Open Access
REVIEW
The role of mesenchymal stem cell-derived exosomes in tumor progression
1 Regenerative Processing Plant, LLC, Florida, USA
2 Institute of Anatomy, University of Bern, Bern, Switzerland
3 Department of Psychology, Faculty of Medical Sciences, University of Kragujevac, Kragujevac, Serbia
4 Department of Genetics, Faculty of Medical Sciences, University of Kragujevac, Kragujevac, Serbia
5 Department of Microbiology and Immunology, Faculty of Medical Sciences, University of Kragujevac, Kragujevac, Serbia
* Corresponding Author: VLADISLAV VOLAREVIC. Email:
(This article belongs to the Special Issue: Extracellular Vesicles and Cancer)
BIOCELL 2023, 47(8), 1757-1769. https://doi.org/10.32604/biocell.2023.028567
Received 25 December 2022; Accepted 08 May 2023; Issue published 28 August 2023
Abstract
Exosomes derived from mesenchymal stem cells (MSC-Exos) are nano-sized extracellular vesicles enriched with bioactive molecules, such as microRNAs, enzymes, cytokines, chemokines, immunomodulatory, trophic, and growth factors. These molecules regulate the survival, phenotype, and function of malignant and tumor-infiltrated immune cells. Due to their nano-size and bilayer lipid envelope, MSC-Exos can easily bypass biological barriers and may serve as drug carriers to deliver chemotherapeutics directly into the tumor cells. Here, we summarize current knowledge regarding molecular mechanisms responsible for MSC-Exos-dependent modulation of tumor progression and discuss insights regarding the therapeutic potential of MSC-Exos in the treatment of malignant diseases.Graphic Abstract
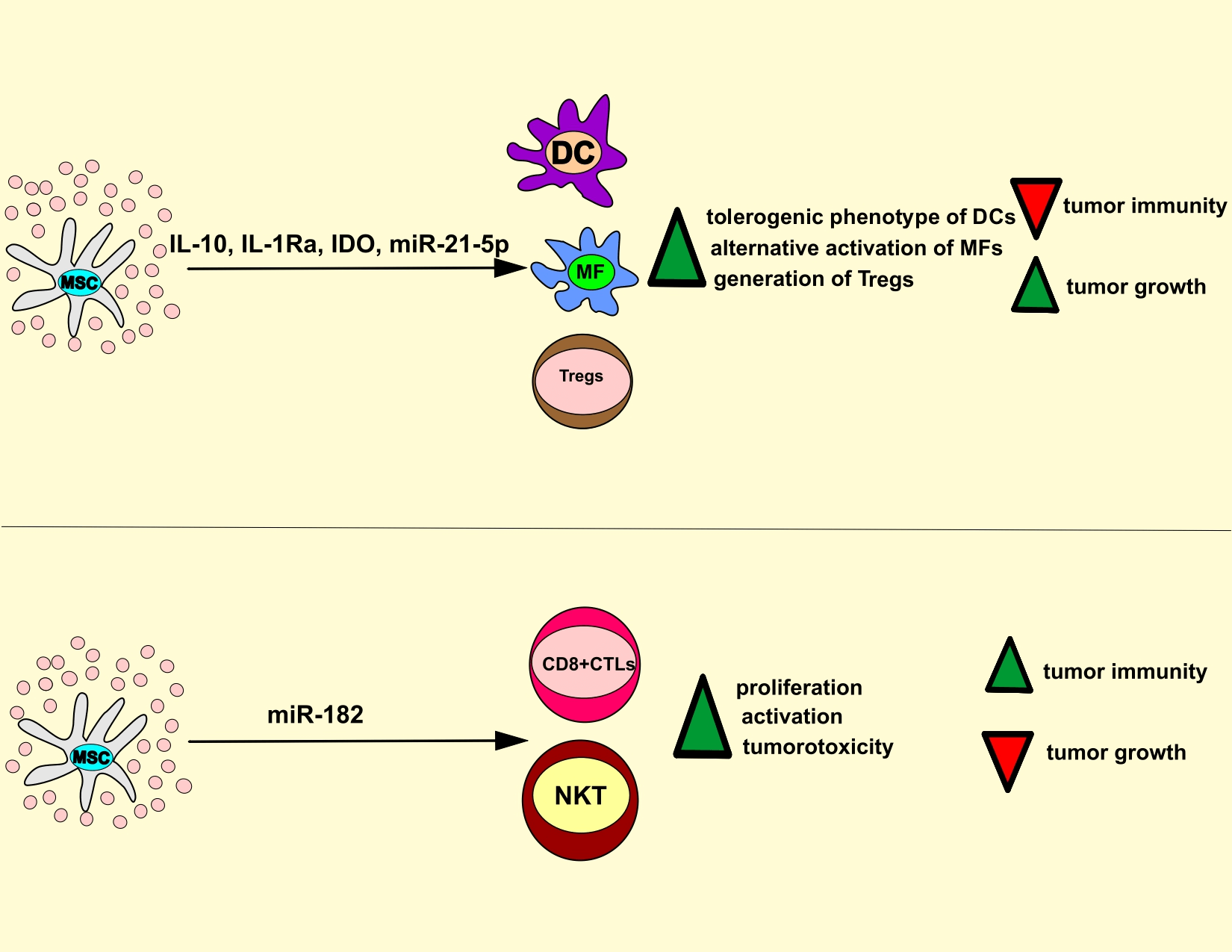
Keywords
Optimal tumor growth requires the generation of a specific microenvironment that enables enhanced viability, proliferation, invasion, and migration of malignant cells (Andreucci et al., 2022). Mesenchymal stem cells (MSC) are adult, self-renewable, rapidly proliferating stem cells that modulate the tumor microenvironment. This modulation can be in a juxtacrine manner (through the cell-to-cell contact-dependent interaction with endothelial, tumor, and immune cells) and paracrine manner (through the activity of MSC-sourced bioactive factors) (Ghollasi et al., 2021). MSCs-derived pro-angiogenic factors, such as vascular endothelial growth factor (VEGF), placental growth factor (PLGF), angiopoietin-1 (Ang1), interleukin (IL)-6, platelet-derived endothelial cell growth factor (PD-ECGF), stromal-derived factor 1 (SDF-1), hepatocyte growth factor (HGF) induce the angiogenesis in the tumor microenvironment by enhancing viability and proliferation of endothelial cells (ECs) (Ghollasi et al., 2021). These MSCs-sourced angiostimulatory factors enable optimal oxygen supply to the rapidly proliferating malignant cells, facilitating tumor growth and progression (Ghollasi et al., 2021). Additionally, MSC-derived immunoregulatory proteins, such as interleukin 1 receptor antagonist (IL-1Ra), soluble receptors of tumor necrosis factor-alpha (sTNFRs), indoleamine 2, 3-dioxygenase (IDO), kynurenine (KYN), IL-10, transforming growth factor beta (TGF-β), and nitric oxide (NO) alter phenotype and function of tumor-infiltrating immune cells and modulate immune response elicited by tumor antigens (Harrell et al., 2021). Through the activity of these immunomodulatory factors, MSCs prevent maturation and alleviate antigen-presenting properties of dendritic cells (DCs), induce the generation of immunosuppressive, alternatively activated phenotype in tumor-associated macrophages, inhibit the cytotoxicity of natural killer (NK), natural killer T (NKT), and CD8+ cytotoxic T cells (CTLs) and promote expansion of pro-tumorigenic myeloid-derived suppressor cells and FoxP3-expressing T regulatory cells. MSC-dependent suppression of anti-tumor immunity results in the uncontrolled expansion of malignant cells and crucially contributes to increased tumor growth (Harrell et al., 2021).
However, even though many experimental findings have demonstrated that MSCs promote tumor progression, MSCs are not constitutively immunosuppressive cells, and their phenotype and function depend on the cytokine milieu to which MSCs are exposed (Miloradovic et al., 2020). MSCs transplanted during the initial phase of tumor growth are exposed to tumor-derived immunosuppressive cytokines and exert a tumor-suppressive effect, while MSCs injected during the progressive stage of melanoma development are exposed to the high levels of immune cell-sourced inflammatory cytokines which induce the generation of immunosuppressive phenotype in MSCs (Miloradovic et al., 2020). These tumor-infiltrated immunosuppressive MSCs produce a large number of immunoregulatory factors, inhibit anti-tumor immunity, and enhance tumor expansion (Harrell et al., 2021).
In addition to their pro-angiogenic and immunomodulatory potential, MSCs secrete various growth factors, including epidermal growth factor, HGF, nerve growth factor, and brain-derived neurotrophic factor, prevent apoptosis and promote the viability of non-malignant, parenchymal cells (epithelial cells, hepatocytes, and neural cells) which are injured during the radio- or chemotherapy of tumor-bearing patients (Ghollasi et al., 2021). Therefore, several studies have demonstrated the beneficial effects of MSC-derived secretome in the repair and regeneration of irradiated or chemotherapeutic-injured tissues, indicating its potential therapeutic use in oncology and regenerative medicine (Zhang et al., 2022).
Essential components of MSC-sourced secretome are MSC-derived exosomes (MSC-Exos), nano-sized extracellular vesicles which contain all MSC-derived angio-modulatory and immunoregulatory proteins, growth factors, and microRNAs (miRNAs) capable of altering survival, phenotype, and function of ECs, tumor-infiltrated immune cells, and malignant cells (Zhang et al., 2022). Exosomes, which range from 30 to 120 nm in diameter and approximately 1.13–1.19 g/mL in density, are released into the extracellular environment after the fusion of late endosomes/multivesicular bodies with the plasma membrane of their parental cells (Simpson et al., 2012; Zhang et al., 2015). Since their generation process seemed to be reversed to endocytosis when they were first observed in rat and sheep reticulocytes forty years ago, these lipid, bilayer-membranous, nano-sized particles were defined as “exosomes” (Simpson et al., 2012; Zhang et al., 2015; Hessvik and Llorente, 2018).
MSC-Exos may deliver their cargo directly into the target cells and, therefore, could act as critical modulators of the tumor microenvironment (Andreucci et al., 2022). Emerging evidence has highlighted the important role of MSC-Exos in MSC-dependent suppression of anti-tumor immunity and cancer therapy resistance, indicating their pathological role in tumor growth and progression (Harrell et al., 2021). On the other side, MSC-Exos have been exploited as a novel therapeutic agent for anti-tumor treatment due to its biocompatibility, promising potential for modification, and lack of cytotoxicity (Zhang et al., 2022). MSC-Exos can be stored for long periods at −80°C (Maroto et al., 2017). Although MSC-Exos exhibit different degrees of enlargement of their diameters and protein leakage after long-term storage, they are still able to easily by-bass all biological barriers in the body (due to their lipid envelope) and, therefore, could be successfully used as vehicles for cell-specific delivery of anti-cancer agents (Maroto et al., 2017; Zhang et al., 2022). MSC-Exos are enriched with MSC-sourced miRNAs, small non-coding RNAs (nearly 18–22 nucleotides) which regulate gene expression on the posttranscriptional level by inducing degradation and/or translational inhibition of specific mRNAs (Sohrabi et al., 2022). MSC-derived miRNAs modulate angiogenesis and anti-tumor immune response and regulate the viability, proliferation, and migration of malignant cells (Sohrabi et al., 2022). Additionally, MSC-Exos transfected with synthetic miRNAs managed to significantly enhance the sensitivity of malignant cells to chemotherapeutic drugs and to remarkably improve the efficacy of anti-cancer treatment (Sohrabi et al., 2022).
To elucidate the role of MSC-Exos in tumor growth and progression, herewith, in this review article, we summarize current knowledge about molecular mechanisms that were responsible for MSC-Exos-based modulation of tumor angiogenesis and anti-tumor immunity. We also emphasize MSC-Exo-dependent alterations of intracellular signaling in tumor cells which enhance or weaken anticancer-therapy resistance and discuss the potential therapeutic use of MSC-Exos as drug delivery vehicles for cell-specific anti-cancer treatment. An extensive literature review was carried out in December 2022 across several databases (MEDLINE, EMBASE, and Google Scholar) from 1990 to the present. Keywords used in the selection were: “mesenchymal stem cells,” “exosomes,” “signaling pathway,” “tumor,” “angiogenesis,” “anti-tumor immunity,” “cancer therapy resistance,” “drug delivery,” and “anti-cancer treatment.” All journals were considered, and the initial search retrieved 327 articles. The abstracts of all these articles were subsequently reviewed independently by two authors (CRH and VV) to check their relevance to the subject of this manuscript. Eligible studies had to delineate molecular and cellular mechanisms responsible for the MSC-Exos-based modulation of tumor growth and progression, and their findings were analyzed in this review.
MSC-Exo-dependent modulation of signaling pathways that regulate proliferation and invasiveness of tumor cells
Several research groups provided evidence that MSC-Exo-sourced miRNAs enhanced proliferative and invasive characteristics of tumor cells by activating protein kinase B (PKB/AKT), extracellular signal-regulated kinase (ERK)1/2 and c-Jun N-terminal kinase (JNK)-driven signaling cascades (Fig. 1) (Zhang et al., 2022). Wang et al. (2014) showed that MSC-Exos promotes the growth and expansion of HGC-27 gastric cancer cells in a miR-221-dependent manner. MSC-Exo-sourced miR-221 down-regulates tumor suppressor genes (suppressor of cytokine signaling 1) and cyclin-dependent kinase inhibitor 1B) and promotes G1/S cell cycle transition, enabling the increased proliferation of gastric cancer cells. Lin and co-workers demonstrated that MSC-Exos-dependent activation of Wnt/β catenin-signaling is mainly responsible for increased invasiveness of MSC-Exo-primed breast cancer cells, while Du et al. (2014) later indicated AKT/ERK-dependent increased synthesis of pro-angiogenic HGF as the main mechanism through which MSC-Exos enhances metastatic potential of renal carcinoma cells (Lin et al., 2013; Du et al., 2014). Several research groups suggest that MSC-Exos increases the invasiveness of tumor cells by inducing epithelial-to-mesenchymal transition (EMT) (Zhang et al., 2022). Shi et al. (2016) found that MSC-Exos-sourced N-cadherin and vimentin activate the fibroblast growth factor 19/fibroblast growth factor receptor 4-dependent ERK signaling cascade and inducing EMT in nasopharyngeal carcinoma cells, thereby enhancing their invasive characteristics and metastatic potential, while Gu et al. (2016) demonstrated that MSC-Exos-dependent activation of AKT-driven EMT is mainly responsible for enhanced invasiveness of gastric cancer cells. MSC-sourced growth factors (such as HGF, platelet-derived growth factor (PDGF), and epidermal growth factor) induce the expression of transcriptional regulators (Slug, Snail, Zeb1, and Twistin), which promotes EMT and increase invasiveness and metastatic potential of hepatocellular and colon cancer cells. Similarly, MSC-sourced leptin increases the expression of EMT-related genes (Serpin family E member 1, IL-6, and matrix metalloproteinase-2) in gastric and breast cancers and significantly enhances their proliferative potential. Additionally, MSC-sourced TGF-β enhances the invasiveness of pancreatic cancer cells by activating the Jagged-1/Notch signaling pathway (Liang et al., 2021).
Figure 1: The effects of MSC-Exos on the signaling pathways that regulate the proliferation and invasion of tumor cells. MSC-Exo-sourced miR-221 down-regulates tumor suppressor genes, promotes G1/S cell cycle transition, and activates Wnt/β catenin-signaling in tumor cells, enabling increased proliferation of tumor cells. MSC-sourced HGF, PDGF, EGF, TGF-β, and leptin promote EMT and increase the invasiveness and metastatic potential of tumor cells. On the contrary, MSC-Exo-sourced miR-100, miR-222-3p, miR-302a, and miR-338-5p inhibit mTOR, IRF2/INPP4B and Wif1/Wnt8/β-catenin signaling pathways in malignant cells, suppressing their viability, invasiveness, and metastatic potential. EGF, epidermal growth factor; EMT, epithelial mesenchymal transition; HGF, hepatocyte growth factor; INPP4B, inositol polyphosphate-4-phosphatase type II; IRF2, interferon regulatory factor 2; MSC, mesenchymal stem cell; mTOR, mammalian target of rapamycin; PDGF, platelet-derived growth factor; TGF-β, transforming growth factor beta.
In contrast to these findings, several recently published studies indicated that MSC-Exos may suppress viability, proliferation, and metastasis of tumor cells in miR-100, miR-222-3p, miR-302a, and miR-338-5p-dependent manner (Pakravan et al., 2017; Zhang et al., 2020a; Li et al., 2019; Yao et al., 2021). MSC-sourced miR-100 inhibited the mammalian target of rapamycin (mTOR)-dependent hypoxia-inducible factor 1-alpha-driven cell cycle progression in breast cancer cells and suppressed tumor growth and progression (Pakravan et al., 2017). MSC-Exo-derived miR-222-3p targets interferon regulatory factor 2 (IRF2) gene expression in THP-1 leukemia cells to inhibit IRF2/ inositol polyphosphate-4-phosphatase type II (INPP4B)-dependent proliferation of malignant cells (Zhang et al., 2020a). In another study, miRNA-146b derived from MSCs-Exos inhibited glioma expansion in the brains of MSC-Exo-treated rats, while MSC-Exo-sourced miR-302a alleviated AKT-dependent expression of cyclin D1 in endometrial cancer cells and suppressed the progression of endometrial cancer (Li et al., 2019) Similarly, MSC-Exos inhibited proliferation of pancreatic cancer cells by suppressing Wnt inhibitory factor 1 (Wif1)/Wnt8/β-catenin axis in a miR-338-5p-dependent manner (Yao et al., 2021).
Dual effects of MSC-Exos in the regulation of tumor neovascularization
The generation of new blood vessels in rapidly proliferating tumors and the restoration of injured capillary networks in the irradiated tumors are essential for cancer growth and progression (Andreucci et al., 2022). MSC-Exos are enriched with MSC-sourced angiomodulatory miRNAs and growth factors which regulate the expression of genes that controls the generation of new blood vessels in the tumor microenvironment (Fig. 2) (Zhang et al., 2022). Studies that investigated the effects of MSC-Exos on tumor neo-angiogenesis have yielded paradoxical results. While majority of studies reported that MSC-Exos promote vasculogenesis (Bridge et al., 2012; de Luca et al., 2012; Lopatina et al., 2014; Baruah and Wary, 2020), several research groups demonstrated MSC-Exo-dependent suppression of neo-angiogenesis in the tumor microenvironment (Lee et al., 2013; Rosenberger et al., 2019; Liu et al., 2022). These contradictory results could be a consequence of the different effects of MSC-sourced angiomodulatory factors on VEGF gene expression in ECs and tumor cells (Zhang et al., 2022). MSC-Exos deliver angiogenesis-related proteins to ECs, thereby enhancing their migratory and proliferative activity (Xiao et al., 2022) Lopatina and co-workers demonstrated that PDGF regulates the secretion of extracellular vesicles by MSCs and enhances their angiogenic potential (Lopatina et al., 2014). Similarly, de Luca et al. (2012) showed that MSC-derived IL-6 promotes breast cancer growth by inducing increased proliferation of ECs and enhancing neovascularization in the tumor microenvironment. Upon binding to its receptors gp80 and gp130, MSC-sourced IL-6 increases EC proliferation via the activation of the Janus kinase (JAK)/signal transducer and activator of transcription (STAT) and mitogen-activated protein kinase (MAPK) pathways (De Luca et al., 2012).
Figure 2: Dual effects of MSC-Exos in the regulation of tumor neovascularization. MSC-Exos may promote the generation of new blood vessels in the tumor microenvironment. MSC-Exo-sourced angiostimulatory factors (PD-ECGF, IL-6, Ang1, HGF, SDF-1) activate JAK/STAT, PI3K, p38/MAPK signaling pathways and enhance DNA synthesis in tumor ECs, increasing their survival, viability, and proliferation. Additionally, MSC-derived miR-424, miR-30b, and miR-30c induce increased VEGF synthesis in tumor cells, enhancing neovascularization in the tumor microenvironment. On the contrary, MSC-Exo-sourced miR-16, miR-100-5p, and miR-1246 may inhibit tumor neoangiogenesis by suppressing the VEGF-driven generation of the capillary network in the tumor microenvironment. Ang1, angiopoietin-1; HGF, hepatocyte growth factor; IL-6, interleukin 6; MAPK, mitogen-activated protein kinase; MSC, mesenchymal stem cell; PD-ECGF, platelet-derived endothelial cell growth factor; SDF-1, stromal-derived factor 1; JAK, Janus kinase; PI3K, phosphoinositide 3-kinase; STAT, signal transducer and activator of transcription.
Ang1 is a very potent anti-apoptotic factor that promotes the survival of ECs (Papapetropoulos et al., 2000). Ang1 activates tyrosine kinase with immunoglobulin-like loops and EGF homology domains-2 receptor, induces phosphorylation of the serine-threonine kinase AKT (PKB) that up-regulates activity of survivin (Papapetropoulos et al., 2000). Survivin acts as an inhibitor of apoptosis and protects ECs from death-inducing stimuli (Papapetropoulos et al., 2000).
MSC-Exos contain HGF and stromal cell-derived factor 1 (SDF-1), which improve migratory properties of ECs and endothelial progenitor cells (EPCs), enabling enhanced repair of irradiation-injured blood vessels in the tumor microenvironment (Baruah and Wary, 2020). MSC-Exo-derived HGF exerts its pro-angiogenic activity through tyrosine phosphorylation of its specific receptor, c-Met, which is expressed on ECs of newly formed blood vessels (Baruah and Wary, 2020). Once HGF activates c-Met, several kinases (ERK, JNK, phosphoinositide 3-kinase (PI3K), and p38/MAPK) become phosphorylated in ECs, resulting in their enhanced proliferation and migration, which finally leads to the restoration of injured blood vessels (You and McDonald, 2008). Similarly, SDF-1 enhances neo-angiogenesis in irradiated tumor tissues (Petit et al., 2007). By binding to C-X-C chemokine receptor type 4 (CXCR-4) on EPCs, MSC-Exo-sourced SDF-1 enhances migration and recruitment of EPCs to the sites of injury, crucially contributing to the restoration of blood vessels integrity (Baruah and Wary, 2020).
MSC-Exos contain pro-angiogenic miRNAs, which regulate MSC: EC cross-talk in the tumor microenvironment (Gong et al., 2017). Among these, MSC-derived miR-424, miR-30b, and miR-30c manage to most efficiently increase tube-like structure formation and induce the generation of new blood vessels in the tumor vasculature (Gong et al., 2017). MSC-Exo-sourced miR-424 induces increased expression of VEGF in tumor cells (Li et al., 2020). VEGF binds to VEGF receptor type 2 and activates phosphoinositide phospholipase Cγ and PI3K in tumor ECs (Li et al., 2020). Phosphoinositide phospholipase Cγ and PI3K modulate the activity of mammalian target of rapamycin (mTOR) and activate protein kinase C and ERK1/2, which suppress caspase-dependent apoptosis and promote cyclin D1 activity in nuclear factor-κB (NF-κB)-dependent manner, enabling enhanced survival of ECs in the tumor microenvironment (Li et al., 2020). MSC-Exo-sourced miR30b and miR30c also regulate VEGF synthesis in tumor cells by controlling delta-like 4 (DLL4) gene expression (Bridge et al., 2012). DLL4 is over-expressed in human cancers, where it regulates the expression of VEGFR and indirectly controls VEGF-dependent sprouting of newly generated blood vessels (Bridge et al., 2012).
In contrast to these findings, experimental studies demonstrated that MSC-Exos significantly attenuates tumor growth and progression by suppressing VEGF-driven generation of capillary network in the tumor microenvironment (Lee et al., 2013; Rosenberger et al., 2019, Liu et al., 2022). Lee et al. (2013) found that MSC-Exo-derived miR-16 suppressed VEGF gene expression in murine mammary carcinoma 4T1 cells and attenuated VEGF-dependent neovascularization in breast cancer. MSC-Exos did not directly affect the viability and proliferation of 4T1 cells but remarkably inhibited their capacity to produce VEGF. The concentration of miR-16 in MSC-Exo-treated 4T1 cells correlated inversely with the levels of VEGF expression (Lee et al., 2013). Additionally, the decreased mRNA level of VEGF in MSC-Exo-treated 4T1 cells was completely restored when miR-16 inhibitor was added in MSC-Exos, suggesting the primary role of MSC-Exo-sourced miR-16 in the down-regulated VEGF expression in MSC-Exo-treated murine breast cancer cells (Lee et al., 2013). MSC-Exo-dependent suppression of VEGF synthesis in 4T1 cells resulted in the significantly lower proliferation and migration of ECs, which were co-cultured with tumor cells (Lee et al., 2013). Consistent with these in vitro results were findings obtained in vivo that showed significantly decreased VEGF mRNA levels in breast cancers of MSC-Exo-treated tumor-bearing mice (Lee et al., 2013). Immunohistochemical analysis of tumor tissues revealed weak expression of VEGF and CD31, confirming that MSC-Exos greatly inhibited the angiogenesis in the tumor environment (Lee et al., 2013). Importantly, MSC-Exos-based treatment significantly reduced the size and weight of breast cancers in MSC-Exo-treated tumor-bearing animals, suggesting that MSC-Exo-dependent suppression of neo-angiogenesis managed to attenuate the growth and progression of murine breast cancers (Lee et al., 2013). Like these findings, Rosenberger et al. (2019) also demonstrated that MSC-Exo-based suppression of VEGF-driven neoangiogenesis inhibited the growth of oral squamous cell (OSS) carcinoma in experimental hamsters. MSC-Exos, which were directly delivered in the OSS cancers, inhibited VEGF synthesis in tumor ECs and reduced blood vessel density in the tumor microenvironment (Rosenberger et al., 2019). Besides their suppressive effects on VEGF expression, MSC-Exos could also induce apoptosis of ECs in OSS carcinomas (Rosenberger et al., 2019). In line with these findings, Liu et al. (2022) recently demonstrated that MSC-Exo-sourced miR-100-5p and miR-1246 were mainly responsible for anti-angiogenic, pro-apoptotic, and tumor-suppressive effects of MSC-Exos in the attenuation of OSS carcinomas. By delivering miR-100-5p and miR-1246 in tumor ECs, MSC-Exos down-regulated the expression of VEGF and Ang1 and significantly reduced vascular density in OSS carcinomas. Accordingly, MSC-Exo-dependent suppression of VEGF and Ang1-driven angiogenesis inhibited the proliferation of OSS cells and reduced the growth and progression of OSS carcinomas in experimental mice (Liu et al., 2022).
MSC-Exos as pro- and anti-tumor immunity
MSC-Exos contain all MSC-sourced immunomodulatory factors and, therefore, possess similar immunoregulatory properties as their parental cells (Harrell et al., 2020). MSC-Exos deliver immunosuppressive and immunostimulatory factors in tumor-infiltrated T lymphocytes, DCs, neutrophils, tumor-associated macrophages (TAMs), NK cells and, by modifying their phenotype and function, regulate anti-tumor immunity and tumor progression (Fig. 3) (Harrell et al., 2021).
Figure 3: MSC-Exo-based regulation of anti-tumor immunity. MSC-Exos deliver immunosuppressive and immunostimulatory factors in tumor-infiltrated immune cells, modulating the anti-tumor immune response. MSC-sourced IL-10, IL-1Ra, IDO, and miR-21-5p induce the generation of tolerogenic and immuno-suppressive phenotype in tumor-infiltrated DCs and macrophages, inhibit IL-1β-driven recruitment of leukocytes, promote generation and expansion of immunosuppressive T regulatory cells which results in the suppression of anti-tumor immunity. On the contrary, MSC-Exos may deliver immunoregulatory miR-182 in tumor-infiltrated CD8+ CTLs and NKT cells to increase their proliferation and cytotoxicity, which results in the attenuation of tumor growth and progression. CTL, cytotoxic T lymphocyte; DC, dendritic cells; MSC, mesenchymal stem cell; IL-10, interleukin 10; IDO, indoleamine 2, 3 dioxygenase; NKT, natural killer T.
MSC-Exo-sourced IL-10 inhibits activation and anti-tumor properties of DCs, T lymphocytes, and TAMs (Volarevic et al., 2017) and induces the generation of tolerogenic, immuno-suppressive phenotype in tumor-infiltrated DCs. These tolerogenic DCs have an immature phenotype, do not optimally produce pro-Th1 and pro-Th17 inflammatory cytokines (tumor necrosis factor-alpha (TNF-α), IL-12, IL-1β, and IL-23), and therefore, are not capable of inducing activation of naïve CD4+ and CD8+ T cells and are incapable of generating Th1 and Th17 cell-driven anti-tumor immune response (Volarevic et al., 2017; Harrell et al., 2021). Additionally, MSC-Exos, in an IL-10-dependent manner, induces alternative activation of TAMs (Biswas et al., 2019). In alternatively activated TAMs, MSC-Exo-derived IL-10 inhibits the synthesis of inflammatory cytokines (IL-1β and TNF-α) and prevents IL-1β and TNF-α-driven recruitment of circulating leukocytes in tumor tissue (Harrell et al., 2021). IL-1β and TNF-α bind to their receptors (IL-1R and TNFRs) on ECs of the tumor vasculature and activate myeloid differentiation primary response 88/MAPK-dependent intracellular cascade, resulting in the activation of several transcriptional factors (NF-κB, activator protein 1) that increase expression of genes responsible for the production of E and P selectins and integrin ligands. Accordingly, by producing IL-1β and TNF-α, TAMs enhance the expression of adhesion molecules (selectins and integrin ligands) on tumor ECs, enabling a massive influx of circulating immune cells in the tumor microenvironment and eliciting a potent anti-tumor immune response (Biswas et al., 2019; Harrell et al., 2021). MSC-Exos contain high levels of MSC-derived IL-1Ra and sTNFRs, which bind to IL-1R and TNF-α and prevent IL-1β and TNF-α-dependent recruitment of leukocytes in tumor tissue. Accordingly, the concentration of IL-1β and TNF-α were significantly lower in the serum samples of MSC-Exo-treated tumor-bearing animals, which was accompanied with reduce the presence of activated immune cells in the tumor tissues (Harrell et al., 2021).
In a similar manner as IL-10, MSC-Exo-derived miR-21-5p induces alternative activation of TAMs, suppresses IL-1β and TNF-α-driven inflammatory response, and inhibits anti-tumor immunity (Ren et al., 2019). Ren et al. (2019) showed that MSC-Exo-derived miR-21-5p down-regulated expression of phosphatase and tensin homolog (PTEN) gene, which suppresses synthesis of arginase I in TAMs and inhibit their alternative activation (Ren et al., 2019; Sahin et al., 2014). Additionally, in lung cancer A549 cells, MSC-Exo-derived miR-21-5p attenuated the expression of PTEN and programmed cell death 4 genes which induces apoptosis and suppresses the proliferation of malignant cells (Ren et al., 2019). Therefore, by delivering miR-21-5p in TAMs and A549 cells, MSC-Exos suppressed macrophage-driven anti-tumor immunity, prevented apoptosis, increased proliferation of A549 cells, and enhanced lung cancer growth and progression in MSC-Exo-treated tumor-bearing animals (Ren et al., 2019).
MSC-Exos are enriched with MSC-sourced TGF-β and prostaglandin E2 (PGE2) that prevent the expansion of activated T cells in the tumor microenvironment (Harrell et al., 2021). TGF-β inhibits the activation of JAK-STAT signaling pathway, induces the G1 cell cycle arrest, and attenuates the expansion of activated cytotoxic CD8+ T lymphocytes (CTLs) and NK cells (Volarevic et al., 2017). Therefore, MSC-Exos, in a TGF-β-dependent manner, suppress the cytotoxicity of CTLs and NK cells and attenuate CTL and NK cell-dependent elimination of malignant cells, enabling enhanced tumor growth and progression (Harrell et al., 2021). Like TGF-β, MSC-sourced PGE2 suppresses the proliferation of activated CTLs by repressing the synthesis of IL-2, which regulates the expansion of activated CD8+ T cells (Volarevic et al., 2017). Additionally, MSC-derived PGE2 is responsible for attenuated antigen-presenting properties of tumor-infiltrated DCs and TAMs since PGE2 down-regulates expression of co-stimulatory molecules (CD80, CD86, and CD40) and major histocompatibility complex class II proteins on these professional antigen-presenting cells (Zhang et al., 2022).
Inflammatory cytokines (particularly interferon gamma and TNF-α), mainly produced by tumor-infiltrated T lymphocytes and inflammatory TAMs, and induce the expression of IDO in tumor-infiltrated MSCs (Miloradovic et al., 2020; Harrell et al., 2021). IDO is an enzyme that converts tryptophan to immunosuppressive kynurenine (KYN) that induces expansion of immunosuppressive T regulatory cells (Tregs) and inhibits generation of inflammatory CD4+ and CD8+Th17 lymphocytes in the tumor microenvironment (Volarevic et al., 2017). KYN prevents transdifferentiation of Tregs in tumorotoxic Th17 cells by activating general control nonderepressible 2 kinase, which inhibits mTOR signaling and prevents expression of Th17-specific retinoic-acid-receptor-related orphan nuclear receptor gamma transcriptional factor in activated Tregs (Volarevic et al., 2017). Additionally, through the immunosuppressive effects of KYN, MSCs-Exos attenuates the cytotoxicity of NK and NKT cells (Harrell et al., 2021; Zhang et al., 2022).
Although a large number of experimental studies demonstrated MSC-Exos-dependent suppression of anti-tumor immunity (Ren et al., 2019; Sahin et al., 2014; Harrell et al., 2021; Zhang et al., 2022), two research groups provided evidence about immunostimulatory effects of MSC-Exos on tumor-infiltrated immune cells (Zhou et al., 2013; Li et al., 2021). By using an orthotopic clear cell renal cell carcinoma mice model, Li et al. (2021) recently showed that MSC-Exos delivered immunoregulatory miR-182 in tumor-infiltrated CD8+ CTLs and NKT cells and increased their proliferation and cytotoxicity. A significantly higher number of activated DCs, CTLs, and NKT cells were observed in the tumors of MSC-Exo-treated mice and were followed by reduced growth and progression of murine renal cancer (Li et al., 2021). Likewise, Zhou et al. (2013) showed that MSC-Exos, in a CCL2-dependent manner, promoted the recruitment of circulating CD4+ and CD8+ T cells in tumor tissue and enhanced T cell-driven anti-tumor immune response.
Molecular mechanisms responsible for MSC-Exos-based enhancement of cancer therapy resistance
The resistance of tumor cells to therapeutic agents impairs the therapeutic efficacy of anti-cancer drugs and negatively affects the survival of cancer patients (Zhang et al., 2022). Therefore, the delineation of molecular mechanisms which are responsible for cancer therapy resistance has been the focus of many research groups. Results of a large number of studies demonstrated that MSC-Exos enhanced the resistance of malignant cells to chemotherapy (Ono et al., 2014; Ji et al., 2015; Xu et al., 2019; Luo et al., 2020; Lyu et al., 2021).
Ji et al. (2015) showed that MSC-Exos prevented 5-fluorouracil (5-FU)-induced apoptosis of malignant cells and significantly enhanced the resistance of gastric cancer cells to 5-FU. MSC-Exos activated calcium/calmodulin-dependent protein kinases and rapidly accelerated fibrosarcoma/ERK signaling pathway in gastric cancer cells that increased expression of multi-drug resistance-associated genes and decreased sensitivity of tumor cells to chemotherapy (Ji et al., 2015).
The dormancy of malignant cells is critically involved in chemoresistance, metastasis, and recurrence of breast cancer (Andreucci et al., 2022). MSC-Exos regulate the cross-talk between MSCs and dormant breast cancer cells and enhance their resistance to chemotherapeutic drugs (Zhang et al., 2022). Ono et al. (2014) revealed that MSC-Exos contain miR-23b, which induces dormancy of BM2 breast cancer cells and promotes their resistance to docetaxel. MSC-Exos-derived miR-23b suppressed the expression of the myristoylated alanine-rich C-kinase substrate gene, which encoded a protein that facilitated cell cycling and motility of BM2 cells (Ono et al., 2014). In line with these findings, Luo et al. (2020) showed that MSC-Exo-sourced miR-21-5p induced increased expression of chemoresistant S100 calcium-binding protein A6 (S100A6) gene in MDA-MB-231 breast cancer cells and promoted their resistance to doxorubicin (DOX) in vitro and in vivo. By inducing G0 cell cycle arrest and dormancy of MDA-MB-231 and T47D breast cancer cells, MSC-Exo-derived miR-222/223 increased their resistance to carboplatin (Luo et al., 2020). Bliss and co-workers designed a new antagomiR-222/223-based therapeutic strategy to target dormant breast cancer cells (Bliss et al., 2016). Intravenously infused MSC loaded with antagomiR-222/223 sensitized dormant breast cancer cells to carboplatin-based therapy and significantly increased survival of breast cancer-bearing animals, indicating the potential therapeutic potential of MSC-Exos in the elimination of dormant tumor cells (Bliss et al., 2016).
MSC-Exos increase the survival and stemness of cancer stem cells (CSCs) and enhance their resistance to anti-cancer drugs (Lyu et al., 2021). MSC-Exos enhanced expression of octamer-binding transcription factor 3/4, Nanog homeobox, and SRY-box transcription factor 2 genes, which regulate viability and pluripotency of CSCs and promote the expression of chemoresistant S100A4 gene, which increase their resistance to chemotherapeutic drugs (Lyu et al., 2021).
Using a xenograft tumor model of chronic myeloid leukemia (CML), Zhang et al. (2020a) demonstrated that MSC-Exos promotes proliferation and decreased sensitivity of CML cells to tyrosine kinase inhibitors (TKIs). MSC-Exos-sourced miR-15a induced G0 cell cycle arrest, increased synthesis of anti-apoptotic B-cell lymphoma 2 (Bcl-2) protein, and suppressed caspase 3-driven apoptosis of CML cells, which attenuated efficacy of TKIs and increased leukemia progression (Zhang et al., 2020a).
As recently evidenced by Xu et al. (2019), the therapeutic efficacy of proteasome inhibitor bortezomib in the treatment of multiple myeloma (MM) was significantly impaired by MSC-Exos, which delivered proteasome subunit alpha type-3 (PSMA3) and long non-coding (lnc) PSMA3-AS1 into MM cells. MSC-Exo-sourced Lnc PSMA3-AS1 formed an RNA duplex with PSMA3-AS1 pre-mRNA and induced enhanced expression of PSMA3 in MM cells (Xu et al., 2019). In vivo studies showed that the up-regulated activity of PSMA3 significantly impaired the sensitivity of MSC-Exo-primed MM cells to bortezomib, confirming that MSC-Exos, in a PSMA3-dependent manner, promoted resistance to proteasome inhibitor in MM cells (Xu et al., 2019).
Therapeutic potential of MSC-Exos in cancer treatment
During the last decade, a large number of experimental studies provided evidence about the therapeutic efficacy of MSC-Exos in cancer treatment (Table 1) (Li et al., 2018; Melzer et al., 2019; Wei et al., 2019; Bagheri et al., 2020; Qiu et al., 2020; Zhang et al., 2022). MSC-Exos inhibit the proliferation of tumor cells and the generation of new blood vessels and enhance tumorotoxicty of tumor-infiltrated immune cells. MSC-Exo-sourced miR-100, miR-222-3p, miR-302a, and miR-338-5p inhibit mTOR, IRF2/INPP4B, and Wif1/Wnt8/β-catenin signaling pathways in malignant cells, suppressing their viability, invasiveness, and metastatic potential (Li et al., 2018; Melzer et al., 2019; Wei et al., 2019; Bagheri et al., 2020; Qiu et al., 2020; Zhang et al., 2022). MSC-Exo-sourced miR-16, miR-100-5p, and miR-1246 inhibit the generation of capillary network in the tumor microenvironment by suppressing VEGF synthesis in ECs while MSC-Exo-derived miR-182 attenuates tumor growth and progression by increasing the cytotoxicity of tumor-infiltrated CD8+ CTLs and NKT cells (Li et al., 2018; Melzer et al., 2019; Wei et al., 2019; Bagheri et al., 2020; Qiu et al., 2020; Zhang et al., 2022).
Additionally, tumor-suppressive effects of MSC-Exos were also based on their ability to increase the sensitivity of malignant cells toward standard chemotherapeutics and on their potential to deliver growth factors into the irradiated parenchymal, non-malignant cells, providing support for enhanced recovery of healthy tissue from radiotherapy-induced damage (Zhang et al., 2022).
MSC-Exos, due to its nano-sized dimension and lipid envelope, avoid all biological barriers and successfully deliver anti-cancer drugs directly into tumors cells, enhancing their selectivity and therapeutic effects (Melzer et al., 2019; Wei et al., 2019; Bagheri et al., 2020; Qiu et al., 2020). MSC-Exo-carrying DOX (MSC-ExosDOX) suppressed osteosarcoma and colorectal cancer cell growth in vitro with higher effectiveness and lower cytotoxicity than free DOX (Wei et al., 2019; Bagheri et al., 2020). Studies on tumor-bearing animals showed a high accumulation of MSC-ExosDOX at the tumor site, where tumor growth was attenuated with higher efficiency and faster liver clearance than free DOX (Bagheri et al., 2020). Like these findings are results obtained by Kanchanapally et al. (2020), who encapsulated the anti-cancer agent honokiol within MSC-Exos and increased its cytotoxicity against MiaPaCa and Colo357 pancreatic cancer cells. MSC-Exo-carrying honokiol significantly improved the uptake of honokiol by tumor cells and more efficiently inhibited CDK-2 and CDK4-dependent cell cycle arrest and apoptosis of pancreatic cancer than free honokiol (Kanchanapally et al., 2020). Qiu et al. (2020) demonstrated the efficacy of cabazitaxel (CTX)-loaded and tumor necrosis factor-related apoptosis-inducing ligand (TRAIL)-overexpressing MSC-Exos (MSC-ExosCTX+TRAIL) against drug-resistant SCC25 oral squamous cell carcinoma cells in vitro and in vivo. Tumor tropism of MSC-Exos enhanced CTX selectivity of SCC25 cells, and MSC-Exo-expressing TRAIL increased CTX capacity for inhibition of PI3K/AKT/mTOR signaling pathway in malignant cells (Qiu et al., 2020). Accordingly, MSC-ExosCTX+TRAIL induced the apoptosis of SCC25 CTX-resistant tumor cells in a dose-dependent manner and significantly reduced the growth and progression of CTX-resistant oral carcinoma in tumor-bearing mice (Qiu et al., 2020). Likewise, Melzer et al. (2019) demonstrated the therapeutic potential of paclitaxel (PTX)-loaded MSC-Exos (MSC-ExosPTX) against highly metastatic MDA-hyb1 breast cancer tumors. Intravenously injected MSC-ExosPTX suppressed primary breast cancer growth by 60% and reduced metastasis in lungs, liver, spleen, and kidneys of tumor-bearing non-obese-diabetic severe combined immunodeficiency mice by 50%. Importantly. MSC-ExosPTX selectively delivered PTX in tumor cells and, accordingly, MSC-ExosPTX-treated breast cancer-bearing mice received a 1000-fold reduced PTX amount than free PTX-treated tumor-bearing animals (Melzer et al., 2019).
MSC-Exos were used for the delivery of small interfering RNA (siRNA) in tumor cells, where they suppressed the synthesis of drug sensitivity-conferring proteins. Grp78 is a protein that induces resistance of hepatocellular carcinoma cells (HCCs) to sorafenib (Li et al., 2018). By delivering GRP78-siRNA-containing MSC-Exos in HCCs, Li et al. (2018) sensitized HCCs to sorafenib and significantly attenuated their viability, proliferative and invasive properties.
MSC-Exos have also been used as cargo carriers of miRNAs for the induction of miRNAs-dependent abrogation of chemoresistance in tumor cells (Munoz et al., 2013; Lou et al., 2015; Yu et al., 2019). Since miR-122 and miR-199a could increase the sensitivity of HCCs to chemotherapeutic agents, Lou et al. (2015) designed miR-122 and miR-199a-overexpressing MSCs, which delivered miR-122 and miR-199a-containing Exos (MSC-ExosmiR-122 and MSC-ExosmiR-199a) directly in HCCs. MSC-ExosmiR-122 significantly increased the sensitivity of HCCs to 5-FU and sorafenib by negatively regulating the expression of miR-122-target genes (cyclin G1, a disintegrin and metalloprotease 10, and insulin-like growth factor receptor 1) in HCCs. MSC-ExosmiR-122 enhanced 5-FU and sorafenib-induced apoptosis of HCCs and reduced tumor growth and progression (Lou et al., 2015). Single intra-tumor injection of MSC-ExosmiR-122 (administered one week after subcutaneous inoculation of HCCs) significantly up-regulated the expression of apoptosis-related genes (caspase 3 and Bcl-2 associated X protein) and reduced the volume and weight of HCC in experimental mice (Lou et al., 2015). MSC-ExosmiR-199a sensitized HCC cells to DOX by inhibiting the mTOR pathway in a miR-199a-dependent manner. MSC-Exos-sourced miR-199a reduced phosphorylation of eukaryotic translation initiation factor 4E and phosphoprotein 70 ribosomal protein S6 kinase and decreased mTOR activity in DOX-treated HCCs (Lou et al., 2015). Intravenously injected MSC-ExosmiR-199a suppressed mTOR activity and increased the sensitivity of HCCs to DOX, which significantly reduced the growth and progression of HCC in DOX-treated tumor-bearing mice (Lou et al., 2015).
In line with these findings are results obtained by Yu and co-workers, who showed that miR-199a-overexpressing MSC-Exos suppressed glioma progression by enhancing the sensitivity of tumor cells to temozolomide (TMZ) (Yu et al., 2019). MSC-Exo-sourced miR-199a inhibited synthesis of AGAP2 protein by down-regulating the expression of the Arf GTPase-activating protein-2 (AGAP2) gene in glioma cells and prevented AGAP2-dependent elimination of TMZ from glioma cells and increased its toxicity (Yu et al., 2019). Enhanced expression of miR-199a increased apoptosis and inhibited invasive characteristics of TMZ-treated glioma cells. Accordingly, MSC-Exos-based treatment significantly reduced glioma growth and progression in TMZ-treated mice, confirming the therapeutic potential of MSC-ExosmiR-199a in cancer therapy (Yu et al., 2019). Beneficial effects of MSC-Exos in glioblastoma treatment were evidenced by Munoz et al. (2013) and Sharif et al. (2018), who demonstrated that MSC-Exo-sourced anti-miR-9 and miR-12d increased chemosensitivity of glioblastoma multiforme (GBM) cells. Munoz et al. (2013) showed that MSC-Exo-derived anti-miR-9 suppressed the expression of the drug efflux transporter, P-glycoprotein in GBM cells, weakened the TMZ resistance, and enhanced TMZ-driven, caspase-dependent apoptosis of GBM cells. Sharif and co-workers demonstrated that miR-124-overexpressing MSC-Exos increased the chemosensitivity of GBM cells and suppressed their proliferative, migratory, and invasive properties by attenuating expression of CDK-6 gene, which regulated cell cycle progression, viability, and senescence of GBM cells (Sharif et al., 2018).
Considering that miR-193a inhibits non-small cell lung cancer (NSCLC) proliferation (Zhang et al., 2022), Wu et al. (2020) designed miR-193a-overexpressing MSCs that produce miR-193-enriched MSC-Exos (MSC-ExosmiR-193-a). By down-regulating expression of leucine-rich repeat-containing 1 gene in NSCLC cells, MSC-ExosmiR-193-a attenuated cisplatin (DDP) resistance of NSCLC cells and inhibited their proliferative, migratory, and invasive characteristics (Wu et al., 2020). Accordingly, results from a murine model of lung cancer showed better efficiency of combined DDP+MSC-Exos therapy in the suppression of lung cancer growth and progression than DDP-single treatment, confirming the potential therapeutic use of MSC-ExosmiR-193-a in NSCLC therapy (Wu et al., 2020).
MSC-Exos could repair radiation-induced tissue damage by delivering growth factors and miRNAs which up-regulate the expression of genes that prevent apoptosis, improve viability, and enhance the proliferation of tumor-neighboring, healthy parenchymal cells (Wen et al., 2016; Kink et al., 2019; Zuo et al., 2019). Wen et al. (2016) showed that MSC-Exos induces the proliferation of hematopoietic progenitor cells (HPCs) in the bone marrows and re-populates leukocytes in the peripheral blood of irradiated tumor-bearing mice. Intravenously injected MSC-Exos accumulate mainly in the bone marrow of experimental animals where, by delivering cell cycle-regulating miR-221, miR-451, miR-654-3p, and apoptosis-regulated miR210-5p, miR-106b-3p, miR-155-5p, reversed radiation-induced DNA damage, prevented apoptosis, and increased proliferation of HPCs (Wen et al., 2016). Similarly, Kink et al. (2019) showed that MSC-Exos restored hematopoiesis in irradiated tumor-bearing mice by delivering MSC-sourced hematopoiesis-related cytokines (IL-6, IL-8, granulocyte colony-stimulating factor, monocyte chemoattractant protein-1) in HPCs. Zuo et al. (2019) provided evidence that MSC-Exos alleviated radiation-induced bone loss by activating the Wnt/β-catenin signaling in bone marrow MSCs, which enhanced their capacity for osteogenic differentiation. Li et al. (2022) demonstrated that MSC-Exo-sourced miR-466f-3p inhibited AKT/glycogen synthase kinase-3 beta pathway in alveolar epithelial cells and prevented irradiation-induced EMT in experimental animals, indicating anti-fibrotic potential and possible therapeutic use of MSC-Exos in the treatment of radiation-induced lung fibrosis.
Conclusions and Future Perspectives
Similar to their parental cells, MSC-Exos possess a dual role that can promote or suppress tumor growth and could act as friends or foes of tumor cells (Harrell et al., 2021). MSC-Exos are enriched with a large number of pro- and anti-tumorigenic factors, and their administration may have opposite effects on cancer progression and treatment (Zhang et al., 2022; Harrell et al., 2021). MSC-Exos contain angiostimulatory and immunosuppressive proteins derived from MSCs that enhance the generation of new blood vessels and attenuate immune response in the tumor microenvironment, enabling rapid growth and progression of malignant cells (Harrell et al., 2020). However, due to their low toxicity, biodegradability, and capacity to avoid biological barriers, MSC-Exos have emerged as promising therapeutic carriers of anti-cancer drugs (Zhang et al., 2022). The miRNAs and siRNAs sourced from MSC-Exo modulate the expression of chemoresistance-related genes in tumor cells, enhancing their sensitivity to chemotherapeutics (Ghollasi et al., 2021). Additionally, MSC-Exos may deliver immunostimulatory proteins to tumor-infiltrated immune cells to enhance their anti-tumor properties (Harrell et al., 2021). Finally, MSC-Exos contain various growth factors that support the regeneration of healthy tissues damaged by radiotherapy, making them potential novel therapeutic agents in regenerative oncology (Zhang et al., 2022).
However, the fact that MSC-Exos may act as an important source of angiostimulatory, immunosuppressive, and pro-tumorigenic factors raises serious concerns regarding their clinical use in cancer treatment (Harrell et al., 2020). To address this safety issue, future studies should delineate the exact molecular mechanisms that modulate the phenotype of tumor-associated MSCs and, consequently, affect the composition of MSC-Exos. Understanding the signaling pathways that alter the secretory profile of tumor-infiltrated MSCs will enable researchers to bioengineer MSCs with “strict anti-tumorigenic phenotype” producing MSC-Exos enriched with tumorotoxic miRNAs and immunostimulatory proteins. Only these MSC-Exos could be considered vehicles for cell-specific delivery of anti-cancer drugs and could be used as new remedies in cancer treatment.
Funding Statement: This work was supported by the European Crohn’s and Colitis Organization (ECCO) (Grant “The Role of Galectin 3 in Acute Colitis”); Serbian Ministry of Science (Grant Nos. ON175069 and ON175103), Faculty of Medical Sciences University of Kragujevac (Grant No. MP01/18).
Author Contributions: The authors confirm their contribution to the paper as follows: study conception and design: CRH, VV; data collection: CRH, VD, AV, DP, VV; figure design: AV, DP; draft manuscript preparation: CRH, VD, VV. All authors approved the final version of the manuscript.
Availability of Data and Materials: The findings presented in this review article are contained in the cited articles.
Ethics Approval: Not Applicable.
Conflicts of Interest: The authors declare that they have no conflicts of interest to report regarding the present study.
References
Andreucci E, Peppicelli S, Ruzzolini J, Bianchini F, Calorini L (2022). Physicochemical aspects of the tumour microenvironment as drivers of vasculogenic mimicry. Cancer and Metastasis Reviews 41: 935–951. [Google Scholar] [PubMed]
Bagheri E, Abnous K, Farzad SA, Taghdisi SM, Ramezani M, Alibolandi M (2020). Targeted doxorubicin-loaded mesenchymal stem cells-derived exosomes as a versatile platform for fighting against colorectal cancer. Life Sciences 261: 118369. [Google Scholar] [PubMed]
Baruah J, Wary KK (2020). Exosomes in the regulation of vascular endothelial cell regeneration. Frontiers in Cell and Developmental Biology 7: 353. [Google Scholar] [PubMed]
Biswas S, Mandal G, Roy Chowdhury S, Purohit S, Payne KK et al. (2019). Exosomes produced by mesenchymal stem cells drive differentiation of myeloid cells into immunosuppressive M2-polarized macrophages in breast cancer. The Journal of Immunology 203: 3447–3460. [Google Scholar] [PubMed]
Bliss SA, Sinha G, Sandiford OA, Williams LM, Engelberth DJ et al. (2016). Mesenchymal stem cell-derived exosomes stimulate cycling quiescence and early breast cancer dormancy in bone marrow. Cancer Research 76: 5832–5844. [Google Scholar] [PubMed]
Bridge G, Monteiro R, Henderson S, Emuss V, Lagos D, Georgopoulou D, Patient R, Boshoff C (2012). The microRNA-30 family targets DLL4 to modulate endothelial cell behavior during angiogenesis. Blood 120: 5063–5072. [Google Scholar] [PubMed]
de Luca A, Lamura L, Gallo M, Maffia V, Normanno N (2012). Mesenchymal stem cell-derived interleukin-6 and vascular endothelial growth factor promote breast cancer cell migration. Journal of Cellular Biochemistry 113: 3363–3370. [Google Scholar] [PubMed]
Du T, Ju G, Wu S, Cheng Z, Cheng J, Zou X, Zhang G, Miao S, Liu G, Zhu Y (2014). Microvesicles derived from human Wharton’s jelly mesenchymal stem cells promote human renal cancer cell growth and aggressiveness through induction of hepatocyte growth factor. PLoS One 9: e96836. [Google Scholar] [PubMed]
Ghollasi M, Ghasembaglou S, Rahban D, Korani M, Motallebnezhad M, Asadi M, Zarredar H, Salimi A (2021). Prospects for manipulation of mesenchymal stem cells in tumor therapy: Anti-angiogenesis property on the spotlight. International Journal of Stem Cells 14: 351–365. [Google Scholar] [PubMed]
Gong M, Yu B, Wang J, Wang Y, Liu M, Paul C, Millard RW, Xiao DS, Ashraf M, Xu M (2017). Mesenchymal stem cells release exosomes that transfer miRNAs to endothelial cells and promote angiogenesis. Oncotarget 8: 45200–45212. [Google Scholar] [PubMed]
Gu H, Ji R, Zhang X, Wang M, Zhu W, Qian H, Chen Y, Jiang P, Xu W (2016). Exosomes derived from human mesenchymal stem cells promote gastric cancer cell growth and migration via the activation of the Akt pathway. Molecular Medicine Reports 14: 3452–3458. [Google Scholar] [PubMed]
Harrell CR, Jovicic N, Djonov V, Volarevic V (2020). Therapeutic use of mesenchymal stem cell-derived exosomes: From basic science to clinics. Pharmaceutics 12: 474. [Google Scholar] [PubMed]
Harrell CR, Volarevic A, Djonov VG, Jovicic N, Volarevic V (2021). Mesenchymal stem cell: A friend or foe in anti-tumor immunity. International Journal of Molecular Sciences 22: 12429. [Google Scholar] [PubMed]
Hessvik NP, Llorente A (2018). Current knowledge on exosome biogenesis and release. Cellular and Molecular Life Sciences 75: 193–208. [Google Scholar] [PubMed]
Ji R, Zhang B, Zhang X, Xue J, Yuan X, Yan Y, Wang M, Zhu W, Qian H, Xu W (2015). Exosomes derived from human mesenchymal stem cells confer drug resistance in gastric cancer. Cell Cycle 14: 2473–2483. [Google Scholar] [PubMed]
Kanchanapally R, Khan MA, Deshmukh SK, Srivastava SK, Khushman M, Singh S, Singh AP (2020). Exosomal formulation escalates cellular uptake of honokiol leading to the enhancement of its antitumor efficacy. ACS Omega 5: 23299–23307. [Google Scholar] [PubMed]
Kink JA, Forsberg MH, Reshetylo S, Besharat S, Childs CJ et al. (2019). Macrophages educated with exosomes from primed mesenchymal stem cells treat acute radiation syndrome by promoting hematopoietic recovery. Biology of Blood and Marrow Transplantation 25: 2124–2133. [Google Scholar] [PubMed]
Lee JK, Park SR, Jung BK, Jeon YK, Lee YS, Kim MK, Kim YG, Jang JY, Kim CW (2013). Exosomes derived from mesenchymal stem cells suppress angiogenesis by down-regulating VEGF expression in breast cancer cells. PLoS One 8: e84256. [Google Scholar] [PubMed]
Li D, Lin F, Li G, Zeng F (2021). Exosomes derived from mesenchymal stem cells curbs the progression of clear cell renal cell carcinoma through T-cell immune response. Cytotechnology 73: 593–604. [Google Scholar] [PubMed]
Li X, Liu LL, Yao JL, Wang K, Ai H (2019). Human umbilical cord mesenchymal stem cell-derived extracellular vesicles inhibit endometrial cancer cell proliferation and migration through delivery of exogenous miR-302a. Stem Cells International 2019: 8108576. [Google Scholar] [PubMed]
Li Y, Shen Z, Jiang X, Wang Y, Yang Z, Mao Y, Wu Z, Li G, Chen H (2022). Mouse mesenchymal stem cell-derived exosomal miR-466f-3p reverses EMT process through inhibiting AKT/GSK3β pathway via c-MET in radiation-induced lung injury. Journal of Experimental and Clinical Cancer Research 41: 128. [Google Scholar] [PubMed]
Li S, Wu Y, Zhang J, Sun H, Wang X (2020). Role of miRNA-424 in cancers. OncoTargets and Therapy 13: 9611–9622. [Google Scholar] [PubMed]
Li H, Yang C, Shi Y, Zhao L (2018). Exosomes derived from siRNA against GRP78 modified bone-marrow-derived mesenchymal stem cells suppress Sorafenib resistance in hepatocellular carcinoma. Journal of Nanobiotechnology 16: 103. [Google Scholar] [PubMed]
Liang W, Chen X, Zhang S, Fang J, Chen M, Xu Y, Chen X (2021). Mesenchymal stem cells as a double-edged sword in tumor growth: Focusing on MSC-derived cytokines. Cellular and Molecular Biological Letters 26: 3. [Google Scholar]
Lin R, Wang S, Zhao RC (2013). Exosomes from human adipose-derived mesenchymal stem cells promote migration through Wnt signaling pathway in a breast cancer cell model. Molecular and Cellular Biochemistry 383: 13–20. [Google Scholar] [PubMed]
Liu P, Zhang Q, Mi J, Wang S, Xu Q et al. (2022). Exosomes derived from stem cells of human deciduous exfoliated teeth inhibit angiogenesis in vivo and in vitro via the transfer of miR-100-5p and miR-1246. Stem Cell Research and Therapy 13: 89. [Google Scholar] [PubMed]
Lopatina T, Bruno S, Tetta C, Kalinina N, Porta M, Camussi G (2014). Platelet-derived growth factor regulates the secretion of extracellular vesicles by adipose mesenchymal stem cells and enhances their angiogenic potential. Cell Communication and Signaling 12: 26. [Google Scholar] [PubMed]
Lou G, Song X, Yang F, Wu S, Wang J, Chen Z, Liu Y (2015). Exosomes derived from miR-122-modified adipose tissue-derived MSCs increase chemosensitivity of hepatocellular carcinoma. Journal of Hematology & Oncology 8: 122. [Google Scholar]
Luo T, Liu Q, Tan A, Duan L, Jia Y et al. (2020). Mesenchymal stem cell-secreted exosome promotes chemoresistance in breast cancer via enhancing miR-21-5p-mediated S100A6 expression. Molecular Therapy Oncolytics 19: 283–293. [Google Scholar] [PubMed]
Lyu T, Wang Y, Li D, Yang H, Qin B et al. (2021). Exosomes from BM-MSCs promote acute myeloid leukemia cell proliferation, invasion and chemoresistance via upregulation of S100A4. Experimental Hematology and Oncology 10: 24. [Google Scholar] [PubMed]
Maroto R, Zhao Y, Jamaluddin M, Popov VL, Wang H et al. (2017). Effects of storage temperature on airway exosome integrity for diagnostic and functional analyses. Journal of Extracellular Vesicles 6: 1359478. [Google Scholar]
Melzer C, Rehn V, Yang Y, Bähre H, von der Ohe J, Hass R (2019). Taxol-loaded MSC-derived exosomes provide a therapeutic vehicle to target metastatic breast cancer and other carcinoma cells. Cancers 11: 798. [Google Scholar] [PubMed]
Miloradovic D, Miloradovic D, Markovic BS, Acovic A, Harrell CR, Djonov V, Arsenijevic N, Volarevic V (2020). The effects of mesenchymal stem cells on antimelanoma immunity depend on the timing of their administration. Stem Cells International 2020: 8842659. [Google Scholar] [PubMed]
Munoz JL, Bliss SA, Greco SJ, Ramkissoon SH, Ligon KL, Rameshwar P (2013). Delivery of functional anti-miR-9 by mesenchymal stem cell-derived exosomes to glioblastoma multiforme cells conferred chemosensitivity. Molecular Therapy Nucleic Acids 2: e126. [Google Scholar] [PubMed]
Ono M, Kosaka N, Tominaga N, Yoshioka Y, Takeshita F, Takahashi RU, Yoshida M, Tsuda H, Tamura K, Ochiya T (2014). Exosomes from bone marrow mesenchymal stem cells contain a microRNA that promotes dormancy in metastatic breast cancer cells. Science Signaling 7: ra63. [Google Scholar] [PubMed]
Pakravan K, Babashah S, Sadeghizadeh M, Mowla SJ, Mossahebi-Mohammadi M, Ataei F, Dana N, Javan M (2017). MicroRNA-100 shuttled by mesenchymal stem cell-derived exosomes suppresses in vitro angiogenesis through modulating the mTOR/HIF-1α/VEGF signaling axis in breast cancer cells. Cellular Oncology 40: 457–470. [Google Scholar]
Papapetropoulos A, Fulton D, Mahboubi K, Kalb RG, O’Connor DS, Li F, Altieri DC, Sessa WC (2000). Angiopoietin-1 inhibits endothelial cell apoptosis via the Akt/survivin pathway. Journal of Biological Chemistry 275: 9102–9105. [Google Scholar] [PubMed]
Petit I, Jin D, Rafii S (2007). The SDF-1-CXCR4 signaling pathway: A molecular hub modulating neo-angiogenesis. Trends in Immunology 28: 299–307. [Google Scholar] [PubMed]
Qiu Y, Sun J, Qiu J, Chen G, Wang X, Mu Y, Li K, Wang W (2020). Antitumor activity of cabazitaxel and MSC-TRAIL derived extracellular vesicles in drug-resistant oral squamous cell carcinoma. Cancer Management Research 12: 10809–10820. [Google Scholar] [PubMed]
Ren W, Hou J, Yang C, Wang H, Wu S, Wu Y, Zhao X, Lu C (2019). Extracellular vesicles secreted by hypoxia pre-challenged mesenchymal stem cells promote non-small cell lung cancer cell growth and mobility as well as macrophage M2 polarization via miR-21-5p delivery. Journal of Experimental and Clinical Cancer Research 38: 62. [Google Scholar] [PubMed]
Rosenberger L, Ezquer M, Lillo-Vera F, Pedraza PL, Ortúzar MI et al. (2019). Stem cell exosomes inhibit angiogenesis and tumor growth of oral squamous cell carcinoma. Scientific Reports 9: 663. [Google Scholar] [PubMed]
Sahin E, Haubenwallner S, Kuttke M, Kollmann I, Halfmann A et al. (2014). Macrophage PTEN regulates expression and secretion of arginase I modulating innate and adaptive immune responses. Journal of Immunology 193: 1717–1727. [Google Scholar]
Sharif S, Ghahremani MH, Soleimani M (2018). Delivery of exogenous miR-124 to glioblastoma multiform cells by Wharton’s jelly mesenchymal stem cells decreases cell proliferation and migration, and confers chemosensitivity. Stem Cell Reviews and Reports 14: 236–246. [Google Scholar] [PubMed]
Shi S, Zhang Q, Xia Y, You B, Shan Y, Bao L, Li L, You Y, Gu Z (2016). Mesenchymal stem cell-derived exosomes facilitate nasopharyngeal carcinoma progression. American Journal of Cancer Research 6: 459–472. [Google Scholar] [PubMed]
Simpson RJ, Kalra H, Mathivanan S (2012). ExoCarta as a resource for exosomal research. Journal of Extracellular Vesicles 1: 18374. [Google Scholar]
Sohrabi B, Dayeri B, Zahedi E, Khoshbakht S, Nezamabadi Pour N, Ranjbar H, Davari Nejad A, Noureddini M, Alani B (2022). Mesenchymal stem cell (MSC)-derived exosomes as novel vehicles for delivery of miRNAs in cancer therapy. Cancer Gene Therapy 29: 1105–1116. [Google Scholar] [PubMed]
Volarevic V, Gazdic M, Simovic Markovic B, Jovicic N, Djonov V, Arsenijevic N (2017). Mesenchymal stem cell-derived factors: Immuno-modulatory effects and therapeutic potential. Biofactors 43: 633–644. [Google Scholar] [PubMed]
Wang M, Zhao C, Shi H, Zhang B, Zhang L et al. (2014). Deregulated microRNAs in gastric cancer tissue-derived mesenchymal stem cells: Novel biomarkers and a mechanism for gastric cancer. British Journal of Cancer 110: 1199–1210. [Google Scholar] [PubMed]
Wei H, Chen J, Wang S, Fu F, Zhu X, Wu C, Liu Z, Zhong G, Lin J (2019). A nanodrug consisting of doxorubicin and exosome derived from mesenchymal stem cells for osteosarcoma treatment in vitro. International Journal of Nanomedicine 14: 8603–8610. [Google Scholar] [PubMed]
Wen S, Dooner M, Cheng Y, Papa E, Del Tatto M et al. (2016). Mesenchymal stromal cell-derived extracellular vesicles rescue radiation damage to murine marrow hematopoietic cells. Leukemia 30: 2221–2231. [Google Scholar] [PubMed]
Wu H, Mu X, Liu L, Wu H, Hu X et al. (2020). Bone marrow mesenchymal stem cells-derived exosomal microRNA-193a reduces cisplatin resistance of non-small cell lung cancer cells via targeting LRRC1. Cell Death and Disease 11: 801. [Google Scholar] [PubMed]
Xiao Y, Zhang Y, Li Y, Peng N, Liu Q, Qiu D, Cho J, Borlongan CV, Yu G (2022). Exosomes derived from mesenchymal stem cells pretreated with ischemic rat heart extracts promote angiogenesis via the delivery of DMBT1. Cell Transplantation 31: 1–14. [Google Scholar]
Xu H, Han H, Song S, Yi N, Qian C et al. (2019). Exosome-transmitted PSMA3 and PSMA3-AS1 promote proteasome inhibitor resistance in multiple myeloma. Clinical Cancer Research 25: 1923–1935. [Google Scholar] [PubMed]
Yao X, Mao Y, Wu D, Zhu Y, Lu J et al. (2021). Exosomal circ_0030167 derived from BM-MSCs inhibits the invasion, migration, proliferation and stemness of pancreatic cancer cells by sponging miR-338-5p and targeting the Wif1/Wnt8/β-catenin axis. Cancer Letters 512: 38–50. [Google Scholar] [PubMed]
You WK, McDonald DM (2008). The hepatocyte growth factor/c-Met signaling pathway as a therapeutic target to inhibit angiogenesis. BMB Reports 41: 833–839. [Google Scholar] [PubMed]
Yu L, Gui S, Liu Y, Qiu X, Zhang G, Zhang X, Pan J, Fan J, Qi S, Qiu B (2019). Exosomes derived from microRNA-199a-overexpressing mesenchymal stem cells inhibit glioma progression by down-regulating AGAP2. Aging 11: 5300–5318. [Google Scholar] [PubMed]
Zhang F, Guo J, Zhang Z, Qian Y, Wang G, Duan M, Zhao H, Yang Z, Jiang X (2022). Mesenchymal stem cell-derived exosome: A tumor regulator and carrier for targeted tumor therapy. Cancer Letters 526: 29–40. [Google Scholar] [PubMed]
Zhang J, Li S, Li L, Li M, Guo C, Yao J, Mi S (2015). Exosome and exosomal microRNA: Trafficking, sorting, and function. Genomics, Proteomics & Bioinformatics 13: 17–24. [Google Scholar]
Zhang F, Lu Y, Wang M, Zhu J, Li J, Zhang P, Yuan Y, Zhu F (2020a). Exosomes derived from human bone marrow mesenchymal stem cells transfer miR-222-3p to suppress acute myeloid leukemia cell proliferation by targeting IRF2/INPP4B. Molecular and Cellular Probes 51: 101513. [Google Scholar] [PubMed]
Zhang X, Yang Y, Yang Y, Chen H, Tu H, Li J (2020b). Exosomes from bone marrow microenvironment-derived mesenchymal stem cells affect CML cells growth and promote drug resistance to tyrosine kinase inhibitors. Stem Cells International 2020: 8890201. [Google Scholar] [PubMed]
Zhou Y, Day A, Haykal S, Keating A, Waddell TK (2013). Mesenchymal stromal cells augment CD4+ and CD8+ T-cell proliferation through a CCL2 pathway. Cytotherapy 15: 1195–1207. [Google Scholar] [PubMed]
Zuo R, Liu M, Wang Y, Li J, Wang W et al. (2019). BM-MSC-derived exosomes alleviate radiation-induced bone loss by restoring the function of recipient BM-MSCs and activating Wnt/β-catenin signaling. Stem Cell Research and Therapy 10: 30. [Google Scholar] [PubMed]
Cite This Article
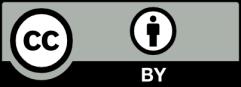