Open Access
REVIEW
Anti-fibrotic and anti-inflammatory effect of mesenchymal stromal cell-derived extracellular vesicles in chronic kidney disease
1 Department of Medical Sciences, University of Torino, Torino, 10126, Italy
2 Molecular Biotechnology Center, University of Torino, Torino, 10126, Italy
* Corresponding Authors: GIULIA CHIABOTTO. Email: ; STEFANIA BRUNO. Email:
(This article belongs to the Special Issue: Cell-Based Regenerative Therapies)
BIOCELL 2023, 47(7), 1499-1508. https://doi.org/10.32604/biocell.2023.028121
Received 30 November 2022; Accepted 20 March 2023; Issue published 21 June 2023
Abstract
Renal fibrosis and inflammation are common pathological features of chronic kidney disease (CKD). Since currently available treatments can only delay the progression of CKD, the outcome of patients with CKD is still poor. One therapeutic option for the prevention of CKD-related complications could be the use of mesenchymal stromal cells (MSCs), which have shown beneficial effects in tissue fibrosis and regeneration after damage. However, safety issues, such as cellular rejection and carcinogenicity, limit their clinical application. Among the bioactive factors secreted by MSCs, extracellular vesicles (EVs) have shown the same beneficial effect of MSCs, without any notable side effects. This heterogeneous population of membranous nano-sized particles can deliver genetic material and functional proteins to injured cells, prompting tissue regeneration. Here we describe the anti-fibrotic and anti-inflammatory properties of MSC-derived EVs in CKD preclinical models and summarize the potential molecular mechanisms involved in the regulation of renal fibrosis and inflammation.Graphical Abstract
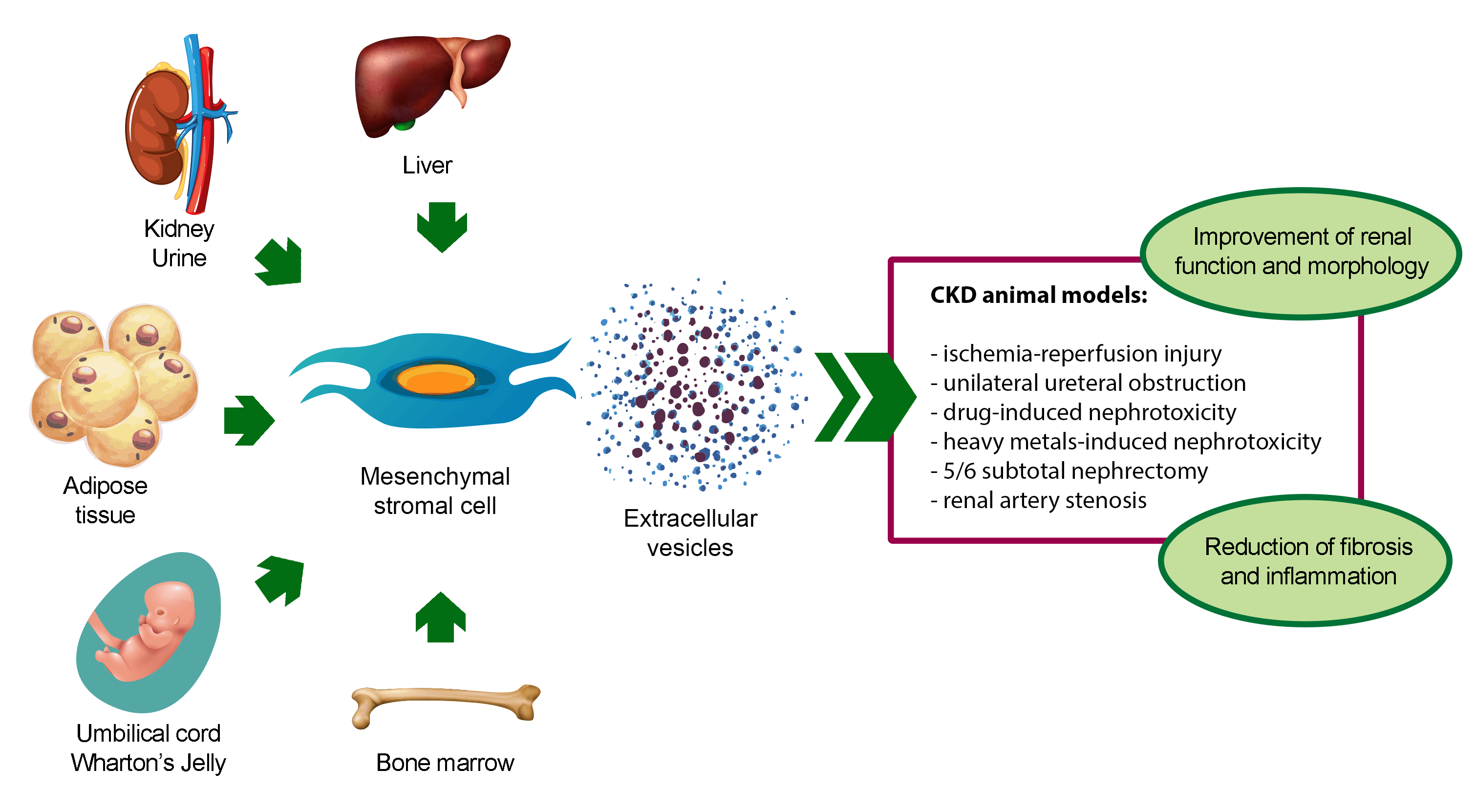
Keywords
List of Abbreviations
α-SMA | Alpha-smooth muscle actin |
AT | Adipose tissue |
AT-MSC-EV | Extracellular vesicles from adipose tissue-derived mesenchymal stromal cells |
BM | Bone marrow |
BCL2 | B-cell lymphoma 2 |
BMP7 | Bone morphogenetic protein 7 |
BM-MSC-EV | Extracellular vesicles from bone marrow-derived mesenchymal stromal cells |
CCL | Chemokine (C-C motif) ligand |
CCR2 | C-C chemokine receptor type 2 |
CKD | Chronic kidney disease |
CX3CL1 | C-X3-C motif chemokine ligand 1 |
EMT | Epithelial-to-mesenchymal transition |
EV | Extracellular vesicle |
HLSC | Human liver stem cell |
IL | Interleukin |
IRI | Ischemia-reperfusion injury |
IFNγ | Interferon gamma |
miRNA | MicroRNA |
MSC | Mesenchymal stromal cell |
MSC-EV | Mesenchymal stromal cell-derived extracellular vesicles |
TEC | Tubular epithelial cell |
TGF-β | Transforming growth factor-beta |
UC-MSC-EV | Extracellular vesicles from umbilical cord-derived mesenchymal stromal cells |
UUO | Unilateral ureteral obstruction |
Chronic kidney disease (CKD) is a progressive and irreversible loss of kidney function that could ultimately lead to end-stage renal failure, one of the major causes of mortality worldwide (Carney, 2020). CKD affects around 10% of the global population, but its incidence is expected to increase due to the enhanced prevalence of diabetes, hypertension, and obesity, which are considered important risk factors for CKD development (Jha et al., 2013; Djudjaj and Boor, 2019; GBD Chronic Kidney Disease Collaboration, 2020) (Fig. 1).
Figure 1: Risk factors associated with chronic kidney disease development and currently available treatments. bottom, two representative micrographs of Sirius Red staining of sections of a murine healthy kidney (left) and a murine CKD kidney after two months of ischemia-reperfusion injury (right). The red color highlights the presence of collagen fibrils, typical signs of fibrosis. Magnification 400x. Abbreviation: CKD: chronic kidney disease.
One of the hallmarks and the final common pathway of CKD is renal fibrosis, characterized by an excessive accumulation of extracellular matrix and responsible for the degeneration of kidney structure and function (Fig. 1). Pro-inflammatory stimuli released by damaged renal cells recruit monocytes from the circulation into the interstitial space, leading to the release of inflammatory and fibrogenic cytokines (Cho, 2010). This process triggers epithelial-to-mesenchymal transition (EMT) in fibroblasts and tubular epithelial cells (TECs), which convert into myofibroblasts and increase the production of extracellular matrix components, thus perpetuating tubulointerstitial fibrosis, glomerulosclerosis, and inflammatory cell infiltration (Liu, 2010; Menon and Ross, 2016).
At present, available therapies to slow CKD progression aim to pharmacologically treat the underlying diseases and to control blood pressure and glycemia (Fig. 1), but the outcome of CKD patients is still poor (Turner et al., 2012; Humphreys, 2018). Renal-replacement therapies, such as hemodialysis and kidney transplantation, are limited by high costs and organ availability (Abecassis et al., 2008). For these reasons, new therapeutic strategies for CKD are urgently needed.
Mesenchymal Stromal Cell-Derived Extracellular Vesicles (MSC-EVs)
Stem cell-based therapy is a novel approach that takes advantage of the pro-regenerative properties of stem cells and their bioproducts to induce the repair of damaged or dysfunctional cells and tissues in the human body (Wong, 2021). In the last decade, the therapeutic contribution of mesenchymal stromal cells (MSCs) in kidney repair and regeneration after the injury has been extensively explored (Morigi et al., 2016; Zhuang et al., 2019). Originally described as fibroblast-like cells in the bone marrow (BM) (Friedenstein et al., 1974), this population of multipotent stem cells can be obtained from different adult and fetal tissues, expanded in vitro, and differentiate into adipocytes, osteoblasts, and chondrocytes (Pittenger et al., 1999).
The pro-regenerative activity of MSC is carried out in a paracrine manner, mainly through the release of soluble nutritional factors and extracellular vesicles (EVs), nanometer-range particles surrounded by a lipid bilayer. Based on the size and biological origin, this heterogeneous population of vesicles can be classified into exosomes, ectosomes, and apoptotic bodies (Meldolesi, 2018; Bruno et al., 2020). At present, EVs can be purified from biological fluids or the supernatant of cultured cells using the ‘gold standard’ method of differential ultracentrifugation, or other more specific techniques, such as density gradient centrifugation, filtration, size exclusion chromatography, and immuno-affinity capture (Chiabotto et al., 2020).
By acting as mediators of intercellular communication, MSC-EVs can transfer a large variety of bioactive molecules between cells, including functional proteins, lipids, and microRNAs (miRNAs), and reprogram cell fate by modulating different molecular pathways (Quesenberry et al., 2015). The cargo of MSC-EVs can also be genetically modified to deliver specific therapeutic molecules to target cells (Varderidou-Minasian and Lorenowicz, 2020).
Several advantages make MSC-EVs more suitable for therapeutic use than MSCs. MSC-EVs preparations are more stable than cells since they are not subjected to deactivation, even after repeated freezing and thawing (Kou et al., 2022), and can be stored for a longer time at −80 °C (Eirin and Lerman, 2021). Due to their nanometer size and low expression of membrane histocompatibility molecules, MSC-EVs have lower immunogenicity than MSCs (Reis et al., 2016). Furthermore, being acellular products, MSC-EVs do not self-replicate and lack potentially hazardous properties, such as ectopic differentiation, genetic instability, and tumor formation (Jafarinia et al., 2020).
Renoprotective Effects of Mesenchymal Stromal Cell-Derived Extracellular Vesicles in Animal Models of Chronic Kidney Disease
The renoprotective effect of MSC-EVs has been demonstrated in different in vivo models of CKD (Table 1) (Grange et al., 2019a; Eirin and Lerman, 2021; Liao et al., 2022). To date, BM-derived MSC-EVs (BM-MSC-EVs) are the most widely used source of EVs for CKD treatment. However, also MSC-EVs from alternative sources, such as perinatal tissues, adipose tissue (AT), liver, kidney, and urine, have shown to ameliorate CKD conditions (Fig. 2).
Figure 2: Pro-regenerative effects of extracellular vesicles derived from mesenchymal stromal cells isolated from different sources in animal models of chronic kidney disease. Abbreviation: CKD: chronic kidney disease.
An important mechanism underlying the renoprotective effect of MSC-EVs consists in the modulation of markers of fibrosis and inflammation, whose expression is increased in the renal tissue of CKD animals. In different in vivo models of CKD, treatment with MSC-EVs from different sources down-regulates the expression of fibrosis-associated genes, such as alpha smooth muscle actin (α-SMA) (He et al., 2015; Choi et al., 2015; Grange et al., 2019b; Kholia et al., 2018, 2020; Wang et al., 2020; Bruno et al., 2022), transforming growth factor-β (TGF-β) (Nagaishi et al., 2016; Grange et al., 2019b; Kholia et al., 2018, 2020; Ishiy et al., 2020; Bruno et al., 2022), and collagen I (Grange et al., 2019b; Kholia et al., 2018, 2020; Ishiy et al., 2020; Bruno et al., 2022). Furthermore, the administration of MSC-EVs can effectively reduce the expression of pro-inflammatory genes, such as interferon gamma (IFNγ) (Grange et al., 2019b; Ramírez-Bajo et al., 2020), interleukin (IL)-6 (Eirin et al., 2017; Bruno et al., 2022), and tumor necrosis factor-α (Eirin et al., 2017; Grange et al., 2019b; Bruno et al., 2022), and increase the expression of anti-inflammatory genes, such as IL-10 (Eirin et al., 2017; Ishiy et al., 2020).
Ischemia-reperfusion injury (IRI)
IRI is considered a major cause of acute kidney injury. It is associated with oxidative stress and inflammation that trigger apoptosis and necrosis of TECs, leading to rapid deterioration of renal function and morphology (Tögel and Westenfelder, 2014). The maladaptive repair following acute kidney injury predisposes the progression to CKD and to the development of fibrosis. In experimental models of IRI-induced CKD, treatment with BM-MSC-EVs improves renal function and morphology, with a significant reduction of fibrosis (Gatti et al., 2011). The ameliorative effect on renal inflammation and fibrosis has also been observed using EVs isolated from umbilical cord-derived MSCs (UC-MSC-EVs) (Zou et al., 2016a, 2016b), Wharton’s Jelly-derived MSCs (Zou et al., 2014), and human liver stem cells (HLSCs) (Bruno et al., 2022). In addition to reducing the expression of fibrosis and inflammation markers, HLSC-EVs modulate several genes associated with EMT, upregulating the expression of epithelial marker zonula occludens-1, and down-regulating the expression of mesenchymal markers Twist-related protein 1 and vimentin in renal tissue (Bruno et al., 2022). By transferring vascular endothelial growth factor to TECs, UC-MSC-EVs may also increase renal angiogenesis (Zou et al., 2016a).
Unilateral ureteral obstruction (UUO)
UUO is another in vivo model of tubulointerstitial fibrosis. It is characterized by alterations in the renal cell phenotype and accumulation of excessive extracellular matrix (Chevalier et al., 2009). Another histologic alteration is renal microvasculature injury, which results in chronic tissue hypoxia, thus contributing to the progression of renal disease (Ohashi et al., 2002). In experimental models of UUO-induced CKD, treatment with BM-MSC-EVs preserved renal function and morphology and reduced fibrosis, as shown by the reduction in α-SMA expression and the increase in E-cadherin expression (He et al., 2015; Wang et al., 2020). In addition, kidney-derived MSC-EVs reduce inflammatory cell infiltration and improve peritubular rarefaction by inhibiting the endothelial-to-mesenchymal transition of peritubular capillaries (Choi et al., 2015).
The protective role of the miRNA content of BM-MSC-EVs in preventing UUO-induced CKD has been demonstrated by two different research groups (He et al., 2015; Wang et al., 2020). He and colleagues have found 81 miRNAs upregulated BM-MSC-EVs compared to BM-MSCs, including miRNAs belonging to the miR-29 and to the miR-30 family (He et al., 2015), whose anti-fibrotic effect is well-described in CKD (Lv et al., 2018). Wang and colleagues have observed a correlation between the downregulation of miR-294 and miR-133 in BM-MSC-EVs of aged rats and a reduced capability of BM-MSC-EVs to inhibit renal fibrosis (Wang et al., 2020).
CKD is frequently associated with nephrotoxicity induced by drugs, in particular immunosuppressors and antineoplastics. In general, treatment with MSC-EVs induced morphological and functional recovery of the kidney, also reducing fibrosis, EMT, and TEC apoptosis (Bruno et al., 2012; Nagaishi et al., 2016; Jiang et al., 2016; Kholia et al., 2018, 2020; Zhong et al., 2018; Grange et al., 2019b; Ramírez-Bajo et al., 2020). In cyclosporine-induced CKD, both the preventive and the curative treatment with BM-MSC-EVs attenuate renal fibrosis by down-regulating the expression of plasminogen activator inhibitor-1, TIMP metallopeptidase inhibitor 1, IFN-γ (Ramírez-Bajo et al., 2020). A protective effect on renal function and morphology was also observed in the aristolochic acid-induced CKD model, treated with BM-MSC-EVs (Kholia et al., 2020) and with HLSC-EVs (Kholia et al., 2018). In both cases, EV treatment attenuated the expression of fibrosis markers, such as α-SMA, collagen I, TGF-β, and latent transforming growth factor-beta binding protein, and restored baseline levels of several fibrosis-related miRNAs, whose expression was altered in the intoxicated kidney (Kholia et al., 2018, 2020).
Interestingly, a considerable number of fibrosis-related genes were also modulated in an experimental model of streptozotocin-induced diabetic nephropathy, treated with BM-MSC-EVs and with HLSC-EVs (Grange et al., 2019b). For these two populations of MSC-EVs, Grange and colleagues have identified distinct miRNA signatures, which include common anti-fibrotic miRNAs, such as miR-21, miR-24, miR-29a, miR-30a, and let-7 family. Moreover, Zhong and colleagues found UC-MSC-EVs enriched in miR-451a, which can restart TEC cycle by inhibiting P15 and P19 and reverse EMT in a streptozotocin-induced diabetic nephropathy model with hyperuricemia (Zhong et al., 2018). Jiang and colleagues also observed that urinary-derived MSC-EVs contain growth factors with reno-protective activity, such as TGF-β1, angiogenin, and bone morphogenetic protein-7, which can reduce podocyte apoptosis and promote vascular regeneration and cell survival in streptozotocin-induced diabetic nephropathy (Jiang et al., 2016).
Other chronic kidney disease models
Treatment with BM-MSC-EVs has been shown to improve kidney damage also in cadmium-induced nephrotoxicity (Matsukura et al., 2019) and in 5/6 subtotal nephrectomy (He et al., 2012), where it can prevent renal fibrosis by reducing tubulointerstitial collagen deposition. Similarly, Wharton’s Jelly-derived MSC-EVs ameliorated renal inflammation and fibrosis by transferring hepatocyte growth factor into the ischemic kidney subjected to partial nephrectomy (Du et al., 2021).
Finally, the anti-inflammatory and anti-fibrotic effect of EVs isolated from AT-derived MSCs (AT-MSC-EVs) was demonstrated in a rat model of renal artery stenosis (Ishiy et al., 2020) and in a porcine model of metabolic syndrome and renal artery stenosis (Eirin et al., 2017, 2018). Eirin and colleagues demonstrated that AT-MSC-EVs contain pro-angiogenic, anti-apoptotic, and anti-oxidant genes and proteins that can restore renal perfusion and ameliorate renal injury (Eirin et al., 2018). In particular, IL-10 was detected among the anti-inflammatory molecules enriched in AT-MSC-EVs, and its knockdown abolished the protective effect of MSC-EVs on the stenotic kidney (Eirin et al., 2017).
Evidence of Renoprotective Effects of Mesenchymal Stromal Cell-Derived Extracellular Vesicles in Human Patients
Several clinical trials have tested the regenerative effect of MSC-EVs in the human body. The first clinical application of MSC-EVs has been described in a patient with steroid-resistant graft vs. host disease (Kordelas et al., 2014). In this study, EV administration was shown to be safe and effective in improving cutaneous and mucosal graft vs. host disease.
The efficacy of UC-MSC-EVs has also been demonstrated in CKD by Nassar et al. (2016). Forty patients with CKD stage III or IV were enrolled in a single-center, randomized, placebo-controlled phase II/III clinical study and followed for 12 months after two administrations of UC-MSC-EVs. Compared with patients administered with placebo, treated patients showed a significant improvement in kidney function and a reduction of inflammation. As no adverse effects were observed throughout the study, EV administration has been proven safe and effective in patients with CKD.
In CKD in vivo experimental models, MSC-EVs isolated from different sources exert pro-regenerative, anti-fibrotic, and anti-inflammatory activities, showing the same therapeutic effects as MSCs. By delivering proteins, mRNAs, and non-coding RNAs into the injured kidney, MSC-EVs modulate several molecular pathways related to inflammation, fibrosis, oxidative stress, apoptosis, and angiogenesis, thus impeding the progression of CKD. Based on the promising results obtained in different in vivo experimental models, together with the results from the first-in-human study in CKD patients, MSC-EVs may represent supportive care to slow the development of CKD, postpone renal replacement therapies, and improve the quality of life of patients.
Compared with cell therapy, MSC-EVs are safer; as nano-sized particles, they possess both low immunogenicity and biocompatibility. However, there are still challenges that need to be overcome before their clinical application. First, the molecular mechanisms underlying the therapeutic effect of MSC-EVs in the kidney are not fully understood, and an in-depth analysis of the MSC-EV cargo could help to elucidate how they carry out their in vivo anti-fibrotic and anti-inflammatory effect. Second, it would be important to determine the duration of the beneficial effects of MSC-EVs and their adequate dose regimen for the clinical application. Luckily, no long-term detrimental effects of MSC-EVs have been described so far in CKD in vivo models. However, possible side effects must be taken into consideration and closely monitored before moving into clinical trials. Finally, the standardization of MSC-EV isolation and characterization techniques, based on the minimum criteria proposed by the International Society of Extracellular Vesicles, could help to increase batch reproducibility and facilitate their clinical application.
Acknowledgement: The authors would like to thank Valeria Chiabotto for her help in figure realization.
Funding Statement: The authors received no specific funding for this study.
Author Contributions: The authors confirm their contribution to the paper as follows: study conception and design: G. Chiabotto and S. Bruno; data collection: G. Chiabotto; analysis and interpretation of results: G. Chiabotto; draft manuscript preparation: G. Chiabotto and S. Bruno. Both authors reviewed the results and approved the final version of the manuscript.
Availability of Data and Materials: Data sharing not applicable to this article as no datasets were generated or analyzed during the current study.
Conflicts of Interest: The authors declare that they have no conflicts of interest to report regarding the present study.
References
Abecassis M, Bartlett ST, Collins AJ, Davis CL, Delmonico FL et al. (2008). Kidney transplantation as primary therapy for end-stage renal disease: A national kidney foundation/kidney disease outcomes quality initiative (NKF/KDOQITM) conference. Clinical Journal of the American Society of Nephrology 3: 471–480. https://doi.org/10.2215/CJN.05021107 [Google Scholar] [PubMed] [CrossRef]
Bruno S, Chiabotto G, Camussi G (2020). Extracellular vesicles: A therapeutic option for liver fibrosis. International Journal of Molecular Sciences 21: E4255. https://doi.org/10.3390/ijms21124255 [Google Scholar] [PubMed] [CrossRef]
Bruno S, Chiabotto G, Cedrino M, Ceccotti E, Pasquino C, De Rosa S, Grange C, Tritta S, Camussi G (2022). Extracellular vesicles derived from human liver stem cells attenuate chronic kidney disease development in an in vivo experimental model of renal ischemia and reperfusion injury. International Journal of Molecular Sciences 23: 1485. https://doi.org/10.3390/ijms23031485 [Google Scholar] [PubMed] [CrossRef]
Bruno S, Grange C, Collino F, Deregibus MC, Cantaluppi V, Biancone L, Tetta C, Camussi G (2012). Microvesicles derived from mesenchymal stem cells enhance survival in a lethal model of acute kidney injury. PLoS One 7: e33115. https://doi.org/10.1371/journal.pone.0033115 [Google Scholar] [PubMed] [CrossRef]
Carney EF (2020). The impact of chronic kidney disease on global health. Nature Reviews Nephrology 16: 251. https://doi.org/10.1038/s41581-020-0268-7 [Google Scholar] [PubMed] [CrossRef]
Chevalier RL, Forbes MS, Thornhill BA (2009). Ureteral obstruction as a model of renal interstitial fibrosis and obstructive nephropathy. Kidney International 75: 1145–1152. https://doi.org/10.1038/ki.2009.86 [Google Scholar] [PubMed] [CrossRef]
Chiabotto G, Pasquino C, Camussi G, Bruno S (2020). Molecular pathways modulated by mesenchymal stromal cells and their extracellular vesicles in experimental models of liver fibrosis. Frontiers in Cell and Developmental Biology 8: 594794. https://doi.org/10.3389/fcell.2020.594794 [Google Scholar] [PubMed] [CrossRef]
Cho MH (2010). Renal fibrosis. Korean Journal of Pediatrics 53: 735–740. https://doi.org/10.3345/kjp.2010.53.7.735 [Google Scholar] [PubMed] [CrossRef]
Choi HY, Lee HG, Kim BS, Ahn SH, Jung A, Lee M, Lee JE, Kim HJ, Ha SK, Park HC (2015). Mesenchymal stem cell-derived microparticles ameliorate peritubular capillary rarefaction via inhibition of endothelial-mesenchymal transition and decrease tubulointerstitial fibrosis in unilateral ureteral obstruction. Stem Cell Research & Therapy 6: 18. https://doi.org/10.1186/s13287-015-0012-6 [Google Scholar] [PubMed] [CrossRef]
Djudjaj S, Boor P (2019). Cellular and molecular mechanisms of kidney fibrosis. Molecular Aspects of Medicine 65: 16–36. https://doi.org/10.1016/j.mam.2018.06.002 [Google Scholar] [PubMed] [CrossRef]
Du T, Ju G, Zhou J, Zhong L, Rong L, Chen W, Zhang X, Zhou R, Ding D, Ji T (2021). Microvesicles derived from human umbilical cord mesenchyme promote M2 macrophage polarization and ameliorate renal fibrosis following partial nephrectomy via hepatocyte growth factor. Human Cell 34: 1103–1113. https://doi.org/10.1007/s13577-021-00525-z [Google Scholar] [PubMed] [CrossRef]
Eirin A, Lerman LO (2021). Mesenchymal stem/stromal cell-derived extracellular vesicles for chronic kidney disease: Are we there yet? Hypertension 78: 261–269. https://doi.org/10.1161/HYPERTENSIONAHA.121.14596 [Google Scholar] [PubMed] [CrossRef]
Eirin A, Zhu XY, Jonnada S, Lerman A, van Wijnen AJ, Lerman LO (2018). Mesenchymal stem cell-derived extracellular vesicles improve the renal microvasculature in metabolic renovascular disease in swine. Cell Transplantation 27: 1080–1095. https://doi.org/10.1177/0963689718780942 [Google Scholar] [PubMed] [CrossRef]
Eirin A, Zhu XY, Puranik AS, Tang H, McGurren KA, van Wijnen AJ, Lerman A, Lerman LO (2017). Mesenchymal stem cell-derived extracellular vesicles attenuate kidney inflammation. Kidney International 92: 114–124. https://doi.org/10.1016/j.kint.2016.12.023 [Google Scholar] [PubMed] [CrossRef]
Friedenstein AJ, Deriglasova UF, Kulagina NN, Panasuk AF, Rudakowa SF, Luriá EA, Ruadkow IA (1974). Precursors for fibroblasts in different populations of hematopoietic cells as detected by the in vitro colony assay method. Experimental Hematology 2: 83–92. [Google Scholar] [PubMed]
Gatti S, Bruno S, Deregibus MC, Sordi A, Cantaluppi V, Tetta C, Camussi G (2011). Microvesicles derived from human adult mesenchymal stem cells protect against ischaemia-reperfusion-induced acute and chronic kidney injury. Nephrology, Dialysis, Transplantation 26: 1474–1483. https://doi.org/10.1093/ndt/gfr015 [Google Scholar] [PubMed] [CrossRef]
GBD Chronic Kidney Disease Collaboration (2020). Global, regional, and national burden of chronic kidney disease, 1990–2017: A systematic analysis for the global burden of disease study 2017. Lancet 395: 709–733. https://doi.org/10.1016/S0140-6736(20)30045-3 [Google Scholar] [PubMed] [CrossRef]
Grange C, Skovronova R, Marabese F, Bussolati B (2019a). Stem cell-derived extracellular vesicles and kidney regeneration. Cells 8: 1240. https://doi.org/10.3390/cells8101240 [Google Scholar] [PubMed] [CrossRef]
Grange C, Tritta S, Tapparo M, Cedrino M, Tetta C, Camussi G, Brizzi MF (2019b). Stem cell-derived extracellular vesicles inhibit and revert fibrosis progression in a mouse model of diabetic nephropathy. Scientific Reports 9: 4468. https://doi.org/10.1038/s41598-019-41100-9 [Google Scholar] [PubMed] [CrossRef]
He J, Wang Y, Lu X, Zhu B, Pei X, Wu J, Zhao W (2015). Micro-vesicles derived from bone marrow stem cells protect the kidney both in vivo and in vitro by microRNA-dependent repairing. Nephrology 20: 591–600. https://doi.org/10.1111/nep.12490 [Google Scholar] [PubMed] [CrossRef]
He J, Wang Y, Sun S, Yu M, Wang C, Pei X, Zhu B, Wu J, Zhao W (2012). Bone marrow stem cells-derived microvesicles protect against renal injury in the mouse remnant kidney model. Nephrology 17: 493–500. https://doi.org/10.1111/j.1440-1797.2012.01589.x [Google Scholar] [PubMed] [CrossRef]
Humphreys BD (2018). Mechanisms of renal fibrosis. Annual Review of Physiology 80: 309–326. https://doi.org/10.1146/annurev-physiol-022516-034227 [Google Scholar] [PubMed] [CrossRef]
Ishiy CSRA, Ormanji MS, Maquigussa E, Ribeiro RS, da Silva Novaes A, Boim MA (2020). Comparison of the effects of mesenchymal stem cells with their extracellular vesicles on the treatment of kidney damage induced by chronic renal artery stenosis. Stem Cells International 2020: 8814574. https://doi.org/10.1155/2020/8814574 [Google Scholar] [PubMed] [CrossRef]
Jafarinia M, Alsahebfosoul F, Salehi H, Eskandari N, Ganjalikhani-Hakemi M (2020). Mesenchymal stem cell-derived extracellular vesicles: A novel cell-free therapy. Immunological Investigations 49: 758–780. https://doi.org/10.1080/08820139.2020.1712416 [Google Scholar] [PubMed] [CrossRef]
Jha V, Garcia-Garcia G, Iseki K, Li Z, Naicker S, Plattner B, Saran R, Wang AYM, Yang CW (2013). Chronic kidney disease: Global dimension and perspectives. The Lancet 382: 260–272. https://doi.org/10.1016/S0140-6736(13)60687-X [Google Scholar] [PubMed] [CrossRef]
Jiang Z, Liu Y, Niu X, Yin J, Hu B, Guo S, Fan Y, Wang Y, Wang N (2016). Exosomes secreted by human urine-derived stem cells could prevent kidney complications from type I diabetes in rats. Stem Cell Research & Therapy 7: 24. https://doi.org/10.1186/s13287-016-0287-2 [Google Scholar] [PubMed] [CrossRef]
Kholia S, Herrera Sanchez MB, Cedrino M, Papadimitriou E, Tapparo M et al. (2020). Mesenchymal stem cell derived extracellular vesicles ameliorate kidney injury in aristolochic acid nephropathy. Frontiers in Cell and Developmental Biology 8: 188. https://doi.org/10.3389/fcell.2020.00188 [Google Scholar] [PubMed] [CrossRef]
Kholia S, Herrera Sanchez MB, Cedrino M, Papadimitriou E, Tapparo M, Deregibus MC, Brizzi MF, Tetta C, Camussi G (2018). Human liver stem cell-derived extracellular vesicles prevent aristolochic acid-induced kidney fibrosis. Frontiers in Immunology 9: 1639. https://doi.org/10.3389/fimmu.2018.01639 [Google Scholar] [PubMed] [CrossRef]
Kordelas L, Rebmann V, Ludwig AK, Radtke S, Ruesing J, Doeppner TR, Epple M, Horn PA, Beelen DW, Giebel B (2014). MSC-derived exosomes: A novel tool to treat therapy-refractory graft-versus-host disease. Leukemia 28: 970–973. https://doi.org/10.1038/leu.2014.41 [Google Scholar] [PubMed] [CrossRef]
Kou M, Huang L, Yang J, Chiang Z, Chen S et al. (2022). Mesenchymal stem cell-derived extracellular vesicles for immunomodulation and regeneration: A next generation therapeutic tool? Cell Death & Disease 13: 118002. https://doi.org/10.1038/s41419-022-05034-x [Google Scholar] [PubMed] [CrossRef]
Liao C, Chen G, Yang Q, Liu Y, Zhou T (2022). Potential therapeutic effect and mechanisms of mesenchymal stem cells-extracellular vesicles in renal fibrosis. Frontiers in Cell and Developmental Biology 10: 824752. https://doi.org/10.3389/fcell.2022.824752 [Google Scholar] [PubMed] [CrossRef]
Liu Y (2010). New insights into epithelial-mesenchymal transition in kidney fibrosis. Journal of the American Society of Nephrology 21: 212–222. https://doi.org/10.1681/ASN.2008121226 [Google Scholar] [PubMed] [CrossRef]
Lv W, Fan F, Wang Y, Gonzalez-Fernandez E, Wang C, Yang L, Booz GW, Roman RJ (2018). Therapeutic potential of microRNAs for the treatment of renal fibrosis and CKD. Physiological Genomics 50: 20–34. https://doi.org/10.1152/physiolgenomics.00039.2017 [Google Scholar] [PubMed] [CrossRef]
Matsukura T, Inaba C, Weygant EA, Kitamura D, Janknecht R, Matsumoto H, Hyink DP, Kashiwada S, Obara T (2019). Extracellular vesicles from human bone marrow mesenchymal stem cells repair organ damage caused by cadmium poisoning in a medaka model. Physiological Reports 7: e14172. https://doi.org/10.14814/phy2.14172 [Google Scholar] [PubMed] [CrossRef]
Meldolesi J (2018). Exosomes and ectosomes in intercellular communication. Current Biology 28: R435–R444. https://doi.org/10.1016/j.cub.2018.01.059 [Google Scholar] [PubMed] [CrossRef]
Menon MC, Ross MJ (2016). Epithelial-to-mesenchymal transition of tubular epithelial cells in renal fibrosis: A new twist on an old tale. Kidney International 89: 263–266. https://doi.org/10.1016/j.kint.2015.12.025 [Google Scholar] [PubMed] [CrossRef]
Morigi M, Rota C, Remuzzi G (2016). Mesenchymal stem cells in kidney repair. Methods in Molecular Biology 1416: 89–107. https://doi.org/10.1007/978-1-4939-3584-0 [Google Scholar] [CrossRef]
Nagaishi K, Mizue Y, Chikenji T, Otani M, Nakano M, Konari N, Fujimiya M (2016). Mesenchymal stem cell therapy ameliorates diabetic nephropathy via the paracrine effect of renal trophic factors including exosomes. Scientific Reports 6: 34842. https://doi.org/10.1038/srep34842 [Google Scholar] [PubMed] [CrossRef]
Nassar W, El-Ansary M, Sabry D, Mostafa MA, Fayad T, Kotb E, Temraz M, Saad A-N, Essa W, Adel H (2016). Umbilical cord mesenchymal stem cells derived extracellular vesicles can safely ameliorate the progression of chronic kidney diseases. Biomaterials Research 20: 21. https://doi.org/10.1186/s40824-016-0068-0 [Google Scholar] [PubMed] [CrossRef]
Ohashi R, Shimizu A, Masuda Y, Kitamura H, Ishizaki M, Sugisaki Y, Yamanaka N (2002). Peritubular capillary regression during the progression of experimental obstructive nephropathy. Journal of the American Society of Nephrology 13: 1795–1805. https://doi.org/10.1097/01.ASN.0000018408.51388.57 [Google Scholar] [PubMed] [CrossRef]
Pittenger MF, Mackay AM, Beck SC, Jaiswal RK, Douglas R, Mosca JD, Moorman MA, Simonetti DW, Craig S, Marshak DR (1999). Multilineage potential of adult human mesenchymal stem cells. Science 284: 143–147. https://doi.org/10.1126/science.284.5411.143 [Google Scholar] [PubMed] [CrossRef]
Quesenberry PJ, Aliotta J, Deregibus MC, Camussi G (2015). Role of extracellular RNA-carrying vesicles in cell differentiation and reprogramming. Stem Cell Research & Therapy 6: 153. https://doi.org/10.1186/s13287-015-0150-x [Google Scholar] [PubMed] [CrossRef]
Ramírez-Bajo MJ, Martín-Ramírez J, Bruno S, Pasquino C, Banon-Maneus E et al. (2020). Nephroprotective potential of mesenchymal stromal cells and their extracellular vesicles in a murine model of chronic cyclosporine nephrotoxicity. Frontiers in Cell and Developmental Biology 8: 296. https://doi.org/10.3389/fcell.2020.00296 [Google Scholar] [PubMed] [CrossRef]
Reis M, Ogonek J, Qesari M, Borges NM, Nicholson L, Preußner L, Dickinson AM, Wang X, Weissinger EM, Richter A (2016). Recent developments in cellular immunotherapy for HSCT-associated complications. Frontiers in Immunology 7: 1343. https://doi.org/10.3389/fimmu.2016.00500 [Google Scholar] [PubMed] [CrossRef]
Turner JM, Bauer C, Abramowitz MK, Melamed ML, Hostetter TH (2012). Treatment of chronic kidney disease. Kidney International 81: 351–362. https://doi.org/10.1038/ki.2011.380 [Google Scholar] [PubMed] [CrossRef]
Tögel F, Westenfelder C (2014). Recent advances in the understanding of acute kidney injury. F1000prime Reports 6: 83. https://doi.org/10.12703/P6-83 [Google Scholar] [PubMed] [CrossRef]
Varderidou-Minasian S, Lorenowicz MJ (2020). Mesenchymal stromal/stem cell-derived extracellular vesicles in tissue repair: Challenges and opportunities. Theranostics 10: 5979–5997. https://doi.org/10.7150/thno.40122 [Google Scholar] [PubMed] [CrossRef]
Wang Y, Guo YF, Fu GP, Guan C, Zhang X, Yang DG, Shi YC (2020). Protective effect of miRNA-containing extracellular vesicles derived from mesenchymal stromal cells of old rats on renal function in chronic kidney disease. Stem Cell Research & Therapy 11: 274. https://doi.org/10.1186/s13287-020-01792-7 [Google Scholar] [PubMed] [CrossRef]
Wong CY (2021). Current advances of stem cell-based therapy for kidney diseases. World Journal of Stem Cells 13: 914–933. https://doi.org/10.4252/wjsc.v13.i7.914 [Google Scholar] [PubMed] [CrossRef]
Zhong L, Liao G, Wang X, Li L, Zhang J et al. (2018). Mesenchymal stem cells-microvesicle-miR-451a ameliorate early diabetic kidney injury by negative regulation of P15 and P19. Experimental Biology and Medicine 243: 1233–1242. https://doi.org/10.1177/1535370218819726 [Google Scholar] [PubMed] [CrossRef]
Zhuang Q, Ma R, Yin Y, Lan T, Yu M, Ming Y (2019). Mesenchymal stem cells in renal fibrosis: The flame of cytotherapy. Stem Cells International 2019: 8387350. https://doi.org/10.1155/2019/8387350 [Google Scholar] [PubMed] [CrossRef]
Zou X, Gu D, Xing X, Cheng Z, Gong D, Zhang G, Zhu Y (2016a). Human mesenchymal stromal cell-derived extracellular vesicles alleviate renal ischemic reperfusion injury and enhance angiogenesis in rats. American Journal of Translational Research 8: 4289–4299. [Google Scholar] [PubMed]
Zou X, Gu D, Zhang G, Zhong L, Cheng Z, Liu G, Zhu Y (2016b). NK cell regulatory property is involved in the protective role of MSC-derived extracellular vesicles in renal ischemic reperfusion injury. Human Gene Therapy 27: 926–935. https://doi.org/10.1089/hum.2016.057 [Google Scholar] [PubMed] [CrossRef]
Zou X, Zhang G, Cheng Z, Yin D, Du T, Ju G, Miao S, Liu G, Lu M, Zhu Y (2014). Microvesicles derived from human Wharton’s Jelly mesenchymal stromal cells ameliorate renal ischemia-reperfusion injury in rats by suppressing CX3CL1. Stem Cell Research & Therapy 5: 40. https://doi.org/10.1186/scrt428 [Google Scholar] [PubMed] [CrossRef]
Cite This Article
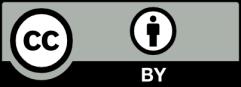