminals respectively. These areas show the contact points for the binding of ligands. The structural differentiation between these two IL-1Rs is present in intracytoplasmic portions in which IL-1RII possesses a short structure of 29 amino-acid. Thus, IL-1RII does not induce any intracellular signal and is internalized after the binding of a ligand.
Soluble murine IL-1R is prepared by showing a constructed cDNA and contains an extracellular portion of 316 amino acids. The extracellular portion contains N-terminal residues of intact IL-1RI and is not present in the shortened protein. The 60 kDa protein is a soluble IL-1RI containing a carbohydrate, and the digestion by N-glycanase reduces the molecular weight by up to 34 kDa (Svenson et al., 1993). The soluble IL-1RI binds to IL-1α which is equal to the IL-1RI’s intact cell surface. A soluble IL-1RI is not naturally occurring and is present in normal human serum in small amounts. The interaction between a serum binding factor, which is 60 kDa, and IL-1Ra is inhibited by the antibodies of rabbits that are specific for the slightly soluble form of IL-1RI. The serum binding factor which is purified interacts with high affinity with IL-1Ra but does not inhibit the binding of IL-1Ra and IL-1β. A recombinant soluble IL-1RI binds 200-fold to IL-1Ra more actively than to IL-1α or IL-1ß which differs from the cell surface of IL-1RI which binds three IL-1 forms having similar affinities (Svenson et al., 1995).
The variations that occur in soluble IL-1RI with the C-terminal structure lead to changes in the relative binding of IL-1Ra, IL-1α, and IL-1β as shown in Table 3. The foregoing results indicate that soluble IL-1RI are present in normal serum in normal amounts and selectively binds to IL-1Ra. The protein-bounded IL-1Ra is capable of interacting with the cell surface receptors that were not determined. Soluble IL-1RI also serves to deliver IL-1Ra into the tissues and maintain it in circulation. Soluble IL-1RIIs are described in synovial fluids, supernatants of human mononuclear cells of PHA-stimulated and human B cells, and normal human plasma. A protease inhibitor, i.e., serine, prevents the release of soluble IL-1RII from human cells, which suggests that it might be cleaved proteolytically from the cell surface (Miller and McGee, 2002). The purification of the binding factor shows that a 47 kDa protein binds an IL-1β with an equal affinity as the surface of cell IL-1RII; although, the soluble IL-1RII fails to bind IL-1Ra and IL-1α that differ from the surface of the cell. IL-1RII weakly binds to the IL-1Ra and IL-1α. The soluble IL-1RII inhibits the binding of IL-1ß with the T-cell line and human B cells but does not inhibit the binding of IL-1α with the T-cell line. The soluble IL-1RII is found in body fluids and human plasma and serves as a selective inhibitor of IL-1β in vivo (Dinarello and Thompson, 1991).
Strategies to encompass the biological half-life of IL-1Ra
Low molecular weight drugs containing proteins are rapidly cleared through the process of deprivation and filtration by the kidneys. The common processes for the quick removal of protein-containing drugs from the flow are degradation by enzymes and mechanisms of biochemical and biophysical clearance. IL-1 receptor antagonist has a low molecular weight and is removed from the kidneys quickly through the mechanism of biochemical and biophysical clearance. The different mechanisms for the clearance of IL-1 receptor antagonist body involve quick distribution in organs of the body, liver metabolism,s and quick removal from the kidneys, all of which lead to changes in the healing dosage of therapeutic agents (Akash et al., 2013a).
Broadly, the materials are categorized into three groups: 1- fusion with polymers and nanoparticles for extension of serum half-life; 2-by the dissolution of loaded particles of IL-1Ra for local or sustained delivery; 3-by gels and injectable coacervate for local or sustained IL-1Ra delivery as described in Table 4. Similarly, to improve the therapeutic efficacy as shown in Table 5 of IL-1Ra, injectable materials are used to extend IL-1Ra half-life and to sustain or localize IL-1Ra delivery as shown in Figs. 1 and 2.
Figure 1: Strategies to encompass the biological half-life of IL-1Ra.
Figure 2: Strategies for the delivery of IL-1Ra are widely divided into 3 different categories 1. Fusion with the polymers 2. Sustained or local delivery through gels and the injectable coacervate 3. Sustained or local delivery through nano- and micro-particles.
IL-1Ra takes part in normal physiology by using specific antibodies and acts as a natural anti-inflammatory agent. Thus, IL-1Ra possesses an anti-inflammatory potential and can be used as a therapeutic agent against inflammatory disorders, including rheumatoid arthritis. Endogenous IL-1Ra expression in diseases of humans is also described, as the molecule is the most important part of host defense in human beings.
Impact of IL-1Ra on human abnormalities
In the following sections, we briefly describe the potential role of IL-1Ra in various diseases.
The blood level of IL-1Ra is increased in patients with juvenile chronic arthritis, SLE, RA, and polymyositis (Nikfar et al., 2018). The serum levels in patients with lupus are increased and correlated to the activity of the disease, along with increased levels of IL-1Ra mRNA in peripheral myocytes and increased formation of IL-1Ra when the culture of these cells sticks on IgG. IL-1 receptor antagonist also circulates through the liver. Patients with rheumatoid arthritis have a lower percentage of IL-1Ra as compared to patients with OA and osteomyelitis. The synthesis of IL-1Ra is decreased in RA patients. In the monocytes of blood, the synthesis of IL-1Ra is increased in response to treatment with gold injections and methotrexate (Dinarello et al., 2012b).
The level of IL-1Ra is also increased in the synovial fluids of patients with RA (Kay and Calabrese, 2004). The main source in synovial rheumatoid patients is neutrophils, but there is a low formation of IL-1 receptor antagonists when compared to neutrophils of peripheral blood. IL-1Ra plays an important role in patients with Lyme disease and knee arthritis. The proportion of IL-1Ra is higher in the synovial fluid of patients with acute arthritis and recovers faster than chronic ones. In RA and OA patients, IL-1Ra causes tissue damage through stimulation of neutral proteinase formation by the cells present in the synovium and through chondrocytes in articular cartilage. Chondrocytes synthesized IL-1Ra. The synthesis and level of IL-1Ra present in balanced amounts are important for pathophysiologic activities in cartilage in patients with OA and RA.
An increased level of IL-1Ra was present in the plasma of patients following surgery, with adult sepsis, neonatal sepsis, and also in patients with fever (de Bont et al., 1995). The in vitro synthesis of IL-1Ra was not regulated in the cells of monocytes and neutrophils in patients with an increased level of IL-1Ra after surgery. This indicated that IL-1Ra was derived from the liver instead of the cells of peripheral blood. Intravenous (IV) endotoxins cause a rapid increase in the levels of plasma IL-1Ra in human beings. The maximum plasma IL-1Ra was 100-fold more than that of IL-11β (Mehta et al., 2019). Hydro cortisol infusion in normal volunteers caused increased levels of IL-1Ra. The response of glucocorticoids showed that the macrophages are the main and first source of IL-1Ra circulating in the blood immediately after injection of endotoxin. Administration of IV injection of TNF into human beings causes enhanced levels of IL-1Ra in plasma. Increased levels of plasma IL-1Ra are also seen in asymptomatic HIV infection. IL-1 receptor antagonist synthesized endogenously is the main and necessary regulator of HIV (Corley, 2000).
Thus, the above descriptions show that maintenance of the levels of IL-1Ra and IL-1 is the main factor for host resistance in patients with HIV infection. Endogenous IL-1Ra has a significant influence on both hepatitis and granulomatous illness. In patients with TB having granulomatous lesions, an increased level of IL-1Ra was demonstrated. iFN and TNF were the main inducers of IL-1Ra synthesis. This establishes the importance of endogenous IL-1Ra in organ damage and host response to infection.
Diseases of the central nervous system
All three members of the IL-1 family are important in diseases of the central nervous system as they are present in the brain (Hewett et al., 2012). The IL-1Ra can cross the barrier between the blood and the brain, and in proteins present in the brain at both peripheral and local origins. The level of plasma IL-1Ra is increased in patients with stroke and has a direct relation with infarct. In systemic inflammation, IL-1Ra was found in the anterior pituitary. IL-1Ra plays an anti-inflammatory role in ischemia and the neuroendocrine system.
The presence of IL-1Ra is also investigated in the large intestine of patients with inflammatory bowel disease (Ludwiczek et al., 2004). A smaller quantity of IL-1Ra was present in the colon tissues of patients with Crohn’s disease and ulcerative colitis. The decreased ratio of IL-1 receptor antagonists is also seen in patients with acute inflammatory bowel disease. Anti-IL-1Ra antibody increased inflammation of the intestine and the rate of mortality. The decreased level of IL-1Ra synthesis increased the chronic inflammation in inflammatory bowel disease.
An increased level of IL-1Ra led to tissue homogenate in patients with idiopathic pulmonary fibrosis (Kolb et al., 2001). In type II pneumocystis and asthmatic patients, an elevated level of IL-1Ra has been found. Moreover, fibroblasts and macrophages were also found at increased levels in their bronchial epithelium. In patients with neutrophil-induced airway inflammation, a relative amount of IL-1Ra is required. For a good prognosis of anti-inflammatory cytokines, a relative amount of IL-1Ra is important in patients with ARDS. Further studies showed that in the inflammation of the lungs, IL-1Ra plays the role of an intrinsic regulator.
The presence of IL-1Ra has also been investigated in kidney diseases (Shu et al., 2000). The level of IL-1Ra was increased in normal children as compared to children with poly-nephritis. In patients with chronic renal failure, an increased amount of plasma IL-1Ra was also determined.
During the gestation period and at the time of delivery, IL-1Ra performs several functions in the ovary and uterus. The complete ovarian extract contains IL-1Ra. In the development process, IL-1Ra was found in follicles and thecal cells but the level of IL-1Ra was increased during ovulation in thecal cells. In human amniotic fluids, increased concentrations of IL-1Ra can also be detected. A high level of IL-1Ra was also detected in the third trimester of pregnancy. In females, an increased concentration of IL-1Ra was present in the amniotic fluid and neonatal urine than in males. Thus, an increased level is helpful in preterm labor and prevents intrauterine infection (Arend, 1993).
Genetic variations in the CIASI gene at a single nucleotide level lead to alterations in the protein NLRP3, which could ultimately produce inflammation, with the potential to induce cryopyrin-associated periodic syndrome. This is called auto-inflammatory disease which involves familial cold auto-inflammatory syndrome. Inflammatory diseases such as Muckle Wall syndrome, long-lasting infantile neurologic, dermal and arthritis syndrome occur due to increased secretion of IL-1β. This involves the auto-inflammatory disease group of which the major syndrome is the TNF-receptor-1-associated syndrome. The decreased level of IL-1Ra and increased expression of IL-1β induce pro-inflammatory effects.
According to an investigational study, it has been found that IL-1 possesses a mediational function in the development of tumors and turned healthy normal cells into cancerous cells. Mature T cell leukemia cells recently obtained from individuals with leukemia were encouraged to proliferate by human IL-1β and IL-1α (Shirakawa et al., 1989). Compared to regular T cells, such cells had greater concentrations of the IL-1 receptor. Interestingly, the introduction of anti-IL1 tended to decrease multiplication, suggesting that the development of these recently separated leukemia cells depended upon an autocrine impact of IL-1. The biological properties of IL-1 in numerous myelomas are congruent with clinical manifestations of the illness, such as osteolytic bone lesions or the migration of myeloma cells toward the bone marrow (Lust and Donovan, 1999). Perhaps, IL-1 development in the pre-myeloma stage plays a crucial role in the development of active myeloma, although this is as yet unknown.
Chronic inflammation and malignancy
IL-1β resolves the acute inflammation which leads to the initiation of the responses of adaptive anti-tumor. Conditions of long-lasting inflammation enhance the possibility of cancer. IL-1 receptors expressed in human breast cancer tissues lead to the activation of cells and take part in angiogenesis, proliferation of tumors, and invasion of tumors in the microenvironment. Recent observations indicated that patients with inflammation promoted by tumors due to IL-1ß, which increases the risk of breast cancer, could benefit from IL-1 blocking therapeutics. In this report, it is shown that the downregulation of gene expressions for IL-1Ra enhances the gene expression of NK cells and CTLs (Kaneko et al., 2019).
The preventive impacts of soluble IL-1 receptors (sIL1R), recombinant IL-1Ra, as well as neutralizing antibodies to IL-1ß and IL-1α have suggested the role of IL-1 in a number of animal illness models. Such drugs reduced the magnitude of graft vs. host disorder as well as overall survival of cardiac allografts in rats, increased longevity in rabbits and rats given endotoxin injections, significantly reduced shock in baboons as well as rabbits with bacteremia, declined the prevalence and mortality of inflammatory arthritis in rabbits, mice, and rats, significantly reduced colonic inflammation in rabbits as well as rats, and hindered innovative autoimmune encephalomyelitis in mice (Arend et al., 1998; Dinarello and Wolff, 1993). The collagen-induced arthritis concept in mice provides an illustration of the therapeutic advantage of inhibiting IL-1; however, a discussion of many of these experiments is outside the purview of this paper. When neutralizing antibodies to IL-1β and IL-1α were given while arthritis appeared, the condition was either slowed or stopped; or in the animals that did, arthritis merely manifested as a minor array of abnormalities (van den Berg et al., 1994). Anti-IL1β and anti-IL-1α treatment also dramatically decreased the synovial invasion, inflammation, as well as cartilage degradation in mice with developed arthritis. The capability of chondrocytes to generate novel cartilage matrix elements was recovered after anti-IL1 therapy.
Hyperglycemia and hyperlipidemia
In diabetic GK rats, hyperglycemia or hyperlipidemia perform a regulatory influence in inducing the release of IL-1 from pancreatic islets, resulting in cell malfunction (Gaisano et al., 2002; Larsen et al., 2009; Maedler et al., 2002). GK rats have increased levels of inflammatory and pro-inflammatory factors, including TNFα, IL-1β, IL-6 and MIP-1α, CD53+ and CD68+, etc., that drive the inflammation and compromised the pancreatic β cells functionality. Moreover, elevated levels of FFAs, TC/HDL, TC, TGs, and/or HDL have been reported which own-regulate the glucose-induced insulin response (Akash et al., 2013b; Briaud et al., 2002; Ehses et al., 2009; Wallis et al., 2004). Excessive amounts of circulating lipid profiles can cause vascular smooth muscles and endothelial cells to release pro-inflammatory chemokines and/or cytokines, increasing oxidative stress (Akash et al., 2013b). Such inflammatory reactions circulate among pancreatic islet beta-cells as well as generate additional pro-inflammatory chemicals that could cause beta-cell degeneration (Akash et al., 2013b; Akash et al., 2012; Dinarello, 2011). Strong affinity IL-1Ra attaches to IL-1RI-cells without eliciting a reaction or stopping IL-1 from adhering. Identical outcomes showing that IL-1Ra reduced lipid profiles were also published in other places (Akash et al., 2013b; Ehses et al., 2009).
A naturally existing anti-inflammatory antagonist of the pro-inflammatory cytokine family is known as IL-1Ra. The broad-spectrum anti-inflammatory properties of IL-1Ra have been studied in relation to a number of auto-immune illnesses, including diabetes and rheumatoid arthritis. In vitro production of the IL-1Ra is induced in human monocytes, via culture on the adherent IgG or culture with intravenous IgG preparations. The inhibited bioactivity is not examined in the monocytes which belong to supernatants and are cultured with complexes of soluble immune molecules, especially with red blood cells which are coated with IgG. IL-1Ra controls several physiological functions via specific antibodies, particularly the IgG family, and acts as an anti-inflammatory protein in models of diseases. IL-1Ra is synthesized in the tissues during the period of active disease and is also systematically measured. The maintenance between levels of IL-1Ra and IL-1 is the main factor for host resistance in patients with HIV infection. Endogenous IL-1Ra expression in diseases of humans is also described as the molecule with the most important role of host defense in human beings. Administration of IL-1Ra to animals is the first step of gene therapy in patients with rheumatoid arthritis.
Funding Statement: Mohammed A. Assiri appreciates the support of the Research Center for Advanced Materials Science (RCAMS) at King Khalid University Abha, Saudi Arabia, through Grant (KKU/RCAMS/22).
Author Contributions: KR and AA wrote and drafted the manuscript. MI and MAA contributed to the in-depth discussion and conception. KR and MSHA revise and finalize the manuscript. All authors approved the final version of the manuscript.
Availability of Data and Materials: All data generated or analyzed during this study are included in this published article.
Ethics Approval: Not applicable.
Conflicts of Interest: The authors declare that they have no conflicts of interest to report regarding the present study.
References
Akash MSH, Rehman K, Chen S (2013a). IL-1Ra and its delivery strategies: Inserting the association in perspective. Pharmaceutical Research 30: 2951–2966. https://doi.org/10.1007/s11095-013-1118-0 [Google Scholar] [PubMed] [CrossRef]
Akash MSH, Rehman K, Chen S (2013b). Role of inflammatory mechanisms in pathogenesis of type 2 diabetes mellitus. Journal of Cellular Biochemistry 114: 525–531. https://doi.org/10.1002/jcb.24402 [Google Scholar] [PubMed] [CrossRef]
Akash MSH, Shen Q, Rehman K, Chen S (2012). Interleukin-1 receptor antagonist: A new therapy for type 2 diabetes mellitus. Journal of Pharmaceutical Sciences 101: 1647–1658. https://doi.org/10.1002/jps.23057 [Google Scholar] [PubMed] [CrossRef]
Arend WP (1990). Interleukin-1 receptor antagonist: Discovery, structure and properties. Progress in Growth Factor Research 2: 193–205. https://doi.org/10.1016/0955-2235(90)90018-F [Google Scholar] [PubMed] [CrossRef]
Arend WP (1993). Interleukin-1 receptor antagonist. Advances in Immunology 54: 167–227. https://doi.org/10.1016/S0065-2776(08)60535-0 [Google Scholar] [PubMed] [CrossRef]
Arend WP (2002). The balance between IL-1 and IL-1Ra in disease. Cytokine & Growth Factor Reviews 13: 323–340. https://doi.org/10.1016/S1359-6101(02)00020-5 [Google Scholar] [PubMed] [CrossRef]
Arend WP, Leung DY (1994). IgG induction of IL-1 receptor antagonist production by human monocytes. Immunological Reviews 139: 71–78. https://doi.org/10.1111/j.1600-065X.1994.tb00857.x [Google Scholar] [PubMed] [CrossRef]
Arend WP, Malyak M, Guthridge CJ, Gabay C (1998). Interleukin-1 receptor antagonist: Role in biology. Annual Review of Immunology 16: 27–55. https://doi.org/10.1146/annurev.immunol.16.1.27 [Google Scholar] [PubMed] [CrossRef]
Bird TA, Gearing AJ, Saklatvala J (1987). Murine interleukin-1 receptor: Differences in binding properties between fibroblastic and thymoma cells and evidence for a two-chain receptor model. FEBS Letters 225: 21–26. https://doi.org/10.1016/0014-5793(87)81124-9 [Google Scholar] [PubMed] [CrossRef]
Borthwick LA (2016). The IL-1 cytokine family and its role in inflammation and fibrosis in the lung. Seminars in Immunopathology 38: 517–534. https://doi.org/10.1007/s00281-016-0559-z [Google Scholar] [PubMed] [CrossRef]
Briaud I, Kelpe CL, Johnson LM, Tran POT, Poitout V (2002). Differential effects of hyperlipidemia on insulin secretion in islets of langerhans from hyperglycemic versus normoglycemic rats. Diabetes 51: 662–668. https://doi.org/10.2337/diabetes.51.3.662 [Google Scholar] [PubMed] [CrossRef]
Chan LS, Hammerberg C, Kang K, Sabb P, Tavakkol A, Cooper KD (1992). Human dermal fibroblast interleukin-1 receptor antagonist (IL-1ra) and interleukin-1β (IL-1β) mRNA and protein are co-stimulated by phorbol ester: Implication for a homeostatic mechanism. Journal of Investigative Dermatology 99: 315–322. https://doi.org/10.1111/1523-1747.ep12616653 [Google Scholar] [PubMed] [CrossRef]
Chow YY, Chin KY (2020). The role of inflammation in the pathogenesis of osteoarthritis. Mediators of Inflammation 2020: 8293921. https://doi.org/10.1155/2020/8293921 [Google Scholar] [PubMed] [CrossRef]
Corley PA (2000). Interleukin-1 receptor antagonist as a treatment of HIV infection. Medical Hypotheses 54: 513–518. https://doi.org/10.1054/mehy.1998.0817 [Google Scholar] [PubMed] [CrossRef]
de Bont ES, de Leij LH, Okken A, Baarsma R, Kimpen JL (1995). Increased plasma concentrations of interleukin-1 receptor antagonist in neonatal sepsis. Pediatric Research 37: 626–629. https://doi.org/10.1203/00006450-199505000-00012 [Google Scholar] [PubMed] [CrossRef]
Dinarello CA (1994). The interleukin-1 family: 10 years of discovery 1. The FASEB Journal 8: 1314–1325. https://doi.org/10.1096/fasebj.8.15.8001745 [Google Scholar] [CrossRef]
Dinarello CA (1996). Biologic basis for interleukin-1 in disease. Blood 87: 2095–2147. https://doi.org/10.1182/blood.V87.6.2095.bloodjournal8762095 [Google Scholar] [CrossRef]
Dinarello CA (1998). Interleukin-1, interleukin-1 receptors and interleukin-1 receptor antagonist. International Reviews of Immunology 16: 457–499. https://doi.org/10.3109/08830189809043005 [Google Scholar] [PubMed] [CrossRef]
Dinarello CA (2011). Blocking interleukin-1β in acute and chronic autoinflammatory diseases. Journal of Internal Medicine 269: 16–28. https://doi.org/10.1111/j.1365-2796.2010.02313.x [Google Scholar] [PubMed] [CrossRef]
Dinarello CA (2018). Overview of the IL-1 family in innate inflammation and acquired immunity. Immunological Reviews 281: 8–27. https://doi.org/10.1111/imr.12621 [Google Scholar] [PubMed] [CrossRef]
Dinarello CA, Novick D, Kim S, Kaplanski G (2013). Interleukin-18 and IL-18 binding protein. Frontiers in Immunology 4: 289. https://doi.org/10.3389/fimmu.2013.00289 [Google Scholar] [PubMed] [CrossRef]
Dinarello CA, Simon A, van der Meer JW (2012a). Treating inflammation by blocking interleukin-1 in a broad spectrum of diseases. Nature Reviews Drug Discovery 11: 633–652. https://doi.org/10.1038/nrd3800 [Google Scholar] [PubMed] [CrossRef]
Dinarello CA, Simon A, van der Meer JWM (2012b). Treating inflammation by blocking interleukin-1 in a broad spectrum of diseases. Nature Reviews Drug Discovery 11: 633–652. https://doi.org/10.1038/nrd3800 [Google Scholar] [PubMed] [CrossRef]
Dinarello CA, Thompson RC (1991). Blocking IL-1: Interleukin 1 receptor antagonist in vivo and in vitro. Immunology Today 12: 404–410. https://doi.org/10.1016/0167-5699(91)90142-G [Google Scholar] [PubMed] [CrossRef]
Dinarello CA, Wolff SM (1993). The role of interleukin-1 in disease. The New England Journal of Medicine 328: 106–113. https://doi.org/10.1056/NEJM199301143280207 [Google Scholar] [PubMed] [CrossRef]
Dripps DJ, Brandhuber BJ, Thompson RC, Eisenberg SP (1991). Interleukin-1 (IL-1) receptor antagonist binds to the 80-kDa IL-1 receptor but does not initiate IL-1 signal transduction. Journal of Biological Chemistry 266: 10331–10336. https://doi.org/10.1016/S0021-9258(18)99230-6 [Google Scholar] [CrossRef]
Dumas A, Lagarde S, Laflamme C, Pouliot M (2012). Oncostatin M decreases interleukin-1β secretion by human synovial fibroblasts and attenuates an acute inflammatory reaction in vivo. Journal of Cellular and Molecular Medicine 16: 1274–1285. https://doi.org/10.1111/j.1582-4934.2011.01412.x [Google Scholar] [PubMed] [CrossRef]
Ehses J, Lacraz G, Giroix M-H, Schmidlin F, Coulaud J, Kassis N et al. (2009). IL-1 antagonism reduces hyperglycemia and tissue inflammation in the type 2 diabetic GK rat. PNAS 106: 13998–14003. https://doi.org/10.1073/pnas.0810087106 [Google Scholar] [PubMed] [CrossRef]
Espat NJ, Rogy MA, Copeland EM, Moldawer LL (1994). Interleukin-1, interleukin-1 receptor, and interleukin-1 receptor antagonist. Proceedings of the Nutrition Society 53: 393–400. https://doi.org/10.1079/PNS19940044 [Google Scholar] [PubMed] [CrossRef]
Espírito-Santo RF, Meira CS, Costa RDS, Souza Filho OP, Evangelista AF, Trossini GHG et al. (2017). The anti-inflammatory and immunomodulatory potential of braylin: Pharmacological properties and mechanisms by in silico, in vitro and in vivo approaches. PLoS One 12: e0179174. https://doi.org/10.1371/journal.pone.0179174 [Google Scholar] [PubMed] [CrossRef]
Evans C (2018). Editorial: Arthritis gene therapy using interleukin-1 receptor antagonist. Arthritis & Rheumatology 70: 1699–1701. https://doi.org/10.1002/art.40675 [Google Scholar] [PubMed] [CrossRef]
Evans RJ, Bray J, Childs JD, Vigers GP, Brandhuber BJ, Skalicky JJ et al. (1995). Mapping receptor binding sites in interleukin (IL)-1 receptor antagonist and IL-1β by site-directed mutagenesis. Identification of a single site in IL-1ra and two sites in IL-1β. Journal of Biological Chemistry 270: 11477–11483. https://doi.org/10.1074/jbc.270.19.11477 [Google Scholar] [PubMed] [CrossRef]
Gabay C, Smith MF, Eidlen D, Arend WP (1997). Interleukin 1 receptor antagonist (IL-1Ra) is an acute-phase protein. Journal of Clinical Investigation 99: 2930–2940. https://doi.org/10.1172/JCI119488 [Google Scholar] [PubMed] [CrossRef]
Gaisano HY, Ostenson CG, Sheu L, Wheeler MB, Efendic S (2002). Abnormal expression of pancreatic islet exocytotic soluble N-ethylmaleimide-sensitive factor attachment protein receptors in Goto-Kakizaki rats is partially restored by phlorizin treatment and accentuated by high glucose treatment. Endocrinology 143: 4218–4226. https://doi.org/10.1210/en.2002-220237 [Google Scholar] [PubMed] [CrossRef]
Granowitz EV, Clark BD, Vannier E, Callahan MV, Dinarello CA (1992). Effect of interleukin-1 (IL-1) blockade on cytokine synthesis: I. IL-1 receptor antagonist inhibits IL-1-induced cytokine synthesis and blocks the binding of IL-1 to its type II receptor on human monocytes. Blood 79: 2356–2363. https://doi.org/10.1182/blood.V79.9.2356.2356 [Google Scholar] [CrossRef]
Hamilton BS, Brede Y, Tolbert TJ (2008). Expression and characterization of human glycosylated interleukin-1 receptor antagonist in Pichia pastoris. Protein Expression and Purification 59: 64–68. https://doi.org/10.1016/j.pep.2008.01.003 [Google Scholar] [PubMed] [CrossRef]
Hewett SJ, Jackman NA, Claycomb RJ (2012). Interleukin-1β in central nervous system injury and repair. European Journal of Neurodegenerative Diseases 1: 195–211. [Google Scholar] [PubMed]
Howard RD, McIlwraith CW, Trotter GW, Nyborg JK (1998). Cloning of equine interleukin 1 receptor antagonist and determination of its full-length cDNA sequence. American Journal of Veterinary Research 59: 712–716. [Google Scholar] [PubMed]
Huang Y, Hu L, Yang YQ, Hu XP, Zhen YS, Liu MY (2012). Construction of IL-1Ra-HSA fusion protein and analysis of its bioactivity and pharmacokinetics. Acta Pharmaceutica Sinica 47: 1210–1218. [Google Scholar] [PubMed]
Iyer SS, Cheng G (2012). Role of interleukin 10 transcriptional regulation in inflammation and autoimmune disease. Critical Reviews in Immunology 32: 23–63. https://doi.org/10.1615/CritRevImmunol.v32.i1.30 [Google Scholar] [PubMed] [CrossRef]
Jang CH, Choi JH, Byun MS, Jue DM (2006). Chloroquine inhibits production of TNF-α, IL-1β and IL-6 from lipopolysaccharide-stimulated human monocytes/macrophages by different modes. Rheumatology 45: 703–710. https://doi.org/10.1093/rheumatology/kei282 [Google Scholar] [PubMed] [CrossRef]
Jayasuriya CT, Goldring MB, Terek R, Chen Q (2012). Matrilin-3 induction of IL-1 receptor antagonist is required for up-regulating collagen II and aggrecan and down-regulating ADAMTS-5 gene expression. Arthritis Research & Therapy 14: R197. https://doi.org/10.1186/ar4033 [Google Scholar] [PubMed] [CrossRef]
Kanangat S, Postlethwaite AE, Higgins GC, Hasty KA (2006). Novel functions of intracellular IL-1ra in human dermal fibroblasts: Implications in the pathogenesis of fibrosis. Journal of Investigative Dermatology 126: 756–765. https://doi.org/10.1038/sj.jid.5700097 [Google Scholar] [PubMed] [CrossRef]
Kaneko N, Kurata M, Yamamoto T, Morikawa S, Masumoto J (2019). The role of interleukin-1 in general pathology. Inflammation and Regeneration 39: 1–16. https://doi.org/10.1186/s41232-019-0101-5 [Google Scholar] [PubMed] [CrossRef]
Kay J, Calabrese L (2004). The role of interleukin-1 in the pathogenesis of rheumatoid arthritis. Rheumatology 43: iii2–iii9. https://doi.org/10.1093/rheumatology/keh201 [Google Scholar] [PubMed] [CrossRef]
Khayata M, Shah NP, Verma BR, Giugni AS, Alkharabsheh S et al. (2020). Usefulness of interleukin-1 receptor antagonists in patients with recurrent pericarditis. American Journal of Cardiology 127: 184–190. https://doi.org/10.1016/j.amjcard.2020.03.041 [Google Scholar] [PubMed] [CrossRef]
Kim DC, Reitz B, Carmichael DF, Bloedow DC (1995). Kidney as a major clearance organ for recombinant human interleukin-1 receptor antagonist. Journal of Pharmaceutical Sciences 84: 575–580. https://doi.org/10.1002/jps.2600840511 [Google Scholar] [PubMed] [CrossRef]
Kolb M, Margetts PJ, Anthony DC, Pitossi F, Gauldie J (2001). Transient expression of IL-1β induces acute lung injury and chronic repair leading to pulmonary fibrosis. Journal of Clinical Investigation 107: 1529–1536. https://doi.org/10.1172/JCI12568 [Google Scholar] [PubMed] [CrossRef]
Krakauer T, Oppenheim JJ (1998). Interleukin 1 and its receptors. In: Delves PJ (ed.Interleukin 1 and Its Receptors, pp. 1429–1435. Oxford: Elsevier. [Google Scholar]
Fields JK, Günther S, Sundberg EJ (2019). Structural basis of IL-1 family cytokine signaling. Frontiers in Immunology 10: 1412. https://doi.org/10.3389/fimmu.2019.01412 [Google Scholar] [PubMed] [CrossRef]
Krumm B, Xiang Y, Deng J (2014). Structural biology of the IL-1 superfamily: Key cytokines in the regulation of immune and inflammatory responses. Protein Science 23: 526–538. https://doi.org/10.1002/pro.2441 [Google Scholar] [PubMed] [CrossRef]
Lan HY, Nikolic-Paterson DJ, Mu W, Vannice JL, Atkins RC (1995). Interleukin-1 receptor antagonist halts the progression of established crescentic glomerulonephritis in the rat. Kidney International 47: 1303–1309. https://doi.org/10.1038/ki.1995.185 [Google Scholar] [PubMed] [CrossRef]
Larsen CM, Faulenbach M, Vaag A, Ehses JA, Donath MY, Mandrup-Poulsen T (2009). Sustained effects of interleukin-1 receptor antagonist treatment in type 2 diabetes. Diabetes Care 32: 1663–1668. https://doi.org/10.2337/dc09-0533 [Google Scholar] [PubMed] [CrossRef]
Lee H, Cheong KA, Kim JY, Kim NH, Noh M, Lee AY (2018). IL-1 receptor antagonist reduced chemical-induced keratinocyte apoptosis through antagonism to IL-1α/IL-1β. Biomolecules & Therapeutics 26: 417–423. https://doi.org/10.4062/biomolther.2017.167 [Google Scholar] [PubMed] [CrossRef]
Lieschke GJ, Rao PK, Gately MK, Mulligan RC (1997). Bioactive murine and human interleukin-12 fusion proteins which retain antitumor activity in vivo. Nature Biotechnology 15: 35–40. https://doi.org/10.1038/nbt0197-35 [Google Scholar] [PubMed] [CrossRef]
Liu M, Huang Y, Hu L, Liu G, Hu X, Liu D et al. (2012). Selective delivery of interleukine-1 receptor antagonist to inflamed joint by albumin fusion. BMC Biotechnology 12: 68. https://doi.org/10.1186/1472-6750-12-68 [Google Scholar] [PubMed] [CrossRef]
Ludwiczek O, Vannier E, Borggraefe I, Kaser A, Siegmund B, Dinarello CA et al. (2004). Imbalance between interleukin-1 agonists and antagonists: Relationship to severity of inflammatory bowel disease. Clinical and Experimental Immunology 138: 323–329. https://doi.org/10.1111/j.1365-2249.2004.02599.x [Google Scholar] [PubMed] [CrossRef]
Lust JA, Donovan KA (1999). The role of interleukin-1β in the pathogenesis of multiple myeloma. Hematology/Oncology Clinics of North America 13: 1117–1125. https://doi.org/10.1016/S0889-8588(05)70115-5 [Google Scholar] [PubMed] [CrossRef]
Maedler K, Sergeev P, Ris F, Oberholzer J, Joller-Jemelka HI, Spinas GA et al. (2002). Glucose-induced β cell production of IL-1β contributes to glucotoxicity in human pancreatic islets. The Journal of Clinical Investigation 110: 851–860. https://doi.org/10.1172/JCI200215318 [Google Scholar] [CrossRef]
Mehta S, Akhtar S, Porter RM, Önnerfjord P, Bajpayee AG (2019). Interleukin-1 receptor antagonist (IL-1Ra) is more effective in suppressing cytokine-induced catabolism in cartilage-synovium co-culture than in cartilage monoculture. Arthritis Research & Therapy 21: 238. https://doi.org/10.1186/s13075-019-2003-y [Google Scholar] [PubMed] [CrossRef]
Miller TL, McGee DW (2002). Epithelial cells respond to proteolytic and non-proteolytic detachment by enhancing interleukin-6 responses. Immunology 105: 101–110. https://doi.org/10.1046/j.0019-2805.2001.01352.x [Google Scholar] [PubMed] [CrossRef]
Nikfar S, Saiyarsarai P, Tigabu BM, Abdollahi M (2018). Efficacy and safety of interleukin-1 antagonists in rheumatoid arthritis: A systematic review and meta-analysis. Rheumatology International 38: 1363–1383. https://doi.org/10.1007/s00296-018-4041-1 [Google Scholar] [PubMed] [CrossRef]
Orino E, Sone S, Nii A, Ogura T (1992). IL-4 up-regulates IL-1 receptor antagonist gene expression and its production in human blood monocytes. Journal of Immunology, 925–931. [Google Scholar]
Perrier S, Kherratia B, Deschaumes C, Ughetto S, Kemeny JL, Baudet-Pommel M et al. (2002). IL-1ra and IL-1 production in human oral mucosal epithelial cells in culture: Differential modulation by TGF-β1 and IL-4. Clinical and Experimental Immunology 127: 53–59. https://doi.org/10.1046/j.1365-2249.2002.01685.x [Google Scholar] [PubMed] [CrossRef]
Peters VA, Joesting JJ, Freund GG (2013). IL-1 receptor 2 (IL-1R2) and its role in immune regulation. Brain, Behavior, and Immunity 32: 1–8. https://doi.org/10.1016/j.bbi.2012.11.006 [Google Scholar] [PubMed] [CrossRef]
Qian J, Zhu L, Li Q, Belevych N, Chen Q, Zhao F et al. (2012). Interleukin-1R3 mediates interleukin-1-induced potassium current increase through fast activation of Akt kinase. PNAS 109: 12189–12194. https://doi.org/10.1073/pnas.1205207109 [Google Scholar] [PubMed] [CrossRef]
Qin J, Qian Y, Yao J, Grace C, Li X (2005). SIGIRR inhibits interleukin-1 receptor- and toll-like receptor 4-mediated signaling through different mechanisms. Journal of Biological Chemistry 280: 25233–25241. https://doi.org/10.1074/jbc.M501363200 [Google Scholar] [PubMed] [CrossRef]
Queen D, Ediriweera C, Liu L (2019). Function and regulation of IL-36 signaling in inflammatory diseases and cancer development. Frontiers in Cell and Developmental Biology 7: 317. https://doi.org/10.3389/fcell.2019.00317 [Google Scholar] [PubMed] [CrossRef]
Raja R, Lata S, Trivedi S, Banerjea AC (2018). Serum deprivation/starvation leads to reactivation of HIV-1 in latently infected monocytes via activating ERK/JNK pathway. Scientific Reports 8: 14496. https://doi.org/10.1038/s41598-018-32316-2 [Google Scholar] [PubMed] [CrossRef]
Rambaldi A, Torcia M, Dinarello CA, Barbui T, Cozzolino F (1993). Modulation of cell proliferation and cytokine production in AML by recombinant interleukin-1 receptor antagonist. Leukemia 7: S10–S12. [Google Scholar] [PubMed]
Rivers-Auty J, Daniels MJD, Colliver I, Robertson DL, Brough D (2018). Redefining the ancestral origins of the interleukin-1 superfamily. Nature Communications 9: 1156. https://doi.org/10.1038/s41467-018-03362-1 [Google Scholar] [PubMed] [CrossRef]
Ruiz de Souza V, Carreno MP, Kaveri SV, Ledur A, Sadeghi H et al. (1995). Selective induction of interleukin-1 receptor antagonist and interleukin-8 in human monocytes by normal polyspecific IgG (intravenous immunoglobulin). European Journal of Immunology 25: 1267–1273. https://doi.org/10.1002/(ISSN)1521-4141 [Google Scholar] [CrossRef]
Schulte W, Bernhagen J, Bucala R (2013). Cytokines in sepsis: Potent immunoregulators and potential therapeutic targets--An updated view. Mediators of Inflammation 2013: 165974. https://doi.org/10.1155/2013/165974 [Google Scholar] [PubMed] [CrossRef]
Shirakawa F, Tanaka Y, Oda S, Eto S, Yamashita U (1989). Autocrine stimulation of interleukin 1α in the growth of adult human T-cell leukemia cells. Cancer Research 49: 1143–1147. [Google Scholar] [PubMed]
Shu KH, Lee SH, Cheng CH, Wu MJ, Lian JD (2000). Impact of interleukin-1 receptor antagonist and tumor necrosis factor-alpha gene polymorphism on IgA nephropathy. Kidney International 58: 783–789. https://doi.org/10.1046/j.1523-1755.2000.00227.x [Google Scholar] [PubMed] [CrossRef]
Silva I, Peccerella T, Mueller S, Rausch V (2019). IL-1 beta-mediated macrophage-hepatocyte crosstalk upregulates hepcidin under physiological low oxygen levels. Redox Biology 24: 101209. https://doi.org/10.1016/j.redox.2019.101209 [Google Scholar] [PubMed] [CrossRef]
Stylianou E (2006). INTERLEUKINS | IL-1 and IL-18. In: Encyclopedia of Respiratory Medicine, pp. 350–354. New York: Elsevier Ltd. [Google Scholar]
Svenson M, Hansen MB, Heegaard P, Abell K, Bendtzen K (1993). Specific binding of interleukin 1 (IL-1) beta and IL-1 receptor antagonist (IL-1ra) to human serum. High-affinity binding of IL-1ra to soluble IL-1 receptor type I. Cytokine 5: 427–435. https://doi.org/10.1016/1043-4666(93)90032-Z [Google Scholar] [PubMed] [CrossRef]
Svenson M, Nedergaard S, Heegaard PM, Whisenand TD, Arend WP, Bendtzen K (1995). Differential binding of human interleukin-1 (IL-1) receptor antagonist to natural and recombinant soluble and cellular IL-1 type I receptors. European Journal of Immunology 25: 2842–2850. https://doi.org/10.1002/(ISSN)1521-4141 [Google Scholar] [CrossRef]
Symons JA, Young PR, Duff GW (1995). Soluble type II interleukin 1 (IL-1) receptor binds and blocks processing of IL-1 beta precursor and loses affinity for IL-1 receptor antagonist. PNAS 92: 1714–1718. https://doi.org/10.1073/pnas.92.5.1714 [Google Scholar] [PubMed] [CrossRef]
Syrop CH, Halme J (1986). A comparison of peritoneal fluid parameters of infertile patients and the subsequent occurrence of pregnancy. Fertility and Sterility 46: 631–635. https://doi.org/10.1016/S0015-0282(16)49640-8 [Google Scholar] [PubMed] [CrossRef]
Tron VA, Harley CB, Caussy D, Sauder DN (1988). In situ detection of interleukin-1 mRNA in human monocytes. Molecular Immunology 25: 439–445. https://doi.org/10.1016/0161-5890(88)90163-0 [Google Scholar] [PubMed] [CrossRef]
van den Berg WB, Joosten LA, Helsen M, van de Loo FA (1994). Amelioration of established murine collagen-induced arthritis with anti-IL-1 treatment. Clinical and Experimental Immunology 95: 237–243. https://doi.org/10.1111/j.1365-2249.1994.tb06517.x [Google Scholar] [PubMed] [CrossRef]
Vicenová B, Vopálenský V, Burtfytfšek L, Pospíšek M (2009). Emerging role of interleukin-1 in cardiovascular diseases. Physiological Research 58: 481–498. https://doi.org/10.33549/physiolres.931673 [Google Scholar] [PubMed] [CrossRef]
Volarevic V, Al-Qahtani A, Arsenijevic N, Pajovic S, Lukic ML (2010). Interleukin-1 receptor antagonist (IL-1Ra) and IL-1Ra producing mesenchymal stem cells as modulators of diabetogenesis. Autoimmunity 43: 255–263. https://doi.org/10.3109/08916930903305641 [Google Scholar] [PubMed] [CrossRef]
Wallis R, Wallace K, Collins S, McAteer M, Argoud K, Bihoreau M et al. (2004). Enhanced insulin secretion and cholesterol metabolism in congenic strains of the spontaneously diabetic (Type 2) Goto Kakizaki rat are controlled by independent genetic loci in rat chromosome 8. Diabetologia 47: 1096–1106. https://doi.org/10.1007/s00125-004-1416-5 [Google Scholar] [PubMed] [CrossRef]
Wawrocki S, Kielnierowski G, Rudnicka W, Seweryn M, Druszczynska M (2020). Interleukin-18, functional IL-18 receptor and IL-18 binding protein expression in active and latent tuberculosis. Pathogens 9: 451. https://doi.org/10.3390/pathogens9060451 [Google Scholar] [PubMed] [CrossRef]
Wei H, Wang D, Qian Y, Liu X, Fan S, Yin HS et al. (2014). Structural basis for the specific recognition of IL-18 by its alpha receptor. FEBS Letters 588: 3838–3843. https://doi.org/10.1016/j.febslet.2014.09.019 [Google Scholar] [PubMed] [CrossRef]
Wu H, Huang J (2018). Optimization of protein and peptide drugs based on the mechanisms of kidney clearance. Protein & Peptide Letters 25: 514–521. https://doi.org/10.2174/0929866525666180530122835 [Google Scholar] [PubMed] [CrossRef]
Zheng R, Longmate WM, DeFreest L, Varney S, Wu L, DiPersio CM et al. (2019). Keratinocyte integrin α3β1 promotes secretion of IL-1α to effect paracrine regulation of fibroblast gene expression and differentiation. Journal of Investigative Dermatology 139: 2029–2038. https://doi.org/10.1016/j.jid.2019.02.025 [Google Scholar] [PubMed] [CrossRef]
Cite This Article
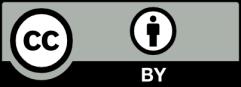